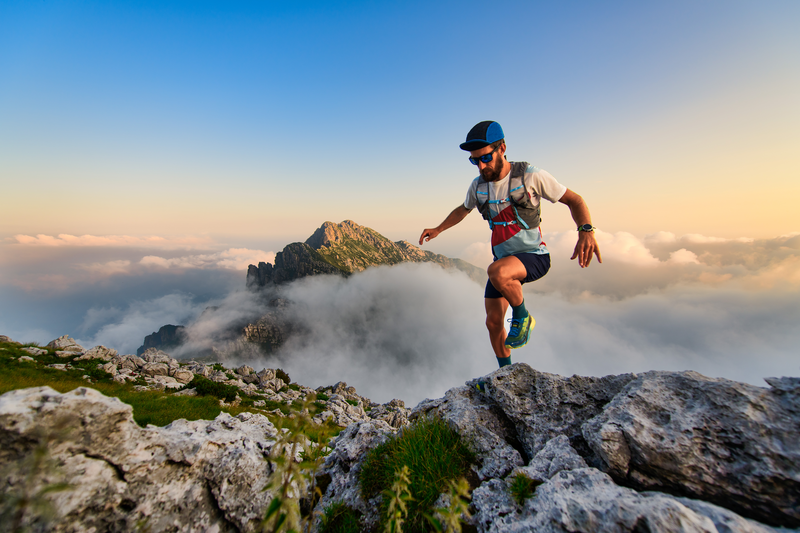
95% of researchers rate our articles as excellent or good
Learn more about the work of our research integrity team to safeguard the quality of each article we publish.
Find out more
BRIEF RESEARCH REPORT article
Front. Energy Res. , 23 May 2022
Sec. Smart Grids
Volume 10 - 2022 | https://doi.org/10.3389/fenrg.2022.920654
This article is part of the Research Topic Increasing Penetration of Renewable Sources in Power Systems: Opportunities and Challenges View all 18 articles
Parts of this article's content have been modified or rectified in:
Erratum: Over-voltage regulation of distribution networks by coordinated operation of PV inverters and demand side management program
The increase of Photovoltaics (PV) units’ penetration factor in the power grids might create overvoltage over the network buses. The active power curtailment (APC) and the reactive power provision methods use inverters to regulate their output active and reactive powers for high PV-penetrated grids. However, the mentioned solutions would reduce the maximum injectable active solar power to the grid, not financially acceptable. Continuous employment of the maximum apparent power capacity of the inverters will practically decrease the inverters’ lifetime, require special design considerations, and make the control system complex. To overcome those issues, a feasible solution would be increasing the load consumption within the time intervals in which the grid faces the over-voltage problem. In this research, the demand response (DR) program is employed. Load shifting techniques are exerted to move a portion of loads from the peak hours to when further power consumption is expected for voltage level reduction purposes. A new long-term strategy based on the coordinated operation of the PV inverters and load shifting techniques is proposed to resolve the over-voltage issue in the network. Consequently, the PV inverter’s contribution to voltage control is reduced; a new sight of DR potential is implemented, and also the under-voltage level in peak times is decreased significantly.
Over recent decades, the aim was to install more PV units, especially PV farms, to address the global warming issue and come up with clean energy. The PV electricity generation cost has been considerably reduced, and the PV units have been employed in a wide range of applications (Wongsaichua et al., 2004). However, the high penetration factor of the PV systems leads to critical challenges in power quality (e.g., over-voltage) (Yang et al., 2020).
