- 1State Key Laboratory of Multiphase Flow in Power Engineering, Xi’an Jiaotong University, Xi’an, China
- 2Department of Physics, Shahid Rajaee Teacher Training University, Tehran, Iran
- 3Department of Civil and Environmental Engineering, Carnegie Mellon University, Pittsburgh, PA, United States
- 4School of Urban Planning and Municipal Engineering, Xi’an Polytechnic University, Xi’an, China
Editorial on the Research Topic
Nanoconfined Fluids in Energy Applications
Nanoconfined fluids (NCFs) (Sun et al., 2020) exhibit a rich array of physical and chemical phenomena. Formally, a fluid is nanoconfined if it is situated in an environment in which the distance between solid boundaries is of nanometer scale in at least one spatial dimension. NCFs can be confined within a diversity of geometries, including those with cross-sections that are planar [e.g., nanochannels (Neek-Amal et al., 2016)], circular (e.g., nanotubes), or irregular [e.g., various nanopores (Thiruraman et al., 2020; Hassani et al., 2021a; Hassani et al., 2021b)]. NCFs are remarkable for a number of reasons. Chief among these (potentially interconnected) phenomena are surface effects [owing to the high surface-area-to-volume ratios typical of NCF systems (Wang et al., 2019; Wang and Hadjiconstantinou, 2019)], finite-size effects [owing to the comparability of the confining length scale with the fluid internal length scale (Wang and Hadjiconstantinou, 2015)], and even quantum-mechanical effects [owing to the rough comparability of the confining length scale with the thermal de Broglie wavelength (Tocci et al., 2014)]. As a consequence of these effects, NCFs may exhibit many distinctive characteristics in their mass, momentum, and energy transport as compared to their unconfined (bulk) counterparts.
Research on NCFs presents a number of new challenges and opportunities, ranging from experimental design, to simulation methodology, to approaches for uncertainty quantification for material and transport properties of fluids under nanoconfinement (Li and Wang, 2022). Such work is critical for advancing fundamental physics and chemistry; from an engineering perspective, this work can also promote the development of novel energy-related technologies, with applications ranging from energy storage [e.g., supercapacitors (Yoo et al., 2011; Salanne, 2017)], to energy harvesting [e.g., solar evaporation (Liu et al., 2018; Chen et al., 2019) or electricity generation via reverse electrodialysis (Vanoppen et al., 2018; Zhu et al., 2021)], to enhanced oil and gas recovery (Lee et al., 2016), to membrane-based separation processes (Gong et al., 2021). A schematic illustration of energy applications of NCFs is presented in Figure 1. In this Research Topic, we aim to present Frontier research in experimental, computational, and theoretical approaches to thermodynamics; mass, momentum, and energy transport; flow physics; and phase behavior of NCFs, especially in energy-related applications. We also aim to discuss and identify research challenges in the field, worthy of the community’s broader attention. The main topics of interest in this Research Topic include 1) reviews on recent developments in the field of NCFs, 2) novel experimental and theoretical investigations of NCFs, 3) novel computational studies of NCFs, and 4) NCFs in energy-related applications. In this Research Topic, four high-quality papers were accepted for publication, which we briefly summarize below.
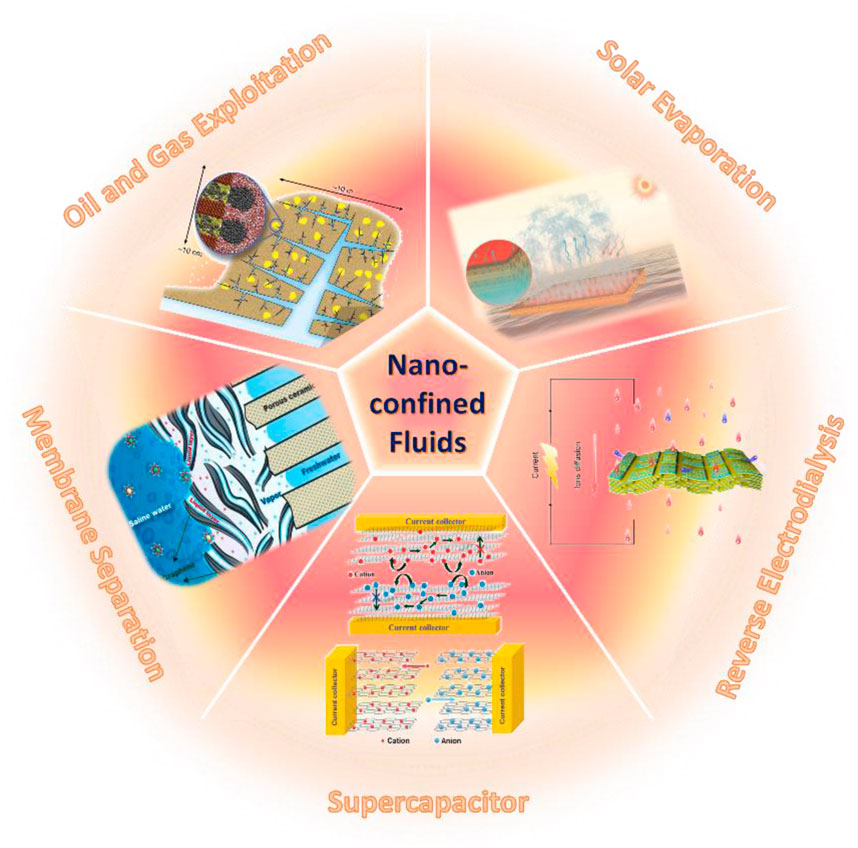
FIGURE 1. Schematic illustration of nanoconfined fluids in energy applications. Inserted figures are adapted from references (Yoo et al., 2011; Lee et al., 2016; Liu et al., 2018; Gong et al., 2021; Zhu et al., 2021).
