- 1Innovation Institute for Sustainable Maritime Architecture Research and Technology, Qingdao University of Technology, Qingdao, China
- 2Faculty of Environmental Engineering, The University of Kitakyushu, Kitakyushu, Japan
The building sector is undergoing a low-carbon transition featuring applications of energy-efficient technologies and management innovations. Herein, we contribute to the study of the residential zero-carbon district demonstration project in Japan, introducing smart practices and examining occupants’ awareness. We proposed a questionnaire survey covering more than 400 smart zero-energy house owners that looks beyond the technologies for smart energy management and recognizes how the residential consumers engaged and perceive delivered energy saving and cost reduction issues, and their usage frequency of home energy management system information-based services is also examined considering the impact of the variables of household income and occupants’ age. Overall, the respondents’ energy and cost-saving awareness vary widely, and the results provide the effectiveness of the visual-based service in engaging end-users in the responsive energy management system. In the context of the detailed operational performances of real zero-energy houses, we find evidence that automatic control has reshaped the characteristics of intraday energy balance and gives consumers greater control over their own energy bills. The findings clearly show the detailed role of integrated energy-efficient technologies over typical periods; the grid-connected photovoltaic system plays a significant role in the realization of net annual zero electricity balance, and micro-cogeneration’s contribution to zero-energy houses’ energy self-sufficiency largely depends on the building heating load.
1 Introduction
1.1 Background
To mitigate the global climate change, many countries launched their social carbon neutrality target to support the Paris Agreement goal of pursuing the limitation of warming to below 2°C, which requires all countries to work together to bring greenhouse gas emissions to zero within the second half of the 21st century. The low-carbon transition is a complex socio-technical problem, and technological improvements, renewable energy exploration, and collaborative cooperation between the central energy supplier and distributed consumers are highlighted in the energy system decarbonization (Rogelj et al., 2015; Creutzig et al., 2018). In December 2020, the Japanese government launched the target of achieving carbon neutrality by 2050. Currently, Japan largely depends on energy imports, and its primary energy self-sufficiency was only 9.6% in 2017, which is a lower level than major Organization for Economic Co-operation and Development countries (Energy AfNRa, 2020a). METI (Ministry of Economy, Trade, and Industry) made various technical efforts and energy market innovations to decarbonize the social energy system, focusing on energy efficiency measures, renewable energy utilization, and demand-side conservation efforts (Energy AfNRa, 2020b).
The residential sector features a high energy density and shares a considerable proportion of social carbon emissions in Japan, accounting for 14.6% of final energy consumption related to carbon emission in 2018 (Actions JCfCC, 2020). In line with the national 2030 greenhouse gas reduction target (cutting its greenhouse gas emissions by 26% in 2030 in comparison with the 2013 level), a 122 million ton CO2 emission reduction mission was assigned to the residential sector (Agency for Natural Resources and Energy M, 2019). Therefore, improving energy efficiency to reduce overall energy consumption is necessary to decarbonize the residential sector (Grinham et al., 2022). Development of near- or net-zero energy buildings is considered a practical approach; the Japanese government had announced the roadmap for net-zero-carbon buildings, accelerating the low-carbon transition (Mah et al., 2013). Decentralized rooftop PV (photovoltaic) and energy-efficient micro-CHP (combined heating and power) systems were widely implemented, and the development of PV systems has been accelerated since the launch of the feed-in tariff scheme in 2012 (Ministry of Economy TaI, 2019; Li et al., 2020). With the widespread deployment of behind smart meters and communication networks, research highlights the role of active demand-side participation in reducing household CO2 emissions (Shigetomi et al., 2018); smart practices and management innovations have been widely demonstrated in low-carbon demonstration projects in Japan (Shimoda et al., 2021; Li et al., 2022).
1.2 Literature Review
As reported in prior works, intensive technological efforts have been made toward low-carbon or zero-energy building transition, such as nearly or net ZEH (zero energy house), ZEB (zero energy building), and ZED (zero-energy district) in different countries (Panagiotidou and Fuller, 2013; Kalaycıoğlu and Yılmaz, 2017; Bandeiras et al., 2020). The US Department of Energy (DOE) defines net-zero-energy residential or commercial buildings as objectives with significantly reduced energy load through energy-efficient efforts, and the remaining energy demand is covered with renewable production (Rajkovich et al., 2013). In order to deal with the mismatch between simultaneous on-site PV production and energy demand, Heine et al. (2019) highlighted the value of implementing battery storage to the ZEH by maximizing the net present value under a time-of-use (ToU) rate structure. European Union EPIE (Building Performance Institute Europe) had clearly described the boundaries where to measure renewable energy share and minimum renewable energy share was defined for net-zero-energy buildings. Colclough et al. (2018) found that nearly ZEB can be achieved at a minimal cost when built to the passive house standard. Net-zero-energy districts as a group of energy-efficient buildings with greatly reduced energy demand achieve net energy balance in terms of integration of renewable energy (Koutra et al., 2018). Sameti and Haghighat (2018) proposed a well-designed combination of the distributed energy system and energy storage; the results indicated that energy share among buildings could bring significant economic and environmental benefits to the net-zero-energy district system. The broad development of behind smart meters and integration of on-site energy resources contributed significantly during the transition toward a zero-carbon building; user-side investments such as existing building retrofit and smart management are increasingly recognized as a strategic priority in phasing out inefficient energy supply (Zeiler et al., 2016; EPIE BPIE, 2020). de Chalendar et al. (2019) pointed out that carbon-aware scheduling that takes advantage of variations in grid power carbon intensity would unlock significant benefits under electrification trends in district heating and cooling networks. Japanese CASBEE (Comprehensive Assessment System for Built Environment Efficiency) is the main tool to assess and rate the energy performances of detached houses considering the environmental load and built indoor quality; nearly ZEH and ZEH could meet the highest-ranking level of the built energy efficiency (Energy Efficiency and Conservation Division Agency for Natural Resources and Energy M, 2015). Oki et al. (2019) introduced the thermal comfort and energy saving of a net-zero-energy house renovated from an existing house in Japan, highlighting the benefits of integrated distributed energy resources. Ren et al. (2016) optimized the residential hybrid PV/fuel cell/battery system from an economic, energy, and environmental perspective and micro-CHP increased the energy self-sufficiency ratio of households; a larger ratio of PV production had to be sold into the grid. The uncertainty associated with variable renewable sources and the detailed mismatch between real-world on-site generation and household demand profiles are exhibited, and our previous work verified the advantage of fuel cells in achieving nearly ZEH and carbon dioxide emission reduction (Li et al., 2020).
