- 1School of Energy and Power Engineering, Jiangsu University, Zhenjiang, China
- 2Department of Applied Chemistry, Government College University, Faisalabad, Pakistan
- 3Department of Agricultural Engineering, Bahauddin Zakariya University, Multan, Pakistan
- 4Department of Mechanical Engineering, University of Engineering and Technology Lahore, Lahore, Pakistan
- 5Nanjing University of Information Science and Technology, Jiangsu, China
- 6School of Environmental and Municipal Engineering, Xian University of Architecture and Technology, Shanxi, China
- 7Mechanical Engineering Department, Abu Dhabi University, Abu Dhabi, United Arab Emirates
The utilization of biomass for cooking and heating is old, occurring from the early stages of human evolution because of its wide and easy availability. In Asia, a majority of the population is dependent on solid biomass for cooking and heating applications. Biomass cookstove produces emissions like carbon monoxide (CO), and particulate matter with aerodynamic diameter ≤2.5 μm (PM2.5) which are dependent on the classifications and characteristics of fuel used in stoves. These emissions trigger many health risks because of the utilization of traditional cookstoves (TCS) which have less thermal efficiency. The literature contains a considerable amount of information on biomass cookstoves; however, it is dispersed particularly in Asian countries. In this principle, this paper gives an overview of available literature on biomass cookstoves for cooking and heating in Asian countries which are involving Bangladesh, China, India, Mongolia, Nepal, Pakistan, Sri Lanka, and Laos. This paper covers a detailed discussion on various aspects of biomass cookstoves: history, classification, fuel characteristics, health risks, design criteria, the scenario in selected Asian countries, thermal efficiency and emission comparison, and barriers to dissemination of improved biomass cookstoves (ICS). Learning from the review and comparison made conclude that the ICS has better thermal efficiency, and lesser emissions, as well as health risks but, have some potential barriers to dissemination.
Background
In the era of computers and technological gadgets, approximately 2.6 billion people do not have clean cooking facilities, and if forecasts are correct this figure will remain roughly the same into 2030 (IEA, 2012). In Asia, about 1,460 million people rely on the traditional use of biomass for cooking with approximately 57% having access to clean cooking by 2018 (IEA, 2020). Figure 1 shows the percentage of the population with access to clean cooking throughout the world. The use of conventional biomass for cooking causes indoor air pollution (IAP) and human health issues, especially in poorly ventilated facilities (Das et al., 2017; Yip et al., 2017; Goldemberg et al., 2018). The IAP, caused by traditional solid fuel cooking, causes the premature deaths of over 2.6 million people each year in low- and middle-income countries (Haines et al., 2009; Arku et al., 2018). Traditional solid fuel cooking contributes significantly to global greenhouse gas and black carbon emissions, accounting for 1–3% of all human-generated global warming (Smith, 1994). The use of household solid biomass for cooking and heating accounts for around a quarter of all annual anthropogenic black carbon emissions worldwide (Bond et al., 2013). It is expected that 3 billion people in the world are directly dependent on the combustion of biomass that causes IAP in terms of smoke and other hazardous materials (Assad et al., 2015). These most common hazardous materials which contain smoke are CO (carbon monoxide), PM2.5, (fine particular matter with aerodynamic diameter ≤2.5 μm), and CO2 (carbon dioxide) which causes health problems.
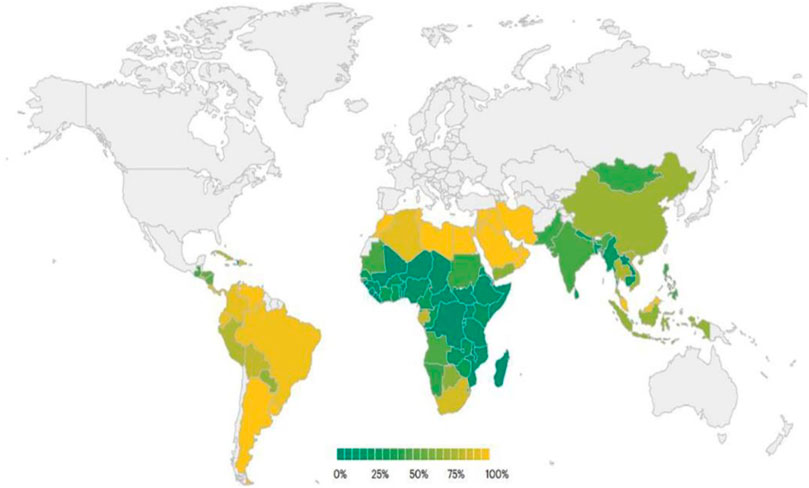
FIGURE 1. Percentage of population access to clean cooking throughout the world (IEA, 2020).
As a solution to the aforementioned global problems, improved biomass cookstoves (ICS) are introduced worldwide to improve cooking efficiency. The motivation behind the development and design of the ICS is to acquire clean burning and reduce negative health effects, emissions, and the quantity of fuel needed. As a result, the expert panel on cookstove technologies set new benchmarks for the ICS at the “Bio-Mass Cookstoves Technical Meeting” in January 2011: “at least 90% emissions reductions and 50% fuel savings over baseline technology (three-stone fire)” (U.S. Department of Energy, 2011). More than 160 cookstove programs are currently active across the world (Ruiz-Mercado et al., 2011).
The major portion of the population in Asian countries is dependent on biomass for cooking and heating purposes. The TCS utilized by these countries has several limitations which are involving, including emissions of CO2, PM2.5, low thermal efficiency, and more fuel consumption. The IAP due to the emissions causes several health risks. The ICS thermal efficiency, emissions performance, and dissemination barriers for the Asian countries are available in the literature, however, these are dispersed. In this regard, this paper is an effort to overview the biomass stoves for cooking and heating scenario in various Asian countries including Bangladesh, China, India, Mongolia, Nepal, Pakistan, Sri Lanka, and Laos. In addition, the study included a comparison of thermal efficiency, CO and PM2.5 emissions of the TCS and ICS, and barriers to dissemination of these ICS in Asian countries. After going through various research conducted on ICS in Asia, we found that the ICS has better thermal efficiency, and emissions performance, thereby fewer health risks. However, we found different barriers to the dissemination of the ICS among the Asian countries such as financial, infrastructure, awareness, market, stove size, and socioeconomic factors. Therefore, these barriers should be the focus of the research community.
Biomass cookstoves
The term “biomass cookstove” refers to a physical structure that incorporates air-fuel combustion for heat release and then transfers that heat to a cooking target. Besides cooking, stoves can be used for space/water heating, in-house lighting, fish/meat smoking, and grain/flour roasting, in addition to cooking. In many cultures, the same device is used for several purposes. Modern cookstoves offer characteristics such as high efficiency, low emissions, and greater user safety than traditional fire stoves. There are a variety of cookstove designs around the world, whether traditional or improved, based on a wide range of food patterns, socio-cultural factors, and fuel types accessible.
History and development
Cookstoves are as old as human history. They have evolved in numerous shapes and sizes, made up of varied materials, and adapted to different cultures and cuisines, with the advent of time. Table 1 shows the history and development of biomass cookstoves with objectives, benefits, and limitations.
Classifications and available technologies of biomass cookstoves
Biomass cookstoves can be classified in a variety of ways. Traditional and improved cookstoves are the two broad categories. The traditional cookstoves have been established for thousands of years in response to community culture and eating habits. These stoves are most affordable, and consumers are acquainted with how to use them, therefore they are broadly accepted in society. The Improved Cookstove is a cook stove that uses scientific concepts to aid better combustion and heat transfer, as well as modern construction to improve emissions and efficiency performance (Kshirsagar and Kalamkar, 2014). In addition, classifications of biomass cookstoves depend on the use of technology, combustion type, construction material, chimney, and portability as represented in Table 2.
The different types of stoves available on the market can be classified differently. The technology of the stove can be classified by the material of construction and whether it is a fixed or portable stove. Additionally, the amount of fuel burned can be determined by how many chimneys the stove has and if it has grates inside the firebox to increase combustion. There are a variety of stoves in use, depending on the location and the type of fuel available. Some stoves are specially designed for burning one fuel; others burn a variety of fuels. A traditional biomass cookstove consists of an air intake and transport system, a bed of fuel, a gas phase combustion zone, and a cooking pot. There are three primary types of traditional household biomass cookstoves based on the treatment of the combustion chamber shown in Figure 2.
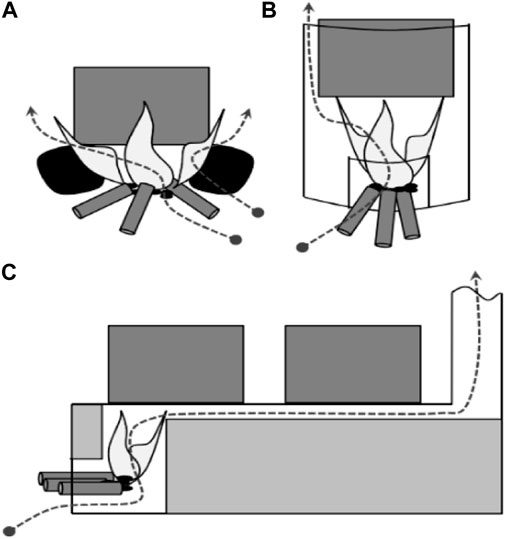
FIGURE 2. Types of cookstoves: (A) Open cooking fire, (B) Shielded cooking fire, (C) Enclosed fire with chimney (MacCarty et al., 2010).
Stove testing standards and protocol
A proper design of stoves enhances the efficiency of combustion ultimately helping to reduce deforestation. The testing of stoves in a standard laboratory leads to improved future designs and also to the adoption of standard terminology. The Global Alliance for Clean Cookstoves (GACC) and International Standards Organization (ISO) have identified a clear need for a common standard for Improved Cookstoves and are working in an attempt to standardize test procedures for all cookstoves. Major stove testing protocol categories include 1) Water Boiling Test (WBT), 2) Heterogeneous Testing Protocol (HTP), which are lab-based tests. The procedure is repeated for any pot/fuel/stove combination tested. The Controlled Cooking Test (CCT) Kitchen Performance Test (KPT) and Uncontrolled Field Test (UFT) are field based tests. The WBT, CCT, and KPT are the most accepted testing protocols for cookstoves.
The WBT is a lab-based test using the laboratory emissions monitoring system (LEMS). The test measures and analyses thermal efficiency, emissions (CO2, CO, and PM), fuel to boil 5 L of water, energy to boil 5 L of water, and time to boil 5 L of water. Thermal efficiency and emissions are measured in Tiers with Tier 4 being the highest performance and Tier 0 being the lowest. The minimum accepted rating by the Global Alliance for Clean Cookstoves is Tier 2 for these 2 parameters. The CCT is designed to assess the performance of the improved stove relative to the common or traditional stoves that the improved model is meant to replace. Stoves are compared on how they perform a standard cooking task that is closer to the actual cooking that local people do every day. However, the tests are designed in a way that minimizes the influence of other factors and allows for the test conditions to be reproduced.
The KPT is the principal field–based procedure to demonstrate the effect of stove interventions on household fuel consumption. It includes 1) an assessment of the qualitative aspects of stove performance through household surveys and 2) comparison of the impact of improved stove(s) on fuel consumption in the kitchens of real households. An important parameter assessed in KPT is the safety of the stove. The following are evaluated: sharp edges/points, cookstove and pot tipping, containment of fuel, obstruction near cooking surface, surface temperature, heat transfer to surroundings, cookstove handle temperature, chimney shielding, flames surrounding the pot, and flames exiting the fuel chamber.
