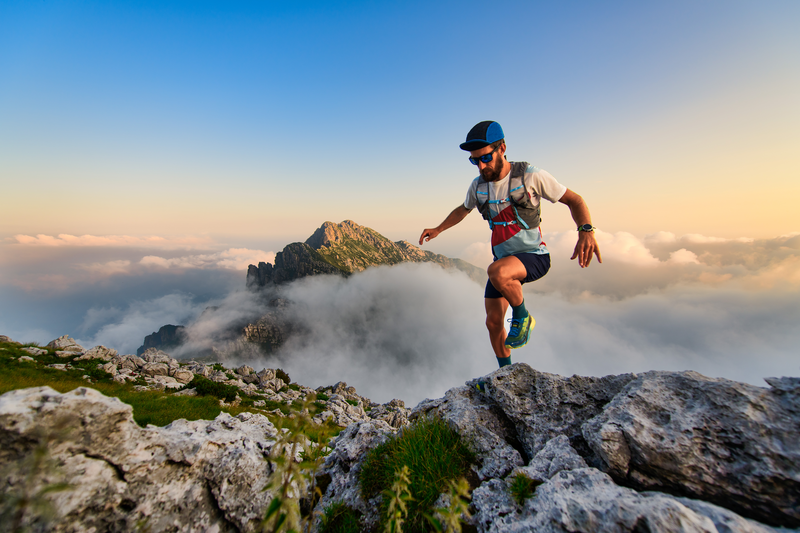
94% of researchers rate our articles as excellent or good
Learn more about the work of our research integrity team to safeguard the quality of each article we publish.
Find out more
OPINION article
Front. Energy Res. , 22 June 2022
Sec. Process and Energy Systems Engineering
Volume 10 - 2022 | https://doi.org/10.3389/fenrg.2022.951085
This article is part of the Research Topic Advanced Thermal Conduction, Thermal Insulation, Thermal Storage Materials and Technologies View all 7 articles
Thermal management systems of buildings can be categorized in active systems (such as direct heating, ventilation and cooling via fossil fuels and electric sources) and passive systems (such as storing/releasing heat in sensible, latent and thermochemical modes). It is true that active systems are more efficient, but the rising energy crisis worldwide demands the potential alternatives or the assisting technologies to save the energy (Liu et al., 2013). In these circumstances, the combination of active-passive systems, for example, the electric systems combined with heat storage systems, has provided sustainable outcomes. Further, in heat storage systems, latent heat storage/release technology is supposed to be competitive due to relatively stable operating temperature and high heat storage density enabled by phase change materials (PCMs) (Huang et al., 2021). PCMs are the core components by which various latent heat-based heat exchangers (LHEs) can be configured and equipped with electric-driven thermal management systems of buildings. LHEs are deemed to be the emerging devices that are mainly implemented outside of buildings, i.e., they are dissimilar compared with PCM-based systems (in-built PCMs) that are incorporated in the tiles, walls, roofs and floors of buildings (Zhang et al., 2021). Therefore, thermal characteristics of PCMs required to design LHEs are also deemed to be different than that of in-built PCMs. In LHEs, heat transfer occurs between the heat transfer fluid and PCMs (Chen et al., 2020a). At present, PCMs that are used to design LHEs pose potential challenges due to their low thermal conductivity, which drastically affects the heat storage/release efficiency of LHEs equipped in thermal management systems of buildings. Therefore, improving the thermal conductivity of PCMs (Liu et al., 2022) and optimizing the design of LHEs need further research efforts.
This opinion includes the current application status of PCMs and designs of LHEs suitable for thermal management of buildings, particularly focusing on the existing challenges and future prospects.
