- Science and Technology on Reactor System Design Technology Laboratory, Nuclear Power Institute of China, Chengdu, China
The design of high-performance lead-based fast reactors (LFRs) has become a hotspot in the advanced reactor system. This study evaluates the neutronics performance improvement based on the small LFR SLBR-50. Parameter sensitivity analyses are conducted, including height-to-diameter ratio (H/D), reflector assembly arrangement, and pitch-to-diameter ratio in fuel assembly, fuel material, and fuel enrichment partitioning. Numerical results of eigenvalue in burnup procedure, assembly power distribution, and energy spectrum are analyzed using the Monte-Carlo code RMC. Our findings indicate that the fuel material, fuel partitioning, and H/D are the three most important factors in LFR design. The neutronics performance analyses will assist in LFR design.
Introduction
Lead-based fast reactor (LFR) (Cinotti, 2009) is one of the six most promising advanced reactor systems among the fourth-generation nuclear reactors (U.S. Doe Nuclear Energy Research Advisory Committee and the Generation IV International Forum, 2002; Cinotti et al., 2010; Zhang et al., 2020a; Zhang et al., 2020b). The lead-based coolant shows good neutronics characteristic in less neutron absorption and moderation, thereby leading to harder neutron spectrum, closed fuel cycle, long-life core without refueling, and better neutron economy. Likewise, the lead-based coolant has better chemical inertness and thermal conductivity. Considering these characteristics, LFR shows good advantages in safety, sustainability, and efficient conversion of uranium.
The idea of LFR was originally proposed for submarine propulsion in 1950s in Russia (Zhang et al., 2020b). The LFR was applied on the nuclear submarine in 1963 (Fazio, 2007), and corrosion behavior was studied for controlling of low oxygen concentrations (Kurata et al., 2008). After the proposition of the fourth-generation nuclear reactor, studies and developments of the lead-based nuclear reactor were conducted. In Russia, SVBR-100 (Zrodnikov et al., 2006; Zrodnikov et al., 2011) and BREST-300 (Smimov, 2012) were designed for power generation. In Europe, a lead-based reactor was rapidly developed under the framework of science project. In addition, a small modular lead-based reactor, SEALER (Wallenius et al., 2018; LeadCold Reactors Company, 2022), was designed by Swedish LeadCold Company for polar region application. Furthermore, an experimental reactor MYRRHA (Abderrahim and D’hondt, 2007), demonstration reactor ALFRED (Frogheri, 2013; Alemberti, 2020), and prototype reactor ELFR (Frogheri, 2013) have been under research and development. In the United States, a small transportable modular lead-cooled reactor SSTAR (Sienicki, 2005; Smith et al., 2012) was conceptually designed with the cooperation of ANL and LLNL. In Asia, several lead-cooled reactors were designed, such as LSPR (Sekimoto, 2001) and CLEAR-SR (Wang et al., 2015a). In general, the LFR attracted great attention after the proposal of a fourth-generation nuclear reactor system. In addition, large sums of conceptual designs were proposed with different reactor parameters. In conceptual design of the lead-based reactor, the refueling cycle was extended to over 5 years. Several designs, such as the SSTAR reactor, cancelled the refueling procedure in full cycle.
In this study in reactor physics, parameter sensitivity analyses were conducted based on a small LFR SLBR-50 (small lead-based breeding reactor), which was conceptually designed in the Nuclear Power Institute of China for scientific research. Several important designing parameters were analyzed using the control-variates method, such as the core height-to-diameter ratio (H/D), reflector assembly arrangement, pin pitch-to-diameter ratio (P/D) in fuel assembly, fuel material, and fuel enrichment partitioning. In theory, these parameters have a great influence on the neutronics design. In the control-variates method, only one parameter was changed and analyzed. The detailed influences will be analyzed in quantity, and the most important design parameter will be pointed out. In this way, compared with the original reactor design, neutronics performance will be improved with sensitivity analyses and more reasonable design parameters.
