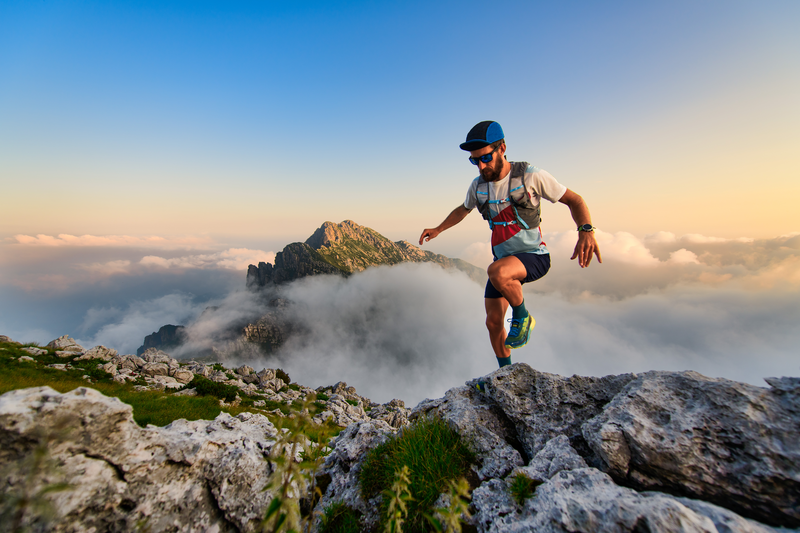
94% of researchers rate our articles as excellent or good
Learn more about the work of our research integrity team to safeguard the quality of each article we publish.
Find out more
OPINION article
Front. Energy Res. , 13 June 2022
Sec. Process and Energy Systems Engineering
Volume 10 - 2022 | https://doi.org/10.3389/fenrg.2022.935100
Thermal energy is stored in a packed bed thermal energy storage (PBTES) system by raising the temperature of the packing elements. This simple mechanism and economic feasibility (Gautam and Saini, 2020) make the PBTES promising for applications such as solar thermal power plants, building cooling systems, and waste heat recovery (Nallusamy et al., 2007). The traditional PBTES is based on sensible heat transfer and is therefore limited by a low energy density and temperature stability (Mawire et al., 2020). Compared with the traditional system, a packed bed latent heat storage (PBLHS) system based on phase-change materials (PCMs) (Wang H. et al., 2018; Wang J. et al., 2018) offers advantages such as a higher energy density, higher temperature stability, and few safety issues (Li et al., 2018; Kumar and Saha, 2021; Wang et al., 2021) and therefore is considered a promising solution for thermal energy storage (Yang et al., 2017; Zhang et al., 2020; Grabo et al., 2021).
This study reviews recent progress on low-temperature PBLHS systems with phase-change points below 100°C. A brief discussion of the working principle and impact factors of the PBLHS system is presented based on results from recent studies (Alptekin and Ezan, 2021). The performance and applications of PBLHS systems are also discussed.
A typical low-temperature PBLHS system (Liu and Zhao, 2021; He et al., 2022) consists of a heat storage tank, a heat transfer fluid (HTF), and heat storage media (HSM). The structure of a PBLHS system is shown in Figure 1A. Capsules containing low-temperature PCMs constitute the HSM, which is supported by a screen in the lower portion of the heat storage tank. The heat storage tank is surrounded by a layer of insulation material to reduce thermal energy loss. During the charging and discharging process, the HTF percolates through the HSM (Singh et al., 2010). The HSM packing is a porous medium with a high surface-to-volume ratio at the macroscopic level, which enhances heat transfer (Yang et al., 2019; Yang et al., 2020). Heat transfer between the HSM and HTF enables the charging and discharging of the PBLHS system (Guo et al., 2021). The buoyancy effect causes the high-temperature HTF to enter the top of the heat storage tank during the charging process (Zanganeh et al., 2012). The HSM near the inlet is heated first. The temperature gradient across the HSM from the inlet to the outlet results in the lower portion of the HSM also being heated by the upper portion of the HSM (Qin et al., 2012). During the discharging process, the thermal energy stored in the HSM is released by heat transfer between the low-temperature HTF and the high-temperature HSM.
FIGURE 1. Structure and applications of a low-temperature PBLHS system. (A) Structure; (B) Applications.