PCMs are defined as the materials undergoing interconvertible thermodynamic states under the influence of the imposed temperatures (Gulfam et al., 2019). In general, the interconvertible thermodynamic state refers to the physical phenomenon of phase change at a particular melting temperature, during which the latent heat is stored/released, establishing the working principle of PCMs. PCMs can be generally classified depending on the chemical attributes, melting temperatures and phase change phenomenon. Based on the chemical attributes, PCMs are classified in organics, inorganics and eutectics (Gulfam et al., 2019). Based on the melting temperatures, they can be categorized into low melting PCMs with <100°C (e.g., paraffin waxes and fatty acids), medium melting PCMs lying in range of 100–300°C (e.g., salt hydrates), and high melting PCMs with >300°C (e.g., metals) (Zhang et al., 2016). The phase change phenomenon is itself of two kinds, including the structural change and the state change (Gulfam et al., 2019). In structural change, crystal structures of the material are internally changed which results in the class of solid-solid PCMs (e.g., polyurethane). In state change, the physical appearance of the material is changed from one form to another, leading to classes of solid-liquid (i.e., paraffin waxes) and liquid-gas (e.g., water) PCMs. The organics are mostly paraffin waxes and fatty acids, while the inorganics are hydrated salts. Paraffin waxes own high latent heat storage capacity (roughly in range of 200–250 J/g) and are declared as the green materials, i.e., environmental-friendly, while fatty acids are declared to be less toxic compared with the inorganic hydrated salts (Gulfam and Zhang, 2019). The main challenge of organics is the low thermal conductivity that is being solved by fabricating the suitable thermal composites. Although inorganics have a slightly higher thermal conductivity compared with organics, the challenges of subcooling and phase separation still exist in hydrated salts which can be solved via certain nucleating agents and thickeners while fabricating thermal composites.
Keeping certain challenges of PCMs ahead as described above, it is necessary to carefully choose and properly fabricate PCM-based thermal composites to ensure safe and stable operations of LHEs. Thermal composites can be fabricated by utilizing various additives (Chen et al., 2020b), known as thermal reinforcements, majorly including the inorganic sorbents (carbonic, silicate and metallic reinforcements) and organic/inorganic encapsulants (polymerics, surfactants and inorganics). For proper design of LHEs, thermal composites should be fabricated conforming to the parameters of primary importance, namely, shape-stabilization, moderate or high thermal conductivity enhancement and stabilization of latent heat storage capacity.
Several experimental case-studies have been successfully presented, leading to fabrication of PCM-based thermal composites and LHEs. For example, PCMs are filled in the metallic fins (uniform and non-uniform fin structures) through which the heat transfer area is considerably increased, as shown in Figure 1A. Metallic fins have the advantages of low cost, easy installation and good stability (Gürtürk and Kok, 2020). In a word, LHEs configured via metallic fin-based PCM thermal composites can work effectively when incorporated in thermal management systems of buildings. Other example includes the metallic foams (i.e., made of copper, aluminum, and nickel, etc.) into which PCMs are infused, as illustrated in Figure 1B. The porosity of metallic foams plays the main role, establishing the thermal paths necessary to expedite the heat transfer of PCMs that finally results in the promising LHEs (Zhang et al., 2020). In encapsulant-based thermal composites as illustrated in Figure 1C, PCMs are packaged as the core which is surrounded by the polymeric/inorganic shell (Gao and Chen, 2019). The shape of the microcapsules can be regular (such as spherical, tubular and elliptical) or irregular. The microcapsules are packaged in LHEs, which increases the specific surface area, thereby enhancing the heat transfer between PCMs and heat transfer fluid.
FIGURE 1. Potential thermal conductivity enhancers including (A) Metallic fins (Huang et al., 2021), (B) Metallic foams (Zhang et al., 2020) (C) Microcapsules. Schematic diagram of implementation of LHEs (latent heat-based heater exchangers) in (D) Independent electric heater (Zhao et al., 2020) and (E) Integrated electric heater based thermal management systems of buildings (Zhao et al., 2020).