In the neutronics design, the Monte-Carlo code RMC (She, 2012; Wang et al., 2015b; Liu et al., 2017) was adopted for critical and burnup calculation with large-scale parallel ability. ENDF/B-VII.0 (Steven, 2006) data library and ACE format cross-section data were applied. In critical transport calculation, the free gas model was built for elastic scatter cross-section, and probability tables were used for unresolved resonance calculation. In burnup calculation, detailed depletion chains containing thousands of isotopes and CRAM (Zhou et al., 2018) depletion method were adopted. Moreover, source convergence acceleration and large-scale parallel methods were applied.
Original design of the SLBR-50 reactor
Table 1 reports the original parameters of the SLBR-50 reactor. The thermal power was 50 MW. The UO2 fuel enrichment was 19.75%, and the total weight of uranium was 3,835.3 kg. Geometry parameters are presented in the right column of Table 1. Basic hexagonal assembly and whole-core models of SLBR-50 are presented in Figures 1 and 2. The radius for fuel, gap, and clad was 0.4, 0.41, and 0.47 cm, respectively, in each fuel rod. The assembly pitch was 9.35 cm and the box thickness was 0.2 cm for the fuel assembly. In the whole core, 144 fuel, 18 control rod, and 48 reflector assemblies were arranged.
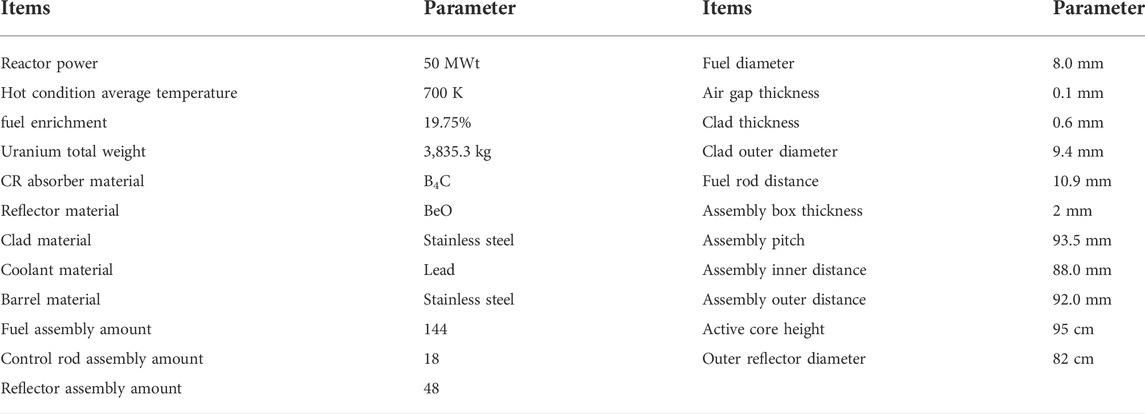
TABLE 1. Original parameters of the SLBR-50 reactor (Zhao et al., 2021).
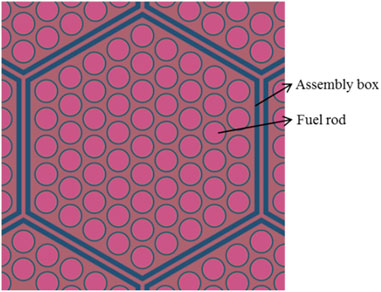
FIGURE 1. Hexagonal assembly geometry of the SLBR-50 reactor (Zhou et al., 2018).
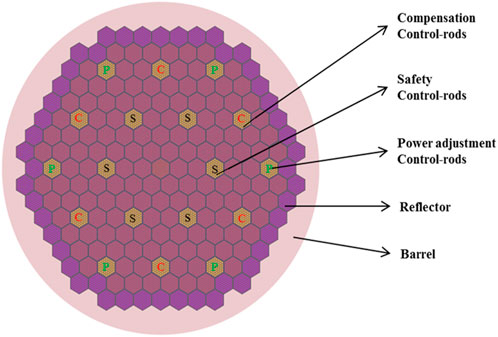
FIGURE 2. Whole-core model of the original design in the SLBR-50 reactor (Zhou et al., 2018).
