- 1School of Safety Engineering, China University of Mining and Technology, Xuzhou, China
- 2School of Resources and Safety Engineering, Henan University of Engineering, Zhengzhou, China
- 3State Key Laboratory Cultivation Base for Gas Geology and Gas Control, Henan Polytechnic University, Jiaozuo, China
- 4College of Resources and Environment, Henan University of Economics and Law, Zhengzhou, China
Permeability is an important parameter in the process of coalbed methane exploitation. To improve the production efficiency of coalbed methane and explore the control mechanism of the gas flow law in coal, the permeability of helium and nitrogen in the same coal sample was tested under different effective stress (the difference between external stress and pore pressure of coal mass) and pressure by using the seepage device. Based on the gas flow theory, the interaction mechanism of effective stress, adsorption effect and Klinkenberg effect in controlling the permeability has been analyzed. Increasing the gas pressure will enhance the adsorption and deformation ability of coal, causing the reduction of pore size, while it will also cause the reduction of effective stress and stress deformation. There is a certain competition between them under the same external stress condition, which will lead to the change of pore and then affect the permeability of coal seam. The Klinkenberg effect will lead to more complex change factors of permeability, especially in laboratory experiments. Both adsorption deformation and stress deformation will affect the pore structure of coal body, which will also lead to changes in the influence degree of Klinkenberg effect on apparent permeability. Under the influence of adsorption effect, the Klinkenberg effect may be a variable. The experimental results in this work elaborate the microscopic control mechanism of gas permeability change in coal. It can not only provide important guidance for gas injection technology, but also enrich the theory of coal seam gas flow.
Introduction
In the process of coal mining, a lot of methane is discharged into the atmosphere casually, however, the greenhouse effect caused by methane is 20 times stronger than carbon dioxide, and long-term random discharge of methane causes serious greenhouse effect (Hou et al., 2022; Li et al., 2022; Xue et al., 2022). On the other hand, methane is a kind of clean energy. As a result, it is particularly important to improve the exploitation of coalbed methane (CBM), which not only mitigates greenhouse effect but also improves energy efficiency (Ji et al., 2020; Liu et al., 2020; Si et al., 2021; Zhou et al., 2019). Coal permeability is an important index for CBM extraction (Pan and Connell 2012; Wei et al., 2021; Xiao et al., 2021; Zhou et al., 2021). It is therefore of great significance to explore the seepage characteristics of gas in coal.
Coal is a special reservoir with dual pore structure (Wang et al., 2021; Wang et al., 2022). Gas flow in coal is closely related to pore structure. Meanwhile, changes in stress and gas pressure will significantly affect permeability of coal (White et al., 2005; Si et al., 2018). The change of gas pressure not only leads to the change of adsorbed gas quantity of coal body, but also causes the change of coal matrix body, which then effects the width of cracks in coal and causes the change of permeability of coal body. On the other hand, under the influence of effective stress, the change of stress will also lead to the change of elastic strain variable of coal, which will also affect the width of coal crack and permeability (Singh and Javadpour 2016). Because of such complicated deformation characteristics of coal, the permeability value is an important variate during the process of coalbed methane exploitation. As a result, it is particularly important to explore the variation law of coal permeability for the prediction of coalbed methane production (Levine and J. 1996; Towler et al., 2016; Rathnayake et al., 2022).
In order to further deeply analyze the flow characteristics of gas in coal, it is critical to clarify the influence of coal stress deformation, adsorption deformation and Klinkenberg effect on permeability. In the aspect of the influence of coal stress deformation, Somerton et al. (1975) analyzed the relationship between average stress and coal permeability through experiments and obtained an empirical fitting equation. Gray (1997) reconstructed the permeability equation of coal based on the horizontal effective stress, considering the matrix expansion factor. Seidle and Huitt (1995) assumed that the coal was a matchstick model, constructed the coal permeability model and considered that the coal permeability decreased with the increase of effective stress Somerton et al. (1975). Selected three bituminous uranium and analyzed the flow characteristics of gas in coal under axial and radial stress conditions, determining the functional relationship between the permeability of coal fissure and applied stress Zhang et al. (2008). Analyzed coal stress deformation and adsorption deformation by theoretical modeling, established FE model of permeability, verified the experimental data, and discussed the control law of coal deformation on permeability in detail. Mitra et al. (2012) have discussed the changes of coal permeability under uniaxial strain field in the laboratory, and proposed that the increase of coal permeability under low pressure is due to matrix Shrinkage effect.
Coal is a kind of rock mass, which conforms to certain elastic mechanical properties. The stress change will lead to deformation of coal structure. The change of pore size of gas flow will lead to the variation of permeability. During deep coal seam mining, the stress will be redistributed under the influence of mining, which will also lead to the change of coal permeability. In early studies, stress variation was also considered as the main factor affecting permeability, and relevant theoretical equations were established. However, with the deepening of experimental research, researchers gradually realized that stress deformation alone could not explain the permeability changes of some high pressure or different gases. With the improvement of testing technology, it is observed that coal is prone to deformation in the process of gas adsorption, especially under high pressure. This phenomenon cannot be ignored and will also have a significant impact on permeability.
