- University of Hassan II of Casablanca, Faculty of Sciences and Technology, Laboratory of Materials, Membranes and Environment, Mohammedia, Morocco
Sustainable energy development is a major challenge, particularly when considering population increase, rising energy demands resulting from the excessive use of nonrenewable fossil fuels, and growing consequences on global pollution and climate change. It is critical to continue to develop new sources of renewable and clean energy. Direct alcohol fuel cell (DAFC) represents a technological approach that meets all the requirements in terms of energy conversion with good electrical efficiency and lower emissions of pollutants. However, it largely suffers from the poisoning of the anode catalyst caused by the accumulation of intermediate products. In this context, this review is devoted to discussing the recent progress in anode catalysts based on conducting polymers (CPs) materials for direct alcohol fuel cells, especially direct methanol and ethanol fuel cells, from the viewpoint of synthesis techniques, morphology, and catalytic performance. In the first part of this review, we will explore the most commonly used methods for preparing metal-conducting polymer-based nanocomposites, including the key factors influencing their morphology regardless of the application. The catalytic performances of mono-, bi-, and tri-metal catalysts deposited on conducting polymer-based supports for the electrooxidation of methanol and ethanol reactions are examined in detail in the second and third parts of this review. We also highlight the effect of the introduction of carbon nanomaterials into conducting polymer supports on the electrical properties and on the performance of the catalysts. Development of anode materials using nanostructured conducting polymers-based support plays a vital role in reducing catalyst loadings and should therefore shed light on the wide-scale commercialization of DAFCs. In a nutshell, this review will give readers a thorough understanding of the principles of direct alcohol fuel cells, new advancements in electrode materials based on non-precious metals, and the main challenges that should be dealt with.
Introduction
Nowadays, fossil fuel is being exhausted and the environmental problem is getting more and more severe with the pollutants that it produces (Corti and Gonzalez, 2014). Consequently, finding alternative fuels as power sources and electrical energy regenerators has become researcher’s most enthralling objective. Direct alcohol fuel cells (DAFCs) demonstrate a technological approach that has all demands for stable energy conversion with excellent electrical efficiency, lower emissions of pollutants, and the probability of simultaneous generation of heat and energy (Akbarfallahi et al., 2020; Mozafari and Basiri Parsa, 2020). Indeed, DAFCs have been attracting great attention because of their efficiency to convert chemical energy into electrical energy as well as numerous features such as environmental friendliness, high power density, independent of any electrical charging and noiseless manipulation (Lamy et al., 2002; Corti and Gonzalez, 2014; Pinto et al., 2018; Karim and Kamarudin, 2021). However, there are still challenges presented by DAFCs that need to be handled. The most problematic issues that hinder DAFCs large commercialization can stem from complications such as high cost, alcohol crossover through the proton exchange membrane, and maintaining long-term stability and durability in operation (Chu and Shul, 2010; Kamarudin et al., 2013; Zakaria et al., 2016). Alcohols, such as methanol and ethanol, are widely known as sources of fuel for DAFCs. Direct Methanol Fuel Cell (DMFC) is the most popular alcohol fuel cell owing to methanol excellent properties, high energy density, consistently available, biodegradable, and cheapness. In addition, it can be easily handled, transported, and stored (Chu and Shul, 2010). Methanol oxidation reaction on Pt catalyst has been widely studied in the literature, and a simplified description of methanol oxidation pathways is reported in Figure 1 (Ozoemena, 2016; Sarmoor et al., 2018). Methanol can be either totally oxidized to carbon dioxide or partially oxidized to intermediates such as formaldehyde, formic acid, or carbon monoxide. However, its toxicity is the main disadvantage that hinders its utilization, so precautions must be considered while handling it (Kamarudin et al., 2007; Ahmed and Dincer, 2011).
