- 1Department of Energy Systems Engineering, Faculty of Technology, Kocaeli University, Kocaeli, Turkey
- 2Departmant of Electric and Energy, Uzunçiftlik Nuh Çimento Vocational School, Kocaeli University, Kocaeli, Turkey
- 3Department of Mechanical and Energy Engineering, School of Engineering, College of Science and Technology, University of Rwanda, Kigali, Rwanda
Due to the inadequacy of distribution networks in developing countries, especially in small residential areas, there are frequent interruptions in the electrical energy provided by the grid. This problem negatively affects the life quality and productivity of the people living in these regions. This problem can be overcome by integrating BESS-supported renewable energy sources into the distribution system. These distributed energy resources contribute significantly to providing energy directly to consumers. On a small scale, such a system is supported by the grid, when possible, to ensure energy supply continuity. This study presents a techno-economic analysis, using PV*SOL simulation software, of a grid-connected solar PV system with BESS that is used to supply a small residential community in Rwanda, Muhanga district, Shyogwe sector. The consumers were a group of one hundred households around a wetland valley. The energy generated from the solar PV system was used to supply home appliances and a water pumping system for agricultural activities. The simulation results showed that the annual energy requirement is 82.34 MWh with a peak load of 30.4 kW. The simulation results also revealed that a PV system, with an installed capacity of 57.33 kWp integrated with a BESS of 89.2 kWh storage capacity, can supply the load with own power consumption of 68.65%, a level of self-sufficiency of 64.38%, and a performance ratio of 86.05% when the desired ratio is set to 110% with a year as the reference period. The financial analysis demonstrated a return on assets of 9.14% and an amortization period of 9.65 years. These results indicate that the proposed method is technically and economically feasible for use in addressing the issue of electrical power outages in developing countries.
1 Introduction
The electricity from solar PV systems has a wide range of applications. Depending on their configuration, PV systems are primarily characterized as off-grid or grid-connected. Off-grid PV systems are most commonly used in remote and rural areas where access to the electrical grid is not possible. Solar-powered home systems, street lighting, water pumping for agriculture use, and large-scale solar PV system mini-grids for the community are examples of off-grid solar PV applications (Jasuan, Nawawi, and Samaulah, 2018). Grid-connected PV systems are often large-scale PV plants that feed generated electricity to the electrical grid. On a small scale, grid-connected PV systems are used for residential and commercial applications (Jasuan, Nawawi, and Samaulah, 2018; Ahmad et al., 2020).
While batteries are used in the off-grid systems to increase the reliability and availability of electricity, grid-connected PV systems supply generated power to the grid (Abdin and Noussan, 2018; Jasuan, Nawawi, and Samaulah, 2018). Batteries are charged when solar PV systems generate more electricity than needed. The stored energy is used during the night and when there is not enough sunlight to satisfy the load. Batteries increase both the reliability and independence of a solar PV system, (Jiang, Kang, and Liu, 2021). For use in residential, commercial, or community (with grid access) applications, battery energy storage systems (BESS) are integrated with grid-connected PV systems to allow more independence from the grid and increase the level of self-consumption (Dorahaki et al., 2022). In such cases, power is taken from the grid only if the power generated by the solar PV system and the energy available in the BESS is not high enough to supply the load. Optimized systems use energy from the grid only to supply the load and batteries are charged from the solar PV systems exclusively, making the overall system more economical.
BESS and solar PV curtailment is a solution to the challenges associated with high PV penetration at distribution networks from a techno-economic perspective. The solutions provided by energy storage integrated with renewable energy sources include discharging stored energy, curtailing or storing energy production, and flexible load (Hargreaves and Jones, 2020). BESS provides energy services such as PV energy time-shift, limiting the PV energy supplied to the grid, and distribution transformer upgrading (Tercan et al., 2022). For more economical PV systems and BESS, a possible strategy is to develop a community energy storage system to reduce individual capital expenditure (Segundo Sevilla et al., 2018).