In high PV-penetrated power systems, the PV generators may cause to flow reverse current in grids’ lines, and then nodal overvoltage might appear. Researchers have used two general techniques, namely the active power curtailment (APC) and reactive power control of PV inverters, to mitigate the overvoltage challenge. Application of the APC techniques (which reduce the solar power generation) and reactive power consumption by the inverters can decrease the nodal voltages down to the acceptable ranges. Many significant efforts have been performed to address the over-voltage issue emerging from high active power injection from renewable sources (Yeh et al., 2012; Kryonidis et al., 2016; Molina-García et al., 2016; Li et al., 2017; Ciocia et al., 2018;; Singhal et al., 2018; Couraud et al., 2019; Fatama et al., 2020; Joseph et al., 2020). A study by (Singhal et al., 2018), has focused on implementing an adaptive volt/Var control system for the PV inverters to resolve the steady-state voltage deviation and improve the system’s response to external faults. In work done by (Ciocia et al., 2018), three different approaches have been recommended and practiced to utilize the voltage control devices and employ a static voltage controller (SVC) instead of a On-Load Tap Changer (OLTC). The authors of (Kryonidis et al., 2016) have proposed a decentralized control strategy to minimize the power losses and provide a fast response in the voltage regulation. Researchers have tried to earn revenue from the participation of the PV inverters in the ancillary services market (Karbouj et al., 2020). A three-level control system consisting of power, voltage, and current control loops has been recommended by (Molina-García et al., 2016) for the PV inverters to overcome the over-voltage issues and the power flow convergence problems. Also, a modified voltage-reactive power curve and a power flow routine have been presented to prevent time-consuming calculations for determining the nodes’ voltage and lines’ current. The utilization of a transformer with tap changing capability, switched capacitor banks, and distributed generation units have also been discussed by (Joseph et al., 2020) to improve the voltage quality. However, this method will reduce the transformer’s lifetime and requires expensive switched capacitors. The over-voltage and under-voltage challenges are expected in high PV-penetrated power systems under cloudy conditions. Therefore, solutions have been proposed by (Li et al., 2017) to mitigate those challenges by regulating the active and reactive power values of the PV inverters. Unbalanced grid voltage conditions can be mitigated by the coordinated operation of distributed inverters and other power quality devices to improve voltage unbalance condition (Peng et al., 2020).
A precise analysis of the literature shows that the APC techniques and reactive power control of PV inverters have been commonly practiced to overcome the over-voltage issue in different conditions. However, relying on those methods will reduce the voltage control freedom. Furthermore, they will initiate other technical problems for the PV inverters and impose a more economic burden on the owners. The reactive power injection/absorption by PV inverters will increase the required Volt-Ampere (VA) of the inverters. Therefore, the size of the inverters would be increased, advanced cooling systems would be required, and investment costs would be increased. In addition, injection of the reactive power through the inverters may potentially decrease their lifetime because of the implied current stresses (Anurag et al., 2015). Employing APC techniques would lead to solar power spillage and would reduce the financial benefits. Also, the network operators should pay a penalty cost to the PV units’ owners for their contribution to the voltage regulation. Therefore, it is essential to reduce the PV inverter’s contributions to improve voltage quality issues.
This study proposes a new technique considering DR program to address the discussed challenges. This letter’s main contribution is proposing simultaneous and coordinated employment of the DR programs and reactive/active power regulation of the PV inverters to improve the voltage quality of the network. Nevertheless, DR techniques have been employed by researchers (Aghaei et al., 2016; Dong et al., 2017; Yao et al., 2019; Antonopoulos et al., 2020; Barik and Das 2020; Li et al., 2020; Xie et al., 2020) to cover different objectives such as voltage stability, short-term voltage stability, and long/short term voltage quality. All mentioned works have been implemented without considering Distributed Generators (DGs) and PV units’ contribution to the voltage support. Also, Electric Vehicles (EVs) have been considered additional tools to improve the voltage quality (Prabawa and Choi, 2021; Dutta et al., 2021; Pournazarian et al., 2019). However, EVs can mainly address dynamic voltage deviations quickly, while utility operators prefer to adopt a long-term voltage control strategy. The literature has not yet studied the DR potential for reducing the PV system’s contribution to voltage control. To be more precise, the aim is to overcome the over-voltage issues of distribution networks by proposing a coordinated operation of DR of loads and APC and reactive power regulation of PV farms. The effective performance of the proposed methodology is examined over the IEEE 33 bus distributed network.