Characteristics of fuel used in biomass cookstove
The majority of solid biomass is woody biomass (some of which is turned into charcoal) obtained directly from forests and it is the most commonly used cooking fuel in developing countries. Solid biomass fuels, which go beyond firewood and charcoal, can be made from agricultural waste and forest wastes, and are becoming significantly more popular. For example, agriculture produces an estimated 140 billion tonnes of biomass residues per year, which is comparable to 50 billion tonnes of oil (UNEP, 2009). Cookstoves can burn a variety of solid biomass fuels like crop waste, dung, wood, charcoal, briquettes, pellets, coal, and woodchips (Sweeney, 2017). Figure 3 shows households in millions (m) or billions 2) relying on solid fuel use for cooking and heating throughout the world. The most common methods for obtaining energy from solid biomass are gasification, direct burning, pyrolysis, liquefaction, anaerobic absorption, alcoholic fermentation, and transesterification (Ahmad et al., 2019). Between the middle of the 1980s and the early 1990s, China designed several coal- and briquette-burning stoves (Gujral, 1992). Although some cookstove manufacturers claim that their stoves can burn a variety of fuels, however mostly cookstoves are built to burn only one type of fuel. Densified biomass pellets as a source of energy have a great influence on low-income populations due to their ease of accessibility, low price, and sustainability. Furthermore, biomass briquettes as a source of energy are carbon neutral and have no impact on the environment (Abbas et al., 2022). In terms of innovation, aside from the energy savings over wood, charcoal may have minimal respiratory health risks to consumers compared to a variety of other traditional fuels (Santín et al., 2015; Sundberg et al., 2020).
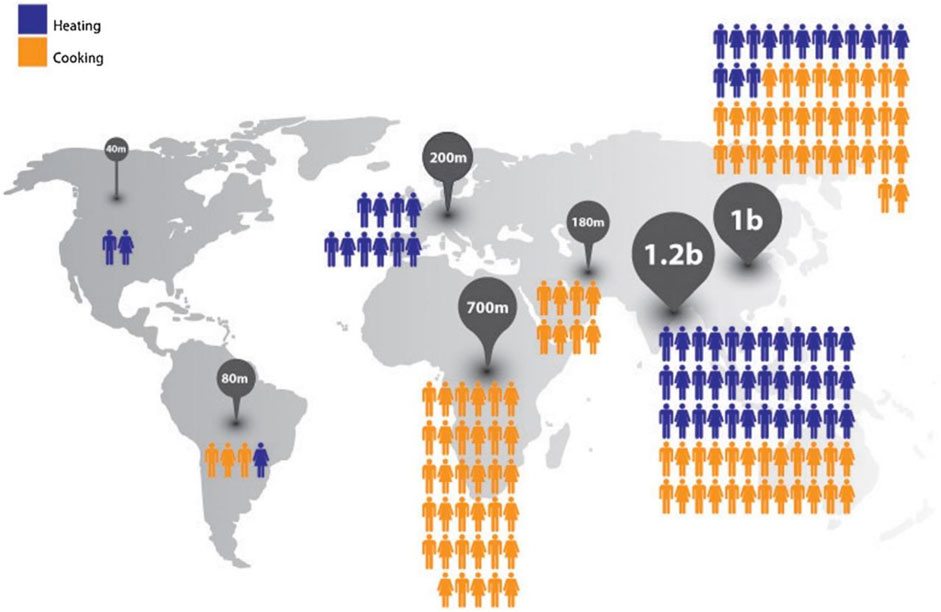
FIGURE 3. Million (m), billion (b) of households rely on solid fuels for cooking and heating throughout the world (Carvalho et al., 2016).
Coal is also an important source of energy for domestic cooking and heating in Asian countries. Coal is a type of carbon that contains a solid, black substance (Ahmad et al., 2022), that is found underground and is one of the most popular fossil fuels (Rahimi et al., 2020). Agricultural residues are non-edible components of plants that are left in rural areas, such as leaves, stalks, straws, husks, shells, peels, and so on. Wood fuels and agricultural residues differ in that they have higher ash, and volatile matter content, low density, and lower energy content. Densifying the crop residues enhances their properties for cooking. Briquettes, also known as cakes, rods, or pellets, are biomass materials made (or densified/extruded) in a variety of sizes and produced using crop wastes, recycled materials, or other materials such as sawdust. In developing countries, pellets or briquettes/cakes can usually be used with a variety of improving biomass stoves (Ahmad et al., 2021a). The types and characteristics of solid fuel for cooking and heating are represented in Figure 4. In rural areas of developing countries, the technique of fuel stacking is very common. Fuel stacking occurs when a home uses multiple fuel types regularly. The fuel stacking depends on the meal to be cooked, the availability of various kinds of fuels, and the family’s present financial situation (Johnson and Bryden, 2012).
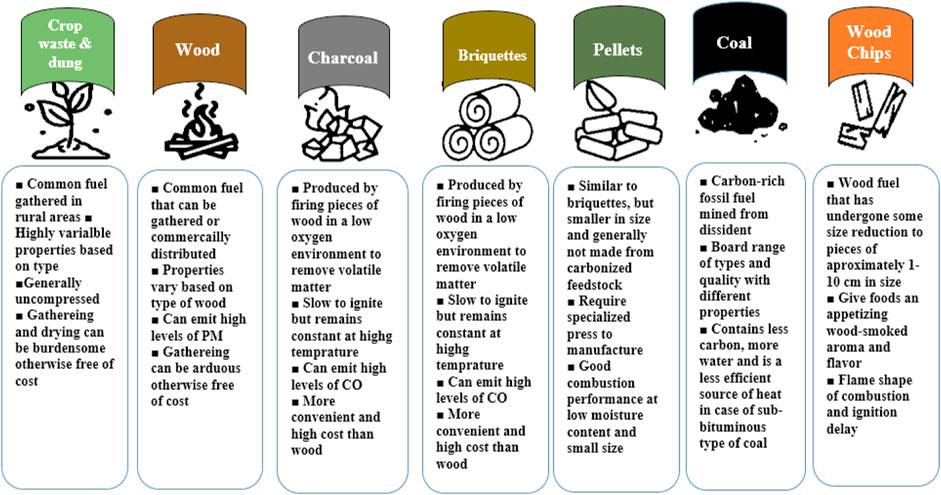
FIGURE 4. Types and characteristics of solid fuel for cooking and heating, data are obtained from (Sweeney, 2017).
Health risks of biomass cookstoves emissions
Approximately three billion people worldwide are exposed to indoor air pollution (IAP) because of solid fuel (wood, charcoal, coal, dung, and crop residues) cookstove emissions (Bonjour et al., 2013). The IAP is generated by inefficient solid fuel combustion, which has several immediate negative effects on human health, particularly among small children and their mothers. According to the 2013 Global Burden of Disease Study (GBD), the IAP accounts for between 3 and 5% of the GBD in terms of disability-adjusted life years, with roughly one-third of those affected being children under the age of five and the rest being adults (Steenland et al., 2018). Carbon monoxide (CO), and PM2.5 (fine particular matter with aerodynamic diameter ≤2.5 μm) are major emissions associated with the IAP and have significant health risks. Respiratory (cough, and dyspnea), and childhood pneumonia are acute health effects while longer-term exposure has been linked to an increased prevalence of chronic diseases such as blood pressure or hypertension, low birth weight, cardiovascular diseases, lung cancer, mutagenicity, and other kinds of neoplasia. Figure 5 shows possible health risks of solid fuel cookstoves emissions. The ICS can be served as an intervention to lessen the health risks by lowering emissions from cookstoves. A recent study has reported the ICS as an intervention to minimize health risks (Pratiti et al., 2020). The authors concluded that the ICS is effective in reducing the effect of air pollution on blood pressure in women over the age of 40 and women who use ICS have a higher health-related quality of life. The use of ICS to tackle childhood pneumonia, birthweight, and cardiovascular diseases is not beneficial, thereby still needs to improve.
Design criteria used for biomass cookstoves
Numerous designs of biomass cookstoves have been developed which can be classified according to the materials used in their construction, the number of pots used, the types of fuel used, and so on (Mehetre et al., 2017). However, there are three major aspects while designing of cookstoves which are involving social, technical, and economical aspects. Social aspects are based on current cultural and local demands and limits and are a prerequisite for the society’s long-term adoption of a cookstove. The present research and development operations in cookstove design are centered on technical aspects such as high efficiency, low emissions, material durability, and user safety (Kshirsagar and Kalamkar, 2014). Finally, the economic factor dictates the investment’s return period, and is thus critical for effective cookstove adoption. Most of the major and sub-criteria listed in Figure 5 are interdependent, despite being classed in distinct categories. For example, technological and economic issues, are inextricably linked, as the cost effectiveness of a stove is usually determined by its technical capabilities.
The ICS reduces the load of fuel collection on women as well as children and saves time due to less firewood consumption. The biomass cooking and heating stoves should be manufactured in such an approach that is appropriate to end-users. While designing the ICS, some general steps should be considered. Firstly, the ICS design must be low-cost and energy efficient as compared to open fire. Second, the ICS design must have the potential to combat heavy use for at least 1 year. Thirdly, the ICS design must be able to be constructed using locally available resources and apparatus. In addition, the scientific understanding, engineering principles, and concepts including are significant for cookstove designing. The steps toward products for household use indicate that one who is going to design the stove should have a better scientific understanding and knowledge about engineering principles as shown in Figure 6.
Safety protocol and modern tool usage
A safety evaluation should be part of the design process for stoves. Humanitarian and private sector organizations have made a commitment to increase the adoption of improved cookstoves (ICS) in low- and middle-income countries in order to mitigate pollution, deforestation, and injuries caused by their use. A timeline of the evolution of laboratory-based protocols is shown in Figure 7. (Kshirsagar and Kalamkar, 2014). provided detailed information regarding testing protocol and modern technology usage in his study.
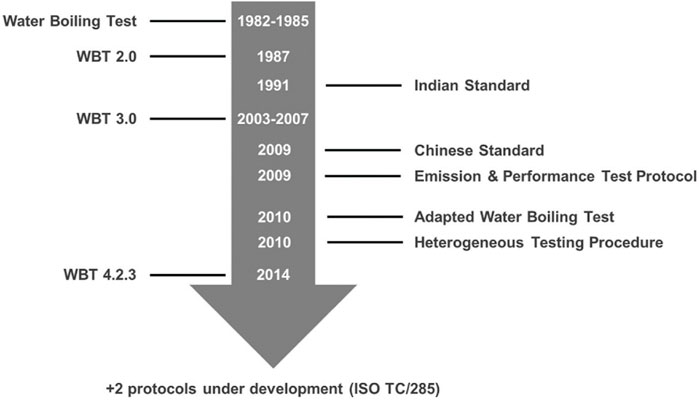
FIGURE 7. Summary of protocols evolution over time (Lombardi et al., 2017).
As part of the International Workshop Agreement (IWA), cookstoves are rated against a series of performance indicators, including Fuel Use (Efficiency), Emissions (Carbon Monoxide and Particulate Matter 2.5), Indoor Emissions (Carbon Monoxide and Particulate Matter 2.5), and Safety. Rather than select a single laboratory protocol to determine cookstove performance, this International Workshop Agreement will enable stove testers to utilize laboratory protocols most appropriate for the stove and performance indicator being tested. The following minimum equipment or methodology is required for certified testing of emissions, performance, and indoor emissions:
1) For carbon monoxide emissions or room measurement: non-dispersive infrared (with calibration consistent with U.S. EPA 40 CFR Part 60,) or electrochemical cell (with pre/post calibration method).