Various studies have been conducted on the design and simulation of PV systems. Dondariya et al. (2018) conducted a performance simulation study on the grid-connected rooftop solar PV systems for small households in Ujjain, India. The findings showed that 85.30% of the energy generated from the PV system was supplied to the grid, a reduction of 41.09% in energy required from the grid, and a 75.01% performance ratio for the system. Rout and Kulkarni (2020) designed and evaluated the performance of a 2 kWp solar PV rooftop grid-connected system in Odisha using PVsyst. The performance ratio of the system was found to be 0.7. Nhau et al. (2021) analyzed and simulated the economic benefits of different types of PV systems in Shanghai. It was found that the economic profits are different depending on load profile and benefits are higher when residential and commercial load profiles are combined. Segundo Sevilla et al. (2018) conducted a study on techno-economic analysis of battery storage and curtailment in a distributed grid with high PV penetration. It was found that 3.2% and 1.3% of total PV electricity generation should be curtailed to avoid the need for distribution transformer upgrading for fixed and dynamic control techniques. Panjwani et al. (2021) conducted a design and performance analysis of a grid-tied PV system of 8 kWp and an energy storage system. In the designed system, batteries contributed to an increased overall system efficiency of 2%. Shahzad et al. (2017) analyzed the techno-economic feasibility of a solar-biomass off-grid hybrid for the electrification of a remote rural area. The purpose of the study was to design an economical and optimized system to supply electricity for residential loads and irrigation activities in a small village of the Layyah district, Punjab province, Pakistan. Krishan and Suhag (2019) performed techno-economic analysis of a hybrid energy system for the energy-poor rural community of the Yamunanagar district, Haryana state, India, to meet the residential and agricultural energy load. Three configurations of the hybrid system were simulated and analyzed using HOMER software: wind/battery, PV/battery, and wind/PV/battery. Wind/PV/battery was found to be the most cost-effective configuration. Vides-Prado et al. (2018) analyzed the techno-economic feasibility of a PV system in remote areas for indigenous communities in La Guajira, Colombia. The sizing of the system and energy demand calculations were performed based on the community load profile, with communities classified as small, medium, or large. The analysis via HOMER software showed that PV systems for the target communities were economically feasible and, due to the high solar potential, the initial and commissioning expenses would also be low.
There are various simulation tools available for use in solar PV system-related studies. These tools include PVsyst (Alnoosani1 et al., 2018; Kumar et al., 2021; Rout and Kulkarni, 2020; Jagadale, Choudhari, and Jadhav, 2022), INSEL (Nigam and Sharma, 2020; Ram et al., 2022), TRNSYS (Sekhar et al., 2017; Rafał, Maciej, and Wojciech, 2020; Cao et al., 2022), PV*SOL (Dondariya et al., 2018; Mrehel and Albgar, 2018; Nhau et al., 2021; Pushpavalli et al., 2021), SOLARPRO (Alsadi and Khatib, 2018; Lee et al., 2021), HOMER (Al, Awasthi, and Ramli, 2018; Morad et al., 2018; Khalil et al., 2021), and Solar Advisor Model (SAM) (Panjwani et al., 2021; Chennaif et al., 2022; Umar et al., 2018). Software selection depends on the nature and the objectives of the study (Kumar et al., 2021). When choosing software for PV system simulation, the objectives and the system configuration must be carefully considered. Technical performance and economic feasibility are among the factors to be assessed when choosing the proper simulation tool. Table 1 summarizes the advantages and disadvantages of different PV system simulation software.
This study demonstrates the potential of grid-connected solar PV systems and promote their use for local consumption to increase the reliability and availability of electricity. The design allows support from the grid to be provided when the energy from the PV system and BESS is insufficient to supply the load. The amount of energy received from the grid is then returned when the solar PV system generates energy in excess of the load demand and the BESS are fully charged.
This article includes the methodology, presented in Section 2, and simulation performance results, presented in Section 3. Section 4 provides a conclusion and discussion of our findings.
2 Methodology
2.1 PV system simulation tool selection
Sunlight is pervasive on the earth, allowing us to take advantage of solar energy from any location. However, the mere presence of the sunlight in a particular location is not strong enough rationale to support installation of a PV system. Other factors have to be considered, including solar intensity, weather conditions, and solar potential. These considerations inform design of a solar PV system that is as efficient as possible from a techno-economic perspective. Therefore, it is critical to design and simulate the output from a solar PV system before its installation.