In terms of the influence of adsorption deformation, Mitra et al. (2012) researched the sorption-induced volumetric strain caused by coal adsorbing gas with experimental methods, and verified and improved the permeability model on this basis. Perera et al. (2013) developed a state-of-the-art core flooding apparatus to verify the influence of expansion factors caused by the adsorption of carbon dioxide on coal permeability. Based on matrix-fractures, Jianhua et al. (2021) improved the adsorption deformation equation, established a permeability model of coal, and discussed the influence of adsorption-induced deformation on coal permeability. Lin and Kovscek (2014) used experimental methods to analyze the flow characteristics of gas in coal, tested the permeability of nitrogen, carbon dioxide and mixed gas, and analyzed the influence characteristics of adsorption deformation on permeability of coal in detail.
Adsorption deformation is the special property of coal body, because of the adsorbability of coal, especially for methane and carbon dioxide gas has extremely significant adsorption effect. After adsorbing gas, the expansion of coal matrix leads to the reduction of coal pore size, which is easy to cause the decrease of gas permeability, which is very obvious in carbon dioxide injection into coal seam. On this basis, researchers have a more and more clear understanding of gas flow in coal seam, and a relevant theoretical model was established to explain the experimental results. The flow phenomenon of gas in tiny pores is significantly different from that of liquid. The Klinkenberg effect is a special phenomenon of gas flow, especially in the state of low pressure, and this effect will be very significant. The coal seam is a low permeability reservoir, and the methane gas pressure in the coal seam is generally low, so the Klinkenberg effect cannot be ignored in the theoretical study of coal seam gas flow. Therefore, researchers take the Klinkenberg effect into account and further modify the current permeability model in order to have a clearer understanding of the gas flow law in coal seam.
Under the condition of low gas pressure, the Klinkenberg effect of gas flow in coal cannot be ignored. According to the deformation characteristics of coal matrix, Wang et al. (2014) established the Klinkenberg effect model towards the change of crack width, and proposed that the Klinkenberg coefficient of gas flow in coal is a variation. Through theoretical analysis, Moghadam and Chalaturnyk (2014) also proposed that the Klinkenberg coefficient of gas flow changes with gas pressure, and established a new model, which enabled be used to verify the field permeability data. In the aspect of the influence of Klinkenberg effect, Zhu et al. (2007) verified the gas permeability equation with Klinkenberg effect using finite element method, and considered that Klinkenberg effect has an important contribution to gas flow in coal. El Amin Mohamed and El Beltagy Mohamed (2021) established a random Klinkenberg slip coefficient model with theoretical method, and analyzed the role of this model in apparent permeability, indicating that this model was suitable for low permeability reservoirs. The factors affecting the gas flow in coal are complex, and it is difficult to explain the change mechanism of coal permeability by a single theoretical method (Zhen et al., 2021). The variation of coal permeability is affected by pore deformation, which is also the key factor to establish permeability model. Therefore, scholars have established classical SD model and PM model based on effective stress deformation and adsorption deformation. These theoretical models have a strong guiding role in laboratory and industrial experiment data (Shi and Durucan 2004). Zhou et al. (2020) constructed a theoretical model of coal permeability based on the changes of coal matrix body, obtaining the variation characteristics of Klinkenberg effect and Knudsen number during the process of gas flow in coal, which accurately predicted coal permeability. These results laid a theoretical foundation for coalbed methane exploitation. Zhou et al. (2016) established a theoretical permeability model coupled with effective stress, adsorption deformation and Klinkenberg effect, which has a higher fitting degree than the conventional model.
There are many complex factors affecting gas flow in coal, and it is impossible to take all of them into account in a single study. Therefore, studies are conducted with one or both factors as the object. A large number of articles taken pore deformation as the research object, discussed the change of gas flow by considering the pore size variation caused by stress and adsorption, and predicted and verified the relationship between mathematical model and actual gas permeability through experiments, numerical simulation and theoretical calculation. These conclusions are of great significance to understand the law of gas flow in coal and help engineers to understand the influence mechanism of stress and other factors on coal permeability. Coal is a low-permeability reservoir, the Klinkenberg effect is an important part of gas flow in coal, which has a significant impact on the prediction of coalbed methane production. With the increasing popularity of CBM exploitation, a large number of researchers gradually focus on the influence of Klinkenberg effect on gas permeability, especially in China. The Klinkenberg effect is a special property in gas flow and is affected by many factors. Although a large number of experiments have been conducted on this effect, there are not enough experiments to quantify its effect on permeability. The adsorption capacity of different gases on coal is clearly different, and different gas pressure will also lead to the variation of effective stress. Stress and gas adsorption will not only affect the permeability of coal, but also lead to the change of Klinkenberg effect. The permeability change law of different gases under different effective stresses is studied by experimental method, and then the contribution of Klinkenberg effect, stress and gas adsorption to permeability is analyzed in detail, which is helpful to understand the change mechanism of gas flow process in coal.
The permeability of gas in coal is mainly controlled by stress, gas adsorption and content (Banerjee et al., 2021), Klinkenberg effect, the fracture and cleats (Banerjee et al., 2022). Although a large number of experimental studies have been done on permeability of coal, the quantitative experiments are lacking to reveal the relationship between adsorption effect and Klinkenberg effect in permeability measurement. Herein, the permeability of helium gas and nitrogen gas in the same coal sample under different effective stress and gas pressure has been tested by seepage device, and the influence of effective stress and gas pressure on permeability change was expounded. Meanwhile, based on the theoretical model of Klinkenberg effect, the effect of effective stress on Klinkenberg coefficient was compared. Moreover, apparent permeability was decomposed, and the contribution degree and control law of adsorption effect and Klinkenberg effect on apparent permeability were quantified. Based on the experiment, this paper could intuitively show the influence of various factors on permeability, which was also suitable for the actual situation of coalbed methane mining. This work will provide theoretical guidance for coalbed methane exploitation.