Owing to the rapid diffusion of the decentralized energy technologies installed at the household level, such as decentralized heat pumps, batteries, rooftop PV, and micro-CHP systems, an important phenomenon is that the number of energy prosumers is rising, offering new opportunities and challenges to the governance of the district energy system (Brown et al., 2020). Those energy prosumers are increasingly considered as important resources in utilizing renewable energy resources and attracted increasing attention to the role of coordinated end-users’ participation (Rathnayaka et al., 2014; Zafar et al., 2018; Zhang et al., 2021a). For example, the civic energy community grows significantly supported by the support of digital technologies and collaborative business practices (Verkade and Höffken, 2019). Prosumers become increasingly involved in community energy management (Rodrigues et al., 2020), and the importance of user engagement in the district energy management system grows (Tachmitzaki et al., 2019). Sattarpour et al. (2018) propose the collaborative home energy management approach in flattening load that assists the microgrid management. Tiefenbeck V. et al. (2018) evaluated the energy conservation effects of induced behavior change from digital activity-specific feedback; it can be considered a cost-effective resource to foster energy conservation. Haghifam et al. (2020) further demonstrated the bi-level optimization to maximize profits from demand response measures and minimize the operating cost of microgrid owners. In order to achieve optimized social outcomes, Bandeiras F. et al. (2020) highlighted the energy services of coordinating demand-side participation and storage application toward achieving net-zero-energy buildings. Diawuo et al. (2020) estimated potential peak residential electricity reduction from demand-side management; the results encourage the use of smart controllable devices and facilitate the interaction between end-users and the public system to support demand response programs.
Encouraging consumers to embrace smart practices toward low-carbon building transition is important (Cruz et al., 2021). The rapid development of smart HEMS (home energy management system), information delivery, and feedback could increase the energy- or cost-saving changes by involving the consumer in the active management. Numbers of empirical studies evidenced the effectiveness of individual participation in shaping energy-related behavior and the corresponding energy-saving effects; demand response, which was considered a promising way to reduce carbon emission, is attracting rising popularity (Parrish et al., 2020). Implementations of information-based visualization of real-time energy consumption induce consumers to make better-informed decisions and in turn bring energy bill-saving benefits (Tiefenbeck, 2017). Home IoT (Internet of things) and behind smart meters increase the level of information provided to community members, and improvement in the function of automatic control aims at motivating active building to encourage “smart behaviors” of energy use in response to dynamic pricing contracts, such as time of use, real-time pricing, and critical peak pricing tariffs (Tiefenbeck, 2017; Li et al., 2018; Yan et al., 2018; Radenković et al., 2020). Interview analysis indicates that householders were willing to modify their energy-related practices in response to price fluctuations to meet their quality of daily life (Ozaki, 2018). Incentive-based demand response programs can serve as sources of contracted flexibility with the aggregator and help deal with challenges brought by the transition to the low-carbon energy system (El Geneidy and Howard, 2020). However, it is still worth noting that individual activity tends to be concerned more about maximizing the cost-saving, and approaches toward sustainable and reliable district energy supply need to be strengthened (Mengolini et al., 2016).
Despite growing technological innovations and interest in smart energy management of low-carbon buildings, few works have revealed in detail how the smart technologies work and the extent consumers could be involved in local energy system management. It is also worth noting that a number of issues may limit decentralized consumers’ engagement in district energy system management (Hargreaves et al., 2010; Bell et al., 2015). Rising onsite intermittent generation and electrification can result in a modified pattern of electricity demand. Effective reduction in energy consumption requires the implementation of efficient technology and behavioral plasticity to enhance technology adoption and appropriate use. With the help of behind-the-meter, vast development of renewable resources can result in significant energy mismatch and create new peak load; it may require an evolution of prosumers’ smart participation toward zero-carbon transition. Hence, to facilitate the sustainable transition toward low-carbon districts and identify the barriers, it is necessary to foster a deeper understanding of the emerging smart energy practices among real ZEHs, including evaluating governance innovation and assessing different management effects as well as detailed operational scenarios of the building energy system.
1.3 Contents and Contributions
Previous efforts for achieving zero-carbon built environments focused more on energy efficiency improvement design and the scheduling optimization of the distributed energy technology. However, the effectiveness evaluation of smart practices and behavioral governance is missing. To the best of the authors’ knowledge, the contributions of this work are as follows:
Recent research emphasizes the importance of active demand-side management in providing energy saving chances; this work will report the technological efforts, management innovation, and business regulation approach in a residential zero-carbon demonstration project.
The contribution of active customer participation is closely related to the occupants’ awareness and accessibility of the smart energy systems. However, the unavailability of occupants’ feedback has constrained the examination of information-based technologies. This work would examine end users’ attitudes, evaluate the impacts of income and age on the usage of HEMS functions, and identify gaps between vision and practice on the basis of a questionnaire survey.
It is necessary to understand the extent to which on-site generators serve the ZEH achievement, even if the annual net-zero is achieved. In terms of behind-meter data, this work further identifies the mismatch between renewable generation and electricity demand based on real data of behind smart meters, characterizes the energy performance of smart energy practice with HEMS control, and provides a better understanding of their role and future efforts toward achieving sustainable ZEH.
The remainder of this paper is organized as follows: Section 2 describes a brief overview and relevant technical solutions regarding energy saving. Section 3 introduces the analysis process. Section 4 reports the results and discussion. Finally, Section 5 draws the conclusion.
2 Materials and Smart Practices
The participants in this research were first recruited in the Jono zero-carbon district demonstration project in Kitakyushu City, a pioneer for the Sustainable Development Goals (SDGs) in Japan. The average ambient temperature of this district is about 2.0°C in January (the coldest month). The selected zero-carbon district demonstration project area is about 18.9 ha, which is defined as an advanced zero-carbon residential district, and the construction was completed in 2016. In 2020, this district was composed of 606 private dwellings, with approximately 1,300 citizens. The photograph of the demonstration project layout is shown in Figure 1. In this demonstration project, the average thermal transmission coefficient of the building envelope is significantly reduced to 0.6 W/(m2 K), which presents the rate of heat transfer over a given envelope area; the heat energy loss rate per floor area can be as low as 1.9 W/(m2 K). Figure 1 shows a picture of a real ZEH; the window to wall ratio of the house is about 0.2. The infill material of ZEH’s structure is steel and wooden, the wall is insulated with glass wool that features a low thermal conductivity value [0.04 W/(m·K)], and the window is composed of Low-E double glazing and aluminum frames with thermal insulation. HEMS has been installed in each ZEH, which deliberately aims to achieve energy conservation and efficient district energy resource management, emphasize the active participation of end-users, and utilize all available resources to achieve the net-zero-carbon goal.
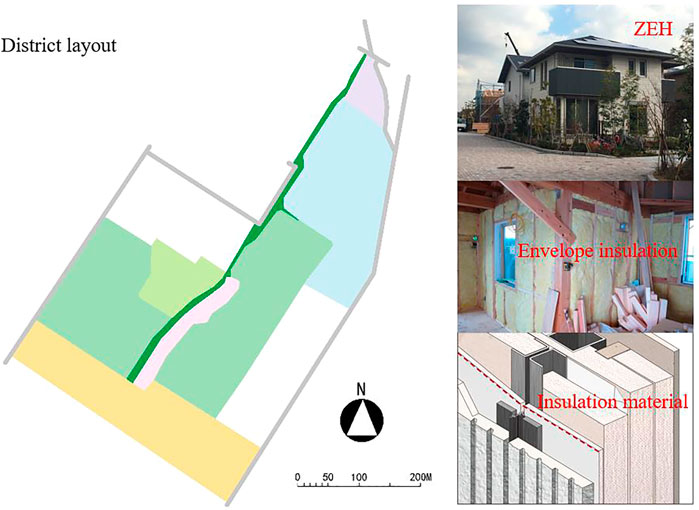
FIGURE 1. Layout of the residential zero-carbon district demonstration project and typical ZEHs (Kitakyushu, Japan).