Despite its high energy density, methanol’s toxicity motivates researchers to drive new studies on other similar alcohols such as Ethanol, which is known to be more renewable since it can be easily provided using sugar-containing raw materials from biomass or agriculture products. Indeed, ethanol seems to be a promising fuel specifically for its non-toxicity. However, it is very important to consider that the ethanol oxidation is a complex reaction, involving the cleavage of C-C bond, which causes an incomplete ethanol oxidation reaction (EOR) compared with MOR (Monyoncho et al., 2016; Xu et al., 2017). In fact, the main issues encountered arise from the fact that the anode catalyst suffers from poisoning due to the accumulation of intermediate (acetyldehyde and acetic acid) products resulting from the incomplete alcohol oxidation reaction (Marinkovic et al., 2019). Many mechanisms have been proposed for ethanol oxidation, but the most widely accepted reaction mechanism follows two major pathways called C1 and C2 (Monyoncho et al., 2016). In both cases, the ethanol (C2H5OH) is the first adsorbed species on the electrocatalyst surface to form an adsorbed intermediate (CxHyOHad) (Lai and Koper, 2009). The first reaction pathway (C1) assumes the direct oxidation of the adsorbed intermediate to COad, which is then oxidized to the final product CO2.
C2H5OH + 12 OH−→2 CO2 + 9 H2O + 12 e− (Alkaline medium).
C2H5OH + 3 H2O→2 CO2 + 12 H+ + 12 e− (Acidic medium).
The second reaction pathway (C2) produces acetic acid (CH3COOH) and acetaldehyde (CH3CHO) as final products (Wang et al., 2015). It should be noted that acetic acid and acetaldehyde cannot be further oxidized into CO2 (Vigier et al., 2004; Raskó et al., 2006; Fang et al., 2010; Christensen et al., 2012; Yang et al., 2014).
C2H5OH + 4 OH−→CH3COOH + 4e− + 3H2O (Alkiline medium).
C2H5OH + H2O→CH3COOH + 4e− + 4 H+ (Acidic medium).
Platinum (Pt) well known as the most used catalyst for alcohol oxidation presents the disadvantages of being costly, rare, and easily deactivated by the carbon monoxide liberated during the oxidation reaction of alcohol (Lai and Koper, 2009). This lowers the performance and the operational time, which increases the complexity of DAFCs limiting their long-term stability and durability. To address these issues, as well as to increase the AOR efficiency, many studies have been put forward to develop nanostructured materials. Therefore, many strategies have been carried out to synthesize low-cost catalysts with suitable functionality to enhance the catalytic performance of direct alcohol fuel cells.
1. The deposition of two metals or three metals on the surface of the electrode.
2. The use of conducting polymers as support prior to metal nanoparticles deposition.
3. The use of carbon nanostructures combined with conducting polymers as electrode substrates.
4. The combination of the three elements, i.e., metal/polymers/CNM.
Therefore, recent investigations are being carried out toward synthesizing catalysts containing reduced amounts of platinum by combining it with other cheaper metals (Huang et al., 2011; Zhang et al., 2011, Zhang et al., 2016 M.; Pushkarev et al., 2019; Caballero-Manrique et al., 2020; Menshchikov et al., 2020). These combinations enhance the performance of the synthesized catalyst in oxidizing alcohol, resulting in a significant improvement in DAFC efficiency. Indeed, the bifunctional mechanism of bimetallic alloys such as Ni, Cu, Co leads to a direct activity promotion by providing hydroxyl groups at potentials more negative compared with pure Pt which thereby enhances the oxidative removal of CO during the AOR (Park et al., 2002; Xu et al., 2004; Wang et al., 2015). Moreover, the alloying metal can modify the Pt electronic