2) For particulate matter emission or indoor air quality measurement: 1) real-time measurement of a particulate matter proxy via light scattering, and 2) PM2.5 gravimetric measurement such as U.S. EPA 40 CFR Part 60, Appendix A, Method 5.
3) For emissions exhaust gas flow: constant volume pump or flow grid both with real-time temperature and pressure correction consistent with U.S. EPA 40 CFR Part 60, Appendix A, Method 1 or 2d, or equivalent.
4) For temperature measurement: Type K thermocouple or equivalent.
5) Computer data logging of all measurements with a minimum time resolution of one measurement per 10 seconds.
6) For measuring fuel and water masses, a calibrated digital scale with 1-g resolution or better.
Computational fluid dynamics and modeling of household biomass cookstoves
The use of computational fluid dynamics (CFD) and modeling in conceptual design can improve the speed, accuracy, and efficiency of cookstoves. Regarding stove design, it provides details of assumptions, theory, results, and validation. CFD provides an accurate means of analyzing the stove’s thermal and emission characteristics. Once it has been experimentally validated, CFD can be used as a standalone tool, in addition to preliminary experimentation (Pande et al., 2020). A CFD-assisted optimization biomass cookstove was developed and tested, incorporating geometric design modifications to achieve uniform air-fuel distribution. As shown in Figure 8, in a recent study, optimal power output and temperatures were obtained when the grate was placed at a height of 25 mm, and the 15-hole plate was located at a height of 105 mm from the bottom of the cookstove.
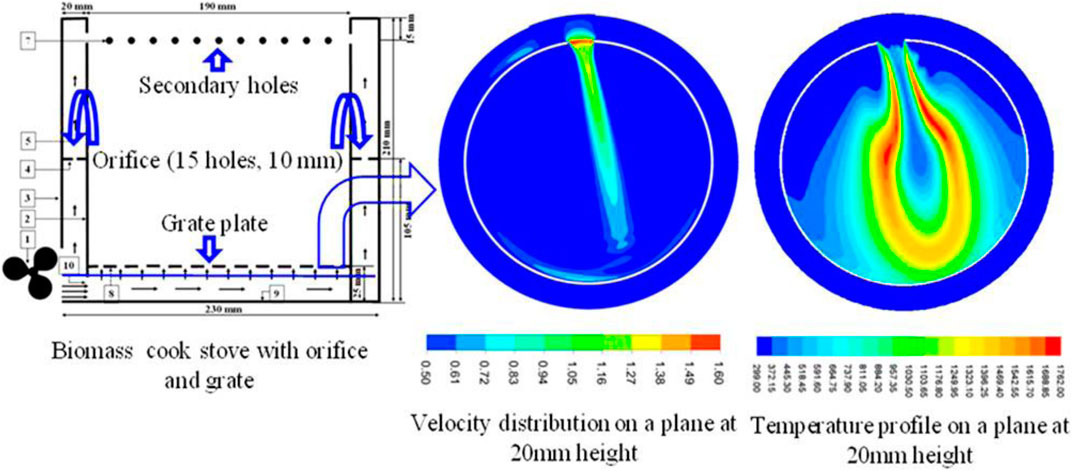
FIGURE 8. A CFD assisted optimized biomass cookstove designed with geometric design modification (Husain et al., 2019).
The three main zones of the stove system, namely the fuel bed, the flame zone, and the heat transfer zone, play a crucial role in stove design. Correlations between heat transfer and fluid flow can be compiled, as can various methods for radiation heat transfer and combustion heat release. Several recent models include steady-state simplified analytic models of packed bed combustion, generalized correlations for convection and radiation, and Computational Fluid Dynamics (CFD) based heat transfer models. Additionally, there are more than a few factors to take into account in stove modeling, including transient processes; models for combustion of various shapes, sizes, and arrangements of fuel; and pollutant formation. A schematic of a small biomass cookstove of the type that is used in virtually all numerical models of cookstoves is shown in Figure 9.
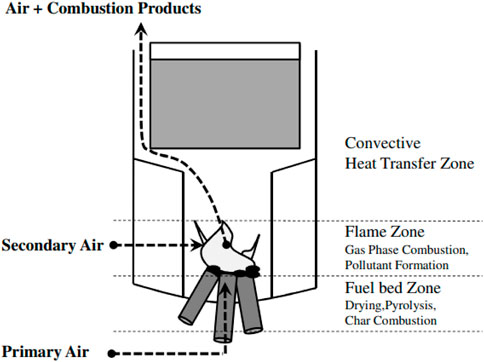
FIGURE 9. Processes within a cookstove (MacCarty et al., 2010).
The purpose of cooking stove modeling is to improve the efficiency of heat transfer by exploring the relationship between the combustion rate, excess air, geometry, and heat transfer. All three major zones of the cookstove system have been described and coupled with zonal models: the reacting fuel bed zone, the gas phase combustion zone, and the heat transfer zone around the cooking pot. Table 3 provides a summary of traditional and improved cookstove modeling efforts.
Application of computational fluid dynamics for combustion modeling of biomass cook stoves
The thermochemical conversion of biomass has attracted a lot of attention over the past 2 decades. Developing reliable designs and scaling up procedures has been the objective. A balanced approach combining experiments and mathematical modeling was effective in achieving the objectives. In addition, there have been significant improvements in the development of efficient and reliable numerical models for simulating hydrodynamics, mixing, combustion, and related phenomena over the past decade (Ngo and Lim, 2020). This has allowed for the detailed analysis of thermochemical reactors such as combustors, pyrolyzers, incinerators, gasifiers, etc. In order to analyze and optimize the above-mentioned thermochemical reactors, CFD simulations have been conducted continuously (Sedighi and Salarian, 2017).
A computational model for the conceptual design of biomass cookstoves would enable a more accurate, faster, and more adaptive understanding of heat transfer and flow within the stove (Commeh et al., 2022). Experimental data sets with thermal performance characteristics in terms of design characteristics are required for model validation. Numerical models of stoves can be divided into two types. Zone models combine processes into zones and calculate efficiency, excess airflow, and average temperatures throughout a system, and may also indicate emissions. Within the prescribed design space, zonal models are fast and flexible, with various measurements available for validation. For the development of a zonal model, design variables include 1) geometry, 2) materials composing the flow path, as well as 3) operational variables such as fuel supply and the power of the flame. Thermal efficiency can be determined by dividing the energy transfer into the pot measured by water temperature rise and evaporation by the energy released by the fuel measured by lower heating value and mass of fuel used during the test. Based on this, the following data are needed for input into the model: 1) Operational variables, including experimental firepower, fuel moisture content, and lower heating value, 2) Geometrical variables providing a full description of the flow path, stove body, and cooking pot dimensions (Figure 10) subject to the constraints of the model, 3) Material variables such that the thermal conductivity of the stove body components can be determined, 4) Thermal efficiency as measured.
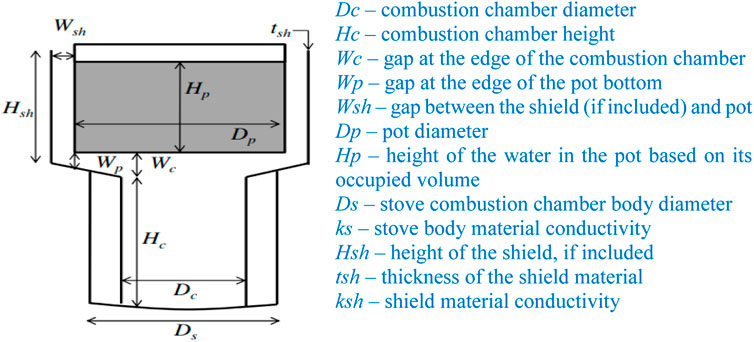
FIGURE 10. Geometrical variables (Maccarty et al., 2013).
User-centric design, experimental and optimization techniques
Globally, biomass cookstoves have been implemented in a variety of ways, and many more are being developed. The adoption rates and effects of new cooking technologies in developing countries have been explained with different models (Ruiz-Mercado et al., 2011). Traditional “energy ladder” models were defined originally (Van Der Kroon et al., 2013). It holds that traditional devices are completely replaced by modern alternatives as a family’s income increases. Transitions are not unidirectional, and people evolve to a “multiple fuel” model as they move up the energy ladder, while maintaining their traditional sources. Researchers have made many new designs and changes to cooking stoves, but users have not adapted to them (Bosque et al., 2021). In order to facilitate the adoption of improved cookstoves and ensure their success, theoretical analyses and user experiences are vital.
There is a distinct cooking situation for every cuisine. The range of cooking power and shape and size of utensils required are almost rigid at the user’s end (Kshirsagar and Kalamkar, 2016). Researchers are focusing on the development of biomass cookstoves that can burn biomass more efficiently, which requires an understanding of pollution emissions caused by different parameters. Using the multi-factor parameters Inlet area ratio, Primary air ratio, Pot gap, Fuel surface to volume ratio, and Pot diameter, ((Kshirsagar et al., 2020) improved the combustion performance of biomass cookstoves. A central composite design combined with Response Surface Methodology and desirability function was found to be an effective way to optimize a natural draft biomass cookstove’s performance. Detailed information on user-centric sizing of biomass cookstoves subjected to different constraints is presented with a mathematical equation (Kshirsagar and Kalamkar, 2016; Kshirsagar et al., 2020).
Use of forced draft or hybrid draft in modern-day stoves
Technology-based cookstove designs have been introduced to the markets of developing countries for domestic and institutional use. Most developing countries face challenges with the sustainability of biomass cookstove designs that have been churned out over the years (Gill-Wiehl et al., 2021). Nowadays, forced drafts and hybrid drafts are very popular. An effective method of increasing air-fuel mixing and combustion is to create turbulence using forced draft. Several factors contributed to the high capital costs, including fan design, manufacturing technique, and the complexity of the fan. Due to the availability of computer-based fans at a relatively low cost, the problem of high-cost fans has been overcome. For eliminating the running cost of fans, researchers used the thermoelectric generator (TEG) to harness a small fraction of the stove’s thermal energy to provide power on demand for fans and surplus power for providing electricity to remote places (Raman et al., 2014; Najjar and Kseibi, 2017). A hybrid draft stove is a small-capacity combustion device that combines natural drafts and forced drafts. Hybrid draft biomass cookstoves designed with the help of the Central Composite Design and Response Surface methodology can achieve low emissions and be efficient in terms of energy and emissions (Kshirsagar and Kalamkar, 2020). A biomass cook stove with affordable fan power was tested using a hybrid draft to reduce CO and PM2.5 emissions. Based on a mathematical model for a hybrid graft stove, a spreadsheet was used to determine the dimensions of the prototype using Visual Basic for Applications. Figure 11 shows a hybrid draft prototype that has 30% higher efficiency than seven other models (Vicente and Alves, 2018; Zhang et al., 2019).
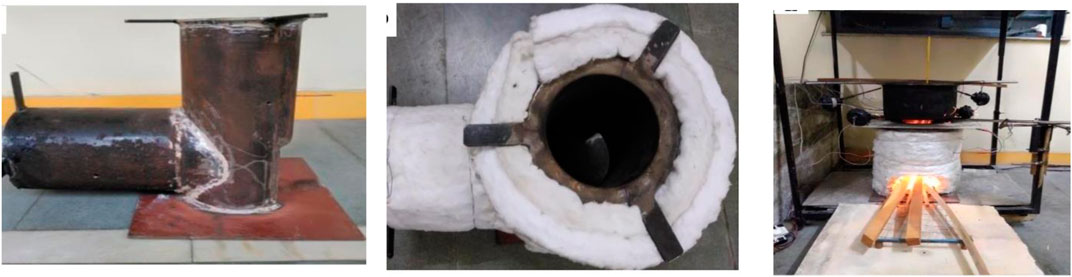
FIGURE 11. The high-efficiency hybrid draft stove prototype (Kshirsagar and Kalamkar, 2022).