2.1 Zero-energy House Concept
ZEH is an acronym for zero-energy house, assuming that the annual net energy consumption is nearly zero. The Japanese government has established the goal of turning more than half of newly constructed houses into ZEHs by 2020, then realizing the target for all newly built houses to be net-zero-energy buildings by 2030 (Ministry of Economy TaI, 2015). Smart ZEH features low lifecycle carbon emission and intelligent characteristics of its control system, significantly reducing energy consumption expenses. However, current ZEHs still are expensive in the current market. In order to increase the popularization of ZEHs, homebuilders and building contractors have been making efforts to improve ZEH affordability through promoting mass production; the government also offers fixed-amount subsidies for the ZEH builders and makes ZEH more attractive.
Meanwhile, the Japanese ZEH Roadmap Examination Committee has introduced the definition and evaluation approach of a net ZEH. The definition of ZEH and how a variety of different measures achieve ZEH are illustrated in Figure 2. First, more than 20% reduction of primary energy consumption of ZEH oriented could be obtained by guiding building design standards, such as high thermal insulation level regulation; the envelope is generally composed of material with a low U-value (Zhang et al., 2021a). Distributed rooftop PV and fuel cell systems are deployed to increase the energy self-sufficiency of the ZEHs; nearly ZEH could be granted when a 75% reduction of energy consumption is achieved. On-site generators enable the detached house to play as an energy prosumer and achieve a net annual electricity balance between the household and public grid when feed-in electricity is taken into consideration. The ZEH status would be granted when more than 100% primary energy reduction is achieved (Energy Efficiency and Conservation Division Agency for Natural Resources and Energy M, 2015). In the current energy market, PV is prioritized as a cost-effective source to increase energy self-sufficiency; house owners can sell surplus PV generation to the public grid by means of feed-in tariff rates.
2.2 HEMS Function
The widespread ZEH raised the concern of strengthening decentralized energy management systems and end users’ collaboration with an energy supplier. According to the historically operational information, the real-time management strategy of HEMS features self-learning and predictive control characteristics and helps end-users manage home appliances as well as generate and store energy according to their willingness (Panasonic. AiSEG2 HOME IoT, 2019). Considering potential demand response benefits, the Japanese government established a target to implement HEMS devices in all households by 2030. In order to improve monitoring and management services of HEMS equipment manufactured by different companies, the OpenADR 2.0 (Open Automated Demand Response) protocol as an international standard for facilitating and automating demand response communication was adopted in HEMS as shown in Figure 3, standardizing the way for system operators to communicate signals with customers. Smart meters provide a connection between utilities and residential customers; ECHONET Lite as the core component can connect and control the distributed energy generators and home appliances from different companies under the HEMS environment (Isshiki et al., 2015). HEMS enables residents to access information on real-time energy generation, consumption, and electricity price. At a glance, the customers can see the devices that use a large ratio of electricity and are familiar with their own energy self-sufficiency rates. The indoor home-display system aims to induce consumers a comfortable lifestyle by encouraging them to manage on-site energy generation and consumption properly. For example, in terms of potential cost-saving benefits, residents can adjust the real-time behaviors of the home equipment, such as adjusting the air conditioning temperature, scheduling the battery considering the real-time electricity rate, and checking the status of appliances when customers are away from home. HEMS can deliver energy consumption and cost reports (weekly or monthly version); customers understand the energy usage situation with respect to the set target value. Customers can make a diagnosis, knowing which equipment is used more than usual, thus guiding power saving and better energy use. Depending on the measurement of the smart meter, the HEMS can also recommend a suitable electricity rate plan for households. HEMS is expected to come into wide use as it creates chances for energy conservation for end-users and promotes services in collaborative communication with the smart community energy system (Ganji and Shahidehpour, 2018; Li et al., 2020).
As shown in Figure 3, the smart metering infrastructures have been installed among ZEHs, the HEMS enables the real-time energy consumption feedback and status monitoring of appliances and distributed power units. The smart meter and information communication framework is capable of monitoring and controlling home appliances remotely; the home display device enables real-time information communication between individual and local energy services. Air conditioners, hot water equipment, lighting, and other devices are connected to HEMS; occupants can receive the price information from the energy service provider and then optimize the scheduling strategy of smart home electrical appliances, such as heat pumps, washing machines, and lighting systems (Li et al., 2020).
In order to facilitate controllability on the subset of smart houses from a community-level perspective, the community energy management system (CEMS) as an energy and information monitoring framework interconnects different energy prosumers, providing a systematic approach for identifying the outcomes expected from the smart management to deliver. The bi-directional information communication network between HEMS and CEMS could automatically collect smart meter data and transmit them through IoT at both household and utility service levels, enabling building occupants to easily access their detailed electricity consumption components and identifying individual energy cost ranks among community members through the online information. The CEMS gathers energy information from integrated HEMS within a community; a detailed HEMS figure of the household energy consumption rank and cost screen is shown in Figure 4. The user-friendly interface of the HEMS smartphone application and indoor display aims at creating an awareness-induced change in lifestyle that favors residents to participate more responsibly in energy consumption reduction and cost savings (Panasonic. AiSEG2 HOME IoT, 2019).
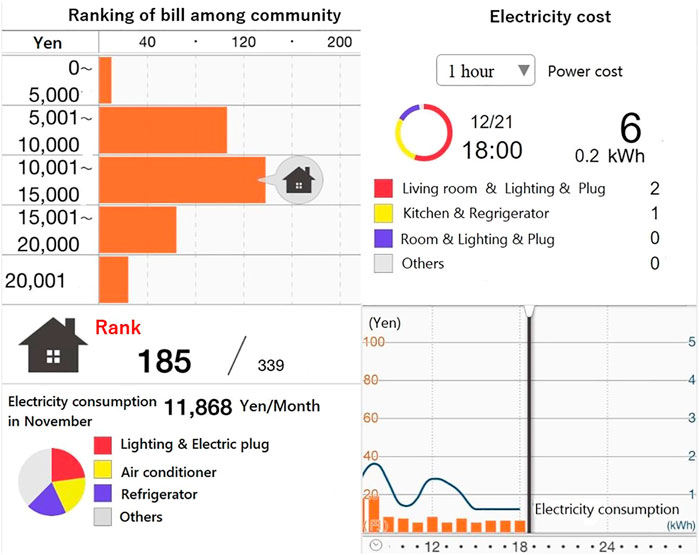
FIGURE 4. HEMS interface and available online information: view of their cost level among the community and detailed real-time electricity consumption and cost.
2.3 Energy Market Innovation
In order to reinforce industrial competitiveness, protect consumer benefits, and create new services, the Japanese government opened the retail electricity market competition to allow business consumers to have more options to manage their energy supply. Energy retail market liberalization was fully achieved in 2016; consumers can choose their own preferences about energy retailers. Cost is the main driver for consumer switching; consumers choose to change their provider if the transaction costs are lower. Electric companies have implemented price-based and incentive-based tariff structures for end users, aiming to support consumers in allocating optimal energy load or reducing the energy consumption bill over periods.