The proposed strategy is introduced in section 2. The criteria, fitness functions, and the case study with its characteristics are presented in section 3. To assess the proposed strategy, simulations are conducted and their results are discussed in Section 4. Finally, section 5 is dedicated to highlighting the main conclusion.
The derivation steps of the proposed methodology are shown in Figure 1. In Figure 1A, the over-voltage issue is clear around noontime due to high PV power generation. In consequence, a real power curtailment has been applied to the PV farm inverters, and reactive power compensation has been applied to mitigate the over-voltage issue, see Figure 1B. Different reactive control methods can also be applied over the PV inverters during the over-voltage conditions, such as adjusting the reactive power considering IEEE 1547–2018. A relatively high APC level has been applied, and the PV inverters have regulated their reactive power generation to mitigate the over-voltage issue.
FIGURE 1. The derivation steps of the proposed methodology: (A) Without voltage support by PV inverters and without implementing the DR program, (B) conventional voltage support by PV inverters and without implementing the DR program, and (C) the proposed strategy for coordinated operation of PV inverters and DR program.
The main challenges of the contribution of PV inverters in voltage control without employing a suitable DR program can be inferred from Figure 1B. In more detail:
• Increasing the reactive power generation of inverters will increase the required VA rates for the PV inverters, and then investment costs would be increased.
• The clipped active power, which is indicated by P1, is very significant; considerable financial losses would be incurred because of employing APC methods.
In the proposed technique, shifting load from the peak times to times in which the PV farm injects high power to the grid (in our case: around noon time) would be an effective approach. It is assumed that the customers allow the network operator to shift a certain percentage of their power consumption from peak times to off-peak times using DR programs. Hence, Figure 1C shows the voltage profile of the grid employing the coordinated operation of PV inverters and DR programs where the over-voltage issue is eliminated. The load-shifting (LSH) technique is selected to implement the DR program. As a result, the PV inverters’ levels of active power curtailment and reactive power generation are reduced. Another advantage would be decreasing the under-voltage issue during peak times which this support is highlighted in Figure 1C.
Considering the discussion above, reactive power support by inverters is still needed in power grids, but its level can be significantly reduced. In this context, a regulation loop should be implemented in the control software of the PV inverters to regulate its reactive power (Q) as a function of grid conditions [voltage level (V)]. The IEEE Std. 1,547 has defined the required Q-V function for the PV inverters, see Figure 1B.
In this part, the DR concept is discussed. Nowadays, loads’ behavior can be predicted with high accuracy. In DR programs, customers contribute voluntarily, contract-based and mandatory-based in some emergency conditions in power consumption patterns. Therefore, there is little concern about unpredictability and uncontrollability from users’ perspectives. Figure 2 clearly shows the DR concept and Eqs 1–3 try to express this concept. It is assumed that customers allow the network operator to be able to shift certain percentages of their power consumption from peak times to non-peak times. The final load at each time after participation in DR programs may be more or less than the base load. Figure 2; Eqs 1–3,
This section aims to clarify the implementation process of the proposed strategy. As discussed in this paper, the contribution of PV units in voltage control means owners of PV farms would lose some revenue due to applying the APC technique, which must be compensated by the grid owner. On the hand, the owner of the grid must pay PV unit owners for their contribution to reactive power control as an ancillary service. To reduce the mentioned costs, it is proposed to employ DR programs in this research. Shifting the loads from peak times to off-peak times is an incentive program and induces costs. However, by implementing optimum schedules, an optimum solution can be found in which the total penalty cost allocated to the owner of the grid would be the lowest. In general, different fitness functions, optimization algorithms, scenarios, and load profiles can be considered to examine the performance of the proposed strategy. But, this study tries to simply implement the proposed strategy. In this context, three fitness functions are first defined.