structure, making Pt atoms less poisoned by CO or alcohol dissociative adsorption (Liu and Nørskov, 2001; Park et al., 2003; Antolini, 2016, 2017; Nosheen et al., 2019). The use of conducting polymers as support for catalysts is also a good strategy adopted by many researchers to improve the catalytic performance. Especially, aromatic conducting polymers such as polyaniline (PANI), polypyrrole (PPy), and poly (3,4-ethylenedioxythiophene) (PEDOT) have been extensively studied as carbon-substitute supporting matrix in numerous catalysts because of their significantly high accessible surface area, increased electronic activity, and most importantly thanks to their excellent thermal and environmental stability and ease of synthesis (Dutta and Kundu, 2014; Memioğlu et al., 2014; Zhou and Shi, 2016; Zac and Sk, 2018; Ghosh and Basu, 2019; Rajathi and Berchmans, 2019; Chemchoub et al., 2020). Owing to the delocalized conjugated structures, excellent electrical, electrochemical, and optical properties, CPs have been widely explored as inherent electrocatalysts and photocatalysts (Zhou and Shi, 2016). The electrons shuttle through polymer chains between the electrode and metal particles to facilitate the electrocatalytic oxidation reaction. In other words, extended p-orbital promotes the electrons movement from one end of the polymer chain to the other as mentioned by Zhengyu Bai et al. (Bai et al., 2015). Exceptional environmental stabilities illustrated in a huge resistance to poisoning intermediate species which promote their use as supporting materials for the elaboration of highly efficient catalysts have been reported by Yaqian Zhao et al. (Zhao et al., 2019). Moreover, conducting polymers play a vital role in reducing catalyst loadings, governing the charge transfer process, thereby lessening the overall fuel cell cost. Consequently, the choice of support material is particularly important in describing the overall fuel cell performance. Owing to the importance of direct alcohol fuel cells field, the number of publications based on metallic and bimetallic particles supported on CPs is constantly increasing as depicted in Figure 2.
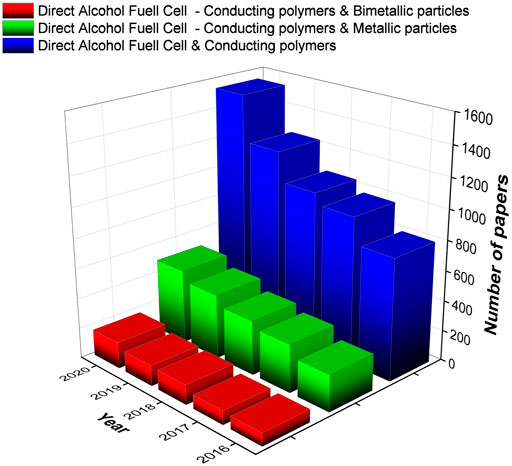
FIGURE 2. Bar chart exhibiting the number of papers published during last 5 years’ basis as given in Science Direct (Keywords: DAFC, CPs, Metallic particles, and Bimetallic particles).
Carbon supports are considered a good strategy to enhance the efficiency of the catalyst. Indeed, they possess several advantages such as high electric conductivity, high specific surface area, and suitable porosity and stability. Carbon nanomaterials such as carbon nanotubes (CNT) (Yang et al., 2019), carbon nanofibers (CNF) (Geng et al., 2020), graphene oxide (GO) (Liu et al., 2020), and reduced graphene oxide (RGO) (Zhang S. et al., 2019) have been largely used as catalyst support and have exhibited a large impact on the electrochemical properties and performance of the catalyst by reducing its particle size down to nanoscale range. Moreover, the porous structure of carbon provides large surface area, which increases the dispersion and distribution of the catalyst and ensures the accessibility and the diffusion of the alcohol molecules (Çağlar et al., 2018; Caglar and Kivrak, 2019). In this sense, Berdan Ulas et al. (Ulas et al., 2019) reported that CNT improve the dispersion and the utilization for metallic catalysts, and hence increase its catalytic performance. Those supporting materials help also avoid catalyst agglomeration throughout the oxidation reaction (Feng et al., 2019). It is demonstrated that the rapid development of nanomaterial, specifically when it comes to carbon nanomaterials, does generate a particularly stable and active catalyst (Zhang et al., 2020a).