For behind-the-meter business models, the battery and power to the heat technology are part of the building’s energy management. The dynamic electricity price aims to subject consumers to price-responsive behavioral changes, such as shifting or reducing their consumption considering the cost of delivered electricity. The ToU tariff is popular among residential houses equipped with a heat pump system, which could motivate users to operate the heat pump to store hot water during the off-peak period and then covers daily heat demand (Company, 2018). Monthly electricity rates are the sum of the demand charge and energy volume charge. The demand charge will be determined by the contracted ampere, while the energy charge will be calculated according to the amount of energy consumption, and charge rates for actual usage are stepped with higher rates as customers use more power (Gas, 2018). Adopt monthly electricity rates can bring potential cost savings for the consumers by increasing energy self-sufficiency, such as by implementing on-site power generators.
3 Methodology
The question of how to design HEMS to alleviate the different subjective and personal barriers of the end-users deserves attention. Learning from survey design experiences in Pan and Pan, 2018; Azizi et al., 2019; Zhang et al., 2021b, we designed and carried out a field questionnaire survey among householders from September 2018 to April 2019. All respondents in the survey are living the demonstration project, aiming at examining their behaviors and perceptions regarding the zero-carbon demonstration project. The first section of the questionnaire examines the basic information including detached house size, occupant age, and household income in different groups as well as the characteristics of the house energy system; the questions can be chosen from defined answers. The second section focuses on investigating respondents’ attitudes and experiences; there are available defined answers for each question. For example, respondents can choose the answers like “cost rise,” “almost unchanged,” “drop,” and “drop obviously” for their awareness of energy cost change. All respondents can also write their comments at the end of the questionnaire survey. In order to ensure the quality of survey data, all respondents can earn a 3,000 Yen intervention reward. A total of 409 effective questionnaire surveys were collected from random 500 samples of households. Considering the wide application of distributed energy systems, the questions look beyond simply the energy-efficient technology and further investigate to what extent consumers could be engaged in demand-side management with access to HEMS services such as an in-home display. Respondents are asked questions to evaluate their perceptions of their own ZEHs, such as energy-saving or bill change, and examine their behaviors engaged in demand-side management. Specifically, the survey offered the following questions related to energy use behaviors:
(1) Compared with the scenario before you lived in the district, how about changing your energy-saving consciousness with smart energy use change?
(2) Your house features a long lifespan and low energy consumption. How do you feel the value?
(3) How about the electricity and gas consumption cost change after moving into the district?
(4) How often do you view information delivered from CEMS?
(5) How about your view frequency of the HEMS function of the in-home display?
(6) If you have any questions about your house energy consumption and management, please write in the blank space reserved in the questionnaire.
Meanwhile, operational data of real ZEHs’ energy systems were obtained from the HEMS measurement. Following the collected information, analysis is applied to reveal scenarios of the demand side participation and dynamic energy behaviors of ZEHs in this zero-carbon district.
4 Results and Discussion
The house-related characteristics may relate to their attitudinal and behavioral responses. Figure 5 summarizes the basic information of the household characteristics gathered from the questionnaire survey, including energy-related choices, detailed percentile shares of house area, annual household income, contracted power ampere, and choices of heating supply resources. All detached ZEHs are equipped with a rooftop PV system; it is worth noting that a large ratio of houseowners adopts fuel cell systems.
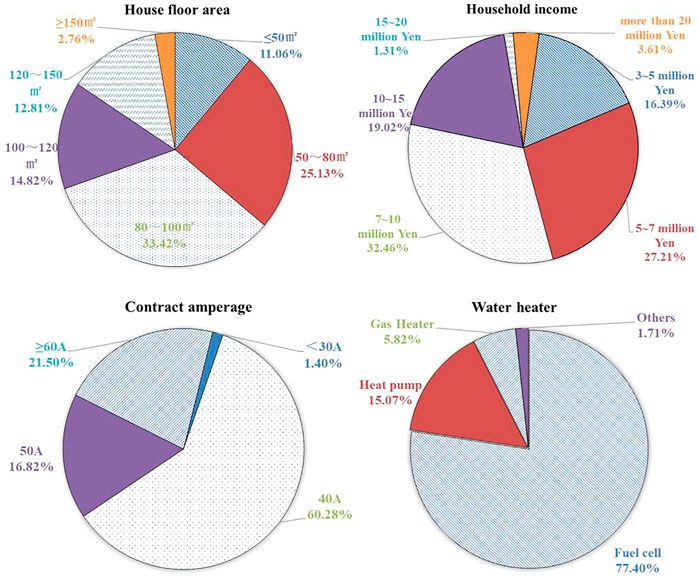
FIGURE 5. Basic information of interviewed households in the zero-carbon district demonstration project.
4.1 Understanding and Attitude of Participants
The following analysis presented the differences in perceptions of respondents. As depicted in Figure 6, we summarized respondents’ recognitions about the attitude and value of their energy-saving consciousness in comparison with their prior levels before moving into this demonstration project; 11.08% of respondents chose the answer “think it increases significantly,” followed by 32.22% of participants with “a moderate increase,” and a small proportion (11.86%) of respondents’ energy-saving awareness dropped.
Regarding their attitudes toward ZEH’s value, the question is “How do you feel the value of ZEH in energy saving and carbon emission reduction?” A large ratio of respondents gave positive comments; 43% of them thought that the feeling was high, and 45% of the respondents partially perceived it; detailed results are shown in Figure 7.
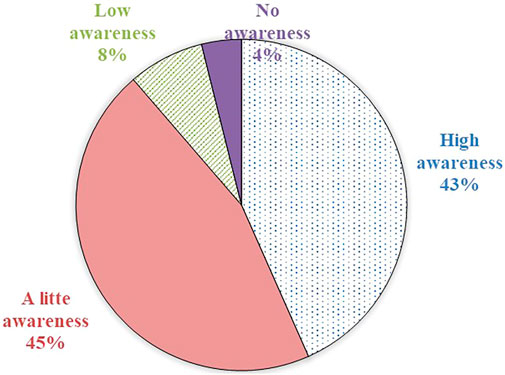
FIGURE 7. Respondents’ attitude toward ZEHs’ value considering energy saving and emission reduction.
As shown by the survey results in Figure 8, respondents gave answers about the change level of monthly electricity and gas consumption costs. Only a small ratio of respondents selected the answer that their monthly electricity cost rises, and 30% of consumers’ perception is that the cost drops obviously. The answer toward the change level of gas cost varied significantly; even 28% of consumers think that the gas cost rises, which could be attributed to the consumption of fuel cells.
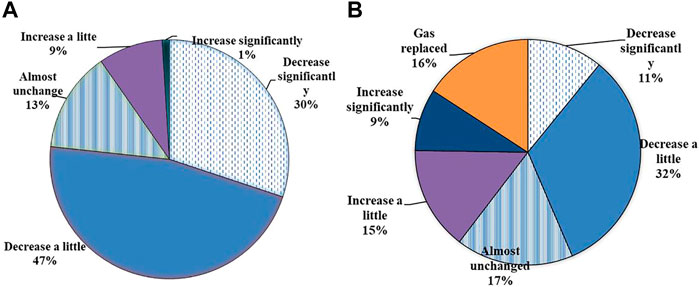
FIGURE 8. Perspectives on monthly electricity (A) and gas (B) cost change after moving into the district.