Cui et al. study diffusion of nanoconfined fluids as motivated by problems of shale gas production. In particular, the authors investigate the diffusivity of high-temperature and high-pressure CH4 gas in silica nanochannels by employing molecular-dynamics simulations. Their results show that the diffusivity is clearly anisotropic, owing to restricted motion of fluid molecules near solid walls. Furthermore, their work reveals the dependence of the diffusivity on the channel height and wall potential interaction.
Zhou et al. make a contribution to the fundamental theory of nanoconfined fluids by studying the specific heat capacity of water in graphene nanochannels. They find a commensurability effect within the specific heat capacity data as channel height decreases to 1.7 nm. The anomalous heat capacity of nanoconfined water (as compared to bulk water) originates from the combination of configurational features (as assessed via density distributions and hydrogen bonds) and vibrational features (as assessed via the vibrational density of states). Specific heat capacity is an important thermophysical property related the heat transfer performance of fluids, and thus this work has significance for thermal energy-related applications of nanoconfined fluids.
Yu et al. study nanoconfined fluids in the context of oil production from reservoirs with a matrix permeability of 68.1 mD and an average pore throat radius of 60 nm. They carry out hydrocarbon gas gravity drainage experiments in two dimensions to investigate the influence of reservoir heterogeneity on the gas-oil interface and sweep characteristics of injected gas. In their experiments, they directly observe the miscible zone of crude oil and hydrocarbon gas, and find that the interlayer has a shielding influence that can destabilize the gas-oil interface and miscible zone.
Sun et al. report on membrane separation with two-dimensional nanoporous materials and study the transport of confined gas in nanopores. They investigate a graphene-hexagonal boron nitride bilayer nanopore to realize high-permeance molecular sieving by virtue of quasi-unidirectional molecular transport through nanopores. The quasi-unidirectional molecular transport results from the discrepancy in the gas adsorption abilities on the two sides of bilayer nanopores. The bilayer pore in their work exhibits 3.7 times higher selectivity of CO2/CH4 as compared to the single-layer graphene nanopore of the same size.
In summary, this Research Topic highlights the promising prospects of nanoconfined fluids in energy applications, showcases several areas of exciting current research, and also highlights several open questions in this field. We sincerely hope that more researchers will invest effort in the study of nanoconfined fluids, from basic theory to application, and especially in the context of energy applications.
Author Contributions
CS organized the writing of this editorial note and CS, MN-A, GW, and ZZ jointly wrote and edited the final text.
Conflict of Interest
The authors declare that the research was conducted in the absence of any commercial or financial relationships that could be construed as a potential conflict of interest.
Publisher’s Note
All claims expressed in this article are solely those of the authors and do not necessarily represent those of their affiliated organizations, or those of the publisher, the editors and the reviewers. Any product that may be evaluated in this article, or claim that may be made by its manufacturer, is not guaranteed or endorsed by the publisher.