At present, there exists mainly two types of active-passive combined thermal management systems for buildings, namely, independent electric heater-LHE system (Figure 1D, (Lee et al., 2022)) and integrated electric heater-LHE system (Figure 1E). The working process of both systems is the same, but the configurations are different, i.e., for the independent electric heater-LHE system, electric heater and LHEs are two separate components; while for the integrated electric heater-LHE system, many LHEs are combined and equipped with an electric heater. PCMs are encapsulated in a cylindrical or spherical container and heat transfer fluid stored in the tube flows through the container to form LHEs (He et al., 2022). Moreover, PCMs can be packaged in serpentine tubes as well. Many serpentine tubes form a heat exchanger plate and many heat exchanger plates constitute the LHE (Lin et al., 2020). During the trough period of electricity consumption at night, the electric heater is turned on for heating (Zhao et al., 2020). Meanwhile, part of the heat is stored by LHEs. During the peak period of electricity consumption, the electric heater is turned off, and the heat is released from LHE to the circulating water which is then supplied to end-users for heating purposes.
Combining the conventional active thermal management systems of buildings with the passive latent heat-based heat exchangers (LHEs) is declared to be the emerging technology, addressing the challenges imposed by the worldwide energy crisis. However, the challenges corresponding to the PCMs selection, fabrication of PCM-based thermal composites, design of LHEs and their proper implementation in conventional thermal management systems of buildings still need serious efforts. The following aspects need to be further studied:
1) Develop PCM-based thermal composites having excellent thermal properties, high heat storage density, long cyclic life and low cost. For example, adding high thermal conductivity particles to improve thermal conductivity in only optimal quantities or using the multiple PCMs to meet the constant temperature difference and longer temperature control capacity.
2) Optimize the internal structural design of LHEs, such as combining the fin technology with porous network to form a fin-porous heat exchanger ensuring the improved heat transfer.
3) Address the stability and reliability-related challenges of LHEs in intermittent operations, with a special focus on realizing the intelligent adjustment and control.
TZ wrote the first draft of the manuscript. FY wrote sections of the manuscript. RG: Review and Writing. All authors contributed to manuscript revision, read, and approved the submitted version.
The authors would like to acknowledge the support received from The National Natural Science Foundation of China (no. 51906170).
The authors declare that the research was conducted in the absence of any commercial or financial relationships that could be construed as a potential conflict of interest.
All claims expressed in this article are solely those of the authors and do not necessarily represent those of their affiliated organizations, or those of the publisher, the editors and the reviewers. Any product that may be evaluated in this article, or claim that may be made by its manufacturer, is not guaranteed or endorsed by the publisher.
Chen, X., Tang, Z., Gao, H., Chen, S., and Wang, G. (2020a). Phase Change Materials for Electro-Thermal Conversion and Storage: from Fundamental Understanding to Engineering Design. iScience 23, 101208. doi:10.1016/j.isci.2020.101208
Chen, X., Gao, H., Tang, Z., Dong, W., Li, A., and Wang, G. (2020b). Optimization Strategies of Composite Phase Change Materials for Thermal Energy Storage, Transfer, Conversion and Utilization. Energy Environ. Sci. 13, 4498–4535. doi:10.1039/d0ee01355b
Gao, W., and Chen, Y. (2019). Microencapsulation of Solid Cores to Prepare Double Emulsion Droplets by Microfluidics. Int. J. Heat Mass Transf. 135, 158–163. doi:10.1016/J.IJHEATMASSTRANSFER.2019.01.136
Gulfam, R., Zhang, P., and Meng, Z. (2019). Advanced Thermal Systems Driven by Paraffin-Based Phase Change Materials - A Review. Appl. Energy 238, 582–611. doi:10.1016/J.APENERGY.2019.01.114