The in-home display device provides real-time information on detailed electricity consumption and cost, which aims at inducing users to modify their lifestyle through real-time visualization of behind smart meter data. The questionnaire survey also quantified how often the consumers accessed the HEMS service data and examined respondents’ acquisition of CMES feedback information. The visualization frequency distributions of in-home display and CEMS information are described in Figures 9, 10, respectively. HEMS browsing behaviors of occupants vary significantly; only a small proportion (8%) of the participants access HEMS once a day, and 26% of the respondents ignore the HEMS function. The result indicated that the majority (82%) of the respondents seldom or never access the information of CEMS reports.
At the end of the questionnaire interview, we also asked them to comment on doubts and obstacles regarding the above-related topics according to their experiences. Based on the suggestions from end-users, the main criticism of the display is that they feel that it is difficult to understand the role of CMES feedback services, they need to be educated in the local network engagement, and some respondents prefer to connect the CEMS or access the HEMS data in a more convenient way. According to the collected comments from the questionnaire survey, occupants often checked the system when they first moved into the community due to the novel function. However, they are gradually less likely to take any notice from HEMS or CEMS as they become accustomed to ignoring them.
The relationship between household income and customers’ active access to HEMS visualization functions (described in Figure 9) is also examined, as described in Figure 11. The y-axis presents HEMS utilization scores, the highest HESM utilization ratio (once a day described in Figure 9) scored the highest value of 1.0, and the scores of other groups are decreased by 0.17 with their decreasing view frequency of HEMS. The x-axis presents the household income of ZEH owners. The distributions show that there is less correlation between household income and HEMS utilization score. Participant groups with 600–800 thousand Yen annual income pay more attention to HEMS functions. Occupants with the lowest income show the least interest in information about HEMS delivered.
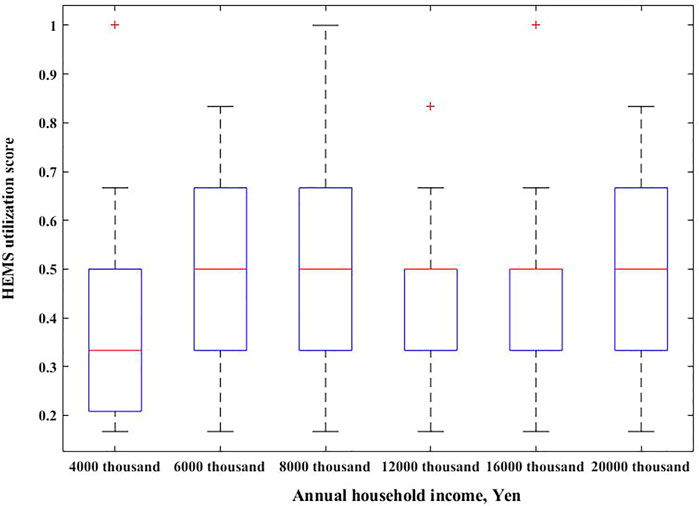
FIGURE 11. Variations in HEMS utilization scores of all participants with different annual household incomes.
The field survey also examines the impact of the population age of occupants on HEMS usage. Figure 12 describes variations of HEMS utilization scores among different age groups; it is worth noting that elderly customers tend to visualize more of the indoor HEMS display. It can conclude that the elderly tend to be more likely to change lifestyles considering energy efficiency measures; it can also be explained by older people spending more time indoors, resulting in the rising chance of visualizing HEMS functions.
4.2 Operational Performance of Zero-energy Houses
Intermittent PV, energy-efficient heat pumps, batteries, and micro-CHP systems are considered as essential technologies for decarbonizing residential energy systems. Considering the components of energy systems, ZEH id1∼3 are all electrified houses with grid-connected rooftop PV systems, and home heat pumps meet the daily hot water demand. id4∼6 presents the ZEHs equipped with hybrid PV and fuel cell systems; the operation of the fuel cell system can simultaneously meet the hot water usage. The capacity of the installed rooftop PV system is about 4.5 kWp, the battery capacity is 6.5 kWh, and the rated power output of the fuel cell is 700 W. Due to the real-time mismatch between residential load and PV generation, only part of PV electricity can be locally self-consumed, excess generation has to be fed into the grid, and grid import is also needed when the output of the fuel cell cannot meet the electricity demand, especially in the absence of PV generation. As described in Figure 13, the dash line presents the net annual grid load; it is shown that PV generation plays a crucial role in offsetting annual electricity consumption. It is worth noting that still more than half of PV production had to be fed into the grid. The implementation of a micro-cogeneration system significantly reduces the grid import, resulting in a nearly zero or negative net annual grid import. This could be explained by the role of the heat pump being replaced with recovered heat during CHP operation. However, in practice, the implementation of fuel cells negatively affects the local PV self-consumption ratio, resulting in suppression of the directly consumed PV production.
As illustrated above, the realization of ZEH is an annual net-zero electricity exchange between on-site generation and the grid. To get a better understanding of energy-efficient technologies’ role in achieving ZEH, Figures 14, 15 illustrate the detailed variations of the intra-week electrical energy flow profiles of selected typical ZEHs at 30-min time intervals in winter, mid-season, and summer weeks, including grid-exchange flows and dispatch behaviors of the battery, micro-CHP, and PV operation, and the rule-based control strategies had been detailed explained in our previous research (Li et al., 2020). Figure 14 depicts the operational scenario of a typical ZEH equipped with PV, battery, and heat pump systems in different seasonal weeks; the residential ToU tariff structure (the peak price lasts from 8:00 am to 22:00; the other time is the off-peak period) influences the intraday scheduling strategy of the heat pump and battery storage systems. The HEMS can operate heat pumps to hourly electricity prices; its operation period is mainly scheduled to the off-peak price period, and daily hot water is pregenerated and stored in water tanks. The cost of hot water generation can be effectively reduced. The blue line presents grid import electricity; the scheduling of the heat pump increases the grid import electricity and results in a sharp peak load during the early morning hours. Compared with the summer days (Figure 14A), the larger hot water demand on winter days (Figure 14B) produces a higher peak load. Behaviors of the battery system are described in the yellow area, the charging activity is concentrated during night off-peak periods, and the discharge period occurs at a high electricity rate, which offers residents a cost reduction chance. Due to the mismatch between on-site electricity generation and demand profiles, direct local PV consumption in the green area is limited by simultaneous electricity load, excess PV production represented by the purple area has to be fed into the grid, and there exists a significant difference in the amount of PV production between mid-season and winter days. Figure 15 presents the detailed operational scenario of a typical ZEH equipped with PV and fuel cell CHP systems in different seasonal weeks; variation in household heating demand has a significant impact on the operational performance of the fuel cell. In general, micro-CHP is operated with the thermal load-following strategy; the maximum output is limited to heating demand. It can be noted that the operations of the micro-CHP system have significant differences in power output between winter and summer days. Compared to winter days, operational hours of the CHP system would be shortened in summer days; this is the effect of the lower domestic hot water needs, resulting in a reduction in daily household energy contribution from micro-CHP. The duration of the fuel cell working period increases with increasing thermal load in the colder week, and the remaining electricity demand would be covered by the grid import. Overall, the generation of the PV system varies significantly; the mismatch between simultaneous electricity load and PV generation profiles resulted in a significant amount of PV production sold into the grid, and the net load volatility significantly increases, especially in mid-season days. After introducing a micro-CHP system to replace the heat pump unit, the variance of net load in Figure 15 is reduced when compared to the scenario in Figure 14.