The costs of the contribution of the PV inverters in voltage regulation can be expressed as:
where,
where CPL-h is the daily price of electricity determined by the distribution network owner for each time interval. In this step, a multi-objective optimization problem is indicated. One of the techniques to simplify multiple objective problems is aggregating all objectives in one expression by assigning a certain weight factor to each objective:
where, ωi is the weighted factor for the ith fitness function It is worthy of mentioning that, concerning the operator’s aims and priorities, certain weight factors can be defined to realize the power quality requirement and maximize the operator’s profit simultaneously.
The IEEE 33-bus, as a test platform, is selected to evaluate the proposed technique. This system is a Medium Voltage (MV) distribution and a suitable network for grid-connected large-scale PV farms. It has a radial feature to enlarge the effect of high PV penetration. The single-line diagram of the case study is illustrated in Figure 3. Since the MW sized and GW sized and grid-connected PV units have been developed around the world (Trindade et al., 2016), it is assumed that the large-scale PV farm is connected to the proposed network. In particular, a PV farm is connected to bus#18 to highlight the operational effect of the PV farm on the over-voltage phenomenon. To simplify the analyses, all the parameters are expressed in Per Unit (p.u.) format for load flow studies, the load types are P-Q, while the PV farm is modeled as a load with the capability to inject and absorb reactive power.
The basic active and reactive power of the loads is reported in the literature (Yuvaraj et al., 2021). However, the load profile is not reported for different time intervals of a day. Thus, a time-based factor (HBFt) should be applied to the base-load data to create a daily load profile. This factor will be changed over a day, see Figure 3, to model the load profile during peak and off-peak times. However, different scenarios considering load and power generation profiles can also be considered. The active
where,
As demonstrated in Figure 3, a large-scale PV farm is connected to the grid. Figure 3 also illustrates the forecasted average power generation of the large-scale PV farm for the day ahead.
To assess the proposed methodology’s effectiveness, the case study and its characteristics during a day are modeled and simulated in the MATLAB software environment. The power flow technique is based on the matrix lines-nodes concept, which is discussed in detail by (Sotkiewicz and Vignolo, 2006). In each step of the MATLAB code, the loads and generation data are called, the reactive power is adjusted, the voltage of nodes is checked, and PV power generation is reduced in the case of over-voltage happening; this process repeats until the requirements of stopping criteria met.
It should mention that the optimization algorithm, load and generation profiles, and the studied test system are selected to highlight this paper’s goals. Different scenarios considering load and power generation profiles can be considered by researchers.
The simulation results are provided in Figure 4. Note that, due to eliminating the slack bus in power flow, the remaining bus numbers are 32 buses. Therefore, the nth bus in voltage profile figures is that of (n+1)th bus over the examined network. The active power profiles of the PV farms in different scenarios are also shown in Figure 4A. The APC level by applying the LSH method is lower than that in the case of LSH absent. Therefore, employing the LSH method can increase the benefits of the PV owners by selling more active power. Moreover, the contribution of the PV farm in consuming reactive power is reduced, which will decrease the inverter power loss and consequently increase the inverter’s lifetime. Considering the employed Q support, see Figure 1, the PV unit should consume the reactive power around noon times to reduce the over-voltage issue. On the other hand, the PV unit is responsible for injecting reactive power into the grid to support the profile voltage during the night with respect to the IEEE 1547–2018 standard. The mentioned contribution of the PV unit in Q support is illustrated in Figure 4B.
FIGURE 4. Profiles of output power with and without load LSH method: (A) active power generation and (B) reactive power compensation, (C) Demand profile variation after performing the LSH method, and (D) Comparison of voltage profile concerning different voltage control strategies.
The demand profile variation of all the grid buses after implementing the LSH method is also provided in Figure 4C, where the most demand variations occur during noontime. In addition, the demand reduction occurs during hours 8 p.m. to 12 a.m. for all the buses. The resultant voltage profile is shown in Figure 4D. The voltage value during the day and for all buses remains under 1.05 p. u. Correspondingly, the under-voltage issue is reduced during peak times.