Biomass stoves scenario in Asia
Around the world, many cookstove programs have been implemented. Those programs aim to reduce fuel consumption in order to reduce deforestation, and to improve health conditions by reducing emissions and therefore environmental pollution. Other objectives include improving social conditions in developing countries and reducing global warming. Improvements to Cookstove programs have reported mixed results. Many of the programs have failed to achieve their target objectives. The improved biomass cookstoves (ICS) have become an important topic of research for more than 40 years, but still 2.6 billion population cook over traditional open fire stoves (Kshirsagar and Kalamkar, 2014). The ICS are biomass cooking stoves that are designed to maximize thermal and fuel efficiency, operate safely, and emit low levels of pollutants that are damaging to human health (Mehetre et al., 2017). Evidence suggests that extensive implementation of cookstoves technology with improvements in energy and combustion efficiencies could significantly help to mitigate adverse human health, energy, and environmental effects (Smith, 1994; Pratiti et al., 2020). According to the International Energy Agency, about half of developing countries’ populations rely on biomass in the form of fuelwood, agricultural residues, animal dung, and biofuel charcoal to meet their cooking and heating needs (International Energy Agency, 2006). Improved cooking stove projects have been launched in most Asian countries in the field of biomass utilization. The programs have been commenced in China, where 129 million stoves had been placed by early 1992, and in India, where 35.2 million stoves had been installed by March 2003 (Rofiqul Islam et al., 2008). Figure 12 represents the percentage of household distribution of improved cookstoves in rural and urban areas of some selected Asian countries.
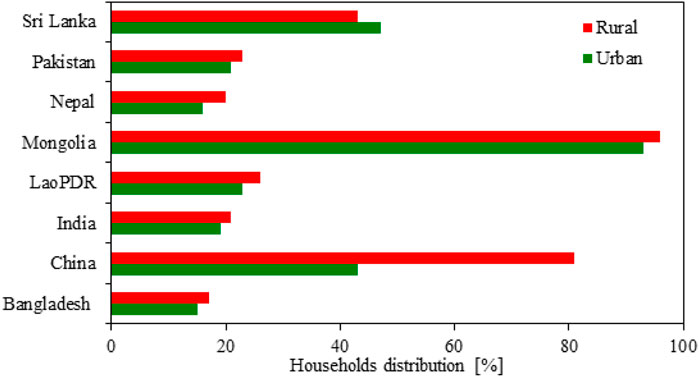
FIGURE 12. Distribution of improved cookstoves (ICS) in rural and urban areas of Asian countries in recent decades (ESCAP, 2018; World Bank, 2014).
Biomass stoves in Bangladesh
In Bangladesh, about 74% of the rural population cooks predominantly with biomass fuels such as straw/leaf (28.6%), husk/bran (4.0%), and jute stick/wood/bamboo (41.2%) (Finance, 2020). The TCS typically consists of a mud-built cylinder with three slightly raised platforms on which utensils are placed. The efficiency of biomass cookstoves is between 5% and 10%, producing greenhouse gases, and creating a health threat in the kitchen. According to the World Health Organization, more than 70,000 people die in Bangladesh each year because of diseases caused by the IAP from the TCS. Despite these health concerns, families have been diffident in moving to cleaner, more efficient cookstoves in the past owing to a lack of understanding and a fear that their meals will lose quality and taste. However, in May 2013, the ICS program began addressing the problem comprehensively by concentrating on the gender and health factors of clean cooking. Furthermore, the ICS program has started assisting women as part of the supply chain for cleaner cookstoves, allowing them to earn money and, as a result, reducing poverty in rural households (World Bank, 2018). By January 2017, this program has distributed one million improved cookstoves, about 2 years ahead of plan. All the program’s direct beneficiaries were women, and the program’s second phase of execution seeks to reach an estimated five million users by 2021 (World Bank, 2018).
Ahmed and Iqbal studied the benefits of improved cookstoves considering Bondhu Chula in rural Bangladesh (Ahmed and Iqbal, 2018). The 2018 household survey data of the Bangladesh Institute of Development Studies, which collected information from 600 users of Bondhu Chula and 396 users of traditional cookstoves, was used to use Propensity Score Matching, a quasi-experiment econometric method. According to the results, homes utilizing Bondhu Chula save roughly 50 kg of biomass fuel per month, or 30–37% of biomass fuel use, when compared to households using the TCS. Bondhu Chula was also proven to improve health outcomes by lowering indoor air pollution and decreasing household cooking time (Ahmed and Iqbal, 2018). Uddin et al. studied thermal performance and emission analysis of metallic, and nonmetallic (cement) improved cookstoves. The authors concluded that metallic improved cookstove has a better thermal performance of about 35–40% as compared to nonmetallic at 20–30%. However, the nonmetallic improved cookstove emits fewer pollutants when a chimney is connected to the exhaust (Uddin et al., 2020).
In Bangladesh, the ICS are available in a variety of fuel (pellets, briquettes, ethanol, and solar) and form/material (cement/clay/concrete, fixed/portable, and locally manufactured/imported) options. Despite its tremendous fuel-saving potential and a three-to 4-month low-cost recovery period, uptake has been slow, with barely 10% countrywide adoption (Finance, 2020). The organizations involved in biomass cookstove-related activities and trying to improve the cookstoves are Bangladesh Energy Research Council, Bangladesh University of Engineering and Technology, Grameen Shakti, German Development Cooperation, Village Education Resource Centre, Bangladesh Council for Scientific and Industrial Research, Building Resources Across Communities, and Bright Green Foundry.
Biomass stoves in China
Most people living in rural areas of China depend on biomass for cooking and space heating. The widespread use of inefficient biomass stoves for cooking and heating in China’s rural areas generates natural and ecological problems; as a result, the Chinese government encouraged the spread of the ICS in 1982. From 1982 to 1994, those ICS were utilized by 144 million household units or around 90% of every improved stove introduced in total which was 62% of the Chinese market (Qiu et al., 1996). However, about 95% of the rural population in China uses wood, coal, and other biomass for cooking and heating resulting in a huge amount of PM2.5 emissions (Bruce et al., 2015; Qu et al., 2015). In this principle, China is focusing on improving biomass stoves for cooking, heating, and low-pressure boilers, thereby constricting high-quality stove testing laboratories (Ahmad et al., 2019). The Key Laboratory of Clean Production and Utilization of Renewable Energy (CPURE), China Agricultural University, Beijing, has constructed a well-designed and modern stove testing lab. The testing system by the laboratory of the CPURE for stoves is shown in Figure 13. Ahmed et al. conducted an experimental study to investigate the performance of the ICS for cooking and heating compared with the TCS by using the testing system for the laboratory of CPURE from viewpoints of thermal and emission efficiency. The authors concluded that the ICS achieved thermal efficiency of 69.6 ± 0.8%, and the lowest emissions of PM2.5 2.9 ± 1.0 mg/MJNET and CO 2.4 ± 0.3 g/MJNET (Ahmad et al., 2021b). Results of the study concluded that utilization of the ICS could result in significant reductions of PM2.5 and CO emissions for a household. The information regarding stove testing and modern lab instruments would be beneficial to other Asian developing countries.
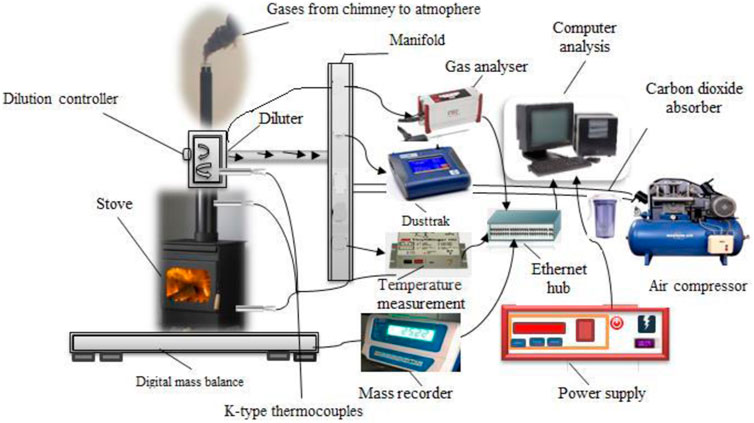
FIGURE 13. Testing system by the laboratory of Clean Production and Utilization of Renewable Energy (CPURE).
Biomass stoves in India
In India, biomass fuels such as wood, crop residues, and animal dung remain the most common source of cooking energy. About 90% of rural households utilized biomass fuel for cooking purposes (Venkataraman et al., 2010). In rural areas, access to modern cooking fuels ranged from 0% for the lowest-income households to 43% for highest-income households (Sinha, 2002). Despite ICS programs like NPIC, and NBCI in the country, a significant portion is still relying on the TCS. Women are the primary users of stoves, but they are frequently excluded from household purchasing decisions, among other decision-making. Improved cookstoves can significantly raise the value of life and the possibilities for women’s future life. Mukhopadhyay et al. did an exploratory study to investigate the improved cookstoves (Phillips and Oorja) performance in Haryana as compared to the TCS in perspective of cooking practice, PM2.5, and CO emissions. The authors considered thirty-two participating households, and they concluded that the participants were satisfied with Phillips cookstove as it meets the local criteria for usability and emission (Mukhopadhyay et al., 2012). Gupta et al. utilized a practical evaluation approach to compare the performance of TCS and ICS which involve Greenway, Envirofit, and Onil with newly designed natural draft ICS from perceptions of multifuel usage, user-friendliness, emissions, and thermal efficiency. The authors found that using solid fuels with a larger energy content in ICS may result in higher efficiency, but it would also result in much higher emissions. The newly designed natural draft ICS attained better performance than TCS and other commercial ICS with a 75% decline in PM2.5 and 63% drop in CO concentrations, respectively. The thermal efficiency of newly designed natural draft ICS achieved a significant increase of 106% from TCS (Gupta et al., 2020). Similarly, Suresh et al., did an experimental study to investigate the performance of two natural draft, and one forced draft ICS as compared to TCS in the preparation of a specific meal using a variety of solid biomass fuels (e.g., fuel wood, dung cake, and crop residue). The study was conducted in Indian rural kitchen, and performance was explored based on emissions and thermal efficiency. The authors found that force draft ICS concentration of PM2.5 and CO were reduced by 21–57%, and 30–74%, respectively as compared to TCS. The thermal efficiency of force draft ICS of 30–35% was found as compared to TCS of 15–17%.
Despite such improvement, the industry is still facing difficulty and government policy has yet to concentrate on market-based solutions in the stove area. The organizations involved in cookstove-related research activities are the Center for Rural Development and Technology, Indian Institute of Technology, The Energy and Resources Institute, Maharana Pratap University of Agriculture and Technology, and Tamil Nadu Agricultural University.
Biomass stoves in Mongolia
Ulaanbaatar is one of the coldest capital cities in the world, with an average low temperature of −20°C. Heating is essential in these conditions, and heat in Ulaanbaatar is generated mostly by coal burning. The ger districts, located on the outer edge of Ulaanbaatar, include between 80,000 and 100,000 additional families that use individual coal stoves for heating and cooking (World Bank, 2014). Because of insufficient coal burning in low-efficiency metal stoves, polluting gases and dust are released, affecting both the interiors of the tents and the overall air quality of the city (Pemberton-pigott, 2006). Mongolia’s largest market, Ulaanbaatar, obtained a very high penetration of improved stoves in a very short period of time. Mongolia’s clean stove endeavor, with its unique problems and near-term success story, has the potential to greatly contribute to the international body of knowledge in the development of cleaner cookstoves. The Mongolian Government and Ulaanbaatar Municipality have developed a multi-year, multi-sector strategy to enhance the air quality in Ulaanbaatar, which includes reducing emissions from heating and cooking (World Bank, 2014).