Parameter sensitivity analyses for neutronics performance improvement
In this section, parameter sensitivity analyses were conducted with the fixed-variable method based on the original scheme of the SLBR-50 reactor. Basic parameters were set to be consistent with the original design of SLBR-50, including the reactor power, fuel enrichment, and pin-cell geometry construction. Other important neutronics results were also analyzed in the research, including fuel weight, power peak factor, and energy spectrum. To simplify the fuel weight analyses, it was converted to the comparison of eigenvalue in burnup procedure in certain fuel weight. Key factors for the parameter sensitivity research and performance improvement included the H/D, the reflector assembly arrangement, the P/D in fuel assembly, fuel material, and fuel enrichment partitioning.
As for the calculation condition, it remained same in analyses. A total of 800,000,000 active particles were used (800 generations comprising 100,000 neutrons per generation, of which 200 generations were skipped). The standard deviation of eigenvalue result was 7 pcm, which is reasonable in design calculation.
Height/diameter
Core H/D is one of the important parameters for research, and it has great influence on neutron leakage. In particular, the neutron leakage is more significant in fast reactors with longer neutron free path. To apply the control-variates method in the research of H/D, uranium weight, fuel enrichment, fuel assembly structure, and barrel thickness were set to be consistent. The active core equivalent diameter was calculated from the number of fuel assemblies, and the active core height was adjusted considering the total uranium weight. Furthermore, radial reflectors were neglected in the H/D analysis. Calculation conditions and part of results are listed in Table 2. As shown, the fuel enrichment and barrel thickness were set to 19.75% and 20 cm in all cases, respectively. Radial fuel assembly amount and axial fuel assembly height were adjusted to make the total fuel weight almost consistent in 3,834–3,840 kg. In this way, H/D was verified to range from 0.73 to 1.31 in five cases. Radial cut of the active core of these five cases is presented in Figure 3.
Eigenvalue results of the burnup procedure are presented in Table 2. The eigenvalue result was the largest in case 3 with a H/D close to 1.0, where the neutron leakage could be fully decreased. It can be concluded that the H/D parameter should be close to 1.0 in the reactor physics design, and it shows a great effect on neutron leakage reduction.
Reflector
The reflector outside of the active core is the second research parameter. It is also an effective way to improve the neutron reaction rate and reduce the neutron leakage rate. The reflector assembly amount and material need to be analyzed based on case 3 (H/D = 1.03) in the H/D research.
Similar to the H/D research, the fixed-variable method was conducted in the reflector analysis. First, the reflector assembly amount was studied on three cases, as shown in Figure 4. In these three cases, the only difference lied in the amount of reflector assemblies. BeO was conducted as the reflector material in the reflector amount analysis. The reflector barrel was modeled explicitly outside the reactor active core.
Eigenvalue and assembly power peak results are presented in Table 3. Reflector assemblies showed good performance in terms of decreasing neutron leakage. The eigenvalue results increased from 1.02989 to 1.04865 with 42 reflector assemblies. The radial assembly power peak also improved, from 1.499 to 1.323 and 1.310. The detailed radial power distribution of beginning of life (BOL) is presented in Figure 5. These results show that the radial reflector has good performance in eigenvalue improvement, and 42 reflector assemblies were enough. The power flattening and assembly power peak decrease were due to the higher reaction rate of 235U near the reflector assembly, which was caused by the obvious thermalization phenomenon of BeO. The thermalization phenomenon of the BeO reactor can be found in the subsequent reflector material analysis.
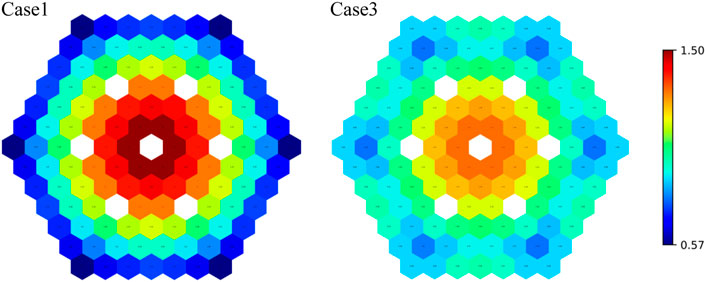
FIGURE 5. Assembly power distribution results on the beginning of life of the reflector amount analysis.