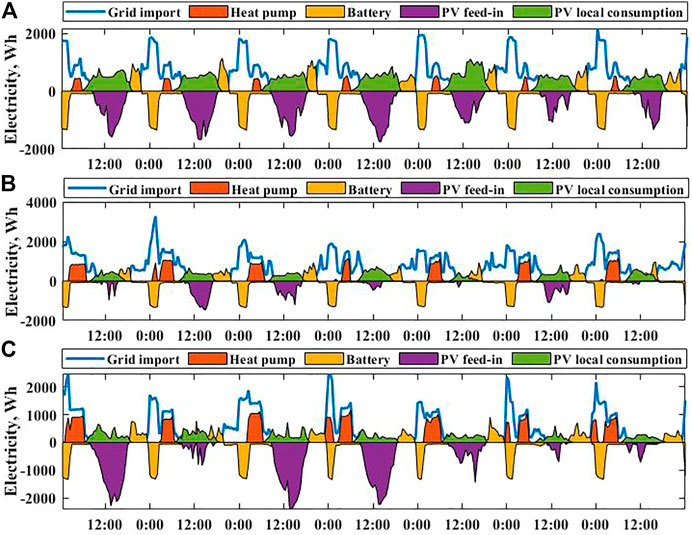
FIGURE 14. Weekly power flows of typical ZEH equipped with PV, battery, and heat pump systems at 30-min intervals in summer from 01 August to 07 August (A), winter from 01 January to 07 January (B), and mid-season from 01 to 07 April (C).
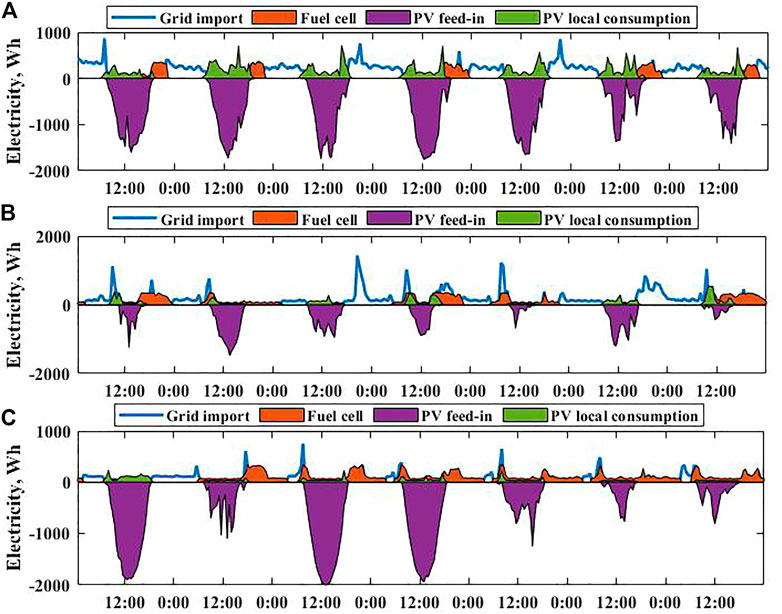
FIGURE 15. Weekly power flows of typical ZEH equipped with PV and fuel cell CHP systems at 30-min intervals in summer 01 August to 07 August (A), winter 01 January to 07 January (B), and mid-season 01 to 07 April (C).
Most zero-energy buildings are grid-connected; the rapid development of grid-connected ZEH raises concerns about grid reliability. The falling trend of the residential rooftop PV feed-in tariff rate is continuous, which has experienced a dropped from 48 Yen/kWh in 2011 to 17 Yen/kWh in 2022. The district low-carbon energy system transition and cost-efficient efforts should emphasize increasing local PV self-consumption through demand-side solutions, for example, by shifting household heat pump operation or the battery charging period to be more consistent with the generation period of the PV system.
5 Conclusion
This work has introduced smart practices and management innovations in the Japanese net-zero-carbon district demonstration project. An in-depth questionnaire survey was carried out to examine their attitude, which explored to what extent households engaged in low-carbon energy system management. The analysis of behind smart meters examined the operational behaviors of the distributed energy technologies among ZEHs.
Regarding ZEHs’ values and customers’ attitudes, we used a field survey to identify the end users’ perceptions of the potential delivered benefits of smart ZEHs and investigate the effectiveness of the IoT-enabled HEMS services. The results reveal that a large ratio of the ZEH owners responded positively toward aspects of electricity consumption and carbon emission reduction. Indeed, findings doubt the effectiveness of in-home display and visual feedback in inducing behavior changes toward actively adjusting their energy consumption, and consumers are less likely to access the information delivered from the CEMS feedback. Furthermore, the results reveal that household income has less impact on the HEMS interaction of occupants; older people tend to visualize more of the HEMS delivered information.
Zero-carbon transition changes the connectivity between household and community. Monitored data of selected typical ZEHs provided insights into the detailed operational performances under HEMS control. Detailed roles of integrated energy-efficient technologies in ZEH are clearly illustrated over typical weekly periods. The energy bill saving chance can be guaranteed by price-based load shift and the feed-in tariff scheme for renewable generation. The PV system plays a key role in achieving annual net-zero-energy exchange between household and power utility. With the integration of distributed energy resources, the pattern of net energy load was significantly shaped. Future efforts toward ZEH need to focus more on energy-efficient systems and be responsive to relieve the imbalance between PV generation and consumer load profiles.
The trial findings of this work provide a detailed consumers’ awareness of a residential zero-carbon district demonstration project; it could be particularly informative to guide future policy making. Analysis of behind-meter data helps decide the most appropriate technologies to be encouraged toward zero-energy house governance, especially considering the effective energy conservation by the end-user active engagement. The findings of this work are expected to enhance the knowledge, offer implications, and guide the management with regard to smart zero-energy buildings.
Data Availability Statement
The raw data supporting the conclusion of this article will be made available by the authors, without undue reservation.
Ethics Statement
The studies involving human participants were reviewed and approved by Kitakyushu University. The patients/participants provided their written informed consent to participate in this study.
Author Contributions
Conceptualization, YL and WG; methodology, YL and XZ; software, XZ; validation, YL and WG; formal analysis, WG; investigation, YL and XZ; resources, YL and XZ; data curation, YL, JQ and XZ; writing—original draft preparation, YL and XZ; writing—review and editing, YL and XZ; supervision, WG; funding acquisition, YL and WG.
Funding
The study has been supported by Shandong Natural Science Foundation “Research on Flexible District Integrated Energy System under High Penetration Level of Renewable Energy,” grant number ZR2021QE084, and China National Key R&D Program “Research on the energy efficiency and health performance improvement of building operations based on lifecycle carbon emission reduction,” grant number 2018YFE0106100.
Conflict of Interest
The authors declare that the research was conducted in the absence of any commercial or financial relationships that could be construed as a potential conflict of interest.
Publisher’s Note
All claims expressed in this article are solely those of the authors and do not necessarily represent those of their affiliated organizations, or those of the publisher, the editors and the reviewers. Any product that may be evaluated in this article, or claim that may be made by its manufacturer is not guaranteed or endorsed by the publisher.
References
Agency for Natural Resources and Energy M (2019). Residential Energy Consumption, FY2018 Annual Report on Energy.