A novel strategy to coordinate the PV units’ active and reactive power controllers and the demand response program is proposed. The proposed strategy mitigated the challenges of the contribution of PV inverters in voltage control. Those challenges consist of high mandatory APC levels and the increased cost and stresses of the PV inverters in reactive power generation. The high contribution of PV units in the voltage control is not cost-effective and may impose complexity in the design and control of inverters. The proposed strategy solved the over-voltage issue and the mentioned challenges by proposing a method for coordinated operation of the PV inverters (considering their active and reactive power exchange) and DR program. Three fitness functions were introduced and integrated into a fitness function. The DR program coordinated the PV unit’s operation to minimize the final fitness function simultaneously and solve the over-voltage issue. The proposed strategy’s effectiveness has been verified successfully on the IEEE 33 bus grid test.
The original contributions presented in the study are included in the article/Supplementary Material, further inquiries can be directed to the corresponding author.
All authors listed have made a substantial, direct, and intellectual contribution to the work and approved it for publication.
This work was supported by the Collaborative Research Project (CRP) grant, Nazarbayev University (Project no. 021220CRP0322).
The authors declare that the research was conducted in the absence of any commercial or financial relationships that could be construed as a potential conflict of interest.
All claims expressed in this article are solely those of the authors and do not necessarily represent those of their affiliated organizations, or those of the publisher, the editors and the reviewers. Any product that may be evaluated in this article, or claim that may be made by its manufacturer, is not guaranteed or endorsed by the publisher.
Aghaei, J., Alizadeh, M. I., Abdollahi, A., and Barani, M. (2016). Allocation of Demand Response Resources: toward an Effective Contribution to Power System Voltage Stability. IET Gener. Transm. & Distrib. 10 (16), 4169–4177. doi:10.1049/iet-gtd.2016.0680
Antonopoulos, I., Robu, V., Couraud, B., Kirli, D., Norbu, S., Kiprakis, A., et al. (2020). Artificial Intelligence and Machine Learning Approaches to Energy Demand-Side Response: A Systematic Review. Renew. Sustain. Energy Rev. 130, 109899. doi:10.1016/j.rser.2020.109899
Anurag, A., Yang, Y., and Blaabjerg, F. (2015). Thermal Performance and Reliability Analysis of Single-phase PV Inverters with Reactive Power Injection outside Feed-In Operating Hours. IEEE J. Emerg. Sel. Top. Power Electron. 3 (4), 870–880. doi:10.1109/jestpe.2015.2428432
Barik, A. K., and Das, D. C. (2020). Coordinated Regulation of Voltage and Load Frequency in Demand Response Supported Biorenewable Cogeneration-based Isolated Hybrid Microgrid with Quasi-oppositional Selfish Herd Optimisation. Int. Trans. Electr. Energy Syst. 30 (1), e12176. doi:10.1002/2050-7038.12176
Ciocia, A., Boicea, V. A., Chicco, G., Di Leo, P., Mazza, A., Pons, E., et al. (2018). Voltage Control in Low-Voltage Grids Using Distributed Photovoltaic Converters and Centralized Devices. IEEE Trans. Industry Appl. 55 (1), 225–237.
Couraud, B., Kumar, P., Robu, V., Jenkins, D., Norbu, S., Flynn, D., et al. (2019). “December. Assessment of Decentralized Reactive Power Control Strategies for Low Voltage PV Inverters,” in 2019 8th International Conference on Power Systems (ICPS), Jaipur, India, 20-22 Dec. 2019 (IEEE), 1–6.
Dong, Y., Xie, X., Shi, W., Zhou, B., and Jiang, Q. (2017). Demand-response-based Distributed Preventive Control to Improve Short-Term Voltage Stability. IEEE Trans. Smart Grid 9 (5), 4785–4795.