Moreover, reflector material was studied with 42 reflector assemblies. According to reference previous study (Liu et al., 2017), Al2O3, BeO, MgO, PbO, and ZrO2 can be applied as the reflector material in LFR. Eigenvalue burnup calculation results are presented in Figure 6, where the EFPD was effective full power days. BOL eigenvalues are listed in Table 4. BeO showed the best performance of eigenvalue results. The eigenvalue result was 1.04885 on the BOL with the BeO reflector, which was 1,366 pcm larger than the PbO reflector.
Further, the energy spectrum and assembly power distribution results on the BOL were analyzed. The energy spectrum was tallied and integrated from 0.1 eV to 1.0 MeV with 28 sectors. The core-averaged energy spectrum results for different reflector materials are shown in Figure 7. The energy spectrum showed the highest distribution in the fast energy range of 104 eV–106 eV for the PbO material. In the thermal energy range of 1–1000 eV, flux was the highest for the BeO material. These results showed that the BeO reflector material had the best moderation ability. Thermalization of energy spectrum occurred, and the reaction rate was improved with the BeO reflector. In the assembly power distribution results of the BeO reflector, in Figure 8 thermalization phenomenon was also observed. The minimum assembly power peak was in the inner assembly and the outmost fuel assembly showed larger power because of the strong moderation ability of the BeO reflector. Thus, it can be concluded that the reflector material has great influence on the reactor energy spectrum and has further effect on the reaction rate. Energy spectrum is thus an important factor for the research.
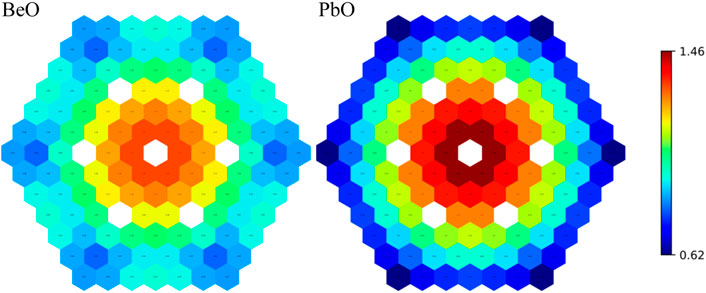
FIGURE 8. Assembly power distribution results on the beginning of life of the reflector material analysis.
Pitch/diameter
The third parameter is in the P/D in the fuel assembly. The P/D parameter influences the scattering and absorbing of coolant, which affects the neutron absorption and leakage. In the P/D parameter research, 42 BeO reflector assemblies were adopted considering the better neutronics performance in reflector analyses. Based on the original SLBR-50 design, three cases were analyzed with different P/D parameters, including 1.16 (case 1), 1.12 (case 2), and 1.06 (case 3). The detailed geometry parameters are shown in Table 5. As for fuel rods, fuel rod diameters remained the same and the pitch was adjusted from 1.09 to 1.00 cm. As for fuel assemblies, the assembly size was changed to maintain equal distance between the outmost fuel rod and assembly box in the three cases. In this way, fuel weight can be consistent in the P/D analysis. The fuel assemblies of these cases are shown in Figure 9. In assembly figures, the decrease in assembly size was too small to be captured. In whole-core figures, the size of the reactor core was reduced as the P/D was decreasing.
Eigenvalue results in the burnup procedure are presented in Figure 10. Case 3 with the smallest P/D showed the best eigenvalue performance. Eigenvalue results of the three cases were 1.04865, 1.06191, and 1.07866, respectively, in BOL. In addition, energy spectrum distributions are presented in Figure 11. The energy spectrum of case 3 was the softest, and the energy spectrum distributions of the three cases showed minor differences compared to reflector material analyses. The assembly power distribution results on BOL are presented in Figure 12. The radial assembly power peak factors were 1.32, 1.31, and 1.30 for the three cases, respectively, which also had unobvious differences.