Pemberton-Pigott et al. developed a low-smoke Mongolian coal stove, and experimentally investigated the performance of the developed natural draft chimney improved stove. The author found about a 99% reduction in PM2.5, and a 90% reduction in CO by utilizing a natural draft chimney improved stove (Pemberton-pigott, 2006). Mongolian households must be persuaded to move permanently to the ICS which will necessitate a multi-year, coordinated set of policies and programs. In Mongolia, cookstove research is being carried out by the Desert Research Institute Reno, and the National University of Mongolia.
Biomass stoves in Nepal
In Nepal, 69% of the country’s population relies on solid biomass (wood, cattle manure, agricultural waste) and 75% of people rely on TCS for cooking and heating (Paudel et al., 2021). In rural areas, high amounts of indoor smoke have been reported in kitchens utilizing (TCS). These emissions are responsible for >18,000 deaths in Nepal (Davidson et al., 1986; Reid et al., 1986; Rupakheti et al., 2019). The ICS are more energy-efficient than previous kitchen ranges and do not pollute the air within the home. In Nepal, efforts to improve the efficiency of stoves are not new. Early initiatives, from the 1950s–1980s, and new initiatives, which began in the 1990s with new stove models that could be built with inexpensive, readily available materials and appropriate methodology from the top-down, were scattered until 1998. The ICS program on a national basis has been started in mid-1999 with the help of the Energy Sector Assistance Program. The point was to set up a reasonable structure and system for making ICS technologically accessible and socially suitable for rural people in the light of the limits of the local societies, and income production (Robinson et al., 2021). The rural energy policy of 2006 attempted to address the ICS to implement economic activities that would help overcome the aforementioned issues and to alleviate poverty. Similarly, the 3-year provisional plan has established a goal of installing 3,00,000 ICS throughout the country’s mid-hill and high-hilly regions, to reduce deforestation and the negative impact on the environment (Bhattarai, 2009).
The mud stove is the most well-known ICS, as it is simple, inexpensive, and can be built with locally available materials. In Nepal, several mud ICS designs have been created (Nienhuys, 2005). The Nepal Alliance for Clean Cookstoves is a collaboration of groups working on renewable clean cooking technology, such as improved cookstoves. Alternative Energy Promotion Center, Agricultural Engineering Division-Nepal Agricultural Research Council, Rural Energy Development Program, Biogas Support Program/Nepal (BSP/N) BSP Nepal, Center for Rural Technology/Nepal, Center for Energy Studies, and the National Academy of Science and Technology are the organizations involved in biomass cookstoves-related activities.
Biomass stoves in Pakistan
In Pakistan, about 63% of the population live in rural areas and cook using TCS. About 72% of the population relies on solid biomass for cooking and heating in the country (Irfan et al., 2020). Solid biomass comprises 63% wood, 21% animal dung, and 16% crop residue and has been used by most of nearly 20 million rural households in Pakistan for cooking and heating through inefficient stoves resulting in 115,000 premature deaths (Mahmood, 2007; Saghir et al., 2019; Tareen et al., 2020). Some NGOs working in different regions of Pakistan have begun interventions to disseminate improved cookstoves in their project areas in order to decrease the social, economic, environmental, and health concerns connected with the use of TCS. A Pak–Swiss collaboration called Kalam Integrated Development Project (KIDP) started one such program in Swat in the early 1990s. However, the operations of KIDP terminated in Swat in 1998 due to a variety of socio-political, financial, and institutional factors. Nonetheless, a few local manufacturers continue to make upgraded cookstoves known as project stoves in the area. In this regard, scientific approaches have been used to improve the performance of cookstoves in the last several decades, and various varieties of ICS have been introduced and disseminated in rural parts of Pakistan (Qaseem et al., 2005). Harijan and Uqaili reported that the ICS has fuel (biomass) saving of 14.5 million tonnes. The ICS has efficiencies of 20–40% and can save roughly 40–50% of the fuel used by the TCS. The ICS has several advantages, including the conservation of biomass fuel, the reduction/removal of indoor air pollutants from kitchens, the reduction of deforestation and environmental degradation, the reduction of the drudgery of tasks performed by women and girls, and the reduction of health risks associated with IAP exposure (Harijan and Uqaili, 2013).
The institutes such as the Pakistan Council of Renewable Energy Technology, and the Alternative Energy Development Board are involved in cookstove research in Pakistan. A few NGOs working in different parts of Pakistan have been forced to intercessions to disseminate improved cookstoves in their target areas (January 2012). The Aga Khan Development Network in Pakistan aims to improve human satisfaction in all provinces of Pakistan. Regardless of the Building and Construction Improvement Initiative program, its cost away at water supply, sanitation, minimal effort lodging, and disaster hazard decreasing US$1 = PKR 165.53 (Pakistan rupees, in October 2020). Typically installed prices for products which are involving efficient stove PKR 4,303 (US$26), efficient stove + water-warming facility PKR 8,275 (US$50), roof hatch window PKR 7,778 (US$47), floor insulation PKR 215 (US$1.3) per square meter, wall insulation is PKR 4940 (US$7.6) per square meter (Nienhuys, 2000).
Biomass stoves in Sri Lanka
About 78% of Sri Lankan households utilize biomass fuel for cooking, and the country’s strong reliance on wood-burning stoves is the largest source of (IAP) (Elledge et al., 2012). About 84% of these households are in rural areas of the country. Even in urban areas, over 34% of the population uses wood as their main fuel source. However, while more than 80% of Sri Lankan households have electricity, it is used primarily for lighting, and wood is used for cooking (Elledge et al., 2012). According to a recent analysis by the Energy Conservation Fund, there are three types of stoves are used in the rural areas of the country, these stove types include TSF, partial-confined, and ICS having the contribution of 47%, 32%, and 21% respectively. Similarly in rural areas, the contribution of three types of stoves is about 56%, 31%, and 13%, respectively (Perera and Sugathapala, 2002).
Since 1972, various institutes in Sri Lanka have contributed to the design, promotion, and commercialization of improved cookstoves. The Sri Lanka Standard Institute distributed two-pot biomass clay cookstoves, which are for improved cook stoves resulting in a reduction of smoke (Sirikumara, 2018). The National Engineering Research & Development Centre of Sri Lanka introduced two types of more efficient wood gasifier stoves: one is a forced draft stove with a small electrically operated blower connected to it, and the other is a natural draft stove with a combustion efficiency of around 35%, compared to the TCS (Joseph, 2011; Musafer, 2013). The institutes like the Sri Lanka Sustainable Energy Authority and the National Institutes of Fundamental Studies are involved in cookstove research in Sri Lanka.
Biomass stoves in Laos
In Laos, about 91% of people continue to cook and heat using solid biomass and TCS, with fuelwood at 67% and charcoal at 24% being the most common sources of fuel. On average, a family in Laos consumes 5 kg of fuelwood each day for cooking, which amounts to almost 2 million tonnes per year. Families that use charcoal consume approximately 1.86 kg per day in rural regions and 2.33 kg per day in urban areas; however, it takes up to 6–10 kg of wood to make 1 kg of charcoal, making it a much larger contributor to emissions and health risks (World Bank, 2013). The use of sophisticated cooking energy sources such as liquefied petroleum gas and electricity is quite limited in Laos, therefore dependent on solid biomass (Dave and Balasundaram, 2016). Since 2012, the Lao Institute for Renewable Energy is working to promote more efficient means of biomass use through skilled design programs (efficient cookstoves), policy and marketing research, and development of solid renewable fuel. The Group for the Environment, Renewable Energy, and Solidarity (GERES) developed a new cookstove, the New Lao Stove (NLS), that uses 22% less wood and charcoal than the TCS. In the country, there are 36 NLS production centers that produce 30,000 stoves per month for local markets. The GERES′ support program, as well as its collaboration with producers and distributors, enable capacity building, monitoring, and quality control of the products. By December 2011, sales of the New Lao Stove had surpassed expectations, with 1,607,283 units sold (Dave and Balasundaram, 2016; Baltruschat, 2019). The institutes involved in cookstoves are the research institute of Laos, the Lao Institute for Renewable Energy, and the National Institute of Public Health.
Stove efficiency and emissions comparison between Asian Countries
Stove efficiency is measuring the heat transfer to the cooking pot as a fraction of the calorific value of input biomass. The TCS have low thermal efficiency of less than 10%, and emissions are different according to the operation of the stoves. The principle of analyzing emissions from the combustion process is used to compute emission factors. By multiplying the amount of a specific biomass fuel utilized in the energy system by the comparable emission factors, the total amount of different pollutants emitted by the system may be computed. Different aspects of the combustion process, such as the kind and design of the stove, the type of fuel used, and the operating conditions, all have a significant impact on the emission factors (Arora et al., 2014). According to a different analysis of the research, China is performing better from viewpoint of the ICS than other Asian countries. Many organizations and institutes are working on improving and testing stoves. In China, producers must obtain a certificate from a recognized stove testing facility to certify their new stove design. The stove testing labs examine new stove designs according to their standards; if the stove passes the lab testing requirements, the institute issues a certificate, and the manufacturers then enable the stove to be sold on the market. If a stove fails to pass the lab testing criteria, the manufacturer will not sell it. Many ministries and organizations in India are working on the ICS, and they are doing a better job than those in other Asian countries. In Pakistan, the thermal efficiency of the ICS is about 20–40% and particulate matter (PM) levels in the kitchen ranged from 4,000 to 8,555 g/m3 but utilizing the ICS with improved solid fuel contributed a range of 200 g/m3 to 5,000 g/m3 (Fatmi et al., 2010; Harijan and Uqaili, 2013). Table 4 shows the types of fuel and stoves used with their thermal efficiencies and emission rate for selected Asian countries.
Barriers to dissemination of improved biomass cookstoves
The ICSs are broadly advertised as a technology that improves the environment and health, however, there remains a huge space among their presumed profit and vague effect of most contributions largely fail to achieve their stated aims. Furthermore, the lack of adequate awareness of customer needs such as convenient operation, purchasing capability, unpredictable earnings in rural areas, and the limited facility development of marketplaces and stove designers generate the distribution agreement (Pampallona and Bollini, 2014). Most of the ICS projects in developing countries appear to be heavily focused on excellent designs from a production and design perspective, as well as improving industrial manufacturing procedures (Brown et al., 2017). Initially, the stoves were distributed, and their contribution was hardly measured after the operators in the model characteristic. As a result, they were either not used by customers or were unfit for genuine cooking (Lindgren, 2020). According to the research of several projects, the most common mutual complaint among stove operators is “stove size too small and not suited for all vessels."
In a typical case of fuel gathering, numerous Indian families associated with the LPG primarily employ wood-based cookstoves, mainly for bread baking, the alleged good flavor of the meal, and, to some extent, economic concerns. LPG is only used carefully for quick cooking, such as making tea (Palit and Bhattacharyya, 2014). Furthermore, wood savings appear to have low application in rural areas, owing to their easy supply from farmsteads, agricultural lands, or forests. The additional key barrier is the lack of statistics and information about cookstove acceptability. If progress is to be stopped in changing trends, a significant study is required to support evidence-based action/policy. To overcome these barriers, the ICS distribution could be attractive, and the market potential for clean cooking fuels and skills will not be understated. However, the marketplaces should be separated according to revenue, as there are numerous misstatements in both traditional and modern fuels (Vahlne and Ahlgren, 2014).