HSMs in the form of spherical capsules have been found to exhibit superior thermohydraulic performance (Singh et al., 2013). In a low-temperature PBLHS system, the HSM consists of spherical capsules filled with PCMs, such as paraffin (Nallusamy et al., 2007; Wang et al., 2017), water (Fang et al., 2010), n-tetradecane (Wu et al., 2010), and composite materials (Cheng and Zhai, 2018). The macro-encapsulated PCMs can be made by filling a pre-made shell with liquid PCM (Liu et al., 2018). The micro-encapsulated PCMs can be fabricated by physical, chemical, or physicochemical methods, that is, spray drying (Alva et al., 2017), interfacial polymerization (Milián et al., 2017), and droplet microfluidics (Chen et al., 2013; Han et al., 2020). The use of large PCM capsules results in poor thermal performance and low energy loss. However, small PCM capsules induce a large pressure drop across the HTF (Li et al., 2018; Alptekin and Ezan, 2021). Therefore, the PCM capsule size should be determined based on a comprehensive evaluation of the thermal performance and pumping power of the PBLHS system (Pakrouh et al., 2017).
Both liquids and gases, that is, water (Cheng and Zhai, 2018), ethylene glycol (Fang et al., 2010), and air (Arfaoui et al., 2017), can be used as the HTF in the PBLHS system. A liquidus HTF has a large heat transfer ecoefficiency and can be used to fabricate a PBLHS system with a high charging/discharging rate (Felix Regin et al., 2009). However, the large flow resistance of a liquidus HTF produces a large pressure drop across the PBLHS system. Air is typically used as a gaseous HTF because of its low cost and high-temperature resistance. However, the low thermal conductivity of a gaseous HTF results in a low charging/discharging rate for the PBLHS system. Thus, a HTF should be selected by considering the influence factors for the system, including the HTF cost, estimated charging/discharging rate of the PBLHS system, and pumping power (de Gracia and Cabeza, 2017).
The various factors affecting the performance of the PBLHS system can be categorized into structural and material factors. As a PBLHS system can be treated as a porous medium at the macroscopic level, the effect of the packing structure determines the heat transfer between the HSM and the HTF, as well as the pressure drop in the PBLHS system (Deng et al., 2017). In order to maintain a balance between the heat transfer and pressure drop, the size and aspect ratio of the HSM should be optimized. A high void fraction, that is, a high ratio of the volume of the voids in the bed to the total bed volume, enhances the thermal conductivity of the HSM but induces a large pressure drop across the PBLHS system.
The thermal properties of the materials in the PBLHS system determine the heat transfer in the system, including convection and conduction. Specifically, heat transfer within the PBLHS system combines conduction between the wall and HSM, convection between the HSM and HTF, convection between the wall surface and HTF, and even radiation from the HSM. The effective thermal conductivity is used as a comprehensive measure of the heat transfer within the PBLHS system.
Energy efficiency is typically used to evaluate the energy storage performance of a PBLHS system and is expressed as the ratio between the energy recovered from the PBLHS system and the energy delivered to the PBLHS system (Yang and Garimella, 2010). Arfaoui et al. used a PBLHS system with air as the HTF and CaCl26H2O as the PCM to increase the efficiency of a solar air collector. The maximum absorbed energy reached 730 kJ, and the daily energy efficiency was approximately 47% Arfaoui et al. (2017).
However, irreversible losses were not considered in the energy analysis. The exergy efficiency, the ratio of the total exergy recovered from the PBLHS system to the total exergy delivered to the PBLHS system, is preferred as a measure of the comprehensive system performance (Saha and Das, 2020; Mawire et al., 2021). Cheng et al. found that the exergy efficiency decreases from 83.1% to 79.4% as the capsule size increases from 9.5 mm to 47.5 mm (Cheng and Zhai, 2018).
As applications of the PBLHS system are sometimes limited by the large capital investment involved, it is important to consider the cost of a PBLHS system. The levelized cost of electricity (LCOE) is the most commonly used indicator of the economic feasibility of a PBLHS system. Tehrani et al. performed a techno-economic comparative analysis on a PBLHS system and a shell-and-tube latent storage system based on the thermal storage capacity for a 19.9 MWe solar power plant. The cost of a PBLHS system with a wall thickness of no more than 0.1 mm is ∼10 US$ kWhth Mostafavi Tehrani et al. (2019).