Azizi, S., Nair, G., and Olofsson, T. (2019). Analysing the House-Owners' Perceptions on Benefits and Barriers of Energy Renovation in Swedish Single-Family Houses. Energy Build. 198, 187–196. doi:10.1016/j.enbuild.2019.05.034
Bandeiras, F., Gomes, M., Coelho, P., and Fernandes, J. (2020). Towards Net-zero-energy in Industrial and Commercial Buildings in Portugal. Renew. Sustain. Energy Rev. 119, 109580. doi:10.1016/j.rser.2019.109580
Bell, S., Judson, E., Bulkeley, H., Powells, G., Capova, K. A., and Lynch, D. (2015). Sociality and Electricity in the United Kingdom: The Influence of Household Dynamics on Everyday Consumption. Energy Res. Soc. Sci. 9, 98–106. doi:10.1016/j.erss.2015.08.027
Brown, D., Hall, S., and Davis, M. E. (2020). What Is Prosumerism for? Exploring the Normative Dimensions of Decentralised Energy Transitions. Energy Res. Soc. Sci. 66, 101475. doi:10.1016/j.erss.2020.101475
Colclough, S., Kinnane, O., Hewitt, N., and Griffiths, P. (2018). Investigation of nZEB Social Housing Built to the Passive House Standard. Energy Build. 179, 344–359. doi:10.1016/j.enbuild.2018.06.069
Creutzig, F., Roy, J., Lamb, W. F., Azevedo, I. M. L., Bruine de Bruin, W., Dalkmann, H., et al. (2018). Towards Demand-Side Solutions for Mitigating Climate Change. Nat. Clim. Change 8, 260–263. doi:10.1038/s41558-018-0121-1
Cruz, C., Palomar, E., Bravo, I., and Aleixandre, M. (2021). Behavioural Patterns in Aggregated Demand Response Developments for Communities Targeting Renewables. Sustain. Cities Soc. 72, 103001. doi:10.1016/j.scs.2021.103001
de Chalendar, J. A., Glynn, P. W., and Benson, S. M. (2019). City-scale Decarbonization Experiments with Integrated Energy Systems. Energy Environ. Sci. 12, 1695–1707. doi:10.1039/c8ee03706j
Diawuo, F. A., Sakah, M., de la Rue du Can, S., Baptista, P. C., and Silva, C. A. (2020). Assessment of Multiple-Based Demand Response Actions for Peak Residential Electricity Reduction in Ghana. Sustain. Cities Soc. 59, 102235. doi:10.1016/j.scs.2020.102235
El Geneidy, R., and Howard, B. (2020). Contracted Energy Flexibility Characteristics of Communities: Analysis of a Control Strategy for Demand Response. Appl. Energy 263, 114600. doi:10.1016/j.apenergy.2020.114600
Energy AfNRa (2020). “10 Questions for Understanding the Current Energy Situation, JAPAN'S EENERGY 2019,” in Ministry of Economy, Trade and Industry.
Energy Efficiency and Conservation Division Agency for Natural Resources and Energy M (2015). Definition of ZEH and Future Measures Proposed by the ZEH Roadmap Examniation Committee.
EPIE BPIE (2020). THE ZERO-CARBON AND CIRCULAR ECONOMY CHALLENGE IN THE BUILT ENVIRONMENTPOLICY OPTIONS FOR THE EUROPEAN UNION AND ITS MEMBER STATES.
Ganji, M., and Shahidehpour, M. (2018). “Development of a Residential Microgrid Using Home Energy Management Systems,” in Application of Smart Grid Technologies, 173–192. doi:10.1016/b978-0-12-803128-5.00005-2
Grinham, J., Fjeldheim, H., Yan, B., Helge, T. D., Edwards, K., Hegli, T., et al. (2022). Zero-carbon Balance: The Case of HouseZero. Build. Environ. 207, 108511. doi:10.1016/j.buildenv.2021.108511
Haghifam, S., Dadashi, M., Zare, K., and Seyedi, H. (2020). Optimal Operation of Smart Distribution Networks in the Presence of Demand Response Aggregators and Microgrid Owners: A Multi Follower Bi-level Approach. Sustain. Cities Soc. 55, 102033. doi:10.1016/j.scs.2020.102033
Hargreaves, T., Nye, M., and Burgess, J. (2010). Making Energy Visible: A Qualitative Field Study of How Householders Interact with Feedback from Smart Energy Monitors. Energy Policy 38, 6111–6119. doi:10.1016/j.enpol.2010.05.068
Heine, K., Thatte, A., and Tabares-Velasco, P. C. (2019). A Simulation Approach to Sizing Batteries for Integration with Net-Zero-energy Residential Buildings. Renew. Energy 139, 176–185. doi:10.1016/j.renene.2019.02.033
Isshiki, M., Umejima, M., Hirahara, M., Minemura, T., Murakami, T., and Owada, S. (2015). “Case Study of an Ecological, Smart Home Network,” in Ecological Design of Smart Home Networks, 91–111. doi:10.1016/b978-1-78242-119-1.00006-0
Kalaycıoğlu, E., and Yılmaz, A. Z. (2017). A New Approach for the Application of Nearly Zero-energy Concept at District Level to Reach EPBD Recast Requirements through a Case Study in Turkey. Energy Build. 152, 680–700. doi:10.1016/j.enbuild.2017.07.040
Koutra, S., Becue, V., Gallas, M.-A., and Ioakimidis, C. S. (2018). Towards the Development of a Net-Zero-energy District Evaluation Approach: A Review of Sustainable Approaches and Assessment Tools. Sustain. Cities Soc. 39, 784–800. doi:10.1016/j.scs.2018.03.011
Li, Y., Gao, W., Ruan, Y., and Ushifusa, Y. (2018). Demand Response of Customers in Kitakyushu Smart Community Project to Critical Peak Pricing of Electricity. Energy Build. 168, 251–260. doi:10.1016/j.enbuild.2018.03.029
Li, Y., Gao, W., Zhang, X., Ruan, Y., Ushifusa, Y., and Hiroatsu, F. (2020). Techno-economic Performance Analysis of Zero-energy House Applications with Home Energy Management System in Japan. Energy Build. 214, 109862. doi:10.1016/j.enbuild.2020.109862
Li, Y., Zhang, X., Gao, W., Xu, W., and Wang, Z. (2022). Operational Performance and Grid-Support Assessment of Distributed Flexibility Practices Among Residential Prosumers under High PV Penetration. Energy 238, 121824. doi:10.1016/j.energy.2021.121824
Mah, D. N.-y., Wu, Y.-Y., Ip, J. C.-m., and Hills, P. R. (2013). The Role of the State in Sustainable Energy Transitions: A Case Study of Large Smart Grid Demonstration Projects in Japan. Energy Policy 63, 726–737. doi:10.1016/j.enpol.2013.07.106
Mengolini, A., Gangale, F., and Vasiljevska, J. (2016). Exploring Community-Oriented Approaches in Demand Side Management Projects in Europe. Sustainability 8, 1266. doi:10.3390/su8121266
Oki, R., Tsuneoka, Y., Yamaguchi, S., Sugano, S., Watanabe, N., Akimoto, T., et al. (2019). Renovating a House to Aim for Net-Zero-energy, Thermal Comfort, Energy Self-Consumption and Behavioural Adaptation: A Method Proposed for ENEMANE HOUSE 2017. Energy Build. 201, 183–193. doi:10.1016/j.enbuild.2019.06.041