To the best of our knowledge, only a few reviews focused on platinum and platinum-free anode catalysts combined with conducting polymers-based nanomaterials. This work highlights the effect of the introduction of metallic nanoparticles or carbon nanostructures and CPs in enhancing EOR and MOR, offering new insights into the design of advanced catalysts for DAFC. In fact, a special attention will be given to mono-, bi-, and tri-metallic nanostructures supported on conducting polymers and CPs-carbon nanomaterials for AOR. This review is divided into three parts, the first part is dealing with synthesis methods of catalysts supported on conducting polymers. In the second part, the introduction of mono-, bi-, and tri-metallic particles into CPs, nature of support materials and their effects, as well as their shape, size, and morphology on the catalytic performance toward AOR will be discussed. Finally, the efficient catalytic activity toward alcohol oxidation related to a variety of carbon nanostructures combined with conducting polymers and metallic particles will be analyzed in detail in the third part. The latest progress of chemical and electrochemical synthesis, experimental parameters for further enhancement and promotion of the catalytic performance and stability in DAFC are also summarized.
Synthesis Methods of Catalysts Supported on Polymer-Based Materials
Conducting polymers (CPs) have been widely proposed as promising supporting materials for metal catalysts by considering their relatively high electrical conductivity, highly porous structure, and cheapness. Owing to their π-conjugated backbones and high porosity structure, CPs display an interesting environment for a highly enhanced dispersion of metal nanostructures and for the formation of metallic nanostructures in a 3-dimensional framework with a high number of catalytic sites available for catalytic reactions. Many CPs have been used as catalyst support such as PANI (Ryu et al., 2002), PPy (Fan and Maier, 2006; Sharma et al., 2008), PEDOT (Anothumakkool et al., 2015), and poly (N-methylpyrrole) (White and Slade, 2003). Nowadays, hybrid polymer-based nanocomposites represent an interesting research field, receiving increased interest in various applications (Salih et al., 2017, 2018; El Rhazi et al., 2018; Kumar et al., 2019; Surmenev et al., 2019). In this context, the combination of CPs with metal nanostructures has generated great curiosity due to their unique optical, electrical, mechanical, and electrochemical properties (Kumar et al., 2019; Nath et al., 2019). Their applications in various areas including catalysis, detection, medical, nanotechnology, and environment have been largely explored (Oularbi, 2018; Oularbi et al., 2019, 2020; Chauhan et al., 2020; El Attar et al., 2020; Kanaujia et al., 2020). CPs have been widely combined with various metal-based nanostructures such as Pt, Pd, Au, Cu, Ni, Fe3O4, TiO2, WO3, SnO2, MoS2, and MnO2(Cho et al., 2011; Wang et al., 2012; Dimitrijevic et al., 2013; Lei et al., 2015; Razzaghi et al., 2015; Li et al., 2016a, 2016b; Lin et al., 2016; Yan et al., 2018; Nath et al., 2019; Kanaujia et al., 2020), with different morphologies and structures. In the past 2 decades, significant progress and development have been achieved in the preparation methods of CPs-metal nanocomposites (Zhang et al., 2018; Begum et al., 2019). Many strategies have been reported to synthesize CP-metal nanocomposites as depicted in Figure 3, namely:
(i) Mixing the pre-synthesized CP with the metal nanostructures,
(ii) Reduction of metallic ions nanostructures on the pre-produced CP,
(iii) Polymerization of a monomer in the presence of the preformed metal nanostructures,
(iv) Mixing the monomer solution with a precursor solution of a metal. The components are synthesized simultaneously to form a nanocomposite material.
In all the above-mentioned methods, many approaches have been involved, including chemical synthesis, hydrothermal method, interfacial synthesis, solvothermal method, photothermal method, plasma, and electrochemical synthesis.