Dutta, A., Ganguly, S., and Kumar, C. (2021). Coordinated Volt/Var Control of PV and EV Interfaced Active Distribution Networks Based on Dual-Stage Model Predictive Control. IEEE Syst. J. 2021, 1–10. doi:10.1109/jsyst.2021.3110509
Fatama, A. Z., Khan, M. A., Kurukuru, V. B., Haque, A., and Blaabjerg, F. (2020). Coordinated Reactive Power Strategy Using Static Synchronous Compensator for Photovoltaic Inverters. Int. Trans. Electr. Energy Syst. 30 (6), e12393. doi:10.1002/2050-7038.12393
Joseph, A., Smedley, K., and Mehraeen, S. (2020). Secure High DER Penetration Power Distribution via Autonomously Coordinated Volt/VAR Control. IEEE Trans. Power Deliv. 35 (5), 2272–2284. doi:10.1109/tpwrd.2020.2965107
Karbouj, H., Rather, Z. H., and Pal, B. C. (2020). Adaptive Voltage Control for Large Scale Solar PV Power Plant Considering Real Life Factors. IEEE Trans. Sustain. Energy 12 (2), 990–998.
Kryonidis, G. C., Demoulias, C. S., and Papagiannis, G. K. (2016). A Nearly Decentralized Voltage Regulation Algorithm for Loss Minimization in Radial MV Networks with High DG Penetration. IEEE Trans. Sustain. Energy 7 (4), 1430–1439. doi:10.1109/tste.2016.2556009
Li, H., Azzouz, M. A., and Hamad, A. A. (2020). Cooperative Voltage Control in MV Distribution Networks with Electric Vehicle Charging Stations and Photovoltaic DGs. IEEE Syst. J. 15 (2), 2989–3000.
Li, J., Xu, Z., Zhao, J., and Wan, C. (2017). A Coordinated Dispatch Model for Distribution Network Considering PV Ramp. IEEE Trans. Power Syst. 33 (1), 1107–1109.
Molina-García, Á., Mastromauro, R. A., García-Sánchez, T., Pugliese, S., Liserre, M., and Stasi, S. (2016). Reactive Power Flow Control for PV Inverters Voltage Support in LV Distribution Networks. IEEE Trans. Smart Grid 8 (1), 447–456.
Peng, Y., Li, Y., Lee, K. Y., Tan, Y., Cao, Y., Wen, M., et al. (2020). Coordinated Control Strategy of PMSG and Cascaded H-Bridge STATCOM in Dispersed Wind Farm for Suppressing Unbalanced Grid Voltage. IEEE Trans. Sustain. Energy 12 (1), 349–359.
Photovoltaics, D. G., and Storage, E. (2018). IEEE Standard for Interconnection and Interoperability of Distributed Energy Resources with Associated Electric Power Systems Interfaces. IEEE Std, 1547–2018.
Pournazarian, B., Karimyan, P., Gharehpetian, G. B., Abedi, M., and Pouresmaeil, E. (2019). Smart Participation of PHEVs in Controlling Voltage and Frequency of Island Microgrids. Int. J. Electr. Power & Energy Syst. 110, 510–522. doi:10.1016/j.ijepes.2019.03.036
Prabawa, P., and Choi, D.-H. (2021). Hierarchical Volt-VAR Optimization Framework Considering Voltage Control of Smart Electric Vehicle Charging Stations under Uncertainty. IEEE Access 9, 123398–123413. doi:10.1109/access.2021.3109621
Singhal, A., Ajjarapu, V., Fuller, J., and Hansen, J. (2018). Real-time Local Volt/var Control under External Disturbances with High PV Penetration. IEEE Trans. Smart Grid 10 (4), 3849–3859.