References
Chen, C., Kuang, Y., and Hu, L. (2019). Challenges and Opportunities for Solar Evaporation. Joule 3, 683–718. doi:10.1016/j.joule.2018.12.023
Gong, D., Yin, Y., Chen, H., Guo, B., Wu, P., Wang, Y., et al. (2021). Interfacial Ions Sieving for Ultrafast and Complete Desalination through 2D Nanochannel Defined Graphene Composite Membranes. ACS Nano 15, 9871–9881. doi:10.1021/acsnano.1c00987
Hassani, N., Ghorbani-Asl, M., Radha, B., Drndić, M., Krasheninnikov, A. V., and Neek-Amal, M. (2021). Gas Permeability and Selectivity of a Porous WS2 MonolayerGas Permeability and Selectivity of a Porous WS2 Monolayer. J. Phys. Chem. C 125, 25055–25066. doi:10.1021/acs.jpcc.1c06894
Hassani, N., Rashidi, R., Milošević, M. V., and Neek-Amal, M. (2021). Evaluating Gas Permeance through Graphene Nanopores and Porous 2D-Membranes: A Generalized approachEvaluating Gas Permeance through Graphene Nanopores and Porous 2D-Membranes: A Generalized Approach. Carbon Trends 5, 100086. doi:10.1016/j.cartre.2021.100086
Lee, T., Bocquet, L., and Coasne, B. (2016). Activated Desorption at Heterogeneous Interfaces and Long-Time Kinetics of Hydrocarbon Recovery from Nanoporous Media. Nat. Commun. 7, 11890. doi:10.1038/ncomms11890
Li, Y., and Wang, G. J. (2022). How to Produce Confidence Intervals Instead of Confidence Tricks: Representative Sampling for Molecular Simulations of Fluid Self-Diffusion under Nanoscale Confinement. J. Chem. Phys. 156, 114113. doi:10.1063/5.0081707
Liu, H., Chen, C., Chen, G., Kuang, Y., Zhao, X., Song, J., et al. (2018). High-Performance Solar Steam Device with Layered Channels: Artificial Tree with a Reversed Design. Adv. Energy Mat. 8, 1701616. doi:10.1002/aenm.201701616
Neek-Amal, M., Peeters, F. M., Grigorieva, I. V., and Geim, A. K. (2016). Commensurability Effects in Viscosity of Nanoconfined waterCommensurability Effects in Viscosity of Nanoconfined Water. ACS Nano 10, 3685–3692. doi:10.1021/acsnano.6b00187
Salanne, M. (2017). Ionic Liquids for Supercapacitor Applications. Top. Curr. Chem. (Z) 375, 63. doi:10.1007/s41061-017-0150-7
Sun, C., Zhou, R., Zhao, Z., and Bai, B. (2020). Nanoconfined Fluids: What Can We Expect from Them? J. Phys. Chem. Lett. 11, 4678–4692. doi:10.1021/acs.jpclett.0c00591
Thiruraman, J. P., Dar, S. A., Das, P. M., Hassani, N., Neek-Amal, M., Keerthi, A., et al. (2020). Gas Flow through Atomic-Scale Apertures. Sci. Adv. 6, eabc7927. doi:10.1126/sciadv.abc7927
Tocci, G., Joly, L., and Michaelides, A. (2014). Friction of Water on Graphene and Hexagonal Boron Nitride from Ab Initio Methods: Very Different Slippage Despite Very Similar Interface Structures. Nano Lett. 14, 6872–6877. doi:10.1021/nl502837d
Vanoppen, M., Criel, E., Walpot, G., Vermaas, D. A., and Verliefde, A. (2018). Assisted Reverse Electrodialysis-Principles, Mechanisms, and Potential. npj Clean. Water 1, 9. doi:10.1038/s41545-018-0010-1
Wang, G. J., Damone, A., Benfenati, F., Poesio, P., Beretta, G. P., and Hadjiconstantinou, N. G. (2019). Physics of Nanoscale Immiscible Fluid Displacement. Phys. Rev. Fluids 4, 124203. doi:10.1103/physrevfluids.4.124203
Wang, G. J., and Hadjiconstantinou, N. G. (2019). Universal Molecular-Kinetic Scaling Relation for Slip of a Simple Fluid at a Solid Boundary. Phys. Rev. Fluids 4, 064201. doi:10.1103/physrevfluids.4.064201
Wang, G. J., and Hadjiconstantinou, N. G. (2015). Why Are Fluid Densities So Low in Carbon Nanotubes? Phys. Fluids 27, 052006. doi:10.1063/1.4921140
Yoo, J. J., Balakrishnan, K., Huang, J., Meunier, V., Sumpter, B. G., Srivastava, A., et al. (2011). Ultrathin Planar Graphene Supercapacitors. Nano Lett. 11, 1423–1427. doi:10.1021/nl200225j
Keywords: nanoconfined fluids, energy, basic theory, engineering application, research methodology
Citation: Sun C, Neek-Amal M, Wang GJ and Zhao Z (2022) Editorial: Nanoconfined Fluids in Energy Applications. Front. Energy Res. 10:919288. doi: 10.3389/fenrg.2022.919288
Received: 13 April 2022; Accepted: 25 April 2022;
Published: 27 May 2022.
Edited and reviewed by:
Francois M. A. Marechal, Swiss Federal Institute of Technology Lausanne, SwitzerlandCopyright © 2022 Sun, Neek-Amal, Wang and Zhao. This is an open-access article distributed under the terms of the Creative Commons Attribution License (CC BY). The use, distribution or reproduction in other forums is permitted, provided the original author(s) and the copyright owner(s) are credited and that the original publication in this journal is cited, in accordance with accepted academic practice. No use, distribution or reproduction is permitted which does not comply with these terms.
*Correspondence: Chengzhen Sun, sun-cz@xjtu.edu.cn