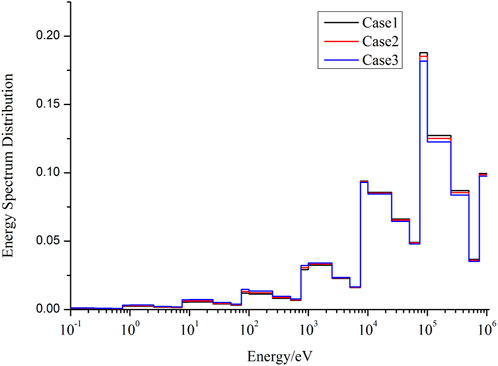
FIGURE 11. Energy spectrum results on the beginning of life of the pitch-to-diameter ratio analysis.
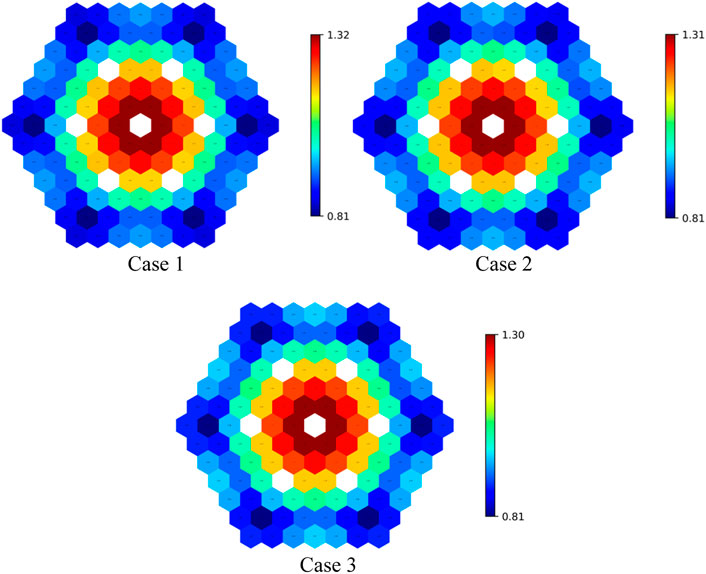
FIGURE 12. Assembly power distribution results on the beginning of life of the pitch-to-diameter ratio analysis.
Based on sensitivity analyses, the P/D parameter had great influence on eigenvalue results. The eigenvalue differences in the BOL can extend to 3,000 pcm in sensitivity analyses. The differences were mainly caused by the neutron absorption for the lead coolant and neutron fission for fuel rods. Decreasing P/D was an effective way to improve eigenvalue and decrease fuel weight. However, the P/D parameter cannot be so small considering the heat exchange capability in thermal-hydraulics analyses.
Fuel material
Fuel material is an important factor in the research. The neutron fission absorbing and scattering are affected by fuel materials. In this study, traditional UO2, new-type UN, and U-Zr alloy fuel materials were analyzed. In the fuel material research, the same fuel assembly and core structure were applied, as shown in Figures 9A,D. Fuel enrichments were adjusted to realize the similar burnup depth with the same reactor geometry design and reactor power. Detailed calculation conditions of the fuel material analysis are listed in Table 6. In particular, fuel densities and uranium weights were significantly different in the analysis. In fuel material analyses, all parameters remained the same, except for fuel material and fuel enrichment.
Eigenvalue results of the fuel material analysis in the burnup procedure are presented in Figure 13. The decrease of eigenvalue results along the burnup procedure is the most obvious for the traditional UO2 material. The U-Zr material showed the best burnup performance according to the eigenvalue results. The phenomenon was conducted by the different energy spectrum performance of these fuel materials, which is presented in Figure 14. New-type U-Zr and UN materials showed greater energy distribution on fast energy > 104 eV. The energy spectrum was harder for the new-type fuel material than traditional UO2 material. Therefore, more conversion and breeding reaction occurred, and the burnup depth can be extended for new-type materials. Likewise, the assembly power distributions are compared and shown in Figure 15. According to assembly power results, the assembly power peak for these three fuel materials were 1.32, 1.35, and 1.33, respectively. Fuel materials had little influence on the power distribution.