Conclusion
The present review was conducted to evaluate the current scenario of biomass cooking and heating stoves in Asian countries including Bangladesh, China, India, Mongolia, Nepal, Pakistan, Sri Lanka, and Laos. The major portion of the population in Asian countries is dependent on biomass for cooking and heating purposes. The TCS utilized by these countries has several limitations which involve emissions of CO2, PM2.5, low thermal efficiency, and greater fuel consumption. Due to these emissions, the IAP causes several health risks. After going through various research conducted on ICS in Asia, we found that the ICS has better thermal efficiency and emissions performance, and thereby fewer health risks. China’s status regarding ICS was found better as compared to other Asian countries. However, we found different barriers in the dissemination of the ICS among the Asian countries such as financial, infrastructure, awareness, market, stove size, and socioeconomic. Therefore, these barriers should be the focus of the research community. In addition, the following guidelines have been suggested for future research focus:
•It is necessary to manufacture high-quality, well-designed, standardized, and cost-effective stoves that are easy to sell and service.
•The cookstoves should be constructed considering the needs of consumers as well as their purchasing power.
•Workshops, schemes, and training programs should be used to encourage the adoption of improved cookstoves.
•For the successful promotion of cookstoves, collaboration between research institutes and support groups should be encouraged.
•The government should support the development of new cookstove designs by providing financing services to stove makers and establishing specialized criteria.
•Before the introduction and after installation in the fields, proper procedures should be created for the frequent evaluation of various cookstove types.
•All stove manufacturers should have an authentic stove testing department to confirm its design, quality, heating, and emission performance.
Author contributions
RA: overall conceptualization and drafting. HI: conceptualization. BL: sources, funding, and review. MS: review, drafting. MA: Review. AA proofread. MI drafting. FR: Review, Editing. All authors read and approved the final manuscript.
Conflict of interest
The authors declare that the research was conducted in the absence of any commercial or financial relationships that could be construed as a potential conflict of interest.
The reviewer (JH) declared a shared affiliation with the author(s) (AA) to the handling editor at the time of review.
Publisher’s note
All claims expressed in this article are solely those of the authors and do not necessarily represent those of their affiliated organizations, or those of the publisher, the editors and the reviewers. Any product that may be evaluated in this article, or claim that may be made by its manufacturer, is not guaranteed or endorsed by the publisher.
Abbreviations
CDM, Clean Development Mechanism; CNISP, Chinese National Improved Stoves Programme; CO, carbon monoxide (mg/MJNET).;CPURE, Clean Production and Utilization of Renewable Energy; GERES, Group for the Environment, Renewable Energy, and Solidarity; IAP, indoor air pollution; ICS, improved biomass cookstoves; KIDP, Kalam Integrated Development Project; LPG, liquid petroleum gas; NBCI, National Biomass Cookstove Initiative; NGOs, Non-Governmental Organizations; NPIC, National Programme on Improved Chulhas; PDR, People’s Democratic Republic; PM2.5, particulate matter with aerodynamic diameter ≤2.5 μm (mg/MJNET); TCS, traditional cookstove; TSF, three stone fire.
References
Abbas, A., Zhao, C., Waseem, M., Ahmed khan, K., and Ahmad, R. (2022). Analysis of energy input–output of farms and assessment of greenhouse gas emissions: A case study of cotton growers. Front. Environ. Sci. 9, 725. doi:10.3389/fenvs.2021.826838
Ahmad, M., Yousaf, M., Wang, S., Cai, W., Sang, L., Li, Z., et al. (2022). Development of rapid CO2 utilizing microbial ecosystem onto the novel & porous FPUF@nZVI@TAC@ASP hybrid for green coal desulphurization. Chem. Eng. J. 433, 134361. doi:10.1016/J.CEJ.2021.134361
Ahmad, R., Zhou, Y. G., Hua, L., Zhao, N., Abbas, A., Sultan, M., et al. (2021b). Study on biomass-based gasifier cookstove for domestic application. Fresenius Environ. Bull. 30, 4365–4374.
Ahmad, R., Abbas, A., Jufei, W., Hua, L., Sultan, M., Li, B., et al. (2021a). Experimental and comparative study of Chinese commercial improved coal-fired cooking and space-heating stoves. Environ. Sci. Pollut. Res. 28, 58135–58141. doi:10.1007/s11356-021-14030-1
Ahmad, R., Zhou, Y., Zhao, N., Pemberton-Pigott, C., Annegarn, H. J., Sultan, M., et al. (2019). Impacts of fuel feeding methods on the thermal and emission performance of modern coal burning stoves. Int. J. Agric. Biol. Eng. 12, 160–167. doi:10.25165/j.ijabe.20191203.3880
Alam, S. M. N., Chowdhury, S. J., Begum, A., and Rahman, M. (2006). Effect of improved earthen stoves: Improving health for rural communities in Bangladesh. Energy sustain. Dev. 10, 46–53. doi:10.1016/s0973-0826(08)60543-8
Arku, R. E., Ezzati, M., Baumgartner, J., Fink, G., Zhou, B., Hystad, P., et al. (2018). Elevated blood pressure and household solid fuel use in premenopausal women: Analysis of 12 Demographic and Health Surveys (DHS) from 10 countries. Environ. Res. 160, 499–505. doi:10.1016/j.envres.2017.10.026
Arora, P., Das, P., Jain, S., and Kishore, V. V. N. (2014). A laboratory based comparative study of Indian biomass cookstove testing protocol and Water Boiling Test. Energy sustain. Dev. 21, 81–88. doi:10.1016/j.esd.2014.06.001
Assad, N. A., Balmes, J., Mehta, S., Cheema, U., and Sood, A. (2015). Chronic obstructive pulmonary disease secondary to household air pollution. Semin. Respir. Crit. Care Med. 36, 408–421. doi:10.1055/s-0035-1554846
Bailis, R., Cowan, A., Berrueta, V., and Masera, O. (2009). Arresting the killer in the kitchen: The promises and pitfalls of commercializing improved cookstoves. World Dev. 37, 1694–1705. doi:10.1016/j.worlddev.2009.03.004
Baltruschat, A. (2019). Adoption of high-technology products in emerging markets: The ACE-1 advanced biomass cookstove in rural Cambodia.
Barnes, D. F., Kumar, P., and Openshaw, K. (2012). Cleaner hearths, better homes: New stoves for India and the developing world. New Delhi: Oxford University Press and World Bank.
Beyene, A. D., and Koch, S. F. (2013). Clean fuel-saving technology adoption in urban Ethiopia. Energy Econ. 36, 605–613. doi:10.1016/j.eneco.2012.11.003
Bhattacharya, S. C., Albina, D. O., and Abdul Salam, P. (2002). Emission factors of wood and charcoal-fired cookstoves. Biomass Bioenergy 23, 453–469. doi:10.1016/S0961-9534(02)00072-7
Bhattacharya, S. C., Salam, P. A., and Sharma, M. (2000). Emissions from biomass energy use in some selected Asian countries. Energy 25, 169–188. doi:10.1016/s0360-5442(99)00065-1
Bhattarai, N. (2009). Implementation of improved cook stove program in Nepal. J. Inst. Eng. 7, 116–120. doi:10.3126/jie.v7i1.2069
Blunck, M., Griebenow, C., Rammelt, M., and Zimm, C. (2011). Carbon markets for improved cooking stoves: A giz guide for project operators. 4th revised edEschborn, Germany: SGIZ-HERA—poverty-oriented Basic Energy Services, 1–63.
Bond, T. C., Doherty, S. J., Fahey, D. W., Forster, P. M., Berntsen, T., Deangelo, B. J., et al. (2013). Bounding the role of black carbon in the climate system: A scientific assessment. J. Geophys. Res. Atmos. 118, 5380–5552. doi:10.1002/jgrd.50171
Bonjour, S., Adair-Rohani, H., Wolf, J., Bruce, N. G., Mehta, S., Prüss-Ustün, A., et al. (2013). Solid fuel use for household cooking: Country and regional estimates for 1980-2010. Environ. Health Perspect. 121, 784–790. doi:10.1289/ehp.1205987
Bosque, E. F., Muneta, L. M., Rey, G. R., Suarez, B., Berrueta, V., Beltran, A., et al. (2021). Using design thinking to improve cook stoves development in Mexico. Sustainability 13, 3843. doi:10.3390/SU13073843
Brown, E., Leary, J., Davies, G., Batchelor, S., and Scott, N. (2017). eCook: what behavioural challenges await this potentially transformative concept? Sustain. Energy Technol. Assessments 22, 106–115. doi:10.1016/j.seta.2017.02.021
Bruce, N., Dora, C., Krzyzanowski, M., Adair-Rohani, H., Morawska, L., and Wangchuk, T. (2013). Tackling the health burden from household air pollution (HAP): Development and implementation of new WHO guidelines. Air Qual. Clim. Chang. 47, 32–38.
Bruce, N., Pope, D., Rehfuess, E., Balakrishnan, K., Adair-Rohani, H., and Dora, C. (2015). WHO indoor air quality guidelines on household fuel combustion: Strategy implications of new evidence on interventions and exposure–risk functions. Atmos. Environ. X. 106, 451–457. doi:10.1016/j.atmosenv.2014.08.064
Bryden, K. M., Ashlock, D. A., McCorkle, D. S., and Urban, G. L. (2003). Optimization of heat transfer utilizing graph based evolutionary algorithms. Int. J. Heat. Fluid Flow. 24, 267–277. doi:10.1016/S0142-727X(02)00243-6
Bussmann, P. J. T., Visser, P., and Prasad, K. (1983). Open fires: Experiments and theory. Proc. Indian Acad. Sci. 6, 1–34. doi:10.1007/bf02843288
Carvalho, R. L., Jensen, O. M., and Tarelho, L. A. C. (2016). Mapping the performance of wood-burning stoves by installations worldwide. Energy Build. 127, 658–679. doi:10.1016/j.enbuild.2016.06.010
Chagunda, M. F., Kamunda, C., Mlatho, J., Mikeka, C., and Palamuleni, L. (2017). Performance assessment of an improved cook stove (esperanza) in a typical domestic setting: Implications for energy saving. Energy sustain. Soc. 7, 19–10. doi:10.1186/s13705-017-0124-1
Chan, S., Sasaki, N., and Ninomiya, H. (2015). Carbon emission reductions by substitution of improved cookstoves and cattle mosquito nets in a forest-dependent community. Glob. Ecol. Conserv. 4, 434–444. doi:10.1016/j.gecco.2015.08.007
Clough, L. (2012). The improved cookstove sector in East Africa: Experience from the developing energy enterprise programme (DEEP). London, UK GVEP-Global Village Energy Partnersh. Int 108.
Commeh, M. K., Agyei-Agyemang, A., Tawiah, P. O., and Asaaga, B. A. (2022). CFD analysis of a flat bottom institutional cookstove. Sci. Afr. 16, e01117. doi:10.1016/J.SCIAF.2022.E01117
Coulson, G., and Ferrari, D. (2019). Advances of science and technology. Springer International Publishing. doi:10.1007/978-3-030-15357-1
Das, I., Jagger, P., and Yeatts, K. (2017). Biomass cooking fuels and health outcomes for women in Malawi. Ecohealth 14, 7–19. doi:10.1007/s10393-016-1190-0
Dave, R., and Balasundaram, R. N. (2016). The Lao cookstove experience: Redefining health through cleaner energy solutions. Washington, D.C:The World Bank.
