- 1Department of Engineering and Technology Management, University of Pretoria, Pretoria, South Africa
- 2DST/NRF/Newton Fund Trilateral Research Chair in Transformative Innovation, The Fourth Industrial Revolution and Sustainable Development, University of Johannesburg, Johannesburg, South Africa
Solar lighting has become the primary lighting source for households within rural Malawi, where many households remain off-grid and are unable to afford the purchase of large, independent power systems. However, this success has not been without its challenges. The paradox is that, historically, even the lowest cost systems require an initial investment beyond the means of low-income households, and hence necessitate the use of expensive and exploitative financing options, such as those offered by micro-financial institutions. In this study, we explore in a case-study, how one solar company, Yellow, has overcome this structural inequity by combining three low-cost technologies, namely pay-as-you-go, mobile money (MoMo), and cloud-based services (XaaS), to develop a novel platform, referred to as Ofeefee, which is able to deliver products into a market characterized by a weak retail infrastructure and low purchasing power. The result was better quality lighting at a lower levelized cost than traditional technologies. In so doing, the paper highlights the importance of thinking not just in broad energy access terms but the importance of discriminating between energy and lighting to disaggregate the needs of energy poor communities more appropriately.
1 Introduction
Based on the International Energy Agency’s (IEA) definition for essential energy access, much of Malawi is below the minimum level (Eales, Alsop, Frame, Strachan and Galloway, 2020). The IEA defines essential energy access as a household having reliable and affordable access to clean cooking facilities and sufficient electricity to supply four lightbulbs operating 4 h per day, a fan 3 h per day, and a television 2 h per day, equating to 500 kW-hours (kWh) per household-per year with standard appliances (International Energy Agency, 2020). This level of access equates broadly to somewhere between Tier 3 and Tier 4 of the Multi-Tier Framework (MTF), the latter developed by the World Bank as a means of measuring energy access (Bhatia, Angelou and Portale, 2015).
The cost of ensuring that households in the remote areas of a country such as Malawi can reach Tier 1 is estimated at about $20 billion (World Bank, 2017), and the attainment of Tier 5 access at about $30 billion per year over a 12-year period (2018–2030) (World Bank, 2017), making the likelihood of achieving Sustainable Development Goal 7 (SDG7) of universal access to clean and affordable energy for many sub-Saharan African (SSA) countries rather unlikely. The goal of universal access is even less achievable if the recent recommendations on a Modern Energy Minimum of 1,000 kWh per person-per year are to be adopted, a value which correlates to an average per capita income of $2,500 per person per year or roughly equivalent of a lower–middle-income country (Energy For Growth Hub, 2021).
Such conditions certainly do not exist in Malawi and the prospects for rural Malawians attaining Tier 5 levels of energy access, based on grid-tied electricity, are grim (Eales et al., 2020). Annual household budgets for lighting are $30–$60 (Adkins, Eapen, Kaluwile, Nair and Modi, 2010; National Statistic Office, 2020) and the country lacks the financial capital to support the construction of a wider distribution or generation infrastructure. Even Tier 1 energy access has been challenging, and much of the rural population remains in energy poverty (Eales and Unyolo, 2018; Smith et al., 2019; Eales et al., 2020).
In this article, we consider how Tier 1 energy access, and particularly access to affordable domestic lighting, has been transformed as a result of several simultaneous changes to both the products and the way in which they are distributed. We have followed a case-study-approach, based on a single company (Yellow), in order to understand how its innovative approach has been possible through the novel use of three novel technology platforms, which led to an increased uptake of pico solar. The research began with an initial proposition that this approach may overcome some of the criticisms of the solar home system (SHS) market and particularly the claim that it may reinforce rather than alleviate energy poverty (Samarakoon, 2020). Two research questions were formulated, covering firstly, the question of the affordability of the pico solar systems, based on the levelised cost of lighting (LCOL), relative to traditional forms of lighting, and secondly, the implications of integrating the three novel technology platforms for the issues of energy justice and access.
To answer these questions, we first provide (in Section 2) the relevant background for the study, including the rise of solar lighting, the development of SHS within the context of energy justice, and the emergence of mobile money (MoMo). This section is followed by an overview of our methodology used in the study (Section 3), then a presentation of its results (Section 4), and a discussion of the implications thereof (Section 5). In Section 6, we conclude with a number of key insights and policy recommendations.
2 Background
2.1 The rise of solar lighting solutions
Despite a policy focus by African governments on grid-based energy supply and access, many households remain off-grid and there is an obvious need for alternative energy solutions that are affordable, clean, and decentralised (Eales et al., 2020). Energy consumers are presented with multiple options, depending on the size of the demand, the lowest-cost energy resource and, in broad terms, the social and cultural contexts. Within SSA countries, the use of SHS has become a major segment of the total energy market. It is estimated that about 22 million units are sold per year of varying sizes between 5 and 50 peak watts (Wp) (Nygaard, Hansen and Larsen, 2016; GOGLA, 2018; Lighting Global, 2020; GOGLA, 2021b). The total annual market value (2017) is estimated at about $660 million, based on an average price per unit of $30. The cumulative sales have now reached $3.9 billion since 2010 and more than 180 million devices have already been installed (Lighting Global, 2020).
SHS are decentralised power systems that provide power and lighting solutions for residential premises using solar photovoltaic panels. They can be designed to meet the energy needs from Tier 1 to Tier 4 of the MTF (Narayan eat al, 2019). At Tier 1, the systems consist of a small solar panel powering a tiny battery that supplies several low-wattage lights, typically light-emitting diodes (LEDs) for a few hours a night and/or recharges a phone. Such systems are known as “pico solar” (Nygaard et al., 2016), and are the main focus of this article.
There are multiple components to the pico solar value chain, from the manufacturers of the panels and batteries to the customer and agents for the assembled systems. Some of the companies, such as d. light and Greenlight Planet (Sun King), are vertically integrated across the whole value chain, including all aspects from manufacturing of the panels, assembly of the SHS devices, and distribution and sales, whereas others, such as Yellow, are focused specifically on retail and the provision of financial services (collection of levies). Major manufacturers of SHS include BioLite and Amped Innovation. Recognizing the importance of quality control and standards within the sector, many of the companies belong to GOGLA, which describes itself as a global association representing more than 180 companies in the off-grid solar energy industry assisting to deliver “quality, affordable off-grid electricity products and services to as many customers as possible across the developing world” (GOGLA, 2021a).
Prospects for SHS relative to other systems for off-grid electricity supply will depend on its development as a technological innovation system relative to alternative systems. There are several factors in favor of its short-term advantages including the increasing efficiency of LEDs, falling prices for photovoltaic (PV) panels, and improvements in battery cost vs. performance. LED efficiencies have increased from 80 lumens per watt in 2010 to 150 lumens per watt in 2018 (Lighting Global, 2018); prices for battery packs have fallen from $1,100 per kWh in 2010 to $126 per kWh in 2020 (BloombergNEF, 2020); and the costs for PV panels have dropped by 90% since 2009 (IRENA, 2020). However, the growing popularity of SHS will only be sustained if it can compete with alternative solutions to off-grid energy demands such as micro-grids based on diverse energy sources or regional energy companies which are able to establish a viable distribution infrastructure. These are particularly effective and efficient for communities that are isolated from the national grid connection but are dense enough to allow multiple connections. SHS are still seen as the best solution for disbursed homes and those with very minimal power needs (USAID, 2018).