Designing, simulating, and optimizing PV systems require simulation tools. Although many solar PV system design and analysis tools exist, their capabilities, options, and applicability differ. In selecting software for PV system simulation, project objectives and system specifications must be considered, along with technical performance and economic feasibility Table 1 compares the characteristics ofavailable PV system simulation software options per their advantages and limitations.For this study, PV*SOL software was chosen for the design and simulation of a PV system with BESS. PV*SOL is one of the leading PV system design and simulation tools. Engineers, planners, architects, installers, and technicians worldwide use PV*SOL to design and build efficient PV systems. With PV*SOL, different types of modern PV systems can be designed and tested via simulation, from small-scale rooftop PV systems of a few panels to large-scale PV power plants of up to 100,000 modules (PV*SOL® premium, 2022).
2.2 Location
Choosing a location for solar PV system application depends on several factors. Solar PV modules must be installed to face the solar radiation, and loss of sunlight exposure due to shading should be minimized. Location selection has a significant impact on the performance and efficiency of a PV system (Kayhan, Ulker, and Elma, 2015).
An optimized location nearby the consumers should be chosen when installing a PV system. For this study, a solar PV system was installed in Rwanda, Southern province, Muhanga district in Shyogwe sector at −2o5′7″ latitude and 29o46′23″ longitude. The selected location is an equatorial region. Geographically, equatorial regions receive higher levels of solar radiation compared to other regions. In the selected region, the annual global irradiation is 1,853 kW/m2, with an average temperature of 19.9°C. High solar energy potential with an average ambient temperature ensures effective operation of a solar PV system. Therefore, in the selected location, the PV system takes advantage of high solar potential and the drawbacks associated with high temperature are minimized. The electricity generated from the solar PV system would be used to supply energy for residential loads and water pumping systems for agricultural activities in an area around a wetland valley. The PV*SOL software contains a climate database based on MeteoNorm from the Swiss climate and weather data experts Meteotest (Climate data, 2022). The irradiance onto horizontal and outside temperature values in summer for the first week of June are shown in Figure 1.
2.3 Load profile
Residential load profile is most commonly modeled using hourly demand or by assumption (Elma and Selamogullari, 2012). The consumers in our study were a group of people in the socio-economic middle class in Rwanda. Most of the people lived in small, three-bedroom houses with a sitting room, and a small kitchen built near the main house. There was access to the grid for some of the households. Grid-connected houses used most of the electricity for lighting purposes. The other uses of electricity were for powering home appliances, such as radios and televisions, as well as charging mobile phones. Water pumping systems for agriculture was another possible use of electricity by the community, with the goal of improving the lives of residents by increasing agricultural production. One hundred households were considered for this study, and Table 2 shows the corresponding daily load estimation and consumption. The load profile was estimated after consulting 20 households (20% of the total load) and the power ratings of the most used home appliances. It was assumed that other remaining households would have the same load demand. The detailed load profile data were entered in the PV*SOL software and the yearly energy consumption and peak load demand values were calculated as 82.34 MWh and 30.4 kW, respectively.
2.4 System configuration
2.4.1 PV modules
PV modules convert solar energy to direct current (DC) electricity. A solar cell is the smallest unit in solar modules. PV modules are made of solar cells connected in series and/or in parallel. Materials used for the production of PV modules include monocrystalline silicon, polycrystalline silicon, amorphous silicon, cadmium telluride, and copper indium gallium selenide/sulfide. PV technologies are classified according to the materials from which PV modules are made, such as monocrystalline silicon, polycrystalline silicon, and thin-film technologies. Monocrystalline technology is suitable for long-term solar PV investment due to its lifespan, performance, and efficiency. As with other solar PV technologies, the monocrystalline market continues to grow.