Davidson, C. I., Lin, S. F., Osborn, J. F., Pandey, M. R., Rasmussen, R. A., and Khalil, M. A. K. (1986). Indoor and outdoor air pollution in the Himalayas. Environ. Sci. Technol. 20, 561–567. doi:10.1021/es00148a003
Elledge, M. F., Phillips, M. J., Thornburg, V. E., Everett, K. H., and Nandasena, S. (2012). A profile of biomass stove use in Sri Lanka. Int. J. Environ. Res. Public Health 9, 1097–1110. doi:10.3390/ijerph9041097
Fatmi, Z., Rahman, A., Kazi, A., Kadir, M. M., and Sathiakumar, N. (2010). Situational analysis of household energy and biomass use and associated health burden of indoor air pollution and mitigation efforts in Pakistan. Int. J. Environ. Res. Public Health 7, 2940–2952. doi:10.3390/ijerph7072940
Gill-Wiehl, A., Price, T., and Kammen, D. M. (2021). What’s in a stove? A review of the user preferences in improved stove designs. Energy Res. Soc. Sci. 81, 102281. doi:10.1016/J.ERSS.2021.102281
Goldemberg, J., Martinez-Gomez, J., Sagar, A., and Smith, K. R. (2018). Household air pollution, health, and climate change: Cleaning the air. Environ. Res. Lett. 13, 030201. doi:10.1088/1748-9326/aaa49d
Greenglass, N., and Smith, K. R. (2007). Current improved cookstove ( ICS ) activities in south Asia 1 A web-based survey. Clean Energy Technologies : Sustainable Development and Climate Co-Benefits in India. September 2006 1–9.
Gupta, A., Mulukutla, A. N. V., Gautam, S., TaneKhan, W., Waghmare, S. S., and Labhasetwar, N. K. (2020). Development of a practical evaluation approach of a typical biomass cookstove. Environ. Technol. Innov. 17, 100613. doi:10.1016/j.eti.2020.100613
Haines, A., McMichael, A. J., Smith, K. R., Roberts, I., Woodcock, J., Markandya, A., et al. (2009). Public health benefits of strategies to reduce greenhouse-gas emissions: Overview and implications for policy makers. Lancet 374, 2104–2114. doi:10.1016/S0140-6736(09)61759-1
Hanbar, R. D., and Karve, P. (2002). National programme on improved chulha (NPIC) of the government of India: An overview. Energy sustain. Dev. 6, 49–55. doi:10.1016/S0973-0826(08)60313-0
Harijan, K., and Uqaili, M. A. (2013). Potential of biomass conservation through dissemination of efficient cook stoves in Pakistan. APCBEE Procedia 5, 358–362. doi:10.1016/j.apcbee.2013.05.061
Husain, Z., Tiwari, S. S., Pandit, A. B., and Joshi, J. B. (2019). Computational fluid dynamics study of biomass cook stove - Part 1: Hydrodynamics and homogeneous combustion. Ind. Eng. Chem. Res. 59, 4161–4176. doi:10.1021/acs.iecr.9b03181
International Energy Agency (2006). Energy for cooking in developing countries. World Energy Outlook, 419–445. doi:10.1787/weo-2006-16-en
Irfan, M., Zhao, Z.-Y., Panjwani, M. K., Mangi, F. H., Li, H., Jan, A., et al. (2020). Assessing the energy dynamics of Pakistan: Prospects of biomass energy. Energy Rep. 6, 80–93. doi:10.1016/j.egyr.2019.11.161
Jan, I. (2012). What makes people adopt improved cookstoves? Empirical evidence from rural northwest Pakistan. Renew. Sustain. Energy Rev. 16, 3200–3205. doi:10.1016/j.rser.2012.02.038
Jetter, J. J., and Kariher, P. (2009). Solid-fuel household cook stoves: Characterization of performance and emissions. Biomass Bioenergy 33, 294–305. doi:10.1016/j.biombioe.2008.05.014
Jetter, J., Zhao, Y., Smith, K. R., Khan, B., Yelverton, T., Decarlo, P., et al. (2012). Pollutant emissions and energy efficiency under controlled conditions for household biomass cookstoves and implications for metrics useful in setting international test standards. Environ. Sci. Technol. 46, 10827–10834. doi:10.1021/es301693f
Johnson, N. G., and Bryden, K. M. (2012). Factors affecting fuelwood consumption in household cookstoves in an isolated rural West African village. Energy 46, 310–321. doi:10.1016/j.energy.2012.08.019
Joshi, J. B., Pandit, A. B., Patel, S. B., Singhal, R. S., Bhide, G. K., Mariwala, K. V., et al. (2012). Development of efficient designs of cooking systems. II. Computational fluid dynamics and optimization. Ind. Eng. Chem. Res. 51, 1897–1922. doi:10.1021/IE2025745
Kishore, V. V. N., and Ramana, P. V. (2002). Improved cookstoves in rural India: How improved are they?: A critique of the perceived benefits from the national programme on improved Chulhas (NPIC). Energy 27, 47–63. doi:10.1016/S0360-5442(01)00056-1
Kshirsagar, M. P., and Kalamkar, V. R. (2014). A comprehensive review on biomass cookstoves and a systematic approach for modern cookstove design. Renew. Sustain. Energy Rev. 30, 580–603. doi:10.1016/j.rser.2013.10.039
Kshirsagar, M. P., and Kalamkar, V. R. (2020). Application of multi-response robust parameter design for performance optimization of a hybrid draft biomass cook stove. Renew. Energy 153, 1127–1139. doi:10.1016/J.RENENE.2020.02.049
Kshirsagar, M. P., and Kalamkar, V. R. (2022). Hybrid draft direct-combustion with secondary air jets in cross-flow for reducing CO and PM2.5 emissions in biomass cookstoves. Sustain. Energy Technol. Assessments 51, 101913. doi:10.1016/J.SETA.2021.101913
Kshirsagar, M. P., Kalamkar, V. R., and Pande, R. R. (2020). Multi-response robust design optimization of natural draft biomass cook stove using response surface methodology and desirability function. Biomass Bioenergy 135, 105507. doi:10.1016/J.BIOMBIOE.2020.105507
Kshirsagar, M. P., and Kalamkar, V. R. (2016). User-centric approach for the design and sizing of natural convection biomass cookstoves for lower emissions. Energy 115, 1202–1215. doi:10.1016/J.ENERGY.2016.09.048
Lambe, F., and Atteridge, A. (2012). Putting the cook before the stove: A user-centred approach to understanding household energy decision-making – a case study of Haryana state, northern India. Stock. Environ. Inst.
Legros, G., Havet, I., Bruce, N., Bonjour, S., Rijal, K., Takada, M., et al. (2009). The energy access situation in developing countries: A review focusing on the least developed countries and sub-saharan africa. New York, NY, USA: World Heal. Organ.
Lindgren, S. A. (2020). Clean cooking for all? A critical review of behavior, stakeholder engagement, and adoption for the global diffusion of improved cookstoves. Energy Res. Soc. Sci. 68, 101539. doi:10.1016/j.erss.2020.101539
Lombardi, F., Riva, F., Bonamini, G., Barbieri, J., and Colombo, E. (2017). Laboratory protocols for testing of improved cooking stoves (ICSs): A review of state-of-the-art and further developments. Biomass Bioenergy 98, 321–335. doi:10.1016/J.BIOMBIOE.2017.02.005
MacCarty, C. J. (2010). “The side-feed fan stove,” in ETHOS Conference, Kirkland, Washington, 29–31.
Maccarty, N. A., Hallam, A., and Wang, X. (2013). A zonal model to aid in the design of household biomass cookstoves.
MacCarty, N., Ogle, D., Still, D., Bond, T., and Roden, C. (2008). A laboratory comparison of the global warming impact of five major types of biomass cooking stoves. Energy sustain. Dev. 12, 56–65. doi:10.1016/S0973-0826(08)60429-9
MacCarty, N., Still, D., and Ogle, D. (2010). Fuel use and emissions performance of fifty cooking stoves in the laboratory and related benchmarks of performance. Energy sustain. Dev. 14, 161–171. doi:10.1016/j.esd.2010.06.002
Mahmood, S. (2007). Good governance reform agenda in Pakistan: Current challenges. New York: Nova Publishers.
Mehetre, S. A., Panwar, N. L., Sharma, D., and Kumar, H. (2017). Improved biomass cookstoves for sustainable development: A review. Renew. Sustain. Energy Rev. 73, 672–687. doi:10.1016/j.rser.2017.01.150
Mohan, R., and Kumar, S. (2011). Enhancement of thermal efficiency of traditional Indian cooking furnace (Chulha). Curr. World Environ. 6, 61–66. doi:10.12944/cwe.6.1.07
Mukhopadhyay, R., Sambandam, S., Pillarisetti, A., Jack, D., Mukhopadhyay, K., Balakrishnan, K., et al. (2012). Cooking practices, air quality, and the acceptability of advanced cookstoves in Haryana, India: An exploratory study to inform large-scale interventions. Glob. Health Action 5, 1–13. doi:10.3402/gha.v5i0.19016
Mukunda, H. S., Dasappa, S., Paul, P. J., Rajan, N. K. S., Yagnaraman, M., Ravi Kumar, D., et al. (2010). Gasifier stoves - science, technology and field outreach. Curr. Sci. 98, 627–638.
Najjar, Y. S. H., and Kseibi, M. (2017). Evaluation of experimental JUST thermoelectric stove for electricity – deprived regions. Renew. Sustain. Energy Rev. 69, 854–861. doi:10.1016/J.RSER.2016.07.041
Nega, T., Tesfaye, A., and Paramasivam, P. (2022). Design and CFD modeling of gasifier stove combined with heat exchanger for water heating application. AIP Adv. 12, 045121. doi:10.1063/5.0081001
Ngo, S. I., and Lim, Y. Il (2020). Multiscale eulerian CFD of chemical processes: A review. ChemEngineering 4, 23. doi:10.3390/CHEMENGINEERING4020023
Nienhuys, Sjoerd (2005). Cooking stove improvements: Design for remote high altitude. Kathmandu: Areas Dolpa Region.
Nienhuys, S. (2000). Research report on BACIP wood stoves for high mountain areas. Pakistan: Gilgit.
Ochieng, C. A., Tonne, C., and Vardoulakis, S. (2013). A comparison of fuel use between a low cost, improved wood stove and traditional three-stone stove in rural Kenya. Biomass Bioenergy 58, 258–266. doi:10.1016/j.biombioe.2013.07.017
Pampallona, S., and Bollini, P. (2014). A participative approach: A rural community develops, tests and adopts an improved cooking stove in India. Barriers Cookstoves 15.
Pande, R. R., Kshirsagar, M. P., and Kalamkar, V. R. (2020). Experimental and CFD analysis to study the effect of inlet area ratio in a natural draft biomass cookstove. Environ. Dev. Sustain. 22, 1897–1911. doi:10.1007/s10668-018-0269-x
Paudel, D., Jeuland, M., and Lohani, S. P. (2021). Cooking-energy transition in Nepal: Trend review. Clean. Energy 5, 1–9. doi:10.1093/ce/zkaa022
Perera, K. K. C. K., and Sugathapala, A. G. T. (2002). Fuelwood-fired cookstoves in Sri Lanka and related issues. Energy sustain. Dev. 6, 85–94. doi:10.1016/S0973-0826(08)60302-6
Pratiti, R., Vadala, D., Kalynych, Z., and Sud, P. (2020). Health effects of household air pollution related to biomass cook stoves in resource limited countries and its mitigation by improved cookstoves. Environ. Res. 186, 109574. doi:10.1016/j.envres.2020.109574
Qaseem, N., Uchiyama, T., and Ohara, K. (2005). Dissemination of cooking energy technologies for sustainable household consumption in Pakistan. arfe. 41, 206–211. doi:10.7310/arfe1965.41.206
Qiu, D., Gu, S., Catania, P., and Huang, K. (1996). Diffusion of improved biomass stoves in China. Energy Policy 24, 463–469. doi:10.1016/0301-4215(96)00004-3
Qu, W., Yan, Z., Qu, G., and Ikram, M. (2015). Household solid fuel use and cardiovascular disease in rural areas in shanxi, China. Iran. J. Public Health 44, 625–638.