A low-temperature PBLHS system can be integrated with residential heating (He et al., 2019b; Xu et al., 2020), greenhouse heating (Baddadi et al., 2019), and solar collectors (Desai et al., 2022). Baddadi et al. (2019)designed a greenhouse with a PBLHS system, utilizing CaCl2.6H2O as the PCM and air as the HTF. The system was found to enhance the greenhouse microclimate. Cheng and Zhai (2018) reported a cascaded packed bed based on cool thermal energy storage, with water and a composite material (C-L/O) as the HTF and PCM, respectively. The most efficient 24-stage unit had a 15.1% lower charging time than a single-stage unit and a similar thermal performance to a 3–5 stage system. He et al. (2019a) designed a PBLHS system integrated with a water tank to enhance the electric power load regulation capacity and the heat-supply capacity. The energy storage capacity increased by 29.62% theoretically. Wu et al. (2022) also discussed the application and optimization of the PBLHS system in hot-water supply, theoretically and experimentally. The system has better thermal stratification when the PCM units are placed at a higher place and near the inlet of the high-temperature water, and the flow rate is 3 L/min. The PBLHS system can also be used for the drying process (Atalay, 2020), with an average energy efficiency of 68.55%.
This study presents a brief introduction to the structure and the working principles of the PBLHS system. The impact factors and performance of the PBLHS system have been discussed. The packing structure of a PBLHS system significantly affects thermal performance. The PBLHS system performance is commonly evaluated using the energy efficiency and exergy efficiency, between which the exergy efficiency is more accurate and therefore preferred. In addition, applications of low-temperature PBLHS systems, especially for buildings, are introduced. However, a few studies have been carried out on the economic aspects of low-temperature PBLHS systems. Further investigations on optimizing the design and economic feasibility of low-temperature PBLHS systems are encouraged.
CZ and SW developed the concept of the study. DC wrote the first draft of the manuscript. CY revised the manuscript. All authors revised the manuscript and read and approved the submitted version.
This study was supported by the Natural Science Foundation of Jiangsu Province (Grant no. BK20210239) and the Postgraduate Research & Practice Innovation Program of Jiangsu Province (Grant no. SJCX22_1738).
The authors declare that the research was conducted in the absence of any commercial or financial relationships that could be construed as a potential conflict of interest.
All claims expressed in this article are solely those of the authors and do not necessarily represent those of their affiliated organizations or those of the publisher, the editors, and the reviewers. Any product that may be evaluated in this article, or claim that may be made by its manufacturer, is not guaranteed or endorsed by the publisher.
Alptekin, E., and Ezan, M. A. (2021). A Systematic Assessment on a Solar Collector Integrated Packed-Bed Single/multi-Layered Latent Heat Thermal Energy Storage System. J. Energy Storage 37, 102410. doi:10.1016/j.est.2021.102410
Alva, G., Lin, Y., Liu, L., and Fang, G. (2017). Synthesis, Characterization and Applications of Microencapsulated Phase Change Materials in Thermal Energy Storage: A Review. Energy Build. 144, 276–294. doi:10.1016/j.enbuild.2017.03.063
Arfaoui, N., Bouadila, S., and Guizani, A. (2017). A Highly Efficient Solution of Off-Sunshine Solar Air Heating Using Two Packed Beds of Latent Storage Energy. Sol. Energy 155, 1243–1253. doi:10.1016/j.solener.2017.07.075