Ozaki, R. (2018). Follow the Price Signal: People's Willingness to Shift Household Practices in a Dynamic Time-Of-Use Tariff Trial in the United Kingdom. Energy Res. Soc. Sci. 46, 10–18. doi:10.1016/j.erss.2018.06.008
Pan, W., and Pan, M. (2018). A Dialectical System Framework of Zero-carbon Emission Building Policy for High-Rise High-Density Cities: Perspectives from Hong Kong. J. Clean. Prod. 205, 1–13. doi:10.1016/j.jclepro.2018.09.025
Panagiotidou, M., and Fuller, R. J. (2013). Progress in ZEBs-A Review of Definitions, Policies and Construction Activity. Energy Policy 62, 196–206. doi:10.1016/j.enpol.2013.06.099
Parrish, B., Heptonstall, P., Gross, R., and Sovacool, B. K. (2020). A Systematic Review of Motivations, Enablers and Barriers for Consumer Engagement with Residential Demand Response. Energy Policy 138, 111221. doi:10.1016/j.enpol.2019.111221
Radenković, M., Bogdanović, Z., Despotović-Zrakić, M., Labus, A., and Lazarević, S. (2020). Assessing Consumer Readiness for Participation in IoT-Based Demand Response Business Models. Technol. Forecast. Soc. Change 150, 119715. doi:10.1016/j.techfore.2019.119715
Rajkovich, N. B., Miller, W. C., and Risser, R. J. (2013). The Prospect of Zero Net Energy Buildings in the United States. Energy Effic.. Editor F. P. Sioshansi (Boston: Academic Press), 253–274. doi:10.1016/b978-0-12-397879-0.00010-4
Rathnayaka, A. J. D., Potdar, V. M., Dillon, T., Hussain, O., and Kuruppu, S. (2014). Goal-oriented Prosumer Community Groups for the Smart Grid. IEEE Technol. Soc. Mag. 33, 41–48. doi:10.1109/mts.2014.2301859
Ren, H., Wu, Q., Gao, W., and Zhou, W. (2016). Optimal Operation of a Grid-Connected Hybrid PV/fuel Cell/battery Energy System for Residential Applications. Energy 113, 702–712. doi:10.1016/j.energy.2016.07.091
Rodrigues, L., Gillott, M., Waldron, J., Cameron, L., Tubelo, R., Shipman, R., et al. (2020). User Engagement in Community Energy Schemes: A Case Study at the Trent Basin in Nottingham, UK. Sustain. Cities Soc. 61, 102187. doi:10.1016/j.scs.2020.102187
Rogelj, J., Luderer, G., Pietzcker, R. C., Kriegler, E., Schaeffer, M., Krey, V., et al. (2015). Energy System Transformations for Limiting End-Of-Century Warming to below 1.5 °C. Nat. Clim. Change 5, 519–527. doi:10.1038/nclimate2572
Sameti, M., and Haghighat, F. (2018). Integration of Distributed Energy Storage into Net-Zero-energy District Systems: Optimum Design and Operation. Energy 153, 575–591. doi:10.1016/j.energy.2018.04.064
Sattarpour, T., Nazarpour, D., and Golshannavaz, S. (2018). A Multi-Objective HEM Strategy for Smart Home Energy Scheduling: A Collaborative Approach to Support Microgrid Operation. Sustain. Cities Soc. 37, 26–33. doi:10.1016/j.scs.2017.09.037
Shigetomi, Y., Matsumoto, K. i., Ogawa, Y., Shiraki, H., Yamamoto, Y., Ochi, Y., et al. (2018). Driving Forces Underlying Sub-national Carbon Dioxide Emissions within the Household Sector and Implications for the Paris Agreement Targets in Japan. Appl. Energy 228, 2321–2332. doi:10.1016/j.apenergy.2018.07.057
Shimoda, Y., Sugiyama, M., Nishimoto, R., and Momonoki, T. (2021). Evaluating Decarbonization Scenarios and Energy Management Requirement for the Residential Sector in Japan through Bottom-Up Simulations of Energy End-Use Demand in 2050. Appl. Energy 303, 117510. doi:10.1016/j.apenergy.2021.117510
Tachmitzaki, E. V., Didaskalou, E. A., and Georgakellos, D. A. (2019). Energy Management Practices' Determinants in Greek Enterprises. Sustainability 12, 133. doi:10.3390/su12010133
Tiefenbeck, V. (2017). Bring Behaviour into the Digital Transformation. Nat. Energy 2, 17085. doi:10.1038/nenergy.2017.85
Tiefenbeck, V., Wörner, A., Schöb, S., Fleisch, E., and Staake, T. (2018). Real-time Feedback Promotes Energy Conservation in the Absence of Volunteer Selection Bias and Monetary Incentives. Nat. Energy 4, 35–41. doi:10.1038/s41560-018-0282-1
Verkade, N., and Höffken, J. (2019). Collective Energy Practices: A Practice-Based Approach to Civic Energy Communities and the Energy System. Sustainability 11, 3230. doi:10.3390/su11113230
Yan, X., Ozturk, Y., Hu, Z., and Song, Y. (2018). A Review on Price-Driven Residential Demand Response. Renew. Sustain. Energy Rev. 96, 411–419. doi:10.1016/j.rser.2018.08.003
Zafar, R., Mahmood, A., Razzaq, S., Ali, W., Naeem, U., and Shehzad, K. (2018). Prosumer Based Energy Management and Sharing in Smart Grid. Renew. Sustain. Energy Rev. 82, 1675–1684. doi:10.1016/j.rser.2017.07.018
Zeiler, W., Gvozdenović, K., de Bont, K., and Maassen, W. (2016). Toward Cost-Effective Nearly Zero-energy Buildings: The Dutch Situation. Sci. Technol. Built Environ. 22, 911–927. doi:10.1080/23744731.2016.1187552
Zhang, X., Gao, W., Li, Y., Wang, Z., Ushifusa, Y., and Ruan, Y. (2021). Operational Performance and Load Flexibility Analysis of Japanese Zero-energy House. Int. J. Environ. Res. Public Health 18, 6782. doi:10.3390/ijerph18136782
Keywords: ZEH, HEMS, demand-side participation, questionnaire survey, smart behavioral practice
Citation: Li Y, Zhang X, Gao W and Qiao J (2022) Lessons Learnt From the Residential Zero Carbon District Demonstration Project, Governance Practice, Customer Response, and Zero-energy House Operation in Japan. Front. Energy Res. 10:915088. doi: 10.3389/fenrg.2022.915088
Received: 07 April 2022; Accepted: 20 May 2022;
Published: 17 June 2022.
Edited by:
Hasim Altan, Arkin University of Creative Arts and Design (ARUCAD), CyprusReviewed by:
Michelangelo Scorpio, University of Campania Luigi Vanvitelli, ItalyZhe Wang, Hong Kong University of Science and Technology, Hong Kong SAR, China
Copyright © 2022 Li, Zhang, Gao and Qiao. This is an open-access article distributed under the terms of the Creative Commons Attribution License (CC BY). The use, distribution or reproduction in other forums is permitted, provided the original author(s) and the copyright owner(s) are credited and that the original publication in this journal is cited, in accordance with accepted academic practice. No use, distribution or reproduction is permitted which does not comply with these terms.
*Correspondence: Yanxue Li, liyanxue@qut.edu.cn