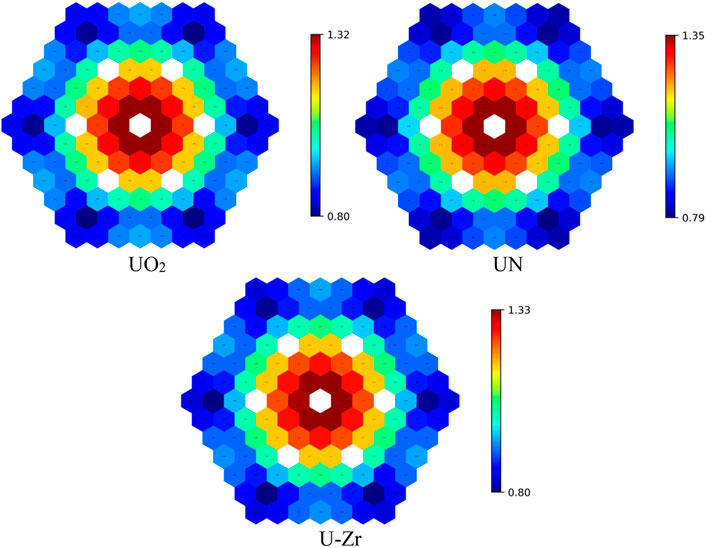
FIGURE 15. Assembly power distribution results on the beginning of life of the fuel material analysis.
Fuel partitioning
The power flattening is an important parameter in neutronics performance analyses. The most straightforward way to improve power flattening performance is in the fuel partitioning with different enrichment. In this study, the fuel partitioning analyses were based on the U-Zr fuel material. Three kinds of fuel with different levels of enrichment were employed, such as 16%, 14%, and 12%. In this way, the fuel partitioning was analyzed using two patterns, including a low-leakage pattern (high enrichment–fuel inner layout) and high-leakage pattern (high enrichment–fuel outer layout). Furthermore, core loading without partitioning was analyzed as the control group. The core configuration of fuel partitioning is shown in Figure 16.
Assembly power distributions on the BOL are presented in Figure 17. The power peak factor increased from 1.32 to 1.71 in the low-leakage pattern. In contrast, the high-leakage pattern showed a great effect on power flattening, and the power peak factor can be decreased to 1.07. In the high-leakage pattern, higher enrichment–fuel assemblies were located at the edge of the active core to improve the fission reaction rate and realize power flattening.
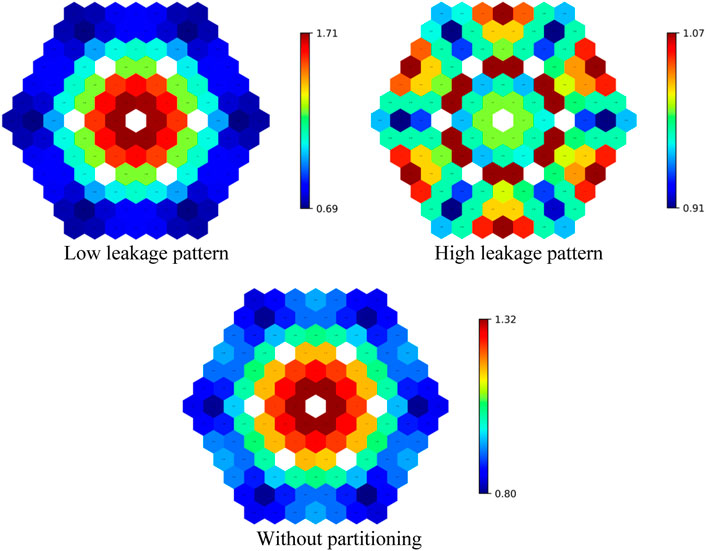
FIGURE 17. Assembly power distribution results on the beginning of life of the radial power flattening analysis.
Conclusion
LFRs show several merits in the advanced reactor systems and have already become a research hotspot in the design of LFR. In this study, parameter sensitivity analyses of neutronics performance improvement were conducted based on a 50-MWt small lead-based reactor (SLBR-50), which was conceptually designed for research.