Gulfam, R., and Zhang, P. (2019). Power Generation and Longevity Improvement of Renewable Energy Systems via Slippery Surfaces - A Review. Renew. Energy 143, 922–938. doi:10.1016/J.RENENE.2019.05.055
Gürtürk, M., and Kok, B. (2020). A New Approach in the Design of Heat Transfer Fin for Melting and Solidification of PCM. Int. J. Heat Mass Transf. 153, 119671. doi:10.1016/J.IJHEATMASSTRANSFER.2020.119671
He, Y., Yuan, W., Cao, J., Li, L., and Ling, Z. (2022). Compact Latent Heat Exchanger for the Fast Supply of Hot Water with Serpentine Tubes in Shape-Stabilized Composite Phase Change Material and Auxiliary Electric Heating. Appl. Therm. Eng. 211, 118469. doi:10.1016/j.applthermaleng.2022.118469
Huang, Y., Sun, Q., Yao, F., and Zhang, C. (2021). Experimental Study on the Thermal Performance of a Finned Metal Foam Heat Sink with Phase Change Material. Heat. Transf. Eng. 42, 579–591. doi:10.1080/01457632.2020.1716482
Lee, D., Jeong, H. J., Ji, H. Y., Park, S. Y., Chung, D.-Y., Kang, C., et al. (2022). Peak Load Shifting Control on Hot Water Supplied from District Heating Using Latent Heat Storage System in Apartment Complex. Case Stud. Therm. Eng. 34, 101993. doi:10.1016/J.CSITE.2022.101993
Lin, W., Ling, Z., Zhang, Z., and Fang, X. (2020). Experimental and Numerical Investigation of Sebacic Acid/expanded Graphite Composite Phase Change Material in a Double-Spiral Coiled Heat Exchanger. J. Energy Storage 32, 101849. doi:10.1016/j.est.2020.101849
Liu, G., Xiao, T., Guo, J., Wei, P., Yang, X., and Hooman, K. (2022). Melting and Solidification of Phase Change Materials in Metal Foam Filled Thermal Energy Storage Tank: Evaluation on Gradient in Pore Structure. Appl. Therm. Eng. 212, 118564. doi:10.1016/J.APPLTHERMALENG.2022.118564
Liu, X., Chen, Y., and Shi, M. (2013). Dynamic Performance Analysis on Start-Up of Closed-Loop Pulsating Heat Pipes (CLPHPs). Int. J. Therm. Sci. 65, 224–233. doi:10.1016/J.IJTHERMALSCI.2012.10.012
Zhang, C., Li, J., and Chen, Y. (2020). Improving the Energy Discharging Performance of a Latent Heat Storage (LHS) Unit Using Fractal-Tree-Shaped Fins. Appl. Energy 259, 114102. doi:10.1016/J.APENERGY.2019.114102
Zhang, G., Guo, Y., Liu, Z., Lu, W., Yan, X., and Du, Y. (2021). Experimental and Numerical Investigation of Composite Phase Change Materials for Building Energy Saving. Front. Energy Res. 9. doi:10.3389/fenrg.2021.721487
Zhang, P., Xiao, X., and Ma, Z. W. (2016). A Review of the Composite Phase Change Materials: Fabrication, Characterization, Mathematical Modeling and Application to Performance Enhancement. Appl. Energy 165, 472–510. doi:10.1016/J.APENERGY.2015.12.043
Keywords: latent heat storage technology, thermal management of buildings, PCM, heat storage device, heating system
Citation: Zhao T, Yao F and Gulfam R (2022) Opinion on Latent Heat-Based Heat Exchangers Equipped With Thermal Management Systems of Buildings. Front. Energy Res. 10:951085. doi: 10.3389/fenrg.2022.951085
Received: 23 May 2022; Accepted: 09 June 2022;
Published: 22 June 2022.
Edited by:
Xiao Chen, Beijing Normal University, ChinaReviewed by:
Hongyi Gao, University of Science and Technology Beijing, ChinaCopyright © 2022 Zhao, Yao and Gulfam. This is an open-access article distributed under the terms of the Creative Commons Attribution License (CC BY). The use, distribution or reproduction in other forums is permitted, provided the original author(s) and the copyright owner(s) are credited and that the original publication in this journal is cited, in accordance with accepted academic practice. No use, distribution or reproduction is permitted which does not comply with these terms.
*Correspondence: Raza Gulfam, Z3VsZmFtQHNldS5lZHUuY24=, Z3VsZmFtcmF6YWhhaWRlcnlAaG90bWFpbC5jb20=
Disclaimer: All claims expressed in this article are solely those of the authors and do not necessarily represent those of their affiliated organizations, or those of the publisher, the editors and the reviewers. Any product that may be evaluated in this article or claim that may be made by its manufacturer is not guaranteed or endorsed by the publisher.
Research integrity at Frontiers
Learn more about the work of our research integrity team to safeguard the quality of each article we publish.