Chemical Synthesis
According to the chemical method, the CP is formed by chemical oxidation polymerization using an oxidant agent, namely APS or H2O2, and the metal nanostructures that could be obtained by a reduction reaction using a reducing agent such as trisodium citrate, hydrazine hydrate. After that, the prepared CP and metal nanostructures are dissolved in appropriate solvents and mixed together to form a CP-metal nanostructures composite after the evaporation of the solvents through a chemical and electrostatic interactions (Han et al., 2017; Zhang et al., 2018). The composition could be controlled and varied by varying the concentration of each component. Several composites have been developed using this way such as PANI/Pt, PANI/Au, and Poly (3-hexylthiophene)/Au. Generally, these composites showed the formation of metallic nanoparticles with an average size of 5–20 nm. However, an aggregation could be formed between these nanoparticles because of the high temperature and the long duration of the solvent’s evaporation process as mentioned by Han el al. Afzal et al. studied the structural and electrical properties of Polyaniline/Gold nanoparticles. Gold nanoparticles were prepared by the reduction of gold salt using extract of tea leaves in 1-methyl-2-pyrrolidinone (NMP) solution. The nanoparticles were then mixed in the NMP solution of polyaniline emeraldine salt and the solvent was evaporated at 120°C for 12 h (Afzal et al., 2009). The metal reduction reaction rate and its morphology are highly influenced by several parameters such as concentration and pH of the precursor’s solution, reaction temperature, and morphology of the prepared polymer and its intrinsic oxidation state. Wong et al.(Wang et al., 2001) reported the preparation of gold and palladium nanoparticles on PANI. The nanoparticles size and the rate metal salt reduction were strongly dependent on the medium used, for which, in organic solvent (NMP) media, the particles size was of approximately 20 nm, whereas in aqueous solution, the particles size was ranging from 50 to 200 nm. In addition, the initial ratio between metal ion to PANI and reaction time influenced both the size and the morphology of the prepared nanoparticles. The larger particles are formed when an acidic media is used, leading to a faster reaction rate and a possibility of aggregation. The oxidation state of the polymer can strongly impact the metal reduction rate. Many studies stated that the deposition of the metal-based nanostructures is higher when the polymer is reduced to its lowest oxidation form in acidic solution (Wang et al., 2001; Milikić et al., 2019). For example, PANI has several oxidation forms. However, to obtain an improved metal reduction rate, the use of PANI in the form of emeraldine salt, known for its good conductivity, is required (Han et al., 2017).
Milikić et al. (2019) reported the synthesis of gold nanorod-polyaniline (Au-PANI) composites using two different methods: an ex-situ method, in which PANI was produced by chemical oxidation polymerization in the presence of preformed gold nanorods, and an in-situ method, where gold nanorods and PANI were formed simultaneously. Regarding the composition of the formed Au-PANI composites, a significant difference in the amount of gold has been identified, and the gold nanorod incorporated into PANI by the in-situ method was over 10 times greater than that formed by the ex-situ method. However, the in-situ method led to aggregated gold nanorods on the PANI surface, contrary to the ex-situ method, which showed the formation of a uniform and nonaggregated structure. Nevertheless, these strategies seem complicated and require a long time, above 24 h, and a huge range of chemicals.
Interfacial Polymerization Method
Interfacial polymerization is a method used for the synthesis of nanomaterials with different morphologies and properties. Interfacial polymerization has been widely used to prepare several polymers such as PANI (Guan et al., 2010; Jain and Annapoorni, 2010; Abdolahi et al., 2012), PPy (Zhang et al., 2010; Qi et al., 2012, 2013), polyamide (Lee et al., 2013, 2015), and polyimide (Gorgojo et al., 2014; Yang et al., 2017), with various morphologies likewise nanoparticles, nanofibers, nanotubes, films, and porous structures (Guan et al., 2010; Abdolahi et al., 2012; Yang et al., 2017). In comparison with bulk polymerization, interfacial polymerization occurs at the interface of an immiscible organic/aqueous biphasic system (Yang et al., 2017). The interface is formed between two immiscible liquids and the monomers are added in one or both liquids. During the reaction, the difference of the chemical potentials of the two liquids caused the monomers diffusion to the interface starting the polymerization in the presence of the oxidant agent (Song et al., 2017).