Experimental methods and theoretical analysis
Samples and experimental devices
To compare the difference of various gas permeability, the permeability of nitrogen and helium was tested with single coal sample under the same conditions. The coal samples were taken from Qincheng Coal Mine in Shanxi Province, and the standard samples of 50*100 mm were drilled after large raw coal were taken out from underground. The coal samples in this area are anthracite. Qincheng is rich in CBM reserves and is the most favorable area for CBM resource development in China. The triaxial seepage device is adopted in the seepage experiment (Figure 1), which enables to accuratly control the confining and axial pressures and meet the seepage experiment requirements for various gases.
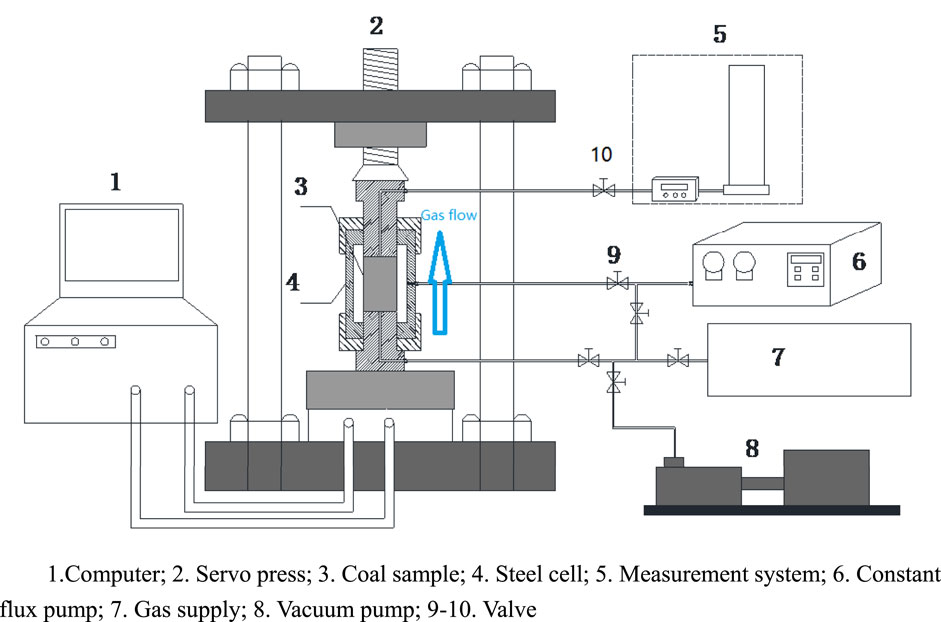
FIGURE 1. Schematic of coal permeability experimental apparatus. 1.Computer; 2. Servo press; 3. Coal sample; 4. Steel cell; 5. Measurement system; 6. Constant flux pump; 7. Gas supply; 8. Vacuum pump; 9-10. Valve.
Experimental methods
For gas seepage experiment, the axial pressure and confining pressure of coal sample gripper are controlled by the device, and the effective stress of coal body is kept fixed in combination with gas pressure. The seepage law of gas in coal under constant effective stress is investigated, and the gas permeability is calculated by steady-state method. The coal sample was vacuum dried for 48 h, and then put into the percolation instrument for degassing. Helium gas was firstly used as the experimental gas to test the permeability of the coal sample under the gas pressure of 0–6 MPa. After the helium experiment, the permeability of nitrogen in the same coal sample was carried out to test the change of permeability of nitrogen under the same pressure range. In addition, the permeabilities of helium and nitrogen were measured under the effective stress of 2 MPa, 3 MPa and 4 MPa.
Theoretical methods
Coal is a kind of special porous medium, which has good adsorption capacity for gases (Abouloifa et al., 2021; Chen et al., 2022). At the same time, the gas adsorption changes the internal pore structure, which has a non-negligible influence on the gas flow. The flow law of gas and liquid in porous media is obviously different. At low pressure condition, the moving velocity of gas molecules near the channel wall is not zero, and the apparent permeability measured is higher in low pressure stage, which is also called Klinkenberg effect (Ertekin et al., 1986; Li et al., 2014; Xian et al., 2022). A theoretical model between apparent permeability and Klinkenberg effect have been established (Wang et al., 2014):
Where ka is apparent permeability, mD. There is a Klinkenberg effect in the flow of gas in coal, which will change the permeability obtained from the experimental test, and this experimental value is also called the apparent permeability. k0 is the absolute permeability of coal. The permeability of porous media is related to the channel and flow medium. The absolute permeability of rock mass is an inherent value that there is no physical and chemical reaction between flow medium and rock mass and is independent of fluid properties. Md. b is the Klinkenberg coefficient, MPa; P is the gas pressure, MPa. The coefficient b reflects the strength of the Klinkenberg effect of gas in porous media, which is controlled by the width of gas channel and the nature of gas. The theoretical model of b have also been summarized (Harpalani and Schraufnagel 1991):
Where c is a constant, which is 0.9; μ is the dynamic viscosity of the gas; M is the molecular mass of the gas; R, T are universal gas constant and absolute temperature, respectively;
Absolute permeability k0 of coal sample is a fixed parameter related to pore structure, and the value increases with the width of the passage. The change of pore structure will lead to the change of permeability. Therefore, the apparent permeability of adsorbent gas in coal can be divided into three parts: k0, Δke, and ΔkK, which jointly control the apparent permeability of gas:
Here,
Analysis of experimental results
Effect of effective stress on gas permeability
Helium and nitrogen were used as experimental gases, owing to that helium do not be adsorbed in coal, which would not lead to adsorption deformation, while nitrogen, as an adsorbent gas, could lead to adsorption deformation of coal. Therefore, the control law of adsorption effect and effective stress on gas flow characteristics in coal can be reflected by comparing the permeability results of the two gases. Figure 2 show the relationship between gas permeability and gas pressure under different effective stresses.