Sotkiewicz, P. M., and Vignolo, J. M. (2006). Allocation of Fixed Costs in Distribution Networks with Distributed Generation. IEEE Trans. Power Syst. 21 (2), 639–652. doi:10.1109/tpwrs.2006.873112
Trindade, F. C., Ferreira, T. S., Lopes, M. G., and Freitas, W. (2016). Mitigation of Fast Voltage Variations during Cloud Transients in Distribution Systems with PV Solar Farms. IEEE Trans. Power Deliv. 32 (2), 921–932. doi:10.1109/TPWRD.2016.2562922
Wongsaichua, W., Lee, W. J., Oraintara, S., Kwan, C., and Zhang, F. (2004). “October. Integrated High Speed Intelligent Utility Tie Unit for Disbursed/renewable Generation Facilities,” in Conference Record of the 2004 IEEE Industry Applications Conference, 2004. 39th IAS Annual Meeting, 2051–2056.3
Xie, Q., Hui, H., Ding, Y., Ye, C., Lin, Z., Wang, P., et al. (2020). Use of Demand Response for Voltage Regulation in Power Distribution Systems with Flexible Resources. IET Gener. Transm. & Distrib. 14 (5), 883–892. doi:10.1049/iet-gtd.2019.1170
Yang, Y., Yeh, H. G., and Tran, K. H. (2020). Cooperative Volt-Ampere Reactive Control for a PV-Enabled Distribution Network with Abnormally High Power Loads. IEEE Syst. J. 15 (4), 5714–5723.
Yao, M., Molzahn, D. K., and Mathieu, J. L. (2019). An Optimal Power-Flow Approach to Improve Power System Voltage Stability Using Demand Response. IEEE Trans. Control Netw. Syst. 6 (3), 1015–1025. doi:10.1109/tcns.2019.2910455
Yeh, H.-G., Gayme, D. F., and Low, S. H. (2012). Adaptive VAR Control for Distribution Circuits with Photovoltaic Generators. IEEE Trans. Power Syst. 27 (3), 1656–1663. doi:10.1109/tpwrs.2012.2183151
Yuvaraj, T., Devabalaji, K. R., Srinivasan, S., Prabaharan, N., Hariharan, R., Haes Alhelou, H., et al. (2021). Comparative Analysis of Various Compensating Devices in Energy Trading Radial Distribution System for Voltage Regulation and Loss Mitigation Using Blockchain Technology and Bat Algorithm. Energy Rep. 7, 8312–8321. doi:10.1016/j.egyr.2021.08.184
Keywords: PV inverters, active power curtailment (APC), over-voltage, demand reposed (DR), high penetrance
Citation: Heidari Yazdi SS, Rahimi T, Khadem Haghighian S, Gharehpetian GB and Bagheri M (2022) Over-Voltage Regulation of Distribution Networks by Coordinated Operation of PV Inverters and Demand Side Management Program. Front. Energy Res. 10:920654. doi: 10.3389/fenrg.2022.920654
Received: 14 April 2022; Accepted: 05 May 2022;
Published: 23 May 2022.
Edited by:
Minghao Wang, Hong Kong Polytechnic University, Hong Kong SAR, ChinaReviewed by:
Jianqiang Luo, Guangdong University of Technology, ChinaCopyright © 2022 Heidari Yazdi, Rahimi, Khadem Haghighian, Gharehpetian and Bagheri. This is an open-access article distributed under the terms of the Creative Commons Attribution License (CC BY). The use, distribution or reproduction in other forums is permitted, provided the original author(s) and the copyright owner(s) are credited and that the original publication in this journal is cited, in accordance with accepted academic practice. No use, distribution or reproduction is permitted which does not comply with these terms.
*Correspondence: Mehdi Bagheri, bWVoZGkuYmFnaGVyaUBudS5lZHUua3o=
Disclaimer: All claims expressed in this article are solely those of the authors and do not necessarily represent those of their affiliated organizations, or those of the publisher, the editors and the reviewers. Any product that may be evaluated in this article or claim that may be made by its manufacturer is not guaranteed or endorsed by the publisher.
Research integrity at Frontiers
Learn more about the work of our research integrity team to safeguard the quality of each article we publish.