Many CP/metal nanocomposites have been developed via the interfacial polymerization method such as PANI-Pt (Huang et al., 2009; Cho et al., 2011), PANI-PSS-Pt (Cho et al., 2011), PANI-Ag (Paulraj et al., 2011; Bagheri and Banihashemi, 2014), PANI-Au (Zhang et al., 2012; Bogdanović et al., 2014, 2015; Jin et al., 2016), PANI-MnO2 (Wang et al., 2012; Xu et al., 2014; Li et al., 2015), PPy-TiO2 (Khan and Jain, 2018), PPy-Ag (Dallas et al., 2007; Bedre et al., 2010), and PT/Au (Inagaki et al., 2018). The synthesis process of these materials was based on a polymerization reaction at room temperature between two phases. In general, the monomer is dissolved in the organic phases (toluene, chloroform, etc.) and the oxidant in the aqueous phase. Polymerization occurs at the interface between the aqueous-organic liquids. The reaction can occur in the presence of preformed metal nanostructures or in one-pot synthesis, whereas the metal nanostructures and the polymer are formed simultaneously. Critical parameters namely the concentration, ratio of reactants, and the reaction time period are identified as key variables affecting the composition and the morphology of the resulting material (Bogdanović et al., 2014, 2015; Jin et al., 2016). Bogdanović et al. (2015) reported the synthesis of PANI-AuNPs nanocomposite via the one-pot interfacial polymerization method performed in a water/toluene biphasic system. The aniline was dissolved in toluene and added on the aqueous solution of HAuCl4, and the reaction was left for 20 h to ensure completion. The obtained nanocomposites showed granular morphology with mostly rod-like AuNPs embedded into the PANI matrix. Au nanorods, with 10 nm in diameter and 85 nm in length, among triangular and hexagonal nanoplates, are dispersed in the PANI polymer. The change in the HAuCl4 and aniline concentrations ratio can strongly influence the composition and the morphology of the nanocomposites and can cause the formation of other undesirable products. The synthesis of Au-PANI using interfacial chemical oxidation of aniline in the presence of preformed AuNPs was also described. Spherical AuNPs with controlled sizes (17 and 30 nm) were dispersed in the aqueous phase in the presence of APS, whereas the aniline monomer was dissolved in toluene. The morphology of the nanocomposite showed the formation of fibrous structure of PANI with a homogenous dispersion of AuNPs, without any change in the size of AuNPs (Bogdanović et al., 2014). In contrast to the PANI-AuNPs nanocomposite formed by the one-pot interfacial polymerization method, uncontrollable morphology and dispersion of the AuNPs into the polymer matrix was observed with the aggregation mechanism resulting in irregularly shaped nanoparticles (Bogdanović et al., 2015). Regarding the impact of the reaction time and the monomer/metal precursor ratio, Inagaki and his coworkers (Inagaki et al., 2018) studied the influence of these parameters on the structure and the properties of polythiophene (PT)-AuNPs nanocomposite, using a liquid–liquid interfacial polymerization route. Under optimal conditions, the nanocomposite displayed the formation of spherical AuNPs with a 3 nm diameter dispersed homogenously into PT film. This study demonstrated an inverse proportion between the reaction time and the concentration of the thiophene monomer. In fact, the formation of small spherical and homogeneous particles distributed in the polymer matrix might require a large amount of the monomer and a short reaction time.
Hydrothermal Method
Hydrothermal method is a liquid phase preparation technique used to crystalize materials from an aqueous solution by a great control of temperature, pressure, and material composition. This method can offer special advantages for preparing nanomaterials due to its ability to control diffusivity in a closed system. Hydrothermal technique has found its place in many areas of research and has been used in several interdisciplinary fields of science, such as chemistry, physics, biology, metallurgy, and geology (Darr et al., 2017; Zhang Q. et al., 2019; Townley et al., 2019; Sahu et al., 2020). Therefore, there has also been increased interest in combining hydrothermal technology with other processes such as microwaves, ultrasound, mechanochemistry, electrochemistry, and other processes (Meng et al., 2016; Darr et al., 2017; Yoshimura et al., 2017; Long et al., 2020; Svietkina et al., 2020). These combinations offer several advantages for improving the hydrothermal reaction kinetics, reducing the experimental time, and preparing new kind of materials (Meng et al., 2016). In the hydrothermal method, the reaction takes place in a closed system using water as a solvent and this last changes its properties at certain temperature and pressure values (Meng et al., 2016; Darr et al., 2017). Under a hydrothermal conduction (high temperature and pressure), the ionization of water increases while its viscosity decreases, which causes a great increase in the molecules and ions mobility in the solution, resulting in improved ions reaction rates and hydrolysis reaction (Meng et al., 2016). Many kinds of materials have been developed using the hydrothermal technology such as fibers and single crystals, metal nanostructures, polymers, and ceramics. It offers the ability to significantly control the morphology of the product and reduce the aggregations by regulating the rate and the uniformity of nucleation and growth processes during the precipitation of the crystalline powder from solution, which offer a material with both optimized properties and structure.