2.2 Solar home systems and energy justice
SHS as an energy solution has been cast in two very different ways within the literature. At the core of the debate is the tension between distributive justice (energy is a universal human right and the state is obligated to provide all its citizens with sufficient energy resources of high quality) and market-based energy access (the supply of clean, reliable, and affordable energy services at market price). It would be naïve to suggest that the two approaches to the supply energy markets could be met simultaneously. Prices and markets cannot do the work of morals and rights despite the neo-classical claim that markets are instruments of distributive justice.
The concept of energy justice has been conceptualized as a framework for the development of a global energy system which fairly disseminates the costs and benefits, is representative in its decision-making and recognizes the perspectives of diverse actors (Sovacool and Dworkin, 2015; Jenkins, McCauley, Heffron, Stephan and Rehner, 2016). The importance of a human-centered approach to energy challenges, and particularly how energy markets may emerge within the principles of the framework, is completely endorsed in this analysis. Evidence in support of energy justice is pervasive in the literature and arguments in favor of the just transition, for instance, are grounded in the fundamental principles on which our societies should be based (Heffron, McCauley and Sovacool, 2015; Sovacool, Burke, Baker, Kotikalapudi and Wlokas, 2017).
The ability of SHS to be able to meet the goals of energy justice has already been questioned (Monyei, Adewumi and Jenkins, 2018; Samarakoon, 2020). Previous studies indicate that SHS may reinforce rather than alleviate energy poverty, and these studies have argued for the immediate incorporation of the energy justice framework into the national energy policy (Monyei et al., 2018), especially to take account of the needs of the ‘energy‐poor’ (Samarakoon, 2020).
Some of these claims are contested by companies and energy associations (GOGLA, 2018). Rural markets in the Global South, where it is countered, are difficult to access and are subject to widespread failure of network-based energy supply (GOGLA, 2018). Moreover, financial resources (in the form of disposable income) and cash (in the form of currency) are limited, communication is unreliable, and infrastructure is weak. Within this context, the delivery of energy services is challenging. The solution needs to be affordable; it needs to operate using the telecommunication infrastructure and the connection technologies, based mostly on cell phones linked to data services, and MoMo; it needs to be supported by local expertise; and it needs to have long term reliability. The important question as to whether the integrated platform, as described in this study, aligns more closely with the goals of energy justice are further discussed in Section 5.
Interestingly, MoMo itself emerged as an example of an inclusive innovation designed to address the failure of commercial banks to respond to the needs of poor households, initially in Kenya, but later in other countries (Onsongo, 2019). Considering the centrality of MoMo to the main argument of this article, namely because greater inclusiveness in the energy market was only possible as a consequence of the simultaneous development of three platforms, of which MoMo is one. In the next section we give a brief overview of the emergence and scope of MoMo.
2.3 Mobile money and financial inclusion
MoMo is a platform which allows users to access banking services without having to open, pay for, and manage a bank account in a commercial bank (Kabengele and Hahn, 2021). Apart from being a least-cost option for poor households, it also is reported to empower women (Suri and Jack, 2016), reduce customers’ vulnerability to crime and theft (Economides and Jeziorski, 2017), and create greater resilience within poor communities to income insecurity (Kabengele and Hahn, 2021).
M-Pesa, one of the earliest examples of MoMo, was developed in Kenya due to an ongoing exclusion of poor households from formal banking services, pressure from the government to address financial inclusion, and the increasing competition between mobile telecommunications operators, the latter leading to the development of value-added services as a means of retaining or even expanding their existing customer base (Onsongo, 2019). Commercial banks were poorly positioned and unwilling to service low-income customers, characterized by a high volume of micro-finance transactions, higher credit risk, and limited infrastructure within rural, and predominantly poor, areas.
According to Onsongo (2019), in 2005, only 19% of Kenya’s 35 million people had a formal bank account. In a series of steps which began with a project initiated by Vodafone and supported by the United Kingdom’s Department for International Development to understand the problem of micro-finance, followed by the establishment of a partnership between Vodafone, Saraficom, Faula Kenya, and the Commercial Bank of Africa, and then a short pilot test, dubbed M-Pesa, the service was launched nationally in 2007. Within 3 years, the number of registered customers had reached 10.3 million, and by 2014, M-Pesa had 26.2 million customers or 71% of the adult population (Onsongo, 2019).
The extraordinary success of M-Pesa was only partly replicated in other countries. In a detailed quantitative study of the factors leading to the widespread adoption of MoMo within other countries, Kabengele and Hahn (2021) identified four different pathways dependant on the local context. Important success factors for service providers include consumer trust, financial resources, technological capabilities, and international support. On the customer side, it was concluded that MoMo’s success was more likely in contexts with sufficient consumer knowledge of mobile telephony and the local infrastructure, coupled with an under-developed financial service sector (Kabengele and Hahn, 2021).
In summary, the emergence of MoMo may be ascribed to a complex blend of local conditions, of which the social exclusion of poor and remote communities from traditional banking services is fundamental. Its adoption has brought many of the benefits of the banking services to these communities, such as reduced reliance on cash as a means of transaction, multiple service points, reduced vulnerability to theft, and greater agency in respect of income, without the cost of commercial services. Similarly, solar home systems, as argued in this article, were developed in response to the need for inclusion in the energy market of poor households. The remainder of this article brings together the three areas of energy access, energy justice, and SHS by asking two important questions; firstly, how affordable is pico solar relative to other lighting solutions, and secondly, what are the implications of the development of a novel platform for the pico solar market in Malawi?
We ask the first question because we find that there has been little economic analysis of pico solar from a lighting perspective. We note that the most frequent analysis conducted to understand the cost efficiency of SHS has focused on the levelized cost of energy (LCOE) as opposed to the levelised cost of lighting (LCOL). However, in a country like Malawi, the ability to purchase any form of clean energy is difficult. Over 50% of the population is below the national poverty line and over 80% of the population lives in rural areas (World Bank, 2017). This makes accessing electricity extremely difficult as only 4% of the rural areas are connected to the national grid (SEA4ALL, 2021). Given the high levels of poverty in Malawi and despite research showing the market potential for larger community micro-grids and the inefficiency of pico solar (Eales et al., 2020), it is likely that the latter will remain an essential energy source for many in rural areas for some time. As such, we ask the first question by interrogating affordability in comparison to traditional forms of lighting by analyzing LCOL.
We also note that there are new entrants, such as Yellow (used as a case-study in this article), which are entering the Malawian solar power space and specifically targeting the pico solar market as opposed to larger systems. It is for this reason that we ask the second question. Given that the government’s 2017 Integrated Resources Plan only envisages around 30% of the population being grid-connected by 2030, there is a significant focus on off-grid solutions although the market is recognized to be underdeveloped despite significant inputs from international development partners (USAID, 2018). Novel approaches to overcoming a key structural impediment, namely the affordability of financing, are therefore pertinent in any discussion about the future of off-grid technologies. As such, we answer the second question through two sub-questions: how are the technology platforms assembled and integrated, and how significant have the various components become in the overall transition?
3 Methodology
This study has been undertaken as a case study with simultaneous qualitative and quantitative arms (in the form of a mixed-methods approach). Each arm is now separately described.
3.1 Qualitative study
In the qualitative arm, a study was undertaken on a single company (Yellow), which is active in the pico solar market within Malawi (see https://www.yellow.africa/). The company was chosen on the basis that it is pioneering a novel business model by combining three technology platforms, and, as a result, there are important learning points on market formation in the renewable energy sector that can be extracted from the case.
The company was also chosen due to the ability to access it and interview staff within the organization. This opportunity was possible because one of the authors has a family member who helped set up the company. As such, the research involved several interviews with the Chief Technology Officer of Yellow and interviews with experienced persons from XaaS and software sectors. The interviews were supplemented by a desk review of multiple sources of secondary data, including a summary review of the academic literature using Google Scholar and Web of Science databases, and a more extensive review of the grey literature identified through a Google search as well as through interviews with the staff at Yellow. The main search terms used in various combinations were: “Malawi”, “Africa”, “solar home systems”, “energy justice”, “energy poverty”, “energy access”, “cost”, “electricity”, and “lighting”.
The qualitative input from these sources was then further explored using a quantitative analysis based on LCOL.
3.2 Quantitative modeling using the levelised cost of lighting
In the first phase, we consider the case of a low-income household in rural Malawi and have used a quantitative approach to calculate the LCOL based on various energy sources. Such households are not connected to a national grid but rely on firewood and batteries/torches as energy sources for cooking and lighting, respectively (National Statistic Office, 2020). For instance, only 1 % of the households surveyed in 2020 used electricity for cooking; the bulk of the households depended on firewood (79 %) and charcoal (18 %). In terms of lighting, 80% of households are reported to use dry-cell batteries/torches; only 11 % of households used electricity (National Statistic Office, 2020).
LCOL is a techno-economic approach which enables the comparison of various energy sources based on delivered light rather than energy consumed. It is an extension of the levelized cost of energy approach, now widely used in the literature (Aldersey-Williams and Rubert, 2019), the basis of which is to compare the cost of different energy systems. Although weakened by assumptions such as overnight capital costs, it nevertheless provides an important insight on relative costs and price targets (Menéndez, Martín, Varela-Candamio and García-Álvarez, 2020).
where:
It = investment expenditure in year t ($)
O&Mt = operational and maintenance expenditures in year t ($)
EEt = expenditure on lighting in year t ($)
r = discount rate
n = expected lifetime of the system
Lt = light output in year t (lux-hour)
Apart from the discount rate, which is a necessary input value for this calculation and for which a value of 9 % has been used, there are several other important variables for the assessment, as listed in Table 1.
Four differently sized SHS systems have been included in the analysis. In terms of battery lifetime, the value is a direct function of the number and the extent of the discharge cycles. Typically, a lithium battery which is discharged completely can be expected to have a lifetime of about 800 cycles, or close to 2 years for SHS systems which discharge almost completely each day; the latter value has been used for all the base-case scenarios in this analysis.
The conversion from the lumen rating of a light source, which is measured close to the source, and usable light, which is measured as the available lux on a working surface, were undertaken using the literature values for wick- and LED-based light losses, as also shown in Table 1. No assumptions were made in respect of focusing or channeling the output light, as may be applicable for desk lamps or torches. Light losses for wick-based systems are high due to the frequency spectrum of the emitted light.
It is noted that the use of LCOL as a measure of affordability does not imply any claims in respect of the morality of markets, and that markets are instruments for distributive justice. Indeed, it would be naïve and incorrect to do so. Markets cannot do the work of morals and should not be positioned as redistributive mechanisms. In the transformation of energy systems, it is important to hold in central focus the issues of identity, community, autonomy, and dignity. The firm, it is acknowledged, is a political organization in which power is repeatedly exercised. The allocation of limited or constrained resources within the firm and indeed, within the household, is not simply a matter of rational distribution based on the optimal return for the overall portfolio. It is, instead, guided by a more complex set of institutions which bring together the social, the cultural, and the economic in the form of a socio-technical system (Cherp, Vinichenko, Jewell, Brutschin and Sovacool, 2018). The relative influence of each dimension was, indeed, one of the foci for this study.
4 Results
4.1 The case study firm: Yellow
The company was established in 2017 as a pay-as-you-go solar power provider to African households (Timm, 2019). The business model is based on the increasing affordability of solar home systems as an alternative to conventional energy sources in SSA countries, particularly in Malawi and Uganda, where the average per capita incomes are low and many households are off-grid. Initially, however, the company struggled to implement a distribution system which was accessible, low cost, and reliable.
The problem was overcome by the development of Ofeefee, a web platform which is able to link customers, a network of agents working on commission, and the head-office staff (Timm, 2019). The platform allows the company to distribute its pico solar products in areas where the retail infrastructure is weak at best, and often non-existent. The significance of Ofeefee and the cloud-based nature of its architecture are discussed later.
The supply of SHS to rural customers requires a distributed network of agents extending from the manufacturer to the consumer. Different companies take different approaches to the value chain, from being completely integrated across manufacturing, distribution, and support to only focusing on a portion of the upstream or downstream. Yellow is focused on the importation, installation, maintenance, and customer support of SHS.
The company is structured into two distinct functions. Head-office activities including business planning, raising finance, development of software systems, and training of staff are managed by a small group of full-time employees operating from Cape Town. Market development and daily operational issues, mostly connected to the maintenance of the installed equipment and the installation of new units, are undertaken by a group of field agents working on commission and located close to the customer base in Malawi and Uganda.
The daily operations of the central office are supported by cloud-based services which provide all the utilities of their localized equivalents but at a fraction of the cost (Ross and Blumenstein, 2015; Sosinsky, 2011:23-44). At the time of the research, the company was a small entity in terms of the standard business classification and subject to the normal cash-flow constraints of such firms. The use of cloud-based services (XaaS)1 is therefore a logical decision given the need to avoid extensive upfront costs in hardware and software which are generally necessary to run a retail business.
All of the benefits of XaaS were considered by Yellow to be important. In addition, the company also valued the global reach of the services, which had enabled it to enter markets in a number of different countries. Options abounded in the deployment and configuration of XaaS for the support and enhancement of business operations. Yellow chose to focus on the use of one particular suite of XaaS called Zoho Creator, offered by Zoho, due to the flexibility of its development software (SaaS), the lower cost, and the availability of its infrastructure for storage (IaaS). Zoho does offer complete packages for customer-relationship management (CRM), but the scope for customization and refinement of the standard solution is more limited. Despite the initial development cost, the benefit of solutions customized to the specific needs of Yellow supported its decision to invest in Zoho Creator.
Once Yellow had their XaaS platform, it needed to be connected to systems in place to deploy and monitor the pico solar systems. Specifically, Yellow’s head office purchased and arranged for the importation of the SHS systems into Malawi and Uganda. Their staff in their Malawi and Uganda offices managed their deployment and the recruitment of sales agents, who interacted directly with the customers providing pre-sales and after-sales services. The interaction between Yellow and its agents and its customers was managed by the XaaS system. However, this is linked to an allied system which involves a mobile network operator (MNO), which provides a mobile money or MoMo platform, and the customer, who uses the SHS through a pay-as-you go (PayGo) MoMo system.
The flows of cash and data in the network between Yellow, its agents, the mobile network operator (MNO), and the customer, are shown in Figure 1.
There are two important, under-appreciated aspects of this network. Firstly, PayGo is a token system i.e., the customer receives a code for the operation of the SHS once payment has been made. In this retail system, the customer does not require to own or use a smartphone, although this is becoming increasingly common in the company’s main markets, but can make use of cash, MoMo credit, or bank credit to purchase a token and hence access the SHS functions. Secondly, all the components and actors are essential to the efficient functioning of the network. Without MoMo, there would be no reliable financial transactions; without PayGo, the customer would not be able to submit monthly installments; without XaaS, Yellow would not be able to manage its network of agents and customers in a cost-effective way.
There are two types of agents in the system. The MNO agents are typically small outlets which act as financial intermediaries, accepting cash as deposits to a MoMo account and in some cases, dispensing cash from these accounts. The Pico Solar agents work on commission, selling new systems to the customers based on their specification and providing customer support to existing installations.
Over a relatively short period, Yellow has been successful in its business model and has managed to raise debt-funding from several partners. For instance, in late 2021, it was granted US $4 million to support the ‘growing sales’ of SHS (SunFunder, 2021). According to the press release, “with over 110,000 units sold, Yellow has improved energy access for more than 400,000 people, mitigating 12,000 tons of CO2 emissions per year as they replace kerosene with clean solar lighting and other devices. The company has also created over 800 local jobs” (SunFunder, 2021).
4.2 Levelised cost of lighting
Now that we have introduced the company’s business model and the important role that an integrated technology solution plays, we need to understand if this provides for a cost-effective lighting option for households.
As noted earlier, there are several different types of SHS available. Customers are able to choose between various configurations depending on their daily energy requirements within the household. Typical sizes of the systems are shown in Table 2; the most popular choice is System A, which covers, as already mentioned, a 6 Wp solar panel, a 20 Wh battery, four light-emitting diodes (LED) , each of 1.25 W or 170 lumens, a charge point for a mobile phone, and a radio (a Tier 1 pico Solar System). The initial cost (of $10 in the case of System A) covers the installation and a portion of the system cost. Table 2 indicates the category of each system according to the Multi-Tier Framework. The high battery utilization rates, as shown in Table 2, reduce their lifetime to about 2 years, which means that regular support is essential to maintain a functioning system.
When comparing SHS, the most critical assumption is the daily energy usage, which is set at 120% of the total battery capacity, implying that at least a portion of the panel’s energy is used during the day when the battery is still being charged by the panel, mostly for the recharging of cell phones and the use of the radio. In other words, for system SHS A, which consists of a 6 Wp photovoltaic (PV) panel and a 20 Wh battery, supporting four LED lights, each of 1.25W, a radio, and a USB recharging port, the total energy usage per day is estimated to be 24 Wh, of which 18 Wh is used for lighting and 6 Wh for recharging electrical devices plus the radio.
The larger systems are preferred, where energy is required for refrigeration and other appliances. Previous studies have presented conflicting results on the cost effectiveness of the larger systems, with some studies concluding that they are sub-optimal, especially in locations with low solar irradiation during particular times of the year (Jamal, 2015; Monyei et al., 2018), and others being more positive about their overall impact (Phiri, 2015; Lemaire, 2018). For Yellow, SHS A is the most popular entry-level product, with the larger systems being used as an interim measure for meeting higher energy needs without having to purchase the hybrid systems such as PV/diesel generator or PV/wind, or even standalone diesel generators.
A number of comparisons are relevant and important in the evaluation of pico solar affordability. As a start, it is useful to compare the cost of lighting using SHS to the cost of the four alternatives available to rural homeowners in Malawi, namely the cost of candles, the cost of kerosene lamps, and the cost of torches or standalone lamps using either LEDs with disposable batteries or LEDs with rechargeable batteries.
A major difficulty with the comparison is the varying quality of light between candles, kerosene lamps, and LEDs. The latter are highly efficient light sources, providing an average light output of 150 lumen per watt vs. the average candle and lamp of 13 and 8 lumens, respectively (Mills, 2003). Replacing the light value of one LED of 0.87 W would require up to 10 candles or 16 hurricane lamps. In practice, this is not achieved; users accept a lower light intensity than might be recommended for tasks such as reading and cooking due to the limitations of the primary light sources and the financial implications of scaling thereof (such as using multiple kerosene lamps).
Recommended lighting levels vary depending on the task being undertaken. Typically, a room will require a lighting level of 300–500 lux (Richman, 2015). The number of lights necessary to achieve this level of illumination will depend on the size of the room, its geometry and the coloring of the surfaces. Assuming a 4 m-by 4 m room size and a standard LED output of 150 lumens, at least 4 LEDS will be required to achieve the required lighting level throughout the room (Richman, 2015). It is noted that surfaces for more intensive tasks such as reading would need a lighting level of at least 400 lux.
The estimated costs for this lighting specification based on a variety of battery configurations are shown in Table 3.
The lowest cost configuration is the system SHS A, followed by SHS D, and then a range of disposable batteries from D to AAA. We have not provided the calculations for SHS B or C to keep the table manageable as the calculations fall between the minimum size (SHS A) and maximum size (SHS D). A review of Table 3 highlights that battery options are clearly expensive alternatives to pico solar and unsuitable for sustained use within the context of this study. Disposable batteries are designed for short-term use within emergency power or mobile devices, and while having the benefit of versatility and convenience, will generally be unaffordable for long-term use.
The energy costs for all the configurations, as shown in Table 3, are inclusive of the equipment necessary to generate the energy, such as the lamps, batteries, and the recharging system, if applicable. Relative to the incremental cost of a grid-based electricity supply, which would typically amount to $4 per year based on an energy cost of $0.2/kWh, all the options are expensive. However, the comparison of incremental cost vs. the total cost for an integrated energy solution is inaccurate for this evaluation. Grid-based power can only be accessed once the initial investment in electrical equipment for direct connection to the grid (isolators and cabling), distribution boards, wiring in the home, Earth leakage systems, lighting equipment, and switches have been made. A comprehensive system, including all these components able to support multiple electrical devices, will cost over $10,000 per connection (Jamal, 2015). However, in many SSA countries, it is possible to install simpler hardware that supports the lighting and a few plug points. The cost of such a system is variable but, in this study, we have assumed a value of $550 per homestead, consisting of the connection fee of $300, covering the cabling and electricity meter, and the behind-the-meter costs of $250, covering the distribution board, inside wiring, plug points, and light fittings. It has been difficult to find the exact costs for Malawi on this as costs depend on the distance from grid connection, size of the house, etc. There appears to be very few published studies (academic or grey literature) that discuss these costs. These figures are based on anecdotal evidence from the region. That said, a recent newspaper report highlighted a connection charge of 92,300 kwacha (over US$ 5,000), although it had also been reported in September 2021 that the national electricity supplier in Malawi will connect over 100,000 households for free as part of a grid-connection drive2.
The LCOL for various types of lighting are shown in Table 4.
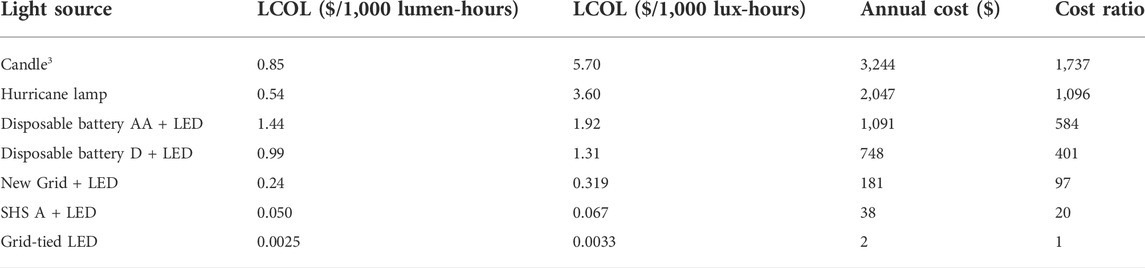
TABLE 4. LCOL and the annual cost for different energy sources at a specified light output (569,400 lux-hours).
The annual costs in this table have been calculated on the basis of 4 LEDs, each of 150 lumen output (1.25 W), operating for 4 h per day as per the data in Table 1. The least-cost option is clearly the option of the grid-tied LEDs (4 LEDs using an existing grid-connected energy system within the dwelling). In this case, the LCOL is the cost of the electrical energy at an input value of $0.17 per kWh and the initial cost of the LEDs with fittings. It is noted, however, that if the initial infrastructure cost for a grid-connection system is included, the LCOL is calculated to be $0.24/1,000 lux-hours, which is 5 times the cost of the SHS A (20 Wh).
Rather than comparing the annual cost for a certain light output, it is easier to visualize the total available lighting time per day for a budget of $60 per year or $5 per month, which is the average budget for lighting in a rural Malawian household (National Statistic Office, 2020). This comparison is shown in Figure 2, from which it is clear that the options of candles, hurricane lamps, and disposable battery/LED provide less than 1% of the required lighting of 150 lux per room on this budget. Given the high cost of providing the absolute minimum lighting using candles, kerosene, or disposable batteries, the options are either a grid-connection subsidy, as a means of offsetting the establishment cost of a direct grid system, or the use of SHS. The lower output, higher cost, and general safety/health inconvenience of candles and paraffin lamps explain their sudden decline in popularity, as mentioned in the introduction. The most cost-effective option, excluding the option of the ‘grid-tied connection’, which is unavailable in rural Malawi (Taulo, Gondwe and Sebitosi, 2015), is SHS A, which meets both the budget and the minimum light requirement for the average household as used in this study.
In summary, the cost of SHS-based lighting, inclusive of the cost of the equipment, is between $0.05 to $0.12 per 1,000 lux-hours, depending on the assumptions about daily energy usage and battery lifetime. This cost is highly relative to the incremental cost of lighting using grid-based electricity, but an order of magnitude lower than the cost of lighting from kerosene and hurricane lamps. This supports similar findings from elsewhere (Mills, 2003; Lemaire, 2018). SHS is cheaper and more effective as a lighting source and safer than the traditional lighting technologies of candles, paraffin lamps, and firewood. These advantages alone, without considering the associated socio-cultural factors, explain the rapid adoption of the systems within off-grid Malawi households.
5 Discussion
Small-scale solar is a major lighting source in developing countries (GOGLA, 2021b). The International Finance Corporation estimates that there are already 184 million existing users of the Tier 1 systems (SHS A and SHS B in this study), and a further 617 million people could be reached with these systems (Lighting Global, 2020). The same report notes that, globally, there are 840 million people without electricity access and 1 billion people connected to an unreliable grid, all of whom require Tier 1 to 4 systems as a primary or secondary lighting source.
Malawi is one such country. The figures for lighting fuel-use in Malawi have changed significantly over the last 8 years. In the 2011 survey, paraffin was the most widely used source of lighting fuel, accounting for 52% of the total households, followed by batteries (27%), and then electricity (National Statistic Office, 2012). By 2020, 88% of rural households were dependant on dry-cell batteries/torches for lighting (National Statistic Office, 2020).
The affordability of the torches/dry batteries is one reason for the almost total replacement of candles and paraffin lamps as the primary lighting sources over this period. The impact on the quality of life for the users has been significant, with the reported benefits including better quality lighting, cost savings, reduced exposure to the health and safety risks of handling paraffin, improvements in study time for children, and more consistent access to mobile networks (Lemaire, 2018). Based on the LCOL analysis of this study, SHS is cheaper, safer, and provides better quality lighting than its predecessors. More importantly, SHS is establishing itself in a neglected sector (rural Malawi) that is considered a high-risk environment for investments which has previously deterred investors seeking to build electricity infrastructures. Interest rates are typically at least 15% in dollar-based terms, or 25% in the local currency, which are an order of magnitude higher than the interest charges presently applicable in developed countries, reflecting the large difference in the perceived risk and the small size of the loans (Valev, 2022).
It is not only the high interest rates which have discouraged investors. As already mentioned, the level of disposable income is low. The average per capital income in rural Malawi is little more than $25 per month (De Magalhães and Santaeulàlia-Llopis, 2018), leading to the obvious conclusion that households are unable to afford an initial capital investment required to electrify their homes, or raise the finance from banks and other financial intermediaries. Hire purchase agreements, whereby households pay regular monthly subscriptions via MoMo and PayGo without the need for sizeable initial deposits, are perhaps the only realistic option for upgrading their energy systems.
Notwithstanding the benefits of SHS to rural homeowners, the systems have been criticized as exploitative of the poor (Samarakoon, 2020). The high-energy cost of SHS-based energy, relative to grid-based electricity, does indeed support this argument, if it is assumed that the grid infrastructure and the housing stock are subsidized in their entirety by the state. In this case, the energy cost may be as low as the grid-connected tariff, which in Malawi is reported to be $0.15 per kWh (International Finance Corporation, 2020). However, SHS at this level of use is not an energy source; it is a lighting solution which competes alongside hurricane lamps and candles. In this market, it has a higher perceived value and hence, market share. At the lowest level on the ladder of abstraction and theory, SHS meets a basic need in Malawian households for low-cost lighting, and simultaneously reduces their carbon footprint while also addressing indoor pollution issues.
The issues of affordability and energy justice could be covered to some extent by state subsidies for the purchase of small-scale solar systems, including SHS. Such an approach would be relatively simple to implement given that the systems use PayGo and MoMo. Funds could be directed to homeowners through MoMo accounts, specifically targeted at state support for monthly energy bills.
It is also possible that the retailers could reduce their cost of capital through government incentives or, more broadly, the use of social-impact bonds. The latter are relevant to the sector as a consequence of the positive contribution of SHS to decarbonization. For every GWh of coal-based electricity generation or paraffin-based lighting displaced or prevented, 750–950 tons of carbon dioxide release will be avoided (IEA, 2019). This benefit implies that companies selling SHS can qualify for carbon credits, or will be able to access lower cost finance. Given that SHS is capital-intensive, lower interest rates will decrease the cost of lighting and the access to new credit lines or lower interest-bearing loans should enable SHS retailers to reduce monthly rentals charged to their customers.
Throughout this discussion, SHS has been treated as a lighting source, with an additional benefit of being able to recharge a mobile device. It has been noted that SHS has emerged as an alternative lighting technology which is quickly replacing the use of fossil fuels in rural Malawi. In the analysis of this transition, it is apparent that market formation and market destruction are applicable only with respect to pico solar and candles/hurricane lamps, respectively. Overall, it is not a new market, but simply an existing market in which the primary energy supply is being changed.
In understanding this transition, a largely quantitative approach has been followed, showing that LED-based lighting has clear cost and quality benefits, and that it is these “technology and finance” issues which are partly responsible for driving the change. Moreover, the application of the intertwined platforms of MoMo, PayGo, and XaaS has enabled the company in this study, and presumably other similar companies, to compete against more established and larger companies, in the provision of lighting services. The intertwining of these platforms, the technological advances in LEDs and batteries, and the low-cost context in rural Malawi have together enabled SHS to become a popular choice as a lighting solution.
However, such a technocratic approach is clearly limited and fails to acknowledge the role of social and political factors that may also be important. Markets are economic institutions which result from political and social processes. Typically, market formation is not the consequence of rational economic actors making decisions to optimally allocate their individual resources. Struggles over market formation are often political processes in which the market institutions (rules and practices) themselves are shaped by powerful political agents (Breslau, 2013). In addition, technologies and products are not independent of their social context, but are socially constructed (Bijker, Hughes, Pinch and Douglas, 2012).
The use of MoMo for SHS is perhaps an example of the interrelationship between the product and its social setting. Based on her ethnographic studies in Kenya, Kusimba (2021 p12) argues that digital banking is “aimed at accessing, building, distributing, accumulating, preserving, and protecting wealth-in-people”. MoMo is a means to build economic and social networks, providing a means to grow and sustain broader communities of care. The link between MoMo and SHS, through which communities of care may indeed be able to swap assets in order to share access to critical resources, including energy, is an interesting proposition worthy of further study. Exchanges of this nature could assemble a new institution in energy markets, where the abandonment by the state of the market to private interests may lead to an alternative expression of public good.
6 Conclusion
In the absence of government programs to develop the electricity infrastructure within the poor areas of rural Malawi, households must rely on the most usable and affordable distributed technologies in order to meet their daily energy needs. The choice of technology will depend on the specific user requirements such as the highest power demand, average daily-energy usage, and the time period over which the energy is required.
For poor households at Tier 1 of the energy access MTF, where electricity is required for lighting at night and for the limited use of electrical appliances such as the recharging of a cell phone, pico solar is becoming the dominant option. However, even Tier 1 users need to use innovative financing options in order to meet the repayment costs of the initial equipment (solar panel, inverter, and LEDs). In this article, we have discussed how the company Yellow has addressed this issue through the development of its Ofeefee platform.
The platform combines the three technologies of PayGo, MoMo, and XaaS in order to support a network of agents and consumers who have purchased and are using pico solar systems as a source of lighting and limited electrical energy in rural Malawi. Growing from a low base, the company has sold more than 110,000 systems and is rapidly becoming a dominant supplier in Malawi’s rural areas (SunFunder, 2021).
The study's focus on the smaller Tier 1 systems from a lighting perspective and, which make use of innovative business models that integrate novel IT solutions (PayGo, MoMo and XaaS) has enabled us to reflect on key issues raised by those studying and theorising on energy justice. Specifically, the issue of fair distribution of the costs of pico solar and the importance of recognising the differentiation of actors and their needs. The paper has interrogated the costs of pico solar and discussed the fairness of difference sized systems in energy poor communities. It has highlighted the importance of thinking not just in broad energy terms but the importance of discriminating between energy and lighting to disaggregate the needs of energy poor communities more appropriately.
It is highly evident that the target of essential energy access, or the humblest principles of energy justice, will not be met through pico solar and private markets. These goals will only be achieved through substantial public investment in energy infrastructure. As such, this paper highlights a number of policy implications. First, in the absence of substantial public investment in energy infrastructure, pico solar is able to meet basic lighting needs within the limits of a household budget for those who are energy-poor. The case of Yellow highlights the possibility of using MoMo and pay-as-you go financing products without invoking more exploitative hire purchase and financing options, which have traditionally been at the core of the market development of solar systems. Moreover, the financial services which are used by the consumers (MoMo and PayGo) lend themselves to the delivery of energy subsidies or social grants, should public policy move in this direction within the foreseeable future. As noted above, such subsidies could be relatively easy to implement given the rise in PayGo and MoMo with funds directed to homeowners through MoMo accounts, specifically targeted at state support for monthly energy bills.
Second, rethinking the tier system to recognise that energy access for the poorest is often not about ‘energy’ as much as ‘lighting’ is important. Our LCOL analysis is a methodological contribution to the field of solar energy studies in Africa being one of the only such studies but also has provided an important policy finding too. It has highlighted the ability of pico solar to deliver better quality lighting at a lower LCOL than the traditional technologies of candles and hurricane lamps. Changing the narrative to consider the relative benefits of solar for its lighting potential is essential with only 11% of the Malawian population using electricity, recognition of the difficulty of grid connection in rural areas (Eales et al., 2020) and the difficulty of ensuring public financing for such connection. It is imperative that policy discussions are considered in terms of lighting and not in terms of the broader term of ‘energy’ to help address the dissatisfaction of households in trying to move to solar solutions in Malawi who often expected systems to power more than they were actually able to (see Samarakoon, 2020). Specifically, additional support – if state subsidy for the least-cost option of grid connection is not available – and promotion for the system SHS A described above (with a 6 Wp photovoltaic (PV) panel and a 20 Wh battery, supporting four LED lights, each of 1.25W, a radio and a USB recharging port) for lighting purposes is recommended.
In summary, innovative solutions for the delivery of low-cost lighting and electrical energy to poor households within SSA countries have been developed, and could be further extended with greater support from public policy. The solutions rely on the use of novel technologies for financial services and information technology, which could transform the access to affordable credit within rural communities. It is recommended that all governments in developing countries look more closely at how this platform could be incorporated within its energy planning as a means of increasing energy access in the absence of public finance for the extension of grid-connected services.
Data availability statement
The raw data supporting the conclusion of this article will be made available by the authors, without undue reservation.
Author contributions
Conceptualization, RH and DW; Formal analysis, DW; Investigation, DW; Methodology, RH and DW; Validation, RH and DW; Visualization, RH and DW; Writing—original draft, RH and DW.
Conflict of interest
The authors certify that they have no financial interest or direct involvement in any of the organizations or other aspects of subject matter as discussed in this manuscript. DW, however, has an indirect link to the company Yellow, which has been used as a case study, through his son, Benjamin Walwyn, who is a founder and shareholder in the company. DW owns no shares in Yellow, or will in any way benefit from the success (or failure) of the company. Neither authors have shares or interests in the solar lighting sector, or consult to any companies in the sector.
The remaining author declares that the research was conducted in the absence of any commercial or financial relationships that could be construed as a potential conflict of interest.
Publisher’s note
All claims expressed in this article are solely those of the authors and do not necessarily represent those of their affiliated organizations, or those of the publisher, the editors, and the reviewers. Any product that may be evaluated in this article, or claim that may be made by its manufacturer, is not guaranteed or endorsed by the publisher.
Footnotes
1XaaS stands for ‘anything as a service’ and usually refers to a number of different cloud-based services. The X is changed to an I when the focus is on infrastructure-based cloud services or S when it refers to the supply of a bespoke software application.
2See: https://allafrica.com/stories/202108300913.html and https://allafrica.com/stories/202109270260.html
3For the option of candles, it is calculated that in order to provide an equivalent light intensity, at least 88 candles need to be burning simultaneously.
References
Adkins, E., Eapen, S., Kaluwile, F., Nair, G., and Modi, V. (2010). Off-grid energy services for the poor: Introducing LED lighting in the Millennium Villages Project in Malawi. Energy Policy 38 (2), 1087–1097. doi:10.1016/j.enpol.2009.10.061
Aldersey-Williams, J., and Rubert, T. (2019). Levelised cost of energy – a theoretical justification and critical assessment. Energy Policy 124, 169–179. doi:10.1016/j.enpol.2018.10.004
Bhatia, M., Angelou, N., and Portale, E. (2015). Beyond connections: Energy access redefined-technical report 008/15. Energy sector management assistance program. Available: https://mtfenergyaccess.esmap.org/data/files/download-documents/full_report_beyond_connection.pdf (Accessed December 30, 2021).
Bijker, W. E., Hughes, T. P., Pinch, T., and Douglas, D. G. (2012). The social construction of technological systems: New directions in the sociology and history of technology. Cambridge: MIT press.
BloombergNEF, (2020). Battery Pack Prices Cited Below $100/kWh for the First Time in 2020, While Market Average Sits at $137/kWh [Online]. New York: Bloomberg. Available: https://about.bnef.com/blog/battery-pack-prices-cited-below-100-kwh-for-the-first-time-in-2020-while-market-average-sits-at-137-kwh/(Accessed March 25, 2021).
Breslau, D. (2013). Designing a market-like entity: Economics in the politics of market formation. Soc. Stud. Sci. 43 (6), 829–851. doi:10.1177/0306312713493962
Cherp, A., Vinichenko, V., Jewell, J., Brutschin, E., and Sovacool, B. (2018). Integrating techno-economic, socio-technical and political perspectives on national energy transitions: A meta-theoretical framework. Energy Res. Soc. Sci. 37, 175–190. doi:10.1016/j.erss.2017.09.015
De Magalhães, L., and Santaeulàlia-Llopis, R. (2018). The consumption, income, and wealth of the poorest: An empirical analysis of economic inequality in rural and urban Sub-Saharan Africa for macroeconomists. J. Dev. Econ. 134, 350–371. doi:10.1016/j.jdeveco.2018.05.014
Eales, A., Alsop, A., Frame, D., Strachan, S., and Galloway, S. (2020). Assessing the market for solar photovoltaic (PV) microgrids in Malawi. Hapres J. Sustain. Res. 2 (1), e200008. doi:10.20900/jsr20200008
Eales, A., and Unyolo, B. (2018). Renewable energy mini-grids in Malawi: Status, barriers and opportunities. Scotland: University of Strathclyde: Strathclyde. Available: https://strathprints.strath.ac.uk/64868/1/Eales_Unyolo_2018_Renewable_Energy_Mini_grids_in_Malawi.pdf (Accessed January 4, 2022).
Economides, N., and Jeziorski, P. (2017). Mobile money in Tanzania. Mark. Sci. 36 (6), 815–837. doi:10.1287/mksc.2017.1027
Energy For Growth Hub (2021). The Modern Energy Minimum: The case for a new global electricity consumption threshold. Washington: Energy For Growth Hub. Available: https://www.energyforgrowth.org/projects/modern-energy-minimum/ (Accessed March 15, 2021).
Global, L. (2018). Off-grid solar market trends report 2018. Washington: Lighting Global. Available: https://www.lightingglobal.org/wp-content/uploads/2018/02/2018_Off_Grid_Solar_Market_Trends_Report_Summary.pdf (Accessed March 15, 2021).
Global, L. (2020). Off-grid solar market trends report 2020. Washington: Lighting Global. Available: https://www.lightingglobal.org/wp-content/uploads/2020/03/VIVID%20OCA_2020_Off_Grid_Solar_Market_Trends_Report_Full_High.pdf (Accessed March 25, 2021).
Gogla, (2021a). About Us [Online]. Available: https://www.gogla.org/about-us.
Gogla, (2021b). Global off-grid solar market report semi-annual sales and impact data. Amsterdam: GOGLA. Available: https://www.gogla.org/ (Accessed January 24, 2022).
Gogla, (2018). Powering opportunity: The economics impact of off-grid solar. Available: https://www.gogla.org/sites/default/files/resource_docs/gogla_powering_opportunity_es.pdf (Accessed February 3rd, 2021).
Heffron, R. J., McCauley, D., and Sovacool, B. K. (2015). Resolving society's energy trilemma through the Energy Justice Metric. Energy Policy 87, 168–176. doi:10.1016/j.enpol.2015.08.033
IEA (2019). Global Energy & CO2 Status Report: The latest trends in energy and emissions in 2018. Paris: International Energy Agency. Available: https://www.iea.org/reports/global-energy-co2-status-report-2019/emissions (Accessed March 29, 2021).
International Finance Corporation (2020). Regulatory and tariff review for distributed generation in the commercial and industrial sectors in southern Africa. Washington: International Finance Corporation. Available: https://www.ifc.org/wps/wcm/connect/090c58a2-2b98-482e-8c6d-b5931ed793e2/202006-Regulatory-Tariff-Review-Southern-Africa.pdf?MOD=AJPERES&CVID=nbDqlVa (Accessed March 31, 2021).
IRENA (2020). Renewable power generation costs in 2019. Abu Dhabi: International Renewable Energy Agency. (IRENA): Abu Dhabi. Available: www.irena.org/home/publications.
Jamal, N. (2015). Options for the supply of electricity to rural homes in South Africa. J. energy South. Afr. 26 (3), 58–65. doi:10.17159/2413-3051/2015/v26i3a2129
Jenkins, K., McCauley, D., Heffron, R., Stephan, H., and Rehner, R. (2016). Energy justice: A conceptual review. Energy Res. Soc. Sci. 11, 174–182. doi:10.1016/j.erss.2015.10.004
Kabengele, C., and Hahn, R. (2021). Institutional and firm-level factors for mobile money adoption in emerging markets–A configurational analysis. Technol. Forecast. Soc. Change 171, 120934. doi:10.1016/j.techfore.2021.120934
Kusimba, S. (2021). Reimagining money: Kenya in the digital finance revolution. Stanford, California: Stanford University Press.
Lemaire, X. (2018). Solar home systems and solar lanterns in rural areas of the Global South: What impact? WIREs Energy Environ. 7 (5), e301. doi:10.1002/wene.301
Menéndez, R. P., Martín, A. P., Varela-Candamio, L., and García-Álvarez, M.-T. (2020). An enhanced techno-economic analysis of LCOE: Public incentives vs private investment. Technol. Econ. Dev. Econ. 27, 1–23. doi:10.3846/tede.2020.11259
Mills, E. (2003). Technical and economic performance analysis of kerosene lamps and alternative approaches to illumination in developing countries. Berkeley: Lawrence Berkeley National Laboratory Report, 1–16.
Monyei, C. G., Adewumi, A. O., and Jenkins, K. E. H. (2018). Energy (in)justice in off-grid rural electrification policy: South Africa in focus. Energy Res. Soc. Sci. 44, 152–171. doi:10.1016/j.erss.2018.05.002
Narayan, N., Chamseddine, A., Vega-Garita, V., Qin, Z., Popovic-Gerber, J., Bauer, P., et al. (2019). Exploring the boundaries of Solar Home Systems (SHS) for off-grid electrification: Optimal SHS sizing for the multi-tier framework for household electricity access. Appl. energy 240, 907–917. doi:10.1016/j.apenergy.2019.02.053
National Statistic Office (2012). The 3rd integrated household report (IHS3). Blantyre: Malawi Government. Available: http://www.nsomalawi.mw/images/stories/data_on_line/economics/ihs/IHS3/IHS3_Report.pdf (Accessed March 19th, 2021).
National Statistic Office (2020). The 5th integrated household report (IHS5). Blantyre: Malawi government. Available: https://microdata.worldbank.org/index.php/catalog/3818 (Accessed March 19th, 2021).
Nygaard, I., Hansen, U. E., and Larsen, T. H. (2016). The emerging market for pico-scale solar PV systems in Sub-Saharan Africa: From donor-supported niches toward market-based rural electrification. Copenhagen: UNEP DTU Partnership. Available:.https://collaboration.worldbank.org/content/usergenerated/asi/cloud/attachments/sites/collaboration-for-development/en/groups/energyaccess/documents/jcr:content/content/primary/blog/the_emerging_market-ZZmf/The-emerging-market-for-pico-scale-solar-PV-systems-in-Sub-Saharan-Africa-From-donor-supported-niches-toward-market-based-rural-electrification.pdf.
Onsongo, E. (2019). Institutional entrepreneurship and social innovation at the base of the pyramid: The case of M-pesa in Kenya. Industry Innovation 26 (4), 369–390. doi:10.1080/13662716.2017.1409104
Phiri, F. O. M. (2015). Energy poverty of rural households in Malawi: Potential for renewable energy options and more efficient use of biomass to reduce vulnerability. Ås: Norwegian University of Life Sciences.
Richman, E. E. (2015). Requirements for lighting levels. Pacific northwest national laboratory. Available: (Accessed April 8, 2015).https://www. wbdg. org/pdfs/usace_lightinglevels.pdf.
Ross, P. K., and Blumenstein, M. (2015). Cloud computing as a facilitator of SME entrepreneurship. Technol. Analysis Strategic Manag. 27 (1), 87–101. doi:10.1080/09537325.2014.951621
Samarakoon, S. (2020). The troubled path to ending darkness: Energy injustice encounters in Malawi’s off-grid solar market. Energy Res. Soc. Sci. 69, 101712. doi:10.1016/j.erss.2020.101712
SEA4ALL (2021). Malawi. Available: https://www.se4all-africa.org/seforall-in-africa/country-data/malawi/(Accessed November 22, 2022).
Smith, K., Eales, A., Frame, D., and Galloway, S. (2019). IEEE, pp 1-7, Sosinsky, B. 2011. Cloud computing bible. Indianapolis: John Wiley & Sons.Techno-economic analysis of PAYG productive uses of energy in Malawi. 2019 IEEE global humanitarian technology conference (GHTC).
Sovacool, B. K., Burke, M., Baker, L., Kotikalapudi, C. K., and Wlokas, H. (2017). New frontiers and conceptual frameworks for energy justice. Energy Policy 105, 677–691. doi:10.1016/j.enpol.2017.03.005
Sovacool, B. K., and Dworkin, M. H. (2015). Energy justice: Conceptual insights and practical applications. Appl. Energy 142, 435–444. doi:10.1016/j.apenergy.2015.01.002
SunFunder (2021). New $4m investment in Yellow in Malawi [online]. London: SunFunder. Available: https://www.sunfunder.com/post/yellow-malawi (Accessed January 3, 2021).
Suri, T., and Jack, W. (2016). The long-run poverty and gender impacts of mobile money. Science 354 (6317), 1288–1292. doi:10.1126/science.aah5309
Taulo, J. L., Gondwe, K. J., and Sebitosi, A. B. (2015). Energy supply in Malawi: Options and issues. J. energy South. Afr. 26 (2), 19–32. doi:10.17159/2413-3051/2015/v26i2a2192
Timm, S. (2019). SA startup Yellow lands $725k to help distribute solar energy device in Uganda. Cape Town: Burn Media. Malawi [Online]Available: https://ventureburn.com/2019/11/sa-startup-yellow-725k-solar-energy/.
USAID (2019). UAAID Southern Africa energy program Malawi country profile. Available: http://pdf.usaid.gov/pdf_docs/PA00TWBQ.pdf(Accessed November 22, 2021).
Valev, N. (2022). Malawi: Lending interest rate [online]. Georgia state: The GlobalEconomy. Available: https://www.theglobaleconomy.com/Malawi/Lending_interest_rate/(Accessed January 24, 2022).
Keywords: pico solar, levelized cost of lighting, mobile money, energy access, energy justice
Citation: Walwyn DR and Hanlin R (2022) Broadening energy access for poor households in rural malawi: How pico solar, mobile money, and cloud-based services are being combined to address energy exclusion. Front. Energy Res. 10:877307. doi: 10.3389/fenrg.2022.877307
Received: 16 February 2022; Accepted: 18 August 2022;
Published: 29 September 2022.
Edited by:
Fateh Belaid, King Abdullah Petroleum Studies and Research Center (KAPSARC), Saudi ArabiaReviewed by:
Idiano D.’ Adamo, Sapienza University of Rome, ItalyJoseph Ntayi, Makerere University, Uganda
Copyright © 2022 Walwyn and Hanlin. This is an open-access article distributed under the terms of the Creative Commons Attribution License (CC BY). The use, distribution or reproduction in other forums is permitted, provided the original author(s) and the copyright owner(s) are credited and that the original publication in this journal is cited, in accordance with accepted academic practice. No use, distribution or reproduction is permitted which does not comply with these terms.
*Correspondence: David R. Walwyn, ZGF2aWQud2Fsd3luQHVwLmFjLnph