The PV*SOL software database lists the PV modules available on the market. PV modules are chosen manually by selecting a list of modules from which PV*SOL then recommends the appropriate module to use. In this study, a list of monocrystalline modules from different manufacturers was selected and PV*SOL recommended the best-fitting modules according to the location and load profiles. The number of modules needed depends on the shading factor, degradation of the module, and desired ratio to consumption. The number of modules can be adjusted as a percentage of the annual or monthly consumption. The desired ratio to consumption has two parameters: the desired ratio (consumption margin) and the reference period. The reference period is defined as the part of the year that PV*SOL takes as a reference for sizing the PV modules to satisfy demand throughout the year. In this study, the shading factor and degradation of the module were set to 5 and 1%, respectively, for a year. For the desired ratio, different values between 90 and 120% were chosen and the results were compared.
2.4.2 Inverters and battery system
Solar PV modules produce DC power as output. Electrical power is transmitted and distributed in AC form and home appliances run on AC power. Thus, the DC power output from the solar PV modules must be converted to AC form. With the help of an inverter, the DC voltage from PV modules is converted into AC voltage. Additionally, PV modules produce electricity only when there is sunlight available, highlighting the importance of storing part of the energy generated via PV modules for later use when sunlight is not available. Batteries are integrated with PV modules for storage of excess energy. The battery system absorbs and supplies electricity through a bidirectional power converter (Batiyah et al., 2020).
In the PV*SOL database, different inverter types with varying configurations are available. Determining the correct inverter type and configuration is done by selecting a list of inverter types from different manufacturers. The PV*SOL software is then used to analyze and optimize the compatibility of the inverters from the selected list and identify convenient inverter configurations for the PV system. The same procedure is followed when choosing the battery system.
Figure 2 shows the circuit diagram of the proposed system and the system’s components. Details about the components in the circuit diagram are in the blue legend at the bottom of Figure 2. Hanwha Q. CELLS is the manufacturer of the chosen PV module. The model is Q. PEAK DUO XL-G9.3 455, which is a silicon monocrystalline module. A monocrystalline solar panel was chosen due to its advantages compared to other types of solar panels, including high efficiency, small area requirement per unit power, and long lifespan. Sonnen GmbH is the battery manufacturer, and the battery model is sonnenBatterie 10/99 kWh −27.6 kW. Table 3 shows the electrical parameters of the battery, the PV module at standard test condition (STC), and the inverter configuration in the designed PV system. The technical parameters used for the design and simulation of the PV system with BESS using PV*SOL software are shown in Table 4.
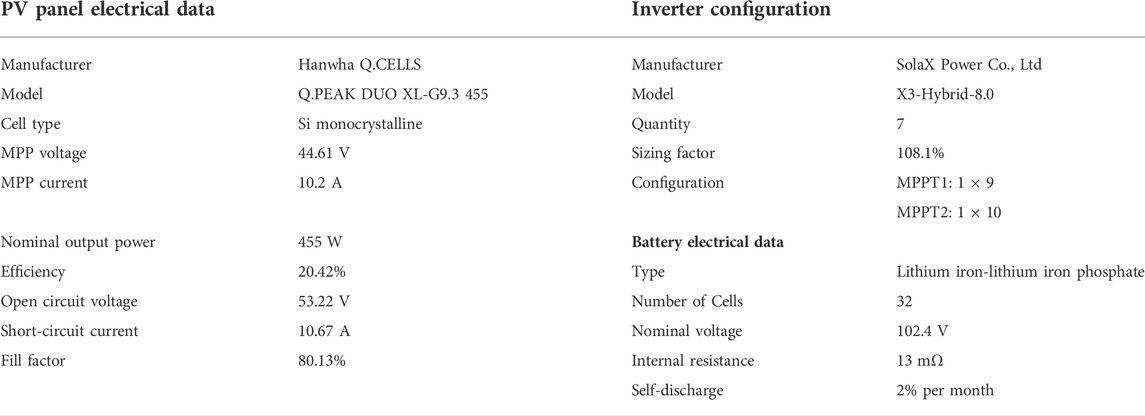
TABLE 3. PV panel characteristics at STC and battery and inverter configuration electrical parameters.
2.5 Financial analysis
Financial analysis helps to determine the needed investment for the realization of the designed PV system. Information such as installation cost, the value of production, operating and maintenance cost, return on investment, and amortization period are obtained from financial analysis. Financial analysis helps in making a decision on whether or not to invest in a solar PV system.