The research of neutronics performance improvement was conducted with the fixed-variable method in performance improvement. Five key parameters were analyzed, including H/D, reflector assembly arrangement, P/D in fuel assembly, fuel material, and fuel partitioning. As for neutronics results, eigenvalue, assembly power distribution, and energy spectrum were analyzed.
According to parameter sensitivity analyses, it can be concluded that parameters optimization show good effect on decreasing neutron leakage, energy spectrum thermalization, and power flattening, which improves the neutronics performance. As for these parameters, fuel material, fuel partitioning, and H/D are the three most important factors.
In the future, a high-performance lead-based reactor will be designed based on the parameter research.
Data availability statement
The original contributions presented in the study are included in the article/Supplementary Material; further inquiries can be directed to the corresponding author.
Author contributions
CZ: Main researcher and manuscript writer, LL: Researcher, BZ: Researcher, BZ: Researcher, LW: Advisor and director, XP: Advisor and director.
Funding
This work is supported by the National Natural Science Foundation of China (Grant No. 12005215) and China Association for Science and Technology (Young Elite Scientists Sponsorship Program 2019QNRC001).
Conflict of interest
The authors declare that the research was conducted in the absence of any commercial or financial relationships that could be construed as a potential conflict of interest.
Publisher’s note
All claims expressed in this article are solely those of the authors and do not necessarily represent those of their affiliated organizations or those of the publisher, the editors, and the reviewers. Any product that may be evaluated in this article or claim that may be made by its manufacturer is not guaranteed or endorsed by the publisher.
References
Abderrahim, H., and D'hondt, P. (2007). Myrrha: a European experimental ads for R&D applications status at mid-2005 and prospective towards implementation. J. Nucl. Sci. Technol. 44, 491–498. doi:10.1080/18811248.2007.9711313
Alemberti, A. (20202012). The ALFRED project on lead-cooled fast reactor. ESNII Conf. Adv. fission Res. Horizon 25.
Cinotti, L. (2009). Lead-cooled fast reactor (LFR) overview and perspectives Livermore, CA: Lawrence Livermore National Lab (LLNL). LLNL-CONF-414708.
Fazio, C. (2007). “Handbook on lead-bismuth eutectic alloy and lead properties[R].” in Materials compatibility, thermal-hydraulics and technologies. Edition. Nuclear science.
Frogheri, M. (2013). The lead fast reactor: Demonstrator (ALFRED) and ELFR design.” in International conference on fast reactors and related fuel cycles. Paris, France.
Kurata, Y., Futakawa, M., and Saito, S. (2008). Corrosion behavior of steels in liquid lead-bismuth with low oxygen concentrations. J. Nucl. Mater. 373, 164–178. doi:10.1016/j.jnucmat.2007.05.051
LeadCold Reactors Company (2022). Available at: http://www.leadcold.com/sealer.html.
Liu, S., Liang, J., Wu, Q., Guo, J., Huang, S., Tang, X., et al. (2017). BEAVRS full core burnup calculation in hot full power condition by RMC code. Ann. Nucl. Energy 101, 434–446. doi:10.1016/j.anucene.2016.11.033
Sekimoto, H. (2001). “A long-life small reactor for developing countriesInternational seminar on status and prospects for small and medium sized reactors,” in International Seminar on Status and Prospects for Small and Medium Sized Reactors, Cairo, Egypt.
She, D. (2012). Using generalized laguerre polynomials to compute the matrix exponential in burnup equations. Knoxville, Tennessee, USA: PHYSOR.
Sienicki, J. (2005). SSTAR lead-cooled, small modular fast reactor with nitride fuel. Workshop on advanced reactors with innovative fuels ARWIF-2005, NEA, 16-18. Oak Ridge.
Smimov, V. (2012). “Lead-cooled fast reactor BREST-Project status and prospects[R],” in Nternational workshop on innovative nuclear reactors cooled by heavy liquid metals: status and perspectives Pisa
Smith, F., Halsey, W. G., Brown, N. W., Sienicki, J. J., Moisseytsev, A., and Wade, D. C. (2012). Sstar: The US lead-cooled fast reactor(LFR). J. Nucl. Mater. 376 (3), 255–259. doi:10.1016/j.jnucmat.2008.02.049
Steven, C. (2006). Benchmarking ENDF/B-VII.0. Nucl. Data Sheets 107, 3061–3118. doi:10.1016/j.nds.2006.11.002
U.S. Doe Nuclear Energy Research Advisory Committee and the Generation IV International Forum, (2002). A Technology roadmap for generation IV nuclear energy systems. Technical report GIF-002-00.