Several materials combining conducting polymer and metal nanostructures have been synthesized through the hydrothermal method such as TiO2-PPy, WO3@PPy, MoS2-PPy, SnO2-PPy, MoS2-PANI, SnO2-PANI, Fe3O4-PEG, and Fe3O4-PANI (Dimitrijevic et al., 2013; Junejo et al., 2013; Lei et al., 2015; Zhang Y. et al., 2016; Das et al., 2019; Mahmoudi-Badiki et al., 2019; Yin et al., 2019). Lie et al. (2015) reported the synthesis of MoS2-PPy nanocomposite using one-pot hydrothermal synthesis, aqueous solution of ammonium tetrathiomolybdate precursor. Then, the mixed solution was transferred into an autoclave and heated at 200°C for 15 h. During the reaction process, MnS2 was self-formed under hydrothermal conditions, whereas pyrrole was directly polymerized by chemical oxidation in the presence of ammonium tetrathiomolybdate. The morphological characterizations showed that the prepared nanocomposites displayed an algue-like structure composed of a few layer MoS2 nanosheet, recovered with PPy film. In another work, the one-pot synthesis by the hydrothermal method was used by Junejo and coworkers to prepare a core-shell structure of Fe3O4-PEG nanocomposite with a superparamagnetic property (Junejo et al., 2013). The in-situ synthesis of Fe3O4 and PEG was carried out in a Teflon-lined autoclave at 180°C for 10 h. The resulting product showed the formation of a large quantity of uniform nanoparticles with a mean size of approximately 40 nm, composed of PEG molecules adsorbed on Fe3O4 nanoparticles through oxygen atoms. Yin et al. (2019) used the hydrothermal method to synthesize PEDOT: PSS/MnO2 material. The nanocomposite displayed the formation of nanorods with a length of approximately 1 μm and a diameter of approximately 100 nm. The results showed that the reaction temperature was a critical parameter, which played an important role in determining the morphology of the prepared material. In another work, Dimitrijevic et al. (2013) prepared the TiO2/PPy catalyst by one-step hydrothermal synthesis, forming a granular structure with approximately 200-300 nm in the diameter.
Electrochemical Method
Electrochemical synthesis consists in running an electric current between two or three electrodes emerged in an electrochemical cell to produce the desired materials at the interface electrode/electrolyte (Halim et al., 2021a). The electrochemical approach ensures a good adhesion of the prepared material on the electrode surface. In general, the morphology of the electrodeposited material depends on the surface and the conductivity of the electrode substrate (Venkata Jagadeesh and Lakshminarayanan, 2019).
The deposition of CP could be performed through electrochemical oxidation of monomer and metal nanostructures, which in its turn could be obtained by the reduction of a metal salt by electrodeposition. The electrosynthesis is based on the deposition of a solid product from the electrolyte containing the dissolved reactant. This deposition could be controlled by (Figure 4):
(i) Applied current (galvanostatic synthesis), when a current is applied, the material can be quickly formed;
(ii) Applied potential (potentiostatic synthesis), when the electrode is polarized to a desired potential with respect to a reference electrode;
(iii) Cyclic voltammetry, when the synthesis is carried out within a potential window.