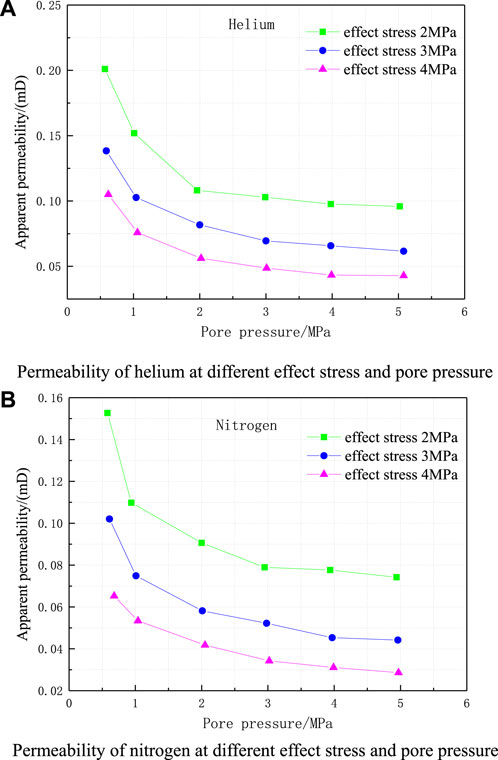
FIGURE 2. The permeability of helium and nitrogen varies with the different effective stress and pressure. (A) Permeability of helium at different effect stress and pore pressure. (B) Permeability of nitrogen at different effect stress and pore pressure.
As can be found in Figure 3 and Table 1, along with the increase of effective stress, the apparent permeability decreases gradually under the same gas pressure. Moreover, the apparent permeability decreases significantly in the initial stage, and the downward trend gradually changes in the later stage. In particular, owing to the influence of gas adsorption, the permeability for nitrogen is obviously lower than that for helium in the same coal sample under the same effective stress and gas pressure.
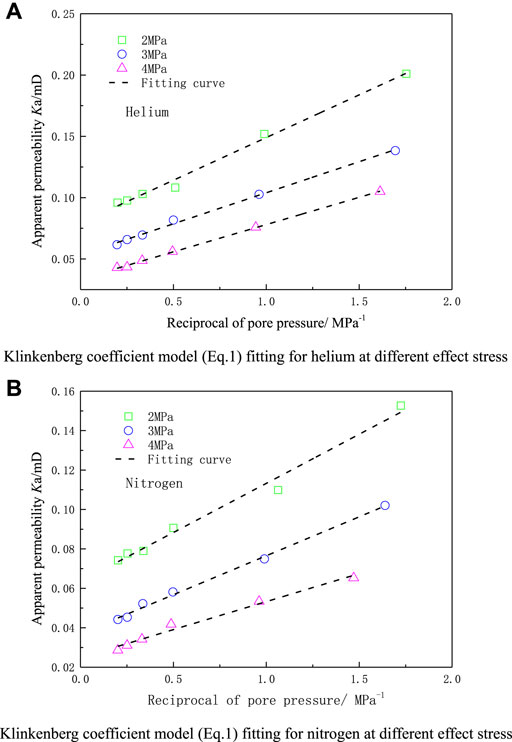
FIGURE 3. Klinkenberg coefficient model fitting results of experimental data. (A) Klinkenberg coefficient model (Eq. 1) fitting for helium at different effect stress (B) Klinkenberg coefficient model (Eq. 1) fitting for nitrogen at different effect stress.
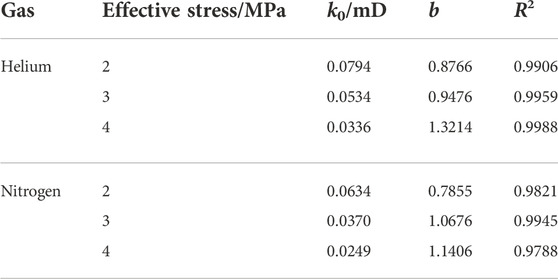
TABLE 1. Absolute permeability and Klinkenberg coefficient of the two gases under different effective stresses.