Wallenius, J., Qvist, S., Mickus, I., Bortot, S., Szakalos, P., and Ejenstam, J. (2018). Design of SEALER, a very small lead-cooled reactor for commercial power production in off-grid applications. Nucl. Eng. Des. 338, 23–33. doi:10.1016/j.nucengdes.2018.07.031
Wang, K., Li, Z., She, D., Liang, J., Xu, Q., Qiu, Y., et al. (2015). Rmc – a Monte Carlo code for reactor core analysis. Ann. Nucl. Energy 82, 121–129. doi:10.1016/j.anucene.2014.08.048
Wang, M., Lian, C., Li, Y., Wang, D., Jiang, J., and Wu, Y. (2015). Preliminary conceptual design of a lead-bismuth cooled small reactor (CLEAR-SR). Int. J. Hydrogen Energy 40, 15132–15136. doi:10.1016/j.ijhydene.2015.03.097
Zhang, T., Liu, X., Xiong, J., and Cheng, X. (2020). Comparisons of reduced moderation small modular reactors with heavy water coolant. Front. Energy Res. 8, 1–5. doi:10.3389/fenrg.2020.00027
Zhang, T., Xiong, J., Liu, X., Chai, X., Li, W., and Cheng, X. (2020). Conceptual design of an innovative reduced moderation thorium-fueled small modular reactor with heavy-water coolant. Int. J. Energy Res. 43, er.4827. doi:10.1002/er.4827
Zhao, C., Lou, L., Peng, X., Zhang, B., and Wang, L. (2021). Application of the spectral-shift effect in the small lead-based reactor SLBR-50. Front. Energy Res. 9, 756106. doi:10.3389/fenrg.2021.756106
Zhou, S., Chen, R., Shao, Y., Cao, L., Bai, B., and Wu, H. (2018). Conceptual core design study of an innovative small transportable lead-bismuth cooled fast reactor (SPARK) for remote power supply. Int. J. Energy Res. 42, 3672–3687. doi:10.1002/er.4119
Zrodnikov, A., Toshinsky, G., Komlev, O., Dragunov, Y., Stepanov, V., Klimov, N., et al. (2006). Nuclear power development in market conditions with use of multi-purpose modular fast reactors SVBR-75/100. Nucl. Eng. Des. 236, 1490–1502. doi:10.1016/j.nucengdes.2006.04.005
Keywords: neutronics performance, small lead-based fast reactor, SLBR-50, RMC, energy spectrum
Citation: Zhao C, Lou L, Zhang B, Zhou B, Wang L and Peng X (2022) Neutronics performance improvement based on the small lead-based fast reactor SLBR-50. Front. Energy Res. 10:982101. doi: 10.3389/fenrg.2022.982101
Received: 30 June 2022; Accepted: 13 July 2022;
Published: 24 August 2022.
Edited by:
Jiankai Yu, Massachusetts Institute of Technology, United StatesReviewed by:
Zhaoyuan Liu, Qilu University of Technology (Shandong Academy of Sciences), ChinaMohammad Alrwashdeh, Khalifa University, United Arab Emirates
Copyright © 2022 Zhao, Lou, Zhang, Zhou, Wang and Peng. This is an open-access article distributed under the terms of the Creative Commons Attribution License (CC BY). The use, distribution or reproduction in other forums is permitted, provided the original author(s) and the copyright owner(s) are credited and that the original publication in this journal is cited, in accordance with accepted academic practice. No use, distribution or reproduction is permitted which does not comply with these terms.
*Correspondence: Lianjie Wang, d2FuZ2xpYW5qaWVAdHNpbmdodWEub3JnLmNu; Xingjie Peng, cGVuZ3hpbmdqaWV0c0AxMjYuY29t