PV*SOL software can be used to analyze the financial aspects of a solar PV system. The financial parameters are entered into the software. Other parameters include the energy balance and feed in concept, price of electricity sold to a third party, net-metering tariffs, and inflation rate for energy price. For this study, the designed PV system simulation included financial analysis that covered 20 years. The financial analysis parameters are shown in Table 5.
3 Simulation results
A study of the design and simulation of a grid-connected solar PV system with electrical appliances was conducted. The consumers were a group of 100 households located in Rwanda, Southern province in the Muhanga district, Shyogwe sector. For the system to become more independent from the grid, the desired ratio was varied using values of 90%, 100%, 110%, and 120%. The reference period for simulation was either a year or the worst month for each desired ratio. The worst month is the month of the year during which the solar radiation is at the minimum for that location. The worst month changes from location to location. The PV*SOL software uses accessible data to guide selection of the worst month for a given location. Thus, during the worst month, the PV system generates the least energy compared to any other comparable timeframe throughout the year. When the worst month is chosen as the reference period, the size of the PV system is designed to satisfy demand during the month of lowest energy production. This helps in optimizing the combination of desired ratio and reference period to allow the system to become more independent, with the capacity to supply the load demand throughout the year. To analyze the effects of shading, the shading values of 0, 5, 10, and 15% were assessed for each combination of desired ratio and reference period. The average values from different shading levels were taken as the result of the corresponding desired ratio and reference period. In total, eight cases were studied. Table 6 provides a summary of the simulation results from different cases.
The performance results from different scenarios are assessed by grading the results from 100% to 60% according to the best and worst results for each parameter. Parameters considered were the level of self-sufficiency, performance ratio, return on assets, amortization period, and payback energy ratio. Table 7 includes a summary of the results of the performance analysis. In this study, the case with the desired ratio of 110% and a year as the reference period was found to be the best scenario, both technically and financially. The direct power consumption, level of self-sufficiency, performance ratio, and payback energy ratio are 68.65, 64.38, 86.05, and 89.14%, respectively. The amortization period was between 9.55 and 10.87 years for all cases. The load was supplied directly from the PV system, battery, or grid. The battery was charged predominately from the PV system and rarely from the grid. Figure 3 shows how energy was supplied to the load from the solar PV system, battery, or grid. Figure 4 is a graph of the energy flow. The financial analysis results demonstrate that the system’s return on assets and the amortization period are 9.14% and 9.65 years, respectively. Figure 5 shows the accrued cash flow.
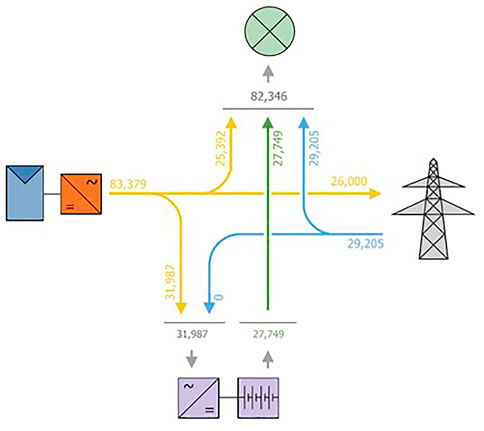
FIGURE 4. Yearly energy flow in kWh for the desired ratio of 110% and a year as the reference period.
4 Conclusion
In this study, design and simulation of a grid-connected solar PV system with a BESS is conducted using PV*SOL. The first step was to design the PV system and determine the load profile. System design involved location choice, the load profile, and other solar-related parameters, such as shading value, degradation of the modules, and desired ratio to consumption. The chosen location was Rwanda, Muhanga district, Shyogwe sector. The load profile consisted of 100 small households and water pumping systems for agricultural activities. The annual consumption was found to be 82.34 MWh with a peak load of 30.4 kW. From analysis of the simulation results, we found that this grid-connected solar PV system with a BESS could supply the load with a direct power consumption of 68.65%, a level of self-sufficiency of 64.38%, a performance ratio of 86.05%, and an energy payback ratio of 89.14%. The financial analysis showed that the return on assets and amortization period were 9.14% and 9.65 years, respectively. The results of this study demonstrate that PV systems with BESS are important to reduce grid dependence and increase the availability and reliability of electricity in developing countries. Additionally, the results indicate that grid-connected PV systems with BESS are techno-economically feasible for developing countries. Future work will focus on the impact of connecting such PV systems with BESS, at various locations in Rwanda, on the national electrical grid and overall grid reliability.
