- 1Department of Biochemistry, Institute for Medical Science, Jeonbuk National University Medical School, Jeonju, South Korea
- 2Department of Emergency Medicine and BioMedical Science Graduate Program (BMSGP), Chonnam National University, Gwangju, South Korea
- 3Department of Emergency Medical Rescue, Nambu University, Jeonbuk National University Medical School, Gwangju, South Korea
The mTOR pathway is a crucial biological regulatory mechanism of cell growth, proliferation and cell death, and its inhibitors were new candidates of anticancer drugs through regulation of energy balance and metabolism. In the present study, whether brazilin and mTOR inhibitor (Torin1) exerts anti-cancer effects was evaluated and the mechanism of its regulation in colorectal cancer cells investigated. Brazilin showed dose- and time-dependent cytotoxicity of colorectal cancer cells (SW480 cells) through apoptosis pathways such as Bcl-2, Bax, as well as cleavage of caspase 3, caspase 9, and PARP1. In addition, brazilin reduced mammalian target of rapamycin (mTOR) phosphorylation in a dose- and time-dependent manner, and the mTOR inhibitor torin 1 blocked this phosphorylation. Brazilin also decreased heme oxygenase-1 (HO-1) expression in a dose- and time-dependent manner; however, hemin, a specific HO-1 substrate, markedly increased HO-1 expression. Torin 1 reduced hemin-induced HO-1 expression and increased colorectal cell death in a dose-dependent manner in the presence and absence of hemin. Moreover, nuclear factor erythroid 2–related factor 2 (Nrf2) translocation into nucleus fraction was crucial role in brazilin-mediated apoptosis of colorectal cancer cells. These results showed that brazilin and torin1 might regulate the mTOR signaling pathway by decreasing mTOR phosphorylation. Furthermore, mTOR signaling was associated with brazilin-regulated HO-1 expression, which induced apoptosis in colorectal cancer cells. These results suggest that synthetic and/or natural mTOR inhibitors were useful candidate for treatment of colorectal cancer cells.
Introduction
The mammalian target of rapamycin (mTOR), the activated downstream molecule in the phosphatidylinositol 3-kinase (PI3K)/Akt pathway, plays a major role in regulation of translation initiation through interactions with two distinct proteins, raptor and rictor. mTORC1 is sensitive to rapamycin, while mTORC2 is insensitive to rapamycin (Sarbassov et al., 2005). Rapamycin interacts with the immunophilin FK506 binding protein (FKBP12) (Sabatini et al., 1994; Jacinto and Hall, 2003), and the rapamycin-FKBP12 complex binds to the FKBP12-rapamycin binding domain (FRB) of mTORC1 but not to the FRB of mTORC2 (Jacinto et al., 2004; Sarbassov et al., 2004). Heme oxygenase-1 (HO-1) is induced by rapamycin and wortmannin in human pulmonary artery endothelial cells and human pulmonary artery smooth muscle cells (Visner et al., 2003). Particularly, mTORC1 correlated to intracellular energy charges on storages/consumptions of ATP, oxygen and effects to DNA replications/damages and regulated metabolic pathway (Saxton and Sabatini, 2017). In addition, HO-1 is overexpressed in rapamycin-treated renal cancer cells (Banerjee et al., 2012). In Tsc2-deficient neurons, HO-1 expression is increased, but rapamycin inhibits this increase (Di Nardo et al., 2009). These findings suggest that the PI3K-mTOR signaling pathway plays a role in HO-1 expression.
HO-1 is a microsomal enzyme that catalyzes the degradation of heme to carbon monoxide (CO), free iron ion, and biliverdin (Maines, 1997). Subsequently, biliverdin is enzymatically converted to bilirubin by biliverdin reductase (Florczyk et al., 2008). HO-1 plays cytoprotective roles, demonstrating antioxidant (Clark et al., 2000), anti-inflammatory (Willis et al., 1996; Kapturczak et al., 2004), anti-proliferative (Lee et al., 1996; Peyton et al., 2002; Deng et al., 2004), and anti-apoptotic properties (Brouard et al., 2000; Choi et al., 2004). However, the anti-apoptotic properties of HO-1 facilitate tumor progression. HO-1 is highly expressed in various human tumor tissues compared with normal tissue, such as prostate cancer (Maines and Abrahamsson, 1996), lung cancer (Degese et al., 2012), oral squamous cell carcinoma (Gandini et al., 2012), and colon cancers (Kang et al., 2012; Yin et al., 2014; Liu et al., 2021). High HO-1 expression in non-small cell lung cancer patients is associated with poor prognosis (Tsai et al., 2012; Wang et al., 2020). Conversely, HO-1 is associated with favorable prognosis in colorectal cancer (Becker et al., 2007). Increased HO-1 expression inhibits apoptosis in colon cancer cell line CaCo2 (Busserolles et al., 2006). However, increased HO-1 expression induces apoptosis in HCT116 cells (Andrés et al., 2014). Therefore, the role of HO-1 remains controversial.
Plants could be a rich source of novel bioactive compounds (Si et al., 2008; Hu et al., 2016; Wang et al., 2021), which are relatively unexplored (Si et al., 2013a; Huayu Liu et al., 2021a). The search for components and compositions isolated from plants is a permanent challenge, which still leads to new discoveries (Si et al., 2013b; Huayu Liu et al., 2021b; Ting Xu et al., 2021). The major component of Caesalpinia sappan L., brazilin (7, 11b-dihydrobenz[b]indeno[1,2-d]pyran-3,6a,9,10 (6H)-tetrol), is a natural red pigment used for morphological observation (Puchtler and Sweat, 1964; Puchtler et al., 1986). Brazilin exhibits various biological activities, such as anti-cancer (Lee et al., 2013; Zhang et al., 2018), anti-inflammation (Hikino et al., 1977; Bae et al., 2005; Sasaki et al., 2007), cell protection from BrCCl3-induced hepatic disorder (Moon et al., 1992), induction of immunological tolerance (Choi and Moon, 1997; Mok et al., 1998), anti-platelet activity (Hwang et al., 1998), inhibition of protein kinase C and insulin receptor kinase (Kim et al., 1998), and induction of vasorelaxation (Hu et al., 2003). Brazilin upregulates HO-1 through phosphoinositide 3-kinase (PI3K), protein kinase B (PKB/Akt) and extracellular signal-regulated kinase (ERK) signaling pathways in auditory cells (Choi and Kim, 2008).
The effects of brazilin have been studied in other cells but not in colorectal cancer. Therefore, it is currently not known how brazilin regulates its target genes in colorectal cancer. In this present study, the anti-cancer effects of brazilin were investigated and its target gene clarified to understand the significance of brazilin in colorectal cancer.
Materials and Methods
Cell Culture and Reagents
Human colorectal cancer cell line SW480 was cultured in Dulbecco’s modified Eagle’s medium supplemented with 10% heat-inactivated fetal bovine serum, 100 U/mL penicillin, and 100 μg/ml streptomycin (Gibco, NY, United States) at 37°C in a humidified atmosphere of 5% CO2. Brazilin was purchased from MP Biomedicals (Santa Ana, CA, United States). Hemin was purchased from Sigma-Aldrich (St. Louis, MO, United States). Torin1 was purchased from Tocris Bioscience (Avonmouth, Bristol, United Kingdom). The reagents were dissolved in dimethyl sulfoxide (DMSO), and aliquots were stored at −80°C. Stock solutions were diluted in culture medium before use to appropriate concentration.
Cell Viability Assay
Cells were seeded in 96 well plates at a numbers of 5 × 104 cells per well. After 18 h incubation, cells were incubated with various dose of brazilin, an inducer (hemin) of HO-1, and mTOR inhibitor (Torin1). After incubation for 48 h or 72 h, 20 μL of the stock solution (final concentration 0.5 mg/ml) of 3-(4,5-dimetylthiazol-2-yl)-2,5-diphenyltetrazolium bromide (MTT, Sigma-Aldrich, MO, United States) was added to individual well, and further incubated for 30 min in CO2 incubator. After removal of the supernatant, 0.1 ml of DMSO were added to each well. The formazan in each well were solubilized for 20 min at room temperature, and the absorbance were measured at an 570 nm using a microplate reader (SPECTRA MAX PLUS, Molecular Devices, CA, United States).