Atalay, H. (2020). Assessment of Energy and Cost Analysis of Packed Bed and Phase Change Material Thermal Energy Storage Systems for the Solar Energy-Assisted Drying Process. Sol. Energy 198, 124–138. doi:10.1016/j.solener.2020.01.051
Baddadi, S., Bouadila, S., and Guizani, A. (2019). Beneficial Use of Two Packed Beds of Latent Storage Energy for the Heating of a Hydroponic Greenhouse. Energy Procedia 162, 156–163. doi:10.1016/j.egypro.2019.04.017
Chen, Y., Liu, X., and Shi, M. (2013). Hydrodynamics of Double Emulsion Droplet in Shear Flow. Appl. Phys. Lett. 102 (5), 051609. doi:10.1063/1.4789865
Cheng, X., and Zhai, X. (2018). Thermal Performance Analysis and Optimization of a Cascaded Packed Bed Cool Thermal Energy Storage Unit Using Multiple Phase Change Materials. Appl. Energy 215, 566–576. doi:10.1016/j.apenergy.2018.02.053
de Gracia, A., and Cabeza, L. F. (2017). Numerical Simulation of a PCM Packed Bed System: A Review. Renew. Sustain. Energy Rev. 69, 1055–1063. doi:10.1016/j.rser.2016.09.092
Deng, Z., Liu, X., Zhang, C., Huang, Y., and Chen, Y. (2017). Melting Behaviors of PCM in Porous Metal Foam Characterized by Fractal Geometry. Int. J. Heat Mass Transf. 113, 1031–1042. doi:10.1016/j.ijheatmasstransfer.2017.05.126
Desai, N. B., Mondejar, M. E., and Haglind, F. (2022). Techno-economic Analysis of Two-Tank and Packed-Bed Rock Thermal Energy Storages for Foil-Based Concentrating Solar Collector Driven Cogeneration Plants. Renew. Energy 186, 814–830. doi:10.1016/j.renene.2022.01.043
Fang, G., Wu, S., and Liu, X. (2010). Experimental Study on Cool Storage Air-Conditioning System with Spherical Capsules Packed Bed. Energy Build. 42 (7), 1056–1062. doi:10.1016/j.enbuild.2010.01.018
Felix Regin, A., Solanki, S. C., and Saini, J. S. (2009). An Analysis of a Packed Bed Latent Heat Thermal Energy Storage System Using PCM Capsules: Numerical Investigation. Renew. Energy 34 (7), 1765–1773. doi:10.1016/j.renene.2008.12.012
Gautam, A., and Saini, R. P. (2020). A Review on Technical, Applications and Economic Aspect of Packed Bed Solar Thermal Energy Storage System. J. Energy Storage 27, 101046. doi:10.1016/j.est.2019.101046
Grabo, M., Acar, E., and Kenig, E. Y. (2021). Modeling and Improvement of a Packed Bed Latent Heat Storage Filled with Non-spherical Encapsulated PCM-Elements. Renew. Energy 173, 1087–1097. doi:10.1016/j.renene.2021.04.022
Guo, W., He, Z., Meng, Z., and Zhang, P. (2021). A Comprehensive Investigation of the Mathematical Models for a Packed Bed Latent Heat Thermal Energy Storage System. Int. J. Energy Res. 45 (10), 15005–15021. doi:10.1002/er.6780
Han, X., Kong, T., Zhu, P., and Wang, L. (2020). Microfluidic Encapsulation of Phase-Change Materials for High Thermal Performance. Langmuir 36 (28), 8165–8173. doi:10.1021/acs.langmuir.0c01171
He, X., Qiu, J., Wang, W., Hou, Y., Ayyub, M., and Shuai, Y. (2022). A Review on Numerical Simulation, Optimization Design and Applications of Packed-Bed Latent Thermal Energy Storage System with Spherical Capsules. J. Energy Storage 51, 104555. doi:10.1016/j.est.2022.104555
He, Z., Wang, X., Du, X., Amjad, M., Yang, L., and Xu, C. (2019a). Experiments on Comparative Performance of Water Thermocline Storage Tank with and without Encapsulated Paraffin Wax Packed Bed. Appl. Therm. Eng. 147, 188–197. doi:10.1016/j.applthermaleng.2018.10.051
He, Z., Wang, X., Du, X., Xu, C., and Yang, L. (2019b). Cyclic Characteristics of Water Thermocline Storage Tank with Encapsulated PCM Packed Bed. Int. J. Heat Mass Transf. 139, 1077–1086. doi:10.1016/j.ijheatmasstransfer.2019.05.093
Kumar, A., and Saha, S. K. (2021). Performance Analysis of a Packed Bed Latent Heat Thermal Energy Storage with Cylindrical‐shaped Encapsulation. Int. J. Energy Res. 45 (9), 13130–13148. doi:10.1002/er.6639