There is an obvious Klinkenberg effect for the gas flow in porous media. Gas molecules have greater mobility at low pressure, leading to the more significant Klinkenberg effect. Thus, the permeability measured at low pressure usually is higher than that measured at high pressure. Due to the influence of Klinkenberg effect, the measured apparent permeability of helium gas is higher than the absolute permeability under the same effective stress. Moreover, along with the increase of gas pressure, the effect decreases, leading to the smaller difference between apparent permeability. Coal is an organic rock mass. Stress change and gas adsorption could lead to the deformation of the pore structure of coal, while the increase of effective stress and gas adsorption could result in the pore contraction of coal and in turn the decrease of permeability. In helium experiment, the increase of effective stress leads to the decrease of permeability, mainly owing to the stress deformation of coal. In nitrogen experiment, the decrease of permeability is controlled by both effective stress and adsorption effect.
Variation analysis of Klinkenberg effect on gas flow
Klinkenberg effect is a phenomenon that cannot be ignored for gas flow. In order to deeply analyze the effect of effective stress and gas adsorption on Klinkenberg effect, a fitting analysis of the experimental results was carried out by Eq. 3 with the results shown in Figure 3.
As shown, the experimental data of the two groups of gases fits well with the Eq. 1. Along with the increase of effective stress, the coal sample is compressed and the width of the fracture decreases, resulting in the decrease of the absolute permeability of the coal sample and the increase of the Klinkenberg coefficient. k0 is positively correlated with channel width, while b is negatively correlated with channel width. The Klinkenberg effect is controlled by gas properties and channel width. Nitrogen can lead to adsorption expansion and channel contraction of coal samples. Under the same effective stress, both the absolute permeability and Klinkenberg coefficient measured by nitrogen are smaller than those by helium. The fitting rates for the two groups of gases show that the average fitting rate for helium is above 0.99, while the average fitting rate for nitrogen is 0.98. This is because that there are more influencing factors for Klinkenberg coefficient in nitrogen experiment due to gas adsorption.
Analysis of the effect of adsorption on apparent permeability
For two groups of gases in the same coal sample, the coal body is only affected by stress deformation under the same effective stress, and the absolute permeability of the coal sample remains unchanged. The test results of helium are adopted for K0 under different effective stresses in calculation. The Klinkenberg coefficient of nitrogen under different effective stresses is calculated by Eq. 3 with the results shown in Table 2.
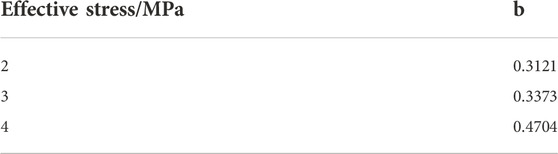
TABLE 2. Calculation results of Nitrogen Klinkenberg coefficient under different effective stresses.
In nitrogen seepage experiment, coal is affected by both stress and gas adsorption. Along with the increase of gas pressure, coal expands and deforms, leading to the gas flow channel shrinks. These make that the measured apparent permeability changes under the influence of gas adsorption effect and Klinkenberg effect. According to Eq. 5, K0 is calculated using the helium test result with the result shown in Figure 4.
As displayed in Figure 4, Δke value is positive under low pressure, but becomes negative along with the increase of pressure. The contribution of Klinkenberg effect to apparent permeability, ΔkK, is related to gas pressure and absolute permeability. The increase of gas pressure or decrease of absolute permeability leads to the decrease of ΔkK. However, this effect results in that the apparent permeability is greater than the absolute permeability in the experiment of non-adsorbent gas. At low pressure stage, the expansion variable of coal body is small, and
Gas flow in coal is a very complicated process, which is not only affected by stress, but also closely related to gas properties (Wang et al., 2021). With the increase of coalbed methane mining intensity, the injection of inert gas (nitrogen and carbon dioxide) has been widely used in the field, and the implementation process is accompanied by the change of effective stress and gas pressure of coal seam. The increasing pressure of adsorbent gas will reduce the Klinkenberg effect of adsorbent gas, while the adsorption effect will narrow the fracture channel and reduce the absolute permeability and apparent permeability. In the process of coalbed methane exploitation, permeability is an important parameter to predict the gas production rate, so it is particularly important to explore the gas flow law under the complex factors in the process of coalbed methane exploitation.
When the pore pressure is the same, the increase of the external stress of coal can lead to the increase of the effective stress, causing the enhancement of the pore size contraction effect. Therefore, the greater the effective stress is, under the same pore pressure, the weaker the contribution of the gas adsorption effect to the apparent permeability is, and the value of
Discussion
Coalbed methane is an important clean energy. Promoting the exploitation of coalbed methane can not only improve the utilization of energy, but also ensure the exploitation of underground coal resources. In China, most coal seams are low permeability rock, greatly limiting the extraction of methane in coal. To improve the production of methane, many scientific and technological workers began to study the technology of injecting inert gas into coal seam to promote methane extraction. This technique is to inject nitrogen or carbon dioxide gas into the coal seam, high pressure gas flow in the coal seam, under the pressure difference and displacement adsorption, promoting methane flow to the extraction borehole.
Coal is a complex porous medium, the process of gas flow is restricted by many factors, and permeability is an important evaluation index in coalbed methane mining. The injection of nitrogen or carbon dioxide into coal seam may cause the increase of gas pressure in coal and the change of effective stress, especially the high strength adsorption gas can produce the ignored pore deformation of coal. These will have a serious impact on the production rate of coalbed methane. Therefore, it is necessary to study the changes of different gas permeability in coal, which has important theoretical guidance for inert gas injection technology and coalbed methane mining.