Rahimi, M. J., Hamedi, M. H., Amidpour, M., and Livani, E. (2020). Technoeconomic evaluation of a gasification plant: Modeling, experiment and software development. Waste Biomass Valorization 11, 6815–6840. doi:10.1007/s12649-019-00925-1
Rahman, M. L. (2015). Improved cooking stoves in south Asia. Islamabad, Pakistan: SAARC Energy Centre.
Raman, P., Ram, N. K., and Gupta, R. (2014). Development, design and performance analysis of a forced draft clean combustion cookstove powered by a thermo electric generator with multi-utility options. Energy 69, 813–825. doi:10.1016/J.ENERGY.2014.03.077
Reed, T. B., Anselmo, E., and Kirchef, K. (2008). Testing & modeling the wood‐gas turbo stove, 693–704. doi:10.1002/9780470694954.ch55
Regional Wood Energy Development (1993). Improved solid biomass burning cookstoves: A development manual.
Reid, H. F., Smith, K. R., and Sherchand, B. (1986). Indoor smoke exposures from traditional and improved cookstoves: Comparisons among rural Nepali women. Mt. Res. Dev. 6, 293–303. doi:10.2307/3673370
Robinson, B. L., Jewitt, S., Clifford, M. J., and Hewitt, J. (2021). Understanding the current market enablers for Nepal’s biomass cookstove industry. Dev. Pract. 32, 52–68. doi:10.1080/09614524.2021.1893659
Rofiqul Islam, M., Rabiul Islam, M., and Rafiqul Alam Beg, M. (2008). Renewable energy resources and technologies practice in Bangladesh. Renew. Sustain. Energy Rev. 12, 299–343. doi:10.1016/j.rser.2006.07.003
Ruiz-Mercado, I., Masera, O., Zamora, H., and Smith, K. R. (2011). Adoption and sustained use of improved cookstoves. Energy Policy 39, 7557–7566. doi:10.1016/j.enpol.2011.03.028
Rupakheti, D., Kim Oanh, N. T., Rupakheti, M., Sharma, R. K., Panday, A. K., Puppala, S. P., et al. (2019). Indoor levels of black carbon and particulate matters in relation to cooking activities using different cook stove-fuels in rural Nepal. Energy sustain. Dev. 48, 25–33. doi:10.1016/j.esd.2018.10.007
Saghir, M., Zafar, S., Tahir, A., Ouadi, M., Siddique, B., and Hornung, A. (2019). Unlocking the potential of biomass energy in Pakistan. Front. Energy Res. 7, 1–18. doi:10.3389/fenrg.2019.00024
Samuel, B. (1987). Biomass stoves: Engineering design development and dissemination. Volunt. Tech. Assist.
Santín, C., Doerr, S. H., Preston, C. M., and González‐Rodríguez, G. (2015). Pyrogenic organic matter production from wildfires: A missing sink in the global carbon cycle. Glob. Chang. Biol. 21, 1621–1633. doi:10.1111/gcb.12800
Sedighi, M., and Salarian, H. (2017). A comprehensive review of technical aspects of biomass cookstoves. Renew. Sustain. Energy Rev. 70, 656–665. doi:10.1016/J.RSER.2016.11.175
Shastri, C. M., Sangeetha, G., and Ravindranath, N. H. (2002). Dissemination of efficient ASTRA stove: Case study of a successful entrepreneur in sirsi, India. Energy sustain. Dev. 6, 63–67. doi:10.1016/S0973-0826(08)60316-6
Shen, G., Chen, Y., Xue, C., Lin, N., Huang, Y., Shen, H., et al. (2015). Pollutant emissions from improved coal-and wood-fuelled cookstoves in rural households. Environ. Sci. Technol. 49, 6590–6598. doi:10.1021/es506343z
Sinha, B. (2002). The Indian stove programme: An insider’s view—the role of society, politics, economics and education. Boil. Point, 23–26.
Sirikumara, K. J. (2018). Requirements for the development of a standard and certification system for sustainable fuel wood trade in Sri Lanka.
Smith, K. R. (1994). Health, energy, and greenhouse-gas impacts of biomass combustion in household stoves. Energy sustain. Dev. 1, 23–29. doi:10.1016/S0973-0826(08)60067-8
Smith, K. R., Shuhua, G., Kun, H., and Daxiong, Q. (1993). One hundred million improved cookstoves in China: How was it done? World Dev. 21, 941–961. doi:10.1016/0305-750X(93)90053-C
Smith, K. R., and Vellekoop, A. (1989). An investigation of the electron transfer properties of modified organocofper reagents and higher-order cuprates. Tetrahedron 24, 517–522. doi:10.1016/0040-4020(89)80079-1
Smith, R., and Francisco, S. (2005). Programmes promoting improved household stoves in China, 50–52.
Sowgath, M. T., Rahman, M. M., Nomany, S. A., Sakib, M. N., and Junayed, M. (2015). CFD study of biomass cooking stove using autodesk simulation CFD to improve energy efficiency and emission characteristics. Chem. Eng. Trans. 45. doi:10.3303/CET1545210
Steenland, K., Pillarisetti, A., Kirby, M., Peel, J., Clark, M., Checkley, W., et al. (2018). Modeling the potential health benefits of lower household air pollution after a hypothetical liquified petroleum gas (LPG) cookstove intervention. Environ. Int. 111, 71–79. doi:10.1016/j.envint.2017.11.018
Sundberg, C., Karltun, E., Gitau, J. K., Kätterer, T., Kimutai, G. M., Mahmoud, Y., et al. (2020). Biochar from cookstoves reduces greenhouse gas emissions from smallholder farms in Africa. Mitig. Adapt. Strateg. Glob. Chang. 25, 953–967. doi:10.1007/s11027-020-09920-7
Sweeney, D. (2017). Handbook for biomass cookstove research, design and development, A practical guide to implementing recent advances. Global Alliance for Clean Cookstove and the MIT D-Lab.
Tareen, W. U. K., Dilbar, M. T., Farhan, M., Nawaz, M. A., Durrani, A. W., Memon, K. A., et al. (2020). Present status and potential of biomass energy in Pakistan based on existing and future renewable resources. Sustainability 12, 249. doi:10.3390/su12010249
Teshome, F., Messele, E., and Kolhe, K. P. (2020). Development and testing of improved double skirt rocket stove for reducing the emission level of carbon monoxide. Lect. Notes Inst. Comput. Sci. Soc. Telecommun. Eng. LNICST 308, 537–547. doi:10.1007/978-3-030-43690-2_39
Tryner, J., Willson, B. D., and Marchese, A. J. (2014). The effects of fuel type and stove design on emissions and efficiency of natural-draft semi-gasifier biomass cookstoves. Energy sustain. Dev. 23, 99–109. doi:10.1016/j.esd.2014.07.009
Uddin, M., Sifat, A. I., Begum, B. A., and Shams, S. M. N. (2020). Thermal performance and emission analysis of available metallic and non-metallic improved cook stoves in Bangladesh.
UNEP (2009). Converting waste agricultural biomass into a resource. Osaka/Shiga, Japan: Compendium of Technologies.
Urmee, T., and Gyamfi, S. (2014). A review of improved Cookstove technologies and programs. Renew. Sustain. Energy Rev. 33, 625–635. doi:10.1016/j.rser.2014.02.019
U.S. Department of Energy (2011). Biomass cookstoves technical meeting: Summary report. Alexandria, VA.
Vahlne, N., and Ahlgren, E. O. (2014). Policy implications for improved cook stove programs—A case study of the importance of village fuel use variations. Energy Policy 66, 484–495. doi:10.1016/j.enpol.2013.11.042
Van Der Kroon, B., Brouwer, R., and Van Beukering, P. J. H. (2013). The energy ladder: Theoretical myth or empirical truth? Results from a meta-analysis. Renew. Sustain. Energy Rev. 20, 504–513. doi:10.1016/J.RSER.2012.11.045
Venkataraman, C., Sagar, A. D., Habib, G., Lam, N., and Smith, K. R. (2010). The Indian national initiative for advanced biomass cookstoves: The benefits of clean combustion. Energy sustain. Dev. 14, 63–72. doi:10.1016/j.esd.2010.04.005
Vicente, E. D., and Alves, C. A. (2018). An overview of particulate emissions from residential biomass combustion. Atmos. Res. 199, 159–185. doi:10.1016/J.ATMOSRES.2017.08.027
Westhoff, B., and Germann, D. (1995). Stove images: A documentation of improved and traditional stoves in africa, Asia and Latin America. Frankfurt: Brandes and Apsel.
World Bank (2020). Accelerating access to clean cooking: The efficient, clean cooking and heating program and the clean cooking Fund.
World Bank (2014). Mongolia national low eemission stove strategy report : Completing the transition to a sustainable market for cleaner stoves in Mongolia.
World Bank (2013). Pathways to cleaner household cooking in Lao PDR : An intervention strategy. Washington DC.
Yip, F., Christensen, B., Sircar, K., Naeher, L., Bruce, N., Pennise, D., et al. (2017). Assessment of traditional and improved stove use on household air pollution and personal exposures in rural Western Kenya. Environ. Int. 99, 185–191. doi:10.1016/j.envint.2016.11.015
Zhang, X., Chen, B., and Fan, X. (2015). Different fuel types and heating approaches impact on the indoor air quality of rural houses in Northern China. Procedia Eng. 121, 493–500. doi:10.1016/j.proeng.2015.08.1097
Keywords: biomass cookstoves, improved biomass cookstoves, health risks, thermal efficiency, emissions performance, asia
Citation: Ahmad R, Ilyas HN, Li B, Sultan M, Amjad M, Aleem M, Abbas A, Imran MA and Riaz F (2022) Current challenges and future prospect of biomass cooking and heating stoves in Asian Countries. Front. Energy Res. 10:880064. doi: 10.3389/fenrg.2022.880064
Received: 20 February 2022; Accepted: 12 August 2022;
Published: 28 September 2022.
Edited by:
Sachin Kumar, Sardar Swaran Singh National Institute of Renewable Energy, IndiaReviewed by:
Milind Kshirsagar, St. Vincent Pallotti College of Engineering and Technology Nagpur, IndiaJianlin Hu, Nanjing University of Information Science and Technology, China
Copyright © 2022 Ahmad, Ilyas, Li, Sultan, Amjad, Aleem, Abbas, Imran and Riaz. This is an open-access article distributed under the terms of the Creative Commons Attribution License (CC BY). The use, distribution or reproduction in other forums is permitted, provided the original author(s) and the copyright owner(s) are credited and that the original publication in this journal is cited, in accordance with accepted academic practice. No use, distribution or reproduction is permitted which does not comply with these terms.
*Correspondence: Riaz Ahmad, cmlhekBjYXUuZWR1LmNu; Bin Li, bGliaW4xOTg1MjBAMTI2LmNvbQ==; Fahid Riaz, ZmFoaWQucmlhekBhZHUuYWMuYWU=