Data availability statement
The original contributions presented in the study are included in the article/Supplementary Material; further inquiries can be directed to the corresponding author.
Author contributions
All authors listed have made a substantial, direct, and intellectual contribution to the work and approved it for publication.
Conflict of interest
The authors declare that the research was conducted in the absence of any commercial or financial relationships that could be construed as a potential conflict of interest.
Publisher’s note
All claims expressed in this article are solely those of the authors and do not necessarily represent those of their affiliated organizations, or those of the publisher, the editors, and the reviewers. Any product that may be evaluated in this article, or claim that may be made by its manufacturer, is not guaranteed or endorsed by the publisher.
References
Abdin, G. C., and Noussan, M. (2018). Electricity storage compared to net metering in residential PV applications. J. Clean. Prod. 176, 175–186. doi:10.1016/j.jclepro.2017.12.132
Ahmad, L., Khordehgah, N., Malinauskaite, J., and Jouhara, H. (2020). Recent advances and applications of solar photovoltaics and thermal technologies. Energy 207, 118254–254. doi:10.1016/j.energy.2020.118254
Al, H. Z., Awasthi, A., and Ramli, M. A. M. (2018). Optimal design and analysis of grid-connected photovoltaic under different tracking systems using HOMER. Energy Convers. Manag. 155, 42–57. doi:10.1016/j.enconman.2017.10.090
Alnoosani1, A., Oreijah, M., Samkari, Y., and Faqeha, H. (2018). Design of 100MW solar PV on-grid connected power plant using (PVsyst) in umm Al-qura university. Int. J. Sci. Res. 8 (11), 356–363. doi:10.21275/30101901
Alsadi, S., and Khatib, T. (2018). Photovoltaic power systems optimization research status: A review of criteria, constrains, models, techniques, and software tools. Appl. Sci. 8 (10), 1761. doi:10.3390/app8101761
Batiyah, S., Sharma, R., Abdelwahed, S., and Zohrabi, N. (2020). An MPC-based power management of standalone DC microgrid with energy storage. Int. J. Electr. Power & Energy Syst. 120, 105949. doi:10.1016/j.ijepes.2020.105949
Cao, Y., Taslimi, M. S., Dastjerdi, S. M., Ahmadi, P., and Ashjaee, M. (2022). Design, dynamic simulation, and optimal size selection of a hybrid solar/wind and battery-based system for off-grid energy supply. Renew. Energy 187, 1082–1099. doi:10.1016/j.renene.2022.01.112
Chennaif, M., Maaouane, M., Zahboune, H., Elhafyani, M., and Zouggar, S. (2022). Tri-objective techno-economic sizing optimization of off-grid and on-grid renewable energy systems using electric system cascade extended analysis and system Advisor model. Appl. Energy 305, 117844. doi:10.1016/j.apenergy.2021.117844
Climate data (2022). Available at: https://help.valentin-software.com/pvsol/en/calculation/irradiation/climate-data/(Accessed April 15, 2022).
Dondariya, C., Porwal, D., Awasthi, A., Shukla, A. K., Sudhakar, K., SR, M. M., et al. (2018). Performance simulation of grid-connected rooftop solar PV system for small households: A case study of Ujjain, India. Energy Rep. 4, 546–553. doi:10.1016/j.egyr.2018.08.002
Dorahaki, S., Rashidinejad, M., Ardestani, S. F. F., Abdollahi, A., and Salehizadeh, M. R. (2022). A home energy management model considering energy storage and smart flexible appliances: A modified time-driven prospect theory approach. J. Energy Storage 48, 104049. doi:10.1016/j.est.2022.104049
Elma, O., and Selamogullari, U. S. (2012). A comparative sizing analysis of A renewable energy supplied stand-alone house considering both demand side and source side dynamics. Appl. Energy 96, 400–408. doi:10.1016/j.apenergy.2012.02.080
Hargreaves, J. J., and Jones, R. A. (2020). Long term energy storage in highly renewable systems. Front. Energy Res. 8, 1–10. doi:10.3389/fenrg.2020.00219
Jagadale, P. R., Choudhari, A. B., and Jadhav, S. S. (2022). Design and simulation of grid connected solar Si-poly photovoltaic plant using PVsyst for pune. India Locat. 3 (1), 41–49.