In this article, through the experimental device, the influence of adsorption expansion caused by adsorbed gas on coal permeability was analyzed in depth, and the decrease degree of coal permeability caused by adsorption expansion was quantified. At the same time, the influence of different effective stress on gas Klinkenberg coefficient was also analyzed. These experimental results play an important role in understanding the microscopic changes of coal permeability. However, in the actual situation of coal seam in situ, there are more influencing factors on gas flow, such as temperature, uneven fracture and so on. These factors cannot be fully reflected in the experiment. In particular, small-scale coal permeability is a conventional method to study coal permeability. While small-scale coal samples cannot represent large-scale coal seams. The application of experimental results in practice still needs further verification and improvement. Although the theoretical and experimental research conditions are significantly different from the actual situation of coalbed methane mining, the research results can also provide suggestions for the improvement of actual coalbed methane mining technology. According to the actual situation, the experimental conditions are constantly improved to promote the continuous optimization and improvement of coalbed methane mining technology.
Permeability is a important index in coalbed methane development, and it has a clear impact on predicting coalbed methane production. Due to the special property of coal seam itself, the pore structure of coal body will change when the stress and gas pressure change, which will affect the permeability of gas in coal. Experimental results showed that with the CBM extraction, gas pressure drop would cause the decrease of the effective stress of coal and the increase of gas permeability, which played an active role in CBM extraction. Meanwhile, low pressure also would cause the more significant phenomenon of the Klinkenberg effect. The permeability value of gas flow in coal can be changed in real time by theoretical calculation. In the process of coalbed methane extraction, the coalbed methane production should be predicted according to the variable permeability. The increase of permeability may lead to the increase of the extraction amount.
Conclusion
(1) With the increase of effective stress, the coal is compressed with the decreased permeabilities of both gases. Under the same effective stress, the apparent permeability gradually decreases along with the increase of gas pressure. Under the same conditions, the permeability of nitrogen is lower than that of helium due to the influence of gas adsorption.
(2) The Klinkenberg effect leads to that the apparent permeability of coal is higher than the absolute permeability, while the gas adsorption effect weakens the fitting degree of the theoretical model. The increase of effective stress can enhance the Klinkenberg coefficient but reduce the absolute permeability at the same time.
(3) At low pressure stage, the decrease of absolute permeability caused by adsorption effect is weaker compared to Klinkenberg effect, giving a positive
Data availability statement
The original contributions presented in the study are included in the article/Supplementary Material; further inquiries can be directed to the corresponding author.
Author contributions
MW: Formal analysis, Investigation, Writing YY: Conceptualization, Resources, Methodology YZ: Conceptualization, Methodology, Writing HS: Formal analysis, Investigation JH: Formal analysis, Investigation.
Funding
The authors are grateful to the National Natural Science Foundation of China (NO. 52174168, NO. 51974301), Key Research and Promotion Projects of Henan Province (NO. 222102320208, NO. 222102320466, NO. 202102310014), Innovation team of colleges and universities in Henan Province (22IRTSTHN009), Key scientific research projects of colleges and universities in Henan Province (NO. 23A620004).
Conflict of interest
The authors declare that the research was conducted in the absence of any commercial or financial relationships that could be construed as a potential conflict of interest.
Publisher’s note
All claims expressed in this article are solely those of the authors and do not necessarily represent those of their affiliated organizations, or those of the publisher, the editors and the reviewers. Any product that may be evaluated in this article, or claim that may be made by its manufacturer, is not guaranteed or endorsed by the publisher.
References
Abouloifa, N., Vandamme, M., and Dangla, P. (2021). Modeling transient variations of permeability in coal seams at the reservoir scale. J. Nat. Gas. Sci. Eng. 88, 103796. doi:10.1016/j.jngse.2021.103796
Banerjee, A., and Chatterjee, R. (2021). A methodology to estimate proximate and gas content saturation with lithological classification in coalbed methane reservoir, bokaro field, India. Nat. Resour. Res. 30, 2413–2429. doi:10.1007/s11053-021-09828-2
Banerjee, A., Chatterjee, R., and Singha, D. K. (2022). Anisotropy and fracture analysis for coalbed methane reservoir development in Bokaro coalfield India. Geophysical Prospecting.13236 doi:10.1111/1365-2478.13236
Chen, M., Masum, S., Sadasivam, S., and Thomas, H. (2022). Modelling anisotropic adsorption-induced coal swelling and stress-dependent anisotropic permeability. Int. J. Rock Mech. Min. Sci. (1997). 153, 105107. doi:10.1016/j.ijrmms.2022.105107
El Amin Mohamed, F., and El Beltagy Mohamed, A. (2021). Stochastic estimation of the slip factor in apparent permeability model of gas transport in porous media. Transp. Porous Media 137, 433–449. doi:10.1007/s11242-021-01575-5
Ertekin, T., King, G. A., and Schwerer, F. C. (1986). Dynamic gas slippage: A unique dual-mechanism approach to the flow of gas in tight formations. Spe Form. Eval. 1 (01), 43–52. doi:10.2118/12045-pa
Gray, Ian. (1997). Reservoir engineering in coal seams: Part 1-the physical process of gas storage and movement in coal seams. SPE Reservoir Engineering.2 doi:10.2118/12514-PA
Harpalani, S., Schraufnagel, A., Ji, H., Mao, Y., and Su, H. (2020). Measurement of parameters impacting methane recovery from coal seamsEffects of organic micromolecules in bituminous coal on its microscopic pore characteristics. Int. J. rock Mech. Min. Sci. geomechanics Abstr. 28262 (6), 116529. doi:10.1016/j.fuel.2019.116529
Hou, P., Xue, Y., Gao, F., Dou, F., Su, S., Cai, C., et al. (2022). Effect of liquid nitrogen cooling on mechanical characteristics and fracture morphology of layer coal under Brazilian splitting test. Int. J. Rock Mech. Min. Sci. 151, 105026. doi:10.1016/j.ijrmms.2021.105026
Jianhua, Li, and Bobo, Li (2021). Modeling of anisotropic coal permeability under the effects of matrix-fracture interaction. J. Nat. Gas Sci. Eng. 93, 104022.