Li, M.-J., Jin, B., Yan, J.-J., Ma, Z., and Li, M.-J. (2018). Numerical and Experimental Study on the Performance of a New Two-Layered High-Temperature Packed-Bed Thermal Energy Storage System with Changed-Diameter Macro-Encapsulation Capsule. Appl. Therm. Eng. 142, 830–845. doi:10.1016/j.applthermaleng.2018.07.026
Liu, H. B., and Zhao, C. Y. (2021). Effect of Radial Porosity Oscillation on the Thermal Performance of Packed Bed Latent Heat Storage. Engineering 7 (4), 515–525. doi:10.1016/j.eng.2020.05.020
Liu, Z., Yu, Z., Yang, T., Qin, D., Li, S., Zhang, G., et al. (2018). A Review on Macro-Encapsulated Phase Change Material for Building Envelope Applications. Build. Environ. 144, 281–294. doi:10.1016/j.buildenv.2018.08.030
Mawire, A., Ekwomadu, C. S., and Shobo, A. B. (2021). Experimental Charging Characteristics of Medium-Temperature Cascaded Packed Bed Latent Heat Storage Systems. J. Energy Storage 42, 103067. doi:10.1016/j.est.2021.103067
Mawire, A., Lefenya, T. M., Ekwomadu, C. S., and Shobo, A. B. (2020). Performance of a Medium Temperature Eutectic Solder Packed Bed Latent Heat Storage System for Domestic Applications. J. Energy Storage 28, 101294. doi:10.1016/j.est.2020.101294
Milián, Y. E., Gutiérrez, A., Grágeda, M., and Ushak, S. (2017). A Review on Encapsulation Techniques for Inorganic Phase Change Materials and the Influence on Their Thermophysical Properties. Renew. Sustain. Energy Rev. 73, 983–999. doi:10.1016/j.rser.2017.01.159
Mostafavi Tehrani, S. S., Shoraka, Y., Nithyanandam, K., and Taylor, R. A. (2019). Shell-and-tube or Packed Bed Thermal Energy Storage Systems Integrated with a Concentrated Solar Power: A Techno-Economic Comparison of Sensible and Latent Heat Systems. Appl. Energy 238, 887–910. doi:10.1016/j.apenergy.2019.01.119
Nallusamy, N., Sampath, S., and Velraj, R. (2007). Experimental Investigation on a Combined Sensible and Latent Heat Storage System Integrated with Constant/varying (Solar) Heat Sources. Renew. Energy 32 (7), 1206–1227. doi:10.1016/j.renene.2006.04.015
Pakrouh, R., Hosseini, M. J., Ranjbar, A. A., and Bahrampoury, R. (2017). Thermodynamic Analysis of a Packed Bed Latent Heat Thermal Storage System Simulated by an Effective Packed Bed Model. Energy 140, 861–878. doi:10.1016/j.energy.2017.08.055
Qin, F. G. F., Yang, X., Ding, Z., Zuo, Y., Shao, Y., Jiang, R., et al. (2012). Thermocline Stability Criterions in Single-Tanks of Molten Salt Thermal Energy Storage. Appl. Energy 97, 816–821. doi:10.1016/j.apenergy.2012.02.048
Saha, S. K., and Das, R. B. (2020). Exergetic and Performance Analyses of Two‐layered Packed Bed Latent Heat Thermal Energy Storage System. Int. J. Energy Res. 44 (3), 2208–2225. doi:10.1002/er.5081
Singh, H., Saini, R. P., and Saini, J. S. (2010). A Review on Packed Bed Solar Energy Storage Systems. Renew. Sustain. Energy Rev. 14 (3), 1059–1069. doi:10.1016/j.rser.2009.10.022
Singh, H., Saini, R. P., and Saini, J. S. (2013). Performance of a Packed Bed Solar Energy Storage System Having Large Sized Elements with Low Void Fraction. Sol. Energy 87, 22–34. doi:10.1016/j.solener.2012.10.004
Wang, F., Dong, Y., Li, Y., Xu, J., and Zhang, G. (2021). Numerical Study on the Thermal Performance of Packed-Bed Latent Heat Thermal Energy Storage System with Biomimetic Alveoli Structure Capsule. Sci. China Technol. Sci. 64 (7), 1544–1554. doi:10.1007/s11431-020-1769-9
Wang, H., Zhao, Z., Liu, Y., Shao, C., Bian, F., and Zhao, Y. (2018a). Biomimetic Enzyme Cascade Reaction System in Microfluidic Electrospray Microcapsules. Sci. Adv. 4 (6), eaat2816. doi:10.1126/sciadv.aat2816