Jasuan, A., Nawawi, Z., and Samaulah, H. (2018). “Comparative analysis of applications off-grid PV system and on-grid PV system for households,” in 2018 International Conference on Electrical Engineering and Computer Science (ICECOS) (IEEE), 253–258.
Jiang, Y., Kang, L., and Liu, Y. (2021). Multi-Objective design optimization of a multi-type battery energy storage in photovoltaic systems. J. Energy Storage 39, 102604. doi:10.1016/j.est.2021.102604
Kayhan, V. A., Ulker, F., and Elma, O. (2015). “Photovoltaic system design, feasibility and financial outcomes for different regions in Turkey,” in 2015 4th International Conference on Electric Power and Energy Conversion Systems (EPECS) (IEEE), 1–6.
Khalil, L., Bhatti, K. L., Awan, M. A. I., Riaz, M., Khalil, K., and Alwaz, N. (2021). Optimization and designing of hybrid power system using HOMER pro. Mater. Today Proc. 47, S110–S115. doi:10.1016/j.matpr.2020.06.054
Krishan, O., and Suhag, S. (2019). Techno-economic analysis of a hybrid renewable energy system for an energy poor rural community. J. Energy Storage 23, 305–319. doi:10.1016/j.est.2019.04.002
Kumar, R., Rajoria, C. S., Sharma, A., and Suhag, S. (2021). Design and simulation of standalone solar PV system using PVsyst software: A case study. Mater. Today Proc. 46, 5322–5328. doi:10.1016/j.matpr.2020.08.785
Lee, K. W., Lee, H. M., Lee, R. D., Kim, D. S., and Yoon, J. H. (2021). The impact of cracks in BIPV modules on power outputs: A case study based on measured and simulated data. Energies 14 (4), 836. doi:10.3390/en14040836
Morad, M., Nayel, M., Elbaset, A. A., and Galal, A. I. A. (2018). “Sizing and analysis of grid-connected microgrid system for assiut university using HOMER software,” in 2018 Twentieth International Middle East Power Systems Conference (MEPCON) (IEEE), 694–699.
Mrehel, O. G., and Albgar, K. A. (2018). “Design and simulation of a large-scale PV power generation in ben-walid city,” in Libyan international conference on electrical engineering and technologies (LICEET2018).
Nhau, N., Li, J., Wang, W., Yu, W., Zhao, C., and Liu, Y. (2021). “Simulation and analysis of economic benefits of different types of PV systems in shanghai,” in 2021 Power System and Green Energy Conference (PSGEC) (IEEE), 291–295. doi:10.1109/PSGEC51302.2021.9542679
Nigam, A., and Sharma, K. K. (2020). Performance evaluation/analysis of distributed generation system. Eur. J. Mol. Clin. Med. 7 (07), 4638–4650.
Panjwani, M. K., Kumar, J., Parhyar, N. R., and Khan, D. (2021). Design and performance analysis of PV grid-tied system with energy storage system. Int. J. Electr. Comput. Eng. 11 (2), 1077–1085. doi:10.11591/ijece.v11i2.pp1077-1085
Pushpavalli, M., Abirami, P., Sivagami, P., and Geetha, V. (2021). “Investigation of grid connected PV system with electrial appliances, electric vehicles and battery systems using PVsol software,” in Proceedings of the Fist International Conference on Advanced Scientific Innovation in Science, Engineering and Technology, ICASISET 2020, Chennai, India, 16-17 May 2020. doi:10.4108/eai.16-5-2020.2304108
PV*SOL® premium (2022). Available at: https://valentin-software.com/en/products/pvsol-premium/(Accessed: May 28, 2022).
Rafał, F., Maciej, Ż., and Wojciech, G. (2020). Dynamic simulation and energy economic analysis of a household hybrid ground-solar-wind system using TRNSYS software. Energies 13 (14), 3523. doi:10.3390/en13143523
Ram, K., Swain, P. K., Vallabhaneni, R., and Kumar, A. (2022). Critical assessment on application of software for designing hybrid energy systems. Mater. Today Proc. 49, 425–432. doi:10.1016/j.matpr.2021.02.452
Rout, K. C., and Kulkarni, P. S. (2020). “Design and performance evaluation of proposed 2 kW solar PV rooftop on grid system in Odisha using PVsyst,” in 2020 IEEE International Students’ Conference on Electrical, Electronics and Computer Science, SCEECS 2020 (IEEE), 1–6. doi:10.1109/SCEECS48394.2020.124
Sekhar, Y. R., Ganesh, D., Kumar, A. S., Abraham, R., and Padmanathan, P. (2017). Performance simulation of a grid connected photovoltaic power system using TRNSYS 17. IOP Conf. Ser. Mat. Sci. Eng. 263 (6), 062078–064229. doi:10.1088/1757-899X/263/6/062078
Sevilla, F. R. S., Parra, D., Wyrsch, N., Patel, M. K., Kienzle, F., and Korba, P. (2018). Techno-economic analysis of battery storage and curtailment in a distribution grid with high PV penetration. J. Energy Storage 17, 73–83. doi:10.1016/j.est.2018.02.001
Shahzad, M. K., Zahid, A., ur Rashid, T., Rehan, M. A., Ali, M., and Ahmad, M. (2017). Techno-economic feasibility analysis of a solar-biomass off-grid system for the electrification of remote rural areas in Pakistan using HOMER software. Renew. Energy 106, 264–273. doi:10.1016/j.renene.2017.01.033
Tercan, S. M., Elma, O., Gokalp, E., and Cali, U. (2021). An expansion planning method for extending distributed energy system lifespan with energy storage systems. London: Energy Exploration & Exploitation. doi:10.1177/01445987211058304
Umar, N., Bora, B., Banerjee, C., and Panwar, B. S. (2018). Comparison of different PV power simulation softwares: Case study on performance analysis of 1 MW grid-connected PV solar power plant. Int. J. Eng. Sci. Invent. (IJESI) 7 (7), 11–24.
Vides-Prado, A., Camargo, E. O., Vides-Prado, C., Orozco, I. H., Chenlo, F., Candelo, J. E., et al. (2018). Techno-economic feasibility analysis of photovoltaic systems in remote areas for indigenous communities in the Colombian guajira. Renew. Sustain. Energy Rev. 82, 4245–4255. doi:10.1016/j.rser.2017.05.101
Keywords: solar energy, PV system, battery energy storage system (BESS), simulation tools, PV∗SOL, energy reliability
Citation: Nkuriyingoma O, Özdemir E and Sezen S (2022) Techno-economic analysis of a PV system with a battery energy storage system for small households: A case study in Rwanda. Front. Energy Res. 10:957564. doi: 10.3389/fenrg.2022.957564
Received: 31 May 2022; Accepted: 18 July 2022;
Published: 19 August 2022.
Edited by:
Onur Elma, Çanakkale Onsekiz Mart University, TurkeyReviewed by:
Mohammad Reza Salehizadeh, Islamic Azad University, Marvdasht, IranHossam A. Gabbar, Ontario Tech University, Canada
Santanu Kumar Dash, Vellore Institute of Technology, India
Ozan Erdinç, Yıldız Technical University, Turkey
Copyright © 2022 Nkuriyingoma, Özdemir and Sezen. This is an open-access article distributed under the terms of the Creative Commons Attribution License (CC BY). The use, distribution or reproduction in other forums is permitted, provided the original author(s) and the copyright owner(s) are credited and that the original publication in this journal is cited, in accordance with accepted academic practice. No use, distribution or reproduction is permitted which does not comply with these terms.
*Correspondence: Obed Nkuriyingoma, nkuliyobas@gmail.com