Levine, J., R. (1996). Model study of the influence of matrix shrinkage on absolute permeability of coal bed reservoirs. Geol. Soc. Lond. Spec. Publ. 109 (1), 197–212. doi:10.1144/gsl.sp.1996.109.01.14
Li, P., Yang, Y., Wang, M., Wang, Y., Zhao, X., and Zhang, Y. (2022). Study on the coal damage effects caused by the CO2-alkaline water two-phase displacing gas and wetting coal. Fuel 310, 122305. doi:10.1016/j.fuel.2021.122305
Li, Y., Tang, D., Xu, H., Meng, Y., and Li, J. (2014). Experimental research on coal permeability: The roles of effective stress and gas slippage. J. Nat. Gas. Sci. Eng. 21, 481–488. doi:10.1016/j.jngse.2014.09.004
Lin, W., and Kovscek, A. R. (2014). Gas sorption and the consequent volumetric and permeability change of coal I: Experimental. Transp. Porous Media 105 (2), 371–389. doi:10.1007/s11242-014-0373-9
Liu, Zhen, Li, Raorao, He, Yang, Tian, Fuchao, and Zhu, Danliang (2021). A new fractal model of coal permeability based on the increasing fractal construction method of the menger sponge. Fractals 19, 2150187. doi:10.1142/s0218348x21501875
Liu, Z., Wang, W., Cheng, W., Yang, H., and Zhao, D. (2020). Study on the seepage characteristics of coal based on the Kozeny-Carman equation and nuclear magnetic resonance experiment. Fuel 266, 117088. doi:10.1016/j.fuel.2020.117088
Mitra, A., Harpalani, S., and Liu, S. (2012). Laboratory measurement and modeling of coal permeability with continued methane production: Part 1–Laboratory results. Fuel 94, 110–116.
Moghadam, A. A., and Chalaturnyk, R. (2014). Expansion of the Klinkenberg's slippage equation to low permeability porous media. Int. J. Coal Geol. 123, 2–9. doi:10.1016/j.coal.2013.10.008
Pan, Z., and Connell, L. D. (2012). Modelling permeability for coal reservoirs: A review of analytical models and testing data. Int. J. Coal Geol. 92, 1–44. doi:10.1016/j.coal.2011.12.009
Perera, M., Ranjith, P. G., and Choi, S. K. (2013). Coal cleat permeability for gas movement under triaxial, non-zero lateral strain condition: A theoretical and experimental study. Fuel 109, 389–399.
Rathnayake, S., Rajora, A., and Firouzi, M. (2022). A machine learning-based predictive model for real-time monitoring of flowing bottom-hole pressure of gas wells. Fuel 317, 123524. doi:10.1016/j.fuel.2022.123524
Seidle, J. P., and Huitt, L. G. (1995). “Experimental measurement of coal matrix shrinkage due to gas desorption and implications for cleat permeability increases [C],” in Society of Petroleum, Engineers International meeting on petroleum engineering, 14–17.
Shi, J., and Durucan, S. (2004). Drawdown induced changes in permeability of coalbeds: A new interpretation of the reservoir response to primary recovery. Transp. Porous Media 56 (1), 1–16. doi:10.1023/b:tipm.0000018398.19928.5a
Si, L., Li, Z., and Yang, Y. (2018). Influence of the pore geometry structure on the evolution of gas permeability. Transp. Porous Media 123 (2), 321–339. doi:10.1007/s11242-018-1044-z
Si, L., Wei, J., Xi, Y., Wang, H., Zhang, H., Li, B., et al. (2021). The influence of long-time water intrusion on the mineral and pore structure of coal. Fuel 290 (1), 119848. doi:10.1016/j.fuel.2020.119848
Singh, H., and Javadpour, F. (2016). Langmuir slip-Langmuir sorption permeability model of shale. Fuel 164, 28–37. doi:10.1016/j.fuel.2015.09.073
Somerton, W. H., Söylemezoḡlu, I. M., and Dudley, R. C. (1975). Effect of stress on permeability of coal. Int. J. Rock Mech. Min. Sci. Geomechanics Abstr. 12, 129–145.