Wang, J., Gao, W., Zhang, H., Zou, M., Chen, Y., and Zhao, Y. (2018b). Programmable Wettability on Photocontrolled Graphene Film. Sci. Adv. 4 (9), eaat7392. doi:10.1126/sciadv.aat7392
Wang, J., Sun, L., Zou, M., Gao, W., Liu, C., Shang, L., et al. (2017). Bioinspired Shape-Memory Graphene Film with Tunable Wettability. Sci. Adv. 3 (6), e1700004. doi:10.1126/sciadv.1700004
Wu, F., Wang, Z., Zhang, H., Qin, Y., You, X., and Lu, J. (2022). Experimental and Simulation Analysis on Thermal Stratification Characteristics in Solar Storage Tanks with Phase Change Materials. J. Energy Storage 46, 103722. doi:10.1016/j.est.2021.103722
Wu, S., Fang, G., and Liu, X. (2010). Thermal Performance Simulations of a Packed Bed Cool Thermal Energy Storage System Using N-Tetradecane as Phase Change Material. Int. J. Therm. Sci. 49 (9), 1752–1762. doi:10.1016/j.ijthermalsci.2010.03.014
Xu, T., Humire, E. N., Chiu, J. N.-W., and Sawalha, S. (2020). Numerical Thermal Performance Investigation of a Latent Heat Storage Prototype toward Effective Use in Residential Heating Systems. Appl. Energy 278, 115631. doi:10.1016/j.apenergy.2020.115631
Yang, X., Feng, S., Zhang, Q., Chai, Y., Jin, L., and Lu, T. J. (2017). The Role of Porous Metal Foam on the Unidirectional Solidification of Saturating Fluid for Cold Storage. Appl. Energy 194, 508–521. doi:10.1016/j.apenergy.2016.09.050
Yang, X., Yu, J., Guo, Z., Jin, L., and He, Y.-L. (2019). Role of Porous Metal Foam on the Heat Transfer Enhancement for a Thermal Energy Storage Tube. Appl. Energy 239, 142–156. doi:10.1016/j.apenergy.2019.01.075
Yang, X., Yu, J., Xiao, T., Hu, Z., and He, Y.-L. (2020). Design and Operating Evaluation of a Finned Shell-And-Tube Thermal Energy Storage Unit Filled with Metal Foam. Appl. Energy 261, 114385. doi:10.1016/j.apenergy.2019.114385
Yang, Z., and Garimella, S. V. (2010). Thermal Analysis of Solar Thermal Energy Storage in a Molten-Salt Thermocline. Sol. Energy 84 (6), 974–985. doi:10.1016/j.solener.2010.03.007
Zanganeh, G., Pedretti, A., Zavattoni, S., Barbato, M., and Steinfeld, A. (2012). Packed-bed Thermal Storage for Concentrated Solar Power - Pilot-Scale Demonstration and Industrial-Scale Design. Sol. Energy 86 (10), 3084–3098. doi:10.1016/j.solener.2012.07.019
Keywords: thermal energy storage, packed bed, latent heat, low-temperature, phase-change materials
Citation: Cao D, Yu C, Wu S and Zhang C (2022) Perspectives on Low-Temperature Packed Bed Latent Heat Storage Systems. Front. Energy Res. 10:935100. doi: 10.3389/fenrg.2022.935100
Received: 03 May 2022; Accepted: 09 May 2022;
Published: 13 June 2022.
Edited by:
Xiaohu Yang, Xi’an Jiaotong University, ChinaReviewed by:
Hao Peng, Nanjing Tech University, ChinaCopyright © 2022 Cao, Yu, Wu and Zhang. This is an open-access article distributed under the terms of the Creative Commons Attribution License (CC BY). The use, distribution or reproduction in other forums is permitted, provided the original author(s) and the copyright owner(s) are credited and that the original publication in this journal is cited, in accordance with accepted academic practice. No use, distribution or reproduction is permitted which does not comply with these terms.
*Correspondence: Suchen Wu, c3VjaGVud3VAc2V1LmVkdS5jbg==
Disclaimer: All claims expressed in this article are solely those of the authors and do not necessarily represent those of their affiliated organizations, or those of the publisher, the editors and the reviewers. Any product that may be evaluated in this article or claim that may be made by its manufacturer is not guaranteed or endorsed by the publisher.
Research integrity at Frontiers
Learn more about the work of our research integrity team to safeguard the quality of each article we publish.