Western Blot Analysis
Cells were plated in 60-mm dishes at a density of 2.4 × 106 cells. After overnight incubation, cells were treated with different concentrations of brazilin and mTOR inhibitor or hemin. After incubation for 48 h or 72 h, cells were scraped and lysed. Proteins (30 μg) were separated on SDS-PAGE and transferred to PVDF membranes (GE Healthcare, United Kingdom). The blots were incubated overnight at 4°C with primary HO-1 (SPA-896) (Stressgen, MI, United States), Bax (#2772), cleaved caspase-3 (#9664), cleaved caspase-9 (#9505), PARP (#9542), mTOR (#2972), phospho-mTOR (Ser2448) (#2971) (Cell Signaling Technology, MA, United States), Bcl-2 (SC-7382), Nrf2 (SC-722), PCNA (SC-7907), β-actin (SC-47778) (Santa Cruz Biotechnology, TX, United States) antibodies. The blots were washed with TBS-T buffer and incubated with secondary horseradish peroxidase conjugated goat anti-rabbit and anti-mouse IgG antibodies (Santa Cruz Biotechnology). The reaction was detected using a chemiluminescence system (Millipore, Bedford, MA, United States). The intensities of positive bands were quantitated by densitometry analysis program (ImageQuant LAS 4000, NJ, United States) and expressed as a ratio of control group on the basis of β-actin level.
Flow Cytometry
Cellular apoptosis was assessed with annexin V and propidium iodide (PI) staining using commercial apoptosis assay kit (V13241, Molecular Probes, OR, United States) according to the manufacturer’s instruction. Briefly, SW480 cells were inoculated in a 6 well plate at a number of 1.5 × 106 cells and incubated with brazilin for 72 h. The cells were detached by trypsinization, washed with DPBS, and then resuspended in buffer at a concentration of 1 × 106 cells/mL. Next, 5 μL of FITC-conjugated annexin V and 2 μL of PI were added to resuspended cells, followed by incubation at room temperature for 15 min. The cells were washed and resuspended in binding buffer. The cells were analyzed using flow cytometry on a FACS Calibur (BD Biosciences, CA, United States).
Evaluation of Apoptosis
Apoptosis was assessed using the commercial assay kit (A1000, Biocolor Ltd., Northern Ireland) according to the manufacturer’s instruction. Briefly, SW480 cells were seeded in a 96-well plate at a number of 5 × 104 cells per well and incubated with brazilin for 72 h. After incubation for the indicated time period, the culture mediums were replaced with fresh mediums containing assay dye. The morphologies of cells were observed under an inverted microscope.
Quantitative Real-Time Polymerase Chain Reaction
Total RNA was extracted (Bioneer, Seoul, Korea) and variation in mRNA level of all genes were normalized to the housekeeping gene, GAPDH. Data were expressed the mean ± S.E.M of three independent experiments (Jang et al., 2020).
Electrophoretic Mobility Shift Assay
Nuclear extracts of the cell were prepared and electrophoretic mobility shift assay (EMSA) were performed as described in previous reports (Hellman and Fried, 2007; Jang et al., 2020). Specific binding were controlled by competitive reaction by treatment of excess of cold Nrf2 oligonucleotide (50 folds).
Statistical Analysis
All data are presented as mean ± standard deviation (S D). Statistical analysis was performed using Student’s t-test. Significant differences between groups were noted at p < 0.05.
Results
Inhibitory Effects of Brazilin on Cell Viability in Colorectal Cancer Cells
To investigate the effects of brazilin on cell viability in SW480 cells, the MTT assay was performed. A significantly greater inhibition of cell viability was observed with increasing concentration and duration of brazilin treatment (Figure 1A). Subsequently, to determine whether the signaling pathway underlying the cytotoxic mechanism of brazilin on SW480 cells was associated with apoptosis, elevation/reduction of apoptotic marker genes was analyzed using Western blot. The Bax expression was significantly increased and Bcl-2 decreased in brazilin-treated cells in a dose dependent manner. Parallelly, the cleaved caspase-3 and -9 level and PARP expression were significantly increased by brazilin treatment in a dose dependent manner in SW480 cells. (Figure 1B).
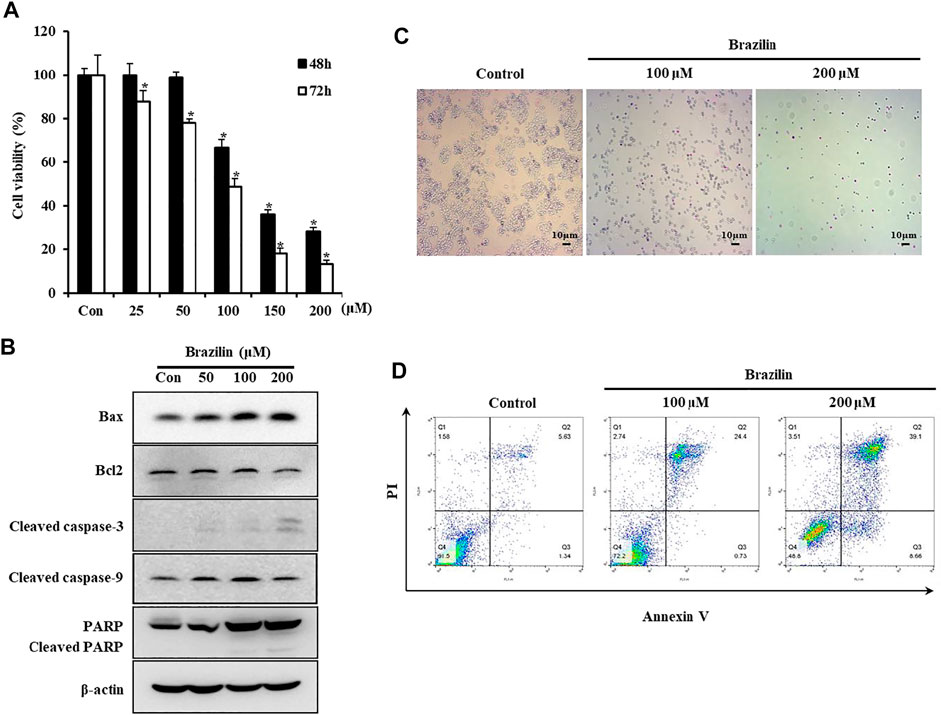
FIGURE 1. The effects of brazilin on cell viability in colorectal cancer SW480 cells. (A) SW480 cells were treated with different dosages of brazilin for 48 h or 72 h. Cell viabilities were measured by MTT assay. Data are shown as mean ± SD of three independent experiments. The p-values determined using Student’s t-test were *p < 0.05 compared with controls. (B) SW480 cells were treated with different concentrations of brazilin for 72 h. Whole cell lysates were prepared, and apoptosis was assessed using Western blot analysis with anti-Bax, anti-Bcl2, anti-cleaved caspase-3, anti-cleaved caspase-9, and anti-PARP/cleaved PARP antibodies. After treatment with brazilin for 72 h, cells were analyzed using APOPercentage Apoptosis Assay kit (C) and flow cytometry with annexin V and PI staining (D). The experiments were repeated for at least of three times.
Brazilin Downregulates Cell Viability via Apoptosis
To further investigate cell death caused by brazilin in SW480 cells, apoptotic cells were assessed using microscopic- and fluorometric-apoptosis assay. Results for microscopic apoptosis assay are presented in Figure 1C. The pink-colored deposits indicated the existence of apoptotic cells, which were observed in brazilin-treated cells. In addition, the identifiable cells were significantly decreased compared with the control in microscopic windows. The Annexin V-FITC and PI double-stained cells also showed a significant increase in apoptotic cells (Figure 1D).
Brazilin Downregulates mTOR Phosphorylation by Reducing HO-1 Expression
To investigate whether brazilin regulates mTOR phosphorylation in SW480 cells, Western blot analysis was performed. Brazilin treatments were reduced mTOR phosphorylation in a dose- (Figure 2A) and time- (100 μM brazilin) (Figure 2B) dependent manner. In contrast, brazilin didn’t show any affects to total endogenous mTOR level in the cells (Figures 2A,B). While torin1, selective and potent ATP-competitive inhibitor of mTOR inhibitor, decreased mTOR phosphorylation (Figure 2C). Similarly, torin1 also no affects were shown in changes of mTOR expression level in SW480 cells.
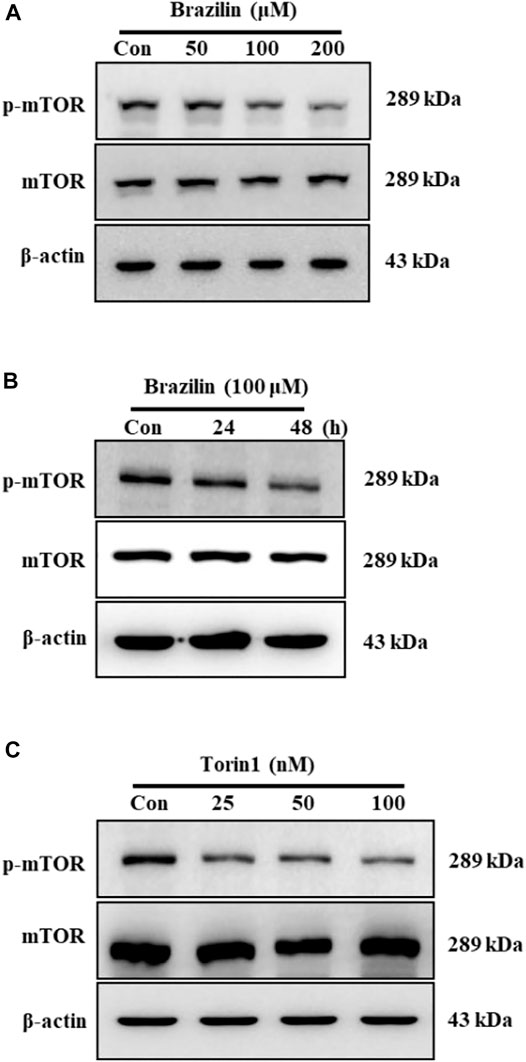
FIGURE 2. Effects of brazilin on mTOR phosphorylation in colorectal cancer SW480 cells. SW480 cells were treated with different concentrations of brazilin for 48 h (A) and for indicated times with 100 μM brazilin (B). (C) SW480 cells were treated with various concentrations of Torin1 for 48 h. Whole cell lysates were prepared, and phospho-mTOR was detected using Western blot analysis with anti-phospho-mTOR. The experiments were repeated for at least of three times.
mTOR, Regulation of HO-1 Expression and Cell Viabilities
In previous studies, the mTOR inhibitor rapamycin was shown to increase or decrease HO-1 expression (Visner et al., 2003; Zhou et al., 2005; Di Nardo et al., 2009). Therefore, Western blot analysis was performed to investigate whether mTOR inhibition decreased HO-1 expression. In addition, hemin-induced HO-1 expression was decreased via increased or decreased downregulation of mTOR phosphorylation mediated by increased concentration of mTOR inhibitor (Figure 3A). Subsequently, the MTT assay was performed with the mTOR inhibitor Torin1 to clarify the effect of mTOR inhibition on cell viability. Cell viability was significantly decreased in Torin1-treated cells (Figure 3B). The cell protective effects of brazilin on HO-1 were confirmed using hemin and Torin1. Pretreatment with hemin prevented brazilin-induced cell death (Figure 3C).
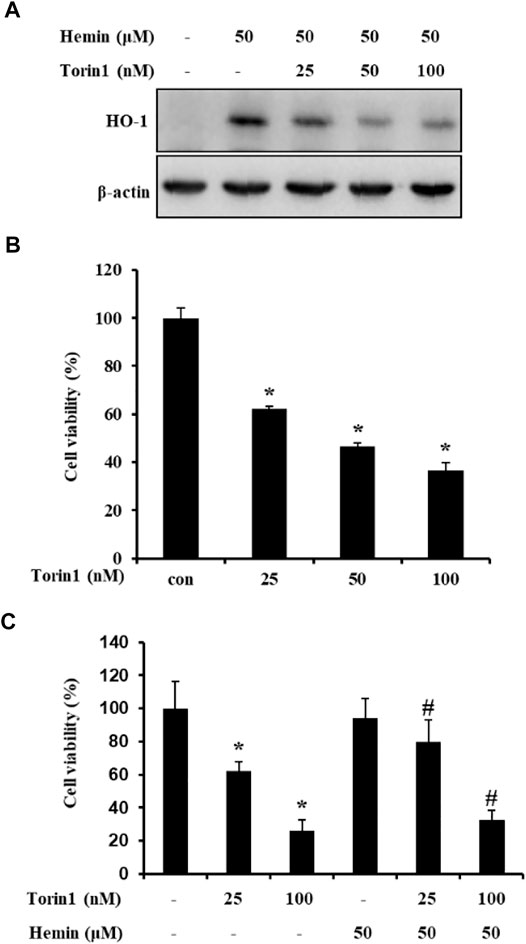
FIGURE 3. Torin1 inhibition of HO-1 expression in colorectal cancer SW480 cells. (A). After pretreatment with hemin for 2 h, SW480 cells were treated with different concentrations of Torin1 for 48 h. Whole cell lysates were prepared, and HO-1 was detected using Western blot analysis with anti-HO-1. (B). SW480 cells were treated with Torin1 for 48 h. (C) After pretreatment with hemin and Torin1 for 2 h, cells were treated with brazilin for 48 h. Cell viability was measured using the MTT assay. Results shown are mean ± SD of three independent experiments. The p-values determined using Student’s t-test were *p < 0.05 compared with cells treated only with torin1.#p < 0.05 vs. compared with cell treated with hemin only. The experiments were repeated for at least of three times.
Effect of Brazilin on Hemin-Induced Nrf2 Activation in SW480 Cells
To further understanding the inhibitory roles of brazilin on HO-1-related regulation on transcription cascades, we determined whether brazilin inhibits Nrf2 activation in SW480 cells after stimulate with hemin as the HO-1 inducer (Figure 4). Treatments of hemin were markedly increased translocation of Nrf2 into nucleus fraction from cytosol. In contrast, brazilin showed inhibitory effects on translocation of Nrf2 from cytosol to nuclear fraction in a dose-dependent manner. Whereas, hemin-induced decrease of Nrf2 level in cytosol were temporally recovered by 50 μM brazilin but no significant changes shown in other high concentration of brazilin. These results indicate that the nuclear translocation of Nrf2 are a crucial key molecules on inhibition of hemin-induced HO-1 elevation and suggest that brazilin able to induces of apoptosis on colorectal cancer cells.
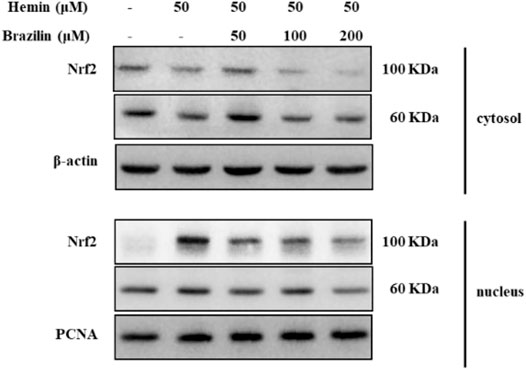
FIGURE 4. Blockage effects brazilin on hemin-mediated Nrf2 translocation into nuclear fraction. Cells were treated with indicated dosages of brazilin in the presence of hemin. After 4 h incubation, fraction extracts of nuclear and cytoplasm were prepared. Nrf2 molecules were identified by Western blotting. PCNA and β-actin were used as loading controls for nuclear- and cytosol-specific marker protein, respectively. The experiments were repeated for at least of three times.
Effect of Brazilin on Regulation of HO-1 Expression and Hemin-Induced Nrf2 Activation in SW480 Cells
To observe the effects of brazilin on hemin-induced HO-1 elevation in SW480 cells, we determined with quantitative real-time PCR (qPCR). The qPCR revealed that treatment of brazilin were markedly reduced mRNA levels of HO-1 in a dose-dependent manner (Figure 5A). Furthermore, to understanding of inhibitory roles of brazilin on regulation of HO-1 transcription, we determined whether brazilin inhibits Nrf2 activation in SW480 cells on hemin-induced HO-1 expression model using EMSA (Figure 5B). DNA binding activity of hemin-induced Nrf2 was markedly inhibited by treatment of brazilin in a dose-dependent manner. These results suggest that Nrf2 nuclear translocation is an pivotal roles on regulation of hemin-induced HO-1 expression and Nrf2 nuclear translocation.
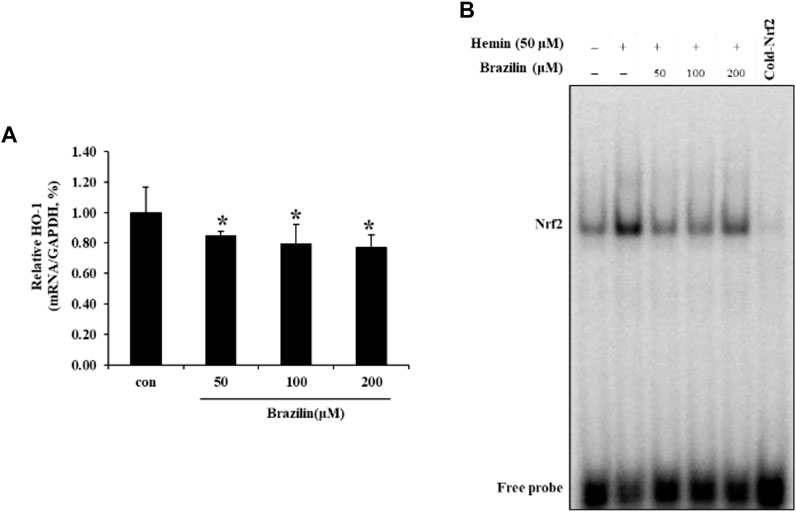
FIGURE 5. Brazilin regulates (A) HO-1 expression and (B) Nrf2 DNA binding activity in SW480 cells. Cells were treated with indicated dosages of brazilin for 1 h, and then further incubated with 50 μM hemin for 24 h. Relative ratio of HO-1 mRNA levels were calculated by qPCR with GAPDH as an internal control. Data are presented as Means ± SD of three independent experiments. * p < 0.05 vs. control group. The experiments were repeated for at least of three times.
Discussion
Colorectal cancer is a major medical burden worldwide. Therefore, many diagnoses and treatment strategies developed and regulated by biomedical approaches have been implemented to solve this problem. Recently, plants extractives have been attracting increasing interests (Si et al., 2009; Liu et al., 2020a; Rui Xu et al., 2020; Liu et al., 2021c; Du et al., 2022), and phytotherapy is thought to be a promising approach to treat diseases (Hu et al., 2014; Lu et al., 2019; Liu et al., 2020b; Liu et al., 2021b). In the present study, the effects of mTOR-mediated signaling on brazilin-induced apoptosis of colorectal cancer cells based on HO-1 expression were investigated. Torin 1 is a potent and selective ATP-competitive inhibitor of mTOR kinase (Thoreen et al., 2009), the catalytic subunit of two functionally distinct complexes (mTORC1 and mTORC2) that promotes cell survival, proliferation, and growth.
Basically, mTOR has various physiological roles including changes in utilizable energy metabolites from dietary energy sources for homeostasis of lives. Whereas these pathways involved to energy storage in fasting condition and/or starvation stages (Saxton and Sabatini, 2017), and regulates downstream pathway of nutrients absorption and utilization, such as sugar and amino acids (Kalender et al., 2010). Therefore, mTOR and its regulation mechanism were important pathway as switch of energy storage and utilization in animals.
Unlike rapamycin, classical mTOR inhibitors, torin 1 was effectively blocked the phosphorylation of mTORC1 and mTORC2 (Liu et al., 2010). Furthermore, Brazilin has been reported to induce apoptosis of various cancer cells (Zhang et al., 2018; Lee et al., 2013), and the present study results showed that brazilin significantly inhibited cell viability and induced apoptosis in SW480 cells. Many natural compounds including brazilin were previously shown to increase HO-1 expression in normal or/and various disease models (Choi and Kim, 2008; Jang et al., 2020; Consoli et al., 2021; Hu et al., 2009); however, effects of brazilin on colorectal cancer cells have not been reported to date. These previous reports have been led to the prediction of useful for cancer mTOR-mediated cancer therapy. In early generation, all rapamycin analogs (CCI-779) (Wyeth, LLC, NJ, United States), AP23573 (Ariad Pharmaceuticals, MA, United States), RAD001 (Novartis International AG, Basel, Switzerland) have been launched (Guertin and Sabatini, 2007; Easton and Houghton, 2006, Faivre et al., 2006, Granville et al., 2006). In late 2010, official clinical trial information site in US (ClinicalTrials.gov, National Cancer Institute, MD, United States) were registered larger than 150 trials with novel mTOR inhibitors to investigation as anticancer therapies for FDA approve (Wander et al., 2011). Recently, mTOR resistance mutant-selective novel mTOR inhibitor (Rodrik-Outmezguine et al., 2016) and the torin family were suggested for cancer therapy (Chen and Zhou, 2020). Whereas, poor information were existed between novel mTOR inhibitor and HO-1 pathway on cancer therapy then mandating more clinical research.
Interestingly, brazilin was reported ameliorative functions on diabetic nephropathy inflammation (Li et al., 2017), antidepressant- and anxiolytic- like effects (Wang et al., 2019), Staphylococcus aureus-induced mastitis (Gao et al., 2015), and renal ischemia-reperfusion injury (Jia et al., 2016) in animal model. However, the animal models for examine the anti-cancer effects of brazilin were poor therefore these results suggest that required novel candidate for screening of drugs with analogues. Moreover, brazilin has not been used for the clinical treatment of disease, then more basic results and evidences of this natural/synthetic molecules (Jung and Kim, 2015; Arredondo et al., 2019) and analogues are urgently needed.
Induction of HO-1 inhibits apoptosis through the Akt pathway (Busserolles et al., 2006). Rapamycin and wortmannin induce HO-1 in HPAEC, HPASMC, and renal cancer cells (Visner et al., 2003; Banerjee et al., 2012). In contrast, in Tsc2-deficient neurons, HO-1 expression is increased, though rapamycin inhibits this increase (Di Nardo et al., 2009). These results indicate that the PI3K/Akt/mTOR signaling pathway is involved in HO-1 expression. Therefore, hypothetically, brazilin regulates the mTOR signaling pathway. In the present study, brazilin decreased mTOR phosphorylation, and an mTOR inhibitor decreased HO-1 expression (Figure 3). Accordingly, the study results showed that mTOR signaling was associated with brazilin-regulated HO-1 expression.
Induction of HO-1 has been shown to promote or inhibit apoptosis in colon cancer cells (Busserolles et al., 2006; Andrés et al., 2014). However, unlike previous studies, HO-1 expression was decreased in brazilin-treated cells in the present study. Furthermore, an HO-1 inducer prevented the reduction of brazilin-mediated cell viability. These results indicate that reduction of HO-1 expression mediates brazilin-induced inhibition of cell viability and apoptosis.
Recently, new-drug discovery and combinative chemotherapy using (nano)carriers and natural compounds are being investigated as new approaches and opportunities for cancer and other disorders treatment (Li et al., 2019; Jiayun Xu et al., 2020; Liu et al., 2022). In theory and practice fields, development of new biochemical/biomedical tools for personalized medicine in cancer treatment is unlimited (Du et al., 2019; Li et al., 2020; Liu et al., 2021a; Rui Xu et al., 2021). Therefore, personalized medicine important in effective therapy with low cost. By discovering the diverse potential of biomedicine, better healthcare tools can be provided based on the present study results.
Conclusion
In the present study, brazilin downregulated HO-1 expression, which can lead to cell death. In addition, brazilin regulated HO-1 expression via reduction of mTOR phosphorylation, Nrf2 nuclear translocation in colorectal cancer cells. mTOR plays a crucial role in cancer biology and has emerged as a potential target for drug development. Therefore, brazilin is a potential therapeutic agent for treatment of colorectal cancer.
Data Availability Statement
The original contributions presented in the study are included in the article, further inquiries can be directed to the corresponding author.
Ethics Statement
Ethical review and approval was not required for the study of human participants in accordance with the local legislation and institutional requirements. Written informed consent from the patients was not required to participate in this study in accordance with the national legislation and the institutional requirements.
Author Contributions
Investigation, O-YH and EC; Supervision, K-HP and J-SK; Writing-original draft, K-HP; Writing-review and editing, K-HP.
Funding
This work was supported by a National Research Foundation of Korea (NRF) grant funded by the Korean government (MSIT) (Nos 2019R1A2C1003454 and 2021R1A2C1091322) and by the Basic Science Research Program through the National Research Foundation of Korea (NRF) funded by the Ministry of Education (NRF-2020R1I1A1A01054100). This research was conducted with the support of BK21FOUR 21st century of Medical Science Creative Human Resource Development Center.
Conflict of Interest
The authors declare that the research was conducted in the absence of any commercial or financial relationships that could be construed as a potential conflict of interest.
Publisher’s Note
All claims expressed in this article are solely those of the authors and do not necessarily represent those of their affiliated organizations, or those of the publisher, the editors and the reviewers. Any product that may be evaluated in this article, or claim that may be made by its manufacturer, is not guaranteed or endorsed by the publisher.
References
Andrés, N. C., Fermento, M. E., Gandini, N. A., Romero, A. L., Ferro, A., Donna, L. G., et al. (2014). Heme Oxygenase-1 Has Antitumoral Effects in Colorectal Cancer: Involvement of P53. Exp. Mol. Pathol. 97, 321–331. doi:10.1016/j.yexmp.2014.09.012
Arredondo, V., Roa, D. E., Gutman, E. S., Huynh, N. O., and Van Vranken, D. L. (2019). Total Synthesis of (±)-Brazilin Using [4 + 1] Palladium-Catalyzed Carbenylative Annulation. J. Org. Chem. 84, 14745–14759. doi:10.1021/acs.joc.9b02343
Bae, I.-K., Min, H.-Y., Han, A.-R., Seo, E.-K., and Lee, S. K. (2005). Suppression of Lipopolysaccharide-Induced Expression of Inducible Nitric Oxide Synthase by Brazilin in RAW 264.7 Macrophage Cells. Eur. J. Pharmacol. 513, 237–242. doi:10.1016/j.ejphar.2005.03.011
Banerjee, P., Basu, A., Wegiel, B., Otterbein, L. E., Mizumura, K., Gasser, M., et al. (2012). Heme Oxygenase-1 Promotes Survival of Renal Cancer Cells through Modulation of Apoptosis- and Autophagy-Regulating Molecules. J. Biol. Chem. 287, 32113–32123. doi:10.1074/jbc.m112.393140
Becker, J. C., Fukui, H., Imai, Y., Sekikawa, A., Kimura, T., Yamagishi, H., et al. (2007). Colonic Expression of Heme Oxygenase-1 Is Associated with a Better Long-Term Survival in Patients with Colorectal Cancer. Scand. J. Gastroenterol. 42, 852–858. doi:10.1080/00365520701192383
Brouard, S., Otterbein, L. E., Anrather, J., Tobiasch, E., Bach, F. H., Choi, A. M. K., et al. (2000). Carbon Monoxide Generated by Heme Oxygenase 1 Suppresses Endothelial Cell Apoptosis. J. Exp. Med. 192, 1015–1026. doi:10.1084/jem.192.7.1015
Busserolles, J., Megías, J., Terencio, M. C., and Alcaraz, M. J. (2006). Heme Oxygenase-1 Inhibits Apoptosis in Caco-2 Cells via Activation of Akt Pathway. Int. J. Biochem. Cel Biol. 38, 1510–1517. doi:10.1016/j.biocel.2006.03.013
Chen, Y., and Zhou, X. (2020). Research Progress of mTOR Inhibitors. Eur. J. Med. Chem. 208, 112820. doi:10.1016/j.ejmech.2020.112820
Choi, B.-M., and Kim, B.-R. (2008). Upregulation of Heme Oxygenase-1 by Brazilin via the Phosphatidylinositol 3-kinase/Akt and ERK Pathways and its Protective Effect against Oxidative Injury. Eur. J. Pharmacol. 580, 12–18. doi:10.1016/j.ejphar.2007.10.044
Choi, B.-M., Pae, H.-O., Jeong, Y.-R., Oh, G.-S., Jun, C.-D., Kim, B.-R., et al. (2004). Overexpression of Heme Oxygenase (HO)-1 Renders Jurkat T Cells Resistant to Fas-Mediated Apoptosis: Involvement of Iron Released by HO-1. Free Radic. Biol. Med. 36, 858–871. doi:10.1016/j.freeradbiomed.2004.01.004
Choi, S.-Y., and Moon, C.-K. (1997). Effects of Brazilin on the Altered Immune Functions in the Early Phase of Halothane Intoxication of C57BL/6 Mice. Planta Med. 63, 400–404. doi:10.1055/s-2006-957721
Clark, J. E., Foresti, R., Green, C. J., and Motterlini, R. (2000). Dynamics of Haem Oxygenase-1 Expression and Bilirubin Production in Cellular protection against Oxidative Stress. Biochem. J. 348, 615–619. doi:10.1042/bj3480615
Consoli, V., Sorrenti, V., Grosso, S., and Vanella, L. (2021). Heme Oxygenase-1 Signaling and Redox Homeostasis in Physiopathological Conditions. Biomolecules 11, 589. doi:10.3390/biom11040589
Degese, M. S., Mendizabal, J. E., Gandini, N. A., Gutkind, J. S., Molinolo, A., Hewitt, S. M., et al. (2012). Expression of Heme Oxygenase-1 in Non-small Cell Lung Cancer (NSCLC) and its Correlation with Clinical Data. Lung Cancer 77, 168–175. doi:10.1016/j.lungcan.2012.02.016
Deng, Y.-M., Wu, B. J., Witting, P. K., and Stocker, R. (2004). Probucol Protects against Smooth Muscle Cell Proliferation by Upregulating Heme Oxygenase-1. Circulation 110, 1855–1860. doi:10.1161/01.cir.0000142610.10530.25
Di Nardo, A., Kramvis, I., Cho, N., Sadowski, A., Meikle, L., Kwiatkowski, D. J., et al. (2009). Tuberous Sclerosis Complex Activity Is Required to Control Neuronal Stress Responses in an mTOR-dependent Manner. J. Neurosci. 29, 5926–5937. doi:10.1523/jneurosci.0778-09.2009
Du, H., Liu, W., Zhang, M., Si, C., Zhang, X., and Li, B. (2019). Cellulose Nanocrystals and Cellulose Nanofibrils Based Hydrogels for Biomedical Applications. Carbohydr. Polym. 209, 130–144. doi:10.1016/j.carbpol.2019.01.020
Du, H., Zhang, M., Liu, K., Parit, M., Jiang, Z., Zhang, X., et al. (2022). Conductive PEDOT:PSS/cellulose Nanofibril Paper Electrodes for Flexible Supercapacitors with superior Areal Capacitance and Cycling Stability. Chem. Eng. J. 428, 131994. doi:10.1016/j.cej.2021.131994
Easton, J. B., and Houghton, P. J. (2006). mTOR and Cancer Therapy. Oncogene 25, 6436–6446. doi:10.1038/sj.onc.1209886
Faivre, S., Kroemer, G., and Raymond, E. (2006). Current Development of mTOR Inhibitors as Anticancer Agents. Nat. Rev. Drug Discov. 5, 671–688. doi:10.1038/nrd2062
Florczyk, U. M., Jozkowicz, A., and Dulak, J. (2008). Biliverdin Reductase: New Features of an Old Enzyme and its Potential Therapeutic Significance. Pharmacol. Rep. 60 (1), 38–48.
Gandini, N. A., Fermento, M. E., Salomón, D. G., Blasco, J., Patel, V., Gutkind, J. S., et al. (2012). Nuclear Localization of Heme Oxygenase-1 Is Associated with Tumor Progression of Head and Neck Squamous Cell Carcinomas. Exp. Mol. Pathol. 93, 237–245. doi:10.1016/j.yexmp.2012.05.001
Gao, X.-j., Wang, T.-c., Zhang, Z.-c., Cao, Y.-g., Zhang, N.-s., and Guo, M.-y. (2015). Brazilin Plays an Anti-inflammatory Role with Regulating Toll-like Receptor 2 and TLR 2 Downstream Pathways in Staphylococcus Aureus-Induced Mastitis in Mice. Int. Immunopharmacology 27, 130–137. doi:10.1016/j.intimp.2015.04.043
Granville, C. A., Memmott, R. M., Gills, J. J., and Dennis, P. A. (2006). Handicapping the Race to Develop Inhibitors of the Phosphoinositide 3-Kinase/Akt/Mammalian Target of Rapamycin Pathway. Clin. Cancer Res. 12, 679–689. doi:10.1158/1078-0432.ccr-05-1654
Guertin, D. A., and Sabatini, D. M. (2007). Defining the Role of mTOR in Cancer. Cancer Cell 12, 9–22. doi:10.1016/j.ccr.2007.05.008
Hellman, L. M., and Fried, M. G. (2007). Electrophoretic Mobility Shift Assay (EMSA) for Detecting Protein-Nucleic Acid Interactions. Nat. Protoc. 2, 1849–1861. doi:10.1038/nprot.2007.249
Hikino, H., Taguchi, T., Fujimura, H., and Hiramatsu, Y. (1977). Antiinflammatory Principles Ofcaesalpinia Sappanwood and Ofhaematoxylon Campechianumwood1. Planta Med. 31, 214–220. doi:10.1055/s-0028-1097516
Hu, C. M., Kang, J. J., Lee, C. C., Li, C. H., Liao, J. W., and Cheng, Y. W. (2003). Induction of Vasorelaxation through Activation of Nitric Oxide Synthase in Endothelial Cells by Brazilin. Eur. J. Pharmacol. 468, 37–45. doi:10.1016/s0014-2999(03)01639-x
Hu, C.-M., Liu, Y.-H., Cheah, K.-P., Li, J.-S., Lam, C.-S. K., Yu, W.-Y., et al. (2009). Heme Oxygenase-1 Mediates the Inhibitory Actions of Brazilin in RAW264.7 Macrophages Stimulated with Lipopolysaccharide. J. Ethnopharmacology 121, 79–85. doi:10.1016/j.jep.2008.09.030
Hu, W., Wang, G., Li, P., Wang, Y., Si, C.-L., He, J., et al. (2014). Neuroprotective Effects of Macranthoin G from Eucommia Ulmoides against Hydrogen Peroxide-Induced Apoptosis in PC12 Cells via Inhibiting NF-Κb Activation. Chemico-Biological Interactions 224, 108–116. doi:10.1016/j.cbi.2014.10.011
Hu, W., Wang, X., Wu, L., Shen, T., Ji, L., Zhao, X., et al. (2016). Apigenin-7-O-β-d-glucuronide Inhibits LPS-Induced Inflammation through the Inactivation of AP-1 and MAPK Signaling Pathways in RAW 264.7 Macrophages and Protects Mice against Endotoxin Shock. Food Funct. 7 (2), 1002–1013. doi:10.1039/C5FO01212K
Huayu Liu, H., Du, H., Zheng, T., Liu, K., Ji, X., Xu, T., et al. (2021a). Cellulose Based Composite Foams and Aerogels for Advanced Energy Storage Devices. Chem. Eng. J. 426, 130817. doi:10.1016/j.cej.2021.130817
Huayu Liu, H., Xu, T., Liu, K., Zhang, M., Liu, W., Li, H., et al. (2021b). Lignin-based Electrodes for Energy Storage Application. Ind. Crops Prod. 165, 113425. doi:10.1016/j.indcrop.2021.113425
Hwang, G.-S., Kim, J.-Y., Chang, T.-S., Jeon, S.-D., So, D.-S., and Moon, C.-K. (1998). Effects of Brazilin on the Phospholipase A2 Activity and Changes of Intracellular Free Calcium Concentration in Rat Platelets. Arch. Pharm. Res. 21, 774–778. doi:10.1007/bf02976775
Jacinto, E., and Hall, M. N. (2003). TOR Signalling in Bugs, Brain and Brawn. Nat. Rev. Mol. Cel Biol 4, 117–126. doi:10.1038/nrm1018
Jacinto, E., Loewith, R., Schmidt, A., Lin, S., Rüegg, M. A., Hall, A., et al. (2004). Mammalian TOR Complex 2 Controls the Actin Cytoskeleton and Is Rapamycin Insensitive. Nat. Cel Biol 6, 1122–1128. doi:10.1038/ncb1183
Jang, H.-Y., Hong, O.-Y., Chung, E.-Y., Park, K.-H., and Kim, J.-S. (2020). Roles of JNK/Nrf2 Pathway on Hemin-Induced Heme Oxygenase-1 Activation in MCF-7 Human Breast Cancer Cells. Medicina 56, 268. doi:10.3390/medicina56060268
Jia, Y., Zhao, J., Liu, M., Li, B., Song, Y., Li, Y., et al. (2016). Brazilin Exerts Protective Effects against Renal Ischemia-Reperfusion Injury by Inhibiting the NF-Κb Signaling Pathway. Int. J. Mol. Med. 38, 210–216. doi:10.3892/ijmm.2016.2616
Jiayun Xu, J., Li, C., Dai, L., Xu, C., Zhong, Y., Yu, F., et al. (2020). Biomass Fractionation and Lignin Fractionation towards Lignin Valorization. Chemsuschem 13 (17), 4284–4295. doi:10.1002/cssc.202001491
Jung, Y., and Kim, I. (2015). Total Synthesis of Brazilin. J. Org. Chem. 80, 2001–2005. doi:10.1021/jo502745j
Kalender, A., Selvaraj, A., Kim, S. Y., Gulati, P., Brûlé, S., Viollet, B., et al. (2010). Metformin, Independent of AMPK, Inhibits mTORC1 in a Rag GTPase-dependent Manner. Cel Metab. 11, 390–401. doi:10.1016/j.cmet.2010.03.014
Kang, K. A., Maeng, Y. H., Zhang, R., Yang, Y. R., Piao, M. J., Kim, K. C., et al. (2012). Involvement of Heme Oxygenase-1 in Korean colon Cancer. Tumor Biol. 33, 1031–1038. doi:10.1007/s13277-012-0336-0
Kapturczak, M. H., Wasserfall, C., Brusko, T., Campbell-Thompson, M., Ellis, T. M., Atkinson, M. A., et al. (2004). Heme Oxygenase-1 Modulates Early Inflammatory Responses. Am. J. Pathol. 165, 1045–1053. doi:10.1016/s0002-9440(10)63365-2
Kim, S.-G., Kim, Y.-M., Khil, L.-Y., Jeon, S.-D., So, D.-S., Moon, C.-H., et al. (1998). Brazilin Inhibits Activities of Protein Kinase C and Insulin Receptor Serine Kinase in Rat Liver. Arch. Pharm. Res. 21, 140–146. doi:10.1007/bf02974018
Liu, K., Du, H., Liu, W., Liu, H., Zhang, M., Xu, T., et al. (2021a). Cellulose Nanomaterials for Oil Exploration Applications. Polym. Rev., in press. 1–41. doi:10.1080/15583724.2021.2007121
Liu, K., Du, H., Zheng, T., Liu, H., Zhang, M., Zhang, R., et al. (2021b). Recent Advances in Cellulose and its Derivatives for Oilfield Applications. Carbohydr. Polym. 259, 117740. doi:10.1016/j.carbpol.2021.117740
Liu, K., Du, H., Zheng, T., Liu, W., Zhang, M., Liu, H., et al. (2021c). Lignin-containing Cellulose Nanomaterials: Preparation and Applications. Green. Chem. 23, 9723–9746. doi:10.1039/D1GC02841C
Lee, P. J., Alam, J., Wiegand, G. W., and Choi, A. M. (1996). Overexpression of Heme Oxygenase-1 in Human Pulmonary Epithelial Cells Results in Cell Growth Arrest and Increased Resistance to Hyperoxia. Proc. Natl. Acad. Sci. 93, 10393–10398. doi:10.1073/pnas.93.19.10393
Lee, D.-Y., Lee, M.-K., Kim, G.-S., Noh, H.-J., and Lee, M.-H. (2013). Brazilin Inhibits Growth and Induces Apoptosis in Human Glioblastoma Cells. Molecules 18, 2449–2457. doi:10.3390/molecules18022449
Li, Z.-y., Zheng, Y., Chen, Y., Pan, M., Zheng, S.-b., Huang, W., et al. (2017). Brazilin Ameliorates Diabetic Nephropathy and Inflammation in Db/db Mice. Inflammation 40, 1365–1374. doi:10.1007/s10753-017-0579-4
Li, X., Xu, R., Yang, J., Nie, S., Liu, D., Liu, Y., et al. (2019). Production of 5-hydroxymethylfurfural and Levulinic Acid from Lignocellulosic Biomass and Catalytic Upgradation. Ind. Crops Prod. 130, 184–197. doi:10.1016/j.indcrop.2018.12.082
Li, X., Lu, X., Nie, S., Liang, M., Yu, Z., Duan, B., et al. (2020). Efficient Catalytic Production of Biomass-Derived Levulinic Acid over Phosphotungstic Acid in Deep Eutectic Solvent. Ind. Crops Prod. 145, 112154. doi:10.1016/j.indcrop.2020.112154
Liu, Q., Chang, J. W., Wang, J., Kang, S. A., Thoreen, C. C., Markhard, A., et al. (2010). Discovery of 1-(4-(4-Propionylpiperazin-1-Yl)-3-(trifluoromethyl)phenyl)-9-(quinolin-3-Yl)benzo[h][1,6]naphthyridin-2(1h)-One as a Highly Potent, Selective Mammalian Target of Rapamycin (mTOR) Inhibitor for the Treatment of Cancer. J. Med. Chem. 53, 7146–7155. doi:10.1021/jm101144f
Liu, W., Du, H., Liu, H., Xie, H., Xu, T., Zhao, X., et al. (2020a). Highly Efficient and Sustainable Preparation of Carboxylic and Thermostable Cellulose Nanocrystals via FeCl3-Catalyzed Innocuous Citric Acid Hydrolysis. ACS Sust. Chem. Eng. 8, 16691–16700. doi:10.1021/acssuschemeng.0c06561
Liu, W., Du, H., Zhang, M., Liu, K., Liu, H., Xie, H., et al. (2020b). Bacterial Cellulose-Based Composite Scaffolds for Biomedical Applications: a Review. ACS Sust. Chem. Eng. 8, 7536–7562. doi:10.1021/acssuschemeng.0c00125
Liu, W., Du, H., Liu, K., Liu, H., Xie, H., Si, C., et al. (2021). Sustainable Preparation of Cellulose Nanofibrils via Choline Chloride-Citric Acid Deep Eutectic Solvent Pretreatment Combined with High-Pressure Homogenization. Carbohydr. Polym. 267, 118220. doi:10.1016/j.carbpol.2021.118220
Liu, H., Xu, T., Cai, C., Liu, K., Liu, W., Zhang, M., et al. (2022). Multifunctional Superelastic, Superhydrophilic, and Ultralight Nanocellulose-Based Composite Carbon Aerogels for Compressive Supercapacitor and Strain Sensor. Adv. Funct. Mater. 32, 2113082. doi:10.1002/adfm.202113082
Lu, J., Zhu, W., Dai, L., Si, C., and Ni, Y. (2019). Fabrication of Thermo- and pH-Sensitive Cellulose Nanofibrils-Reinforced Hydrogel with Biomass Nanoparticles. Carbohydr. Polym. 215, 289–295. doi:10.1016/j.carbpol.2019.03.100
Maines, M. D., and Abrahamsson, P.-A. (1996). Expression of Heme Oxygenase-1 (HSP32) in Human Prostate: normal, Hyperplastic, and Tumor Tissue Distribution. Urology 47, 727–733. doi:10.1016/s0090-4295(96)00010-6
Maines, M. D. (1997). The Heme Oxygenase System:A Regulator of Second Messenger Gases. Annu. Rev. Pharmacol. Toxicol. 37, 517–554. doi:10.1146/annurev.pharmtox.37.1.517
Mok, M.-S., Jeon, S.-D., Yang, K.-M., So, D.-S., and Moon, C.-K. (1998). Effects of Brazilin on Induction of Immunological Tolerance by Sheep Red Blood Cells in C57BL/6 Female Mice. Arch. Pharm. Res. 21, 769–773. doi:10.1007/bf02976774
Moon, C.-K., Park, K.-S., Kim, S.-G., Won, H.-S., and Chung, J.-H. (1992). BRAZILIN PROTECTS CULTURED RAT HEPATOCYTES FROM BrCCI3-INDUCED TOXICITY. Drug Chem. Toxicol. 15, 81–91. doi:10.3109/01480549209035174
Peyton, K. J., Reyna, S. V., Chapman, G. B., Ensenat, D., Liu, X.-m., Wang, H., et al. (2002). Heme Oxygenase-1-Derived Carbon Monoxide Is an Autocrine Inhibitor of Vascular Smooth Muscle Cell Growth. Blood 99, 4443–4448. doi:10.1182/blood.v99.12.4443
Puchtler, H., Meloan, S. N., and Waldrop, F. S. (1986). Application of Current Chemical Concepts to Metal-Hematein And-Brazilein Stains. Histochemistry 85, 353–364. doi:10.1007/bf00982665
Puchtler, H., and Sweat, F. (1964). On the Mechanism of Sequence Iron-Hematein Stains. Histochemie 4, 197–208. doi:10.1007/bf00290864
Rodrik-Outmezguine, V. S., Okaniwa, M., Yao, Z., Novotny, C. J., McWhirter, C., Banaji, A., et al. (2016). Overcoming mTOR Resistance Mutations with a New-Generation mTOR Inhibitor. Nature 534, 272–276. doi:10.1038/nature17963
Rui Xu, R., Liu, K., Du, H., Liu, H., Cao, X., Zhao, X., et al. (2020). Falling Leaves Return to Their Roots: A Review on the Preparation of γ‐Valerolactone from Lignocellulose and its Application in the Conversion of Lignocellulose. ChemSusChem 13, 6461–6476. doi:10.1002/cssc.202002008
Rui Xu, R., Du, H., Liu, C., Liu, H., Wu, M., Zhang, X., et al. (2021). An Efficient and Magnetic Adsorbent Prepared in a Dry Process with Enzymatic Hydrolysis Residues for Wastewater Treatment. J. Clean. Prod. 313, 127834. doi:10.1016/j.jclepro.2021.127834
Sabatini, D. M., Erdjument-Bromage, H., Lui, M., Tempst, P., and Snyder, S. H. (1994). RAFT1: A Mammalian Protein that Binds to FKBP12 in a Rapamycin-dependent Fashion and Is Homologous to Yeast TORs. Cell 78, 35–43. doi:10.1016/0092-8674(94)90570-3
Sarbassov, D. D., Ali, S. M., Kim, D.-H., Guertin, D. A., Latek, R. R., Erdjument-Bromage, H., et al. (2004). Rictor, a Novel Binding Partner of mTOR, Defines a Rapamycin-Insensitive and Raptor-independent Pathway that Regulates the Cytoskeleton. Curr. Biol. 14, 1296–1302. doi:10.1016/j.cub.2004.06.054
Sarbassov, D. D., Ali, S. M., and Sabatini, D. M. (2005). Growing Roles for the mTOR Pathway. Curr. Opin. Cel Biol. 17, 596–603. doi:10.1016/j.ceb.2005.09.009
Sasaki, Y., Hosokawa, T., Nagai, M., and Nagumo, S. (2007). In Vitro Study for Inhibition of NO Production about Constituents of Sappan Lignum. Biol. Pharm. Bull. 30, 193–196. doi:10.1248/bpb.30.193
Saxton, R. A., and Sabatini, D. M. (2017). mTOR Signaling in Growth, Metabolism, and Disease. Cell 168, 960–976. doi:10.1016/j.cell.2017.02.004
Si, C.-L., Liu, Z., Kim, J.-K., and Bae, Y.-S. (2008). Structure Elucidation of Phenylethanoid Glycosides from Paulownia Tomentosa Steud. Var. Tomentosa wood. Holzforschung 62, 197–200. doi:10.1515/hf.2008.047
Si, C.-L., Kim, J.-K., Bae, Y.-S., and Li, S.-M. (2009). Phenolic Compounds in the Leaves ofPopulus Ussuriensisand Their Antioxidant Activities. Planta Med. 75, 1165–1167. doi:10.1055/s-0029-1185476
Si, C.-L., Jiang, J.-Z., Liu, S.-C., Hu, H.-Y., Ren, X.-D., Yu, G.-J., et al. (2013a). A New Lignan Glycoside and Phenolics from the branch wood of Pinus Banksiana Lambert. Holzforschung 67, 357–363. doi:10.1515/hf-2012-0137
Si, C.-L., Shen, T., Jiang, Y.-Y., Wu, L., Yu, G.-J., Ren, X.-D., et al. (2013b). Antioxidant Properties and Neuroprotective Effects of Isocampneoside II on Hydrogen Peroxide-Induced Oxidative Injury in PC12 Cells. Food Chem. Toxicol. 59, 145–152. doi:10.1016/j.fct.2013.05.051
Thoreen, C. C., Kang, S. A., Chang, J. W., Liu, Q., Zhang, J., Gao, Y., et al. (2009). An ATP-Competitive Mammalian Target of Rapamycin Inhibitor Reveals Rapamycin-Resistant Functions of mTORC1. J. Biol. Chem. 284, 8023–8032. doi:10.1074/jbc.m900301200
Ting Xu, T., Du, H., Liu, H., Liu, W., Zhang, X., Si, C., et al. (2021). Advanced Nanocellulose‐Based Composites for Flexible Functional Energy Storage Devices. Adv. Mater. 33, 2101368. doi:10.1002/adma.202101368
Tsai, J.-R., Wang, H.-M., Liu, P.-L., Chen, Y.-H., Yang, M.-C., Chou, S.-H., et al. (2012). High Expression of Heme Oxygenase-1 Is Associated with Tumor Invasiveness and Poor Clinical Outcome in Non-small Cell Lung Cancer Patients. Cell Oncol. 35, 461–471. doi:10.1007/s13402-012-0105-5
Visner, G. A., Lu, F., Zhou, H., Liu, J., Kazemfar, K., and Agarwal, A. (2003). Rapamycin Induces Heme Oxygenase-1 in Human Pulmonary Vascular Cells. Circulation 107, 911–916. doi:10.1161/01.cir.0000048191.75585.60
Wander, S. A., Hennessy, B. T., and Slingerland, J. M. (2011). Next-generation mTOR Inhibitors in Clinical Oncology: How Pathway Complexity Informs Therapeutic Strategy. J. Clin. Invest. 121, 1231–1241. doi:10.1172/jci44145
Wang, X., Xiu, Z., Du, Y., Li, Y., Yang, J., Gao, Y., et al. (2019). Brazilin Treatment Produces Antidepressant- and Anxiolytic-like Effects in Mice. Biol. Pharm. Bull. 42, 1268–1274. doi:10.1248/bpb.b18-00882
Wang, H., Xie, H., Du, H., Wang, X., Liu, W., Duan, Y., et al. (2020). Highly Efficient Preparation of Functional and Thermostable Cellulose Nanocrystals via H2SO4 Intensified Acetic Acid Hydrolysis. Carbohydr. Polym. 239, 116233. doi:10.1016/j.carbpol.2020.116233
Wang, H., Du, H., Liu, K., Liu, H., Xu, T., Zhang, S., et al. (2021). Sustainable Preparation of Bifunctional Cellulose Nanocrystals via Mixed H2SO4/formic Acid Hydrolysis. Carbohydr. Polym. 266, 118107. doi:10.1016/j.carbpol.2021.118107
Willis, D., Moore, A. R., Frederick, R., and Willoughby, D. A. (1996). Heme Oxygenase: A Novel Target for the Modulation of Inflammatory Response. Nat. Med. 2, 87–90. doi:10.1038/nm0196-87
Yin, H., Fang, J., Liao, L., Maeda, H., and Su, Q. (2014). Upregulation of Heme Oxygenase-1 in Colorectal Cancer Patients with Increased Circulation Carbon Monoxide Levels, Potentially Affects Chemotherapeutic Sensitivity. BMC Cancer 14, 436. doi:10.1186/1471-2407-14-436
Zhang, T., He, J., Zhang, S., Wang, G., Ma, E., Wang, J., et al. (2018). Brazilin Induces T24 Cell Death through C-Fos and GADD45β Independently Regulated Genes and Pathways. IUBMB Life 70, 1101–1110. doi:10.1002/iub.1921
Keywords: mammalian target of rapamycin (mTOR), mTOR kinase inhibitor, mTOR phosphorylation, torin1, brazilin, heme oxygenase-1, colorectal cancer
Citation: Hong O-, Cho E, Kim J- and Park K- (2022) Brazilin From Caesalpinia sappan L. Induced Apoptosis via mTOR and HO-1 Pathway in SW480 Human Colon Cancer Cells. Front. Energy Res. 10:860627. doi: 10.3389/fenrg.2022.860627
Received: 23 January 2022; Accepted: 28 February 2022;
Published: 08 April 2022.
Edited by:
Shuangxi Nie, Guangxi University, ChinaReviewed by:
Chang-Gu Hyun, Jeju National University, South KoreaGuang-Hua Xie, Yanbian University Hospital, China
Copyright © 2022 Hong, Cho, Kim and Park. This is an open-access article distributed under the terms of the Creative Commons Attribution License (CC BY). The use, distribution or reproduction in other forums is permitted, provided the original author(s) and the copyright owner(s) are credited and that the original publication in this journal is cited, in accordance with accepted academic practice. No use, distribution or reproduction is permitted which does not comply with these terms.
*Correspondence: Kwang-Hyun Park, a2hwYXJrQGpibnUuYWMua3I=