Towler, B., Firouzi, M., Underschultz, J., Rifkin, W., Garnett, A., Schultz, H., et al. (2016). An overview of the coal seam gas developments in Queensland. J. Nat. Gas. Sci. Eng. 31, 249–271. doi:10.1016/j.jngse.2016.02.040
Wang, C., Zhang, J., Zang, Y., Zhong, R., Wang, J., Wu, Y., et al. (2021b). Time-dependent coal permeability: Impact of gas transport from coal cleats to matrices. J. Nat. Gas. Sci. Eng. 88, 103806. doi:10.1016/j.jngse.2021.103806
Wang, G., Ren, T., Wang, K., and Zhou, A. (2014). Improved apparent permeability models of gas flow in coal with Klinkenberg effect. Fuel 128, 53–61. doi:10.1016/j.fuel.2014.02.066
Wang, G., Shi, G. Q., Wang, Y. M., and Shen, H. Y. (2021a). Numerical study on the evolution of methane explosion regions in the process of coal mine fire zone sealing. Fuel 289, 119744. doi:10.1016/j.fuel.2020.119744
Wang, G., Shi, G., Yang, Y., and Liu, S. (2022). Experimental study on the exogenous fire evolution and flue gas migration during the fire zone sealing period of the coal mining face. Fuel 320, 123879. doi:10.1016/j.fuel.2022.123879
Wei, M., Liu, J., Elsworth, D., Liu, Y., and He, Z. (2021). Impact of equilibration time lag between matrix and fractures on the evolution of coal permeability. Fuel 290 (1), 120029. doi:10.1016/j.fuel.2020.120029
White, C. M., Smith, D. H., Jones, K. L., Goodman, A. L., Jikich, S. A., Lacount, R. B., et al. (2005). Sequestration of carbon dioxide in coal with enhanced coalbed methane recovery—a review. Energy fuels. 19 (3), 659–724. doi:10.1021/ef040047w
Xian, B., Liu, G., Bi, Y., Gao, D., Wang, L., Cao, Y., et al. (2022). Coalbed methane recovery enhanced by screen pipe completion and jet flow washing of horizontal well double tubular strings. J. Nat. Gas. Sci. Eng. 99, 104430. doi:10.1016/j.jngse.2022.104430
Xiao, K., Zhang, Z., Zhang, R., Gao, M., Xie, J., Zhang, A., et al. (2021). Anisotropy of the effective porosity and stress sensitivity of coal permeability considering natural fractures. Energy Rep. 7 (3), 3898–3910. doi:10.1016/j.egyr.2021.06.067
Xue, Y., Liu, J., Liang, X., Wang, S., and Ma, Z. (2022). Ecological risk assessment of soil and water loss by thermal enhanced methane recovery: Numerical study using two-phase flow simulation. J. Clean. Prod. 334, 130183. doi:10.1016/j.jclepro.2021.130183
Zhang, H., Liu, J., and Elsworth, D. (2008). How sorption-induced matrix deformation affects gas flow in coal seams: A new FE model. Int. J. Rock Mech. Min. Sci. (1997). 45 (8), 1226–1236. doi:10.1016/j.ijrmms.2007.11.007
Zhang, S., Sang, Q., and Dong, M. (2021). Experimental study of pressure sensitivity in shale rocks: Effects of pore shape and gas slippage. J. Nat. Gas. Sci. Eng. 89 (1), 103885. doi:10.1016/j.jngse.2021.103885
Zhou, Y., Li, H., Huang, J., Zhang, R., Wang, S., Hong, Y., et al. (2021). Influence of coal deformation on the Knudsen number of gas flow in coal seams. Energy 233, 121161. doi:10.1016/j.energy.2021.121161
Zhou, Y., Li, Z., Yang, Y., Zhang, L., Qi, Q., Si, L., et al. (2016). Improved porosity and permeability models with coal matrix block deformation effect. Rock Mech. Rock Eng. 49 (9), 3687–3697. doi:10.1007/s00603-016-1005-1
Zhou, Y., Li, Z., Zhang, R., Wang, G., Yu, H., Sun, G., et al. (2019). CO2 injection in coal: Advantages and influences of temperature and pressure. Fuel 236, 493–500. doi:10.1016/j.fuel.2018.09.016
Zhou, Y., Zhang, R., Huang, J., Li, Z., Chen, Z., Zhao, Z., et al. (2020). Influence of alkaline solution injection for wettability and permeability of coal with CO2 injection. Energy 202, 117799. doi:10.1016/j.energy.2020.117799
Keywords: coal reservoir, gas adsorption, permeability, Klinkenberg effect, coalbed methane exploitation
Citation: Wang M, Yang Y, Zhou Y, Shi H and Huang J (2023) The influence mechanism of effective stress, adsorption effect and Klinkenberg effect on coal seam permeability. Front. Energy Res. 10:979117. doi: 10.3389/fenrg.2022.979117
Received: 27 June 2022; Accepted: 31 October 2022;
Published: 12 January 2023.
Edited by:
Hussein Hoteit, King Abdullah University of Science and Technology, Saudi ArabiaReviewed by:
Yi Xue, Xi’an University of Technology, ChinaLiang Huang, Chengdu University of Technology, China
Copyright © 2023 Wang, Yang, Zhou, Shi and Huang. This is an open-access article distributed under the terms of the Creative Commons Attribution License (CC BY). The use, distribution or reproduction in other forums is permitted, provided the original author(s) and the copyright owner(s) are credited and that the original publication in this journal is cited, in accordance with accepted academic practice. No use, distribution or reproduction is permitted which does not comply with these terms.
*Correspondence: Yongliang Yang, eXlsaWFuZzQ1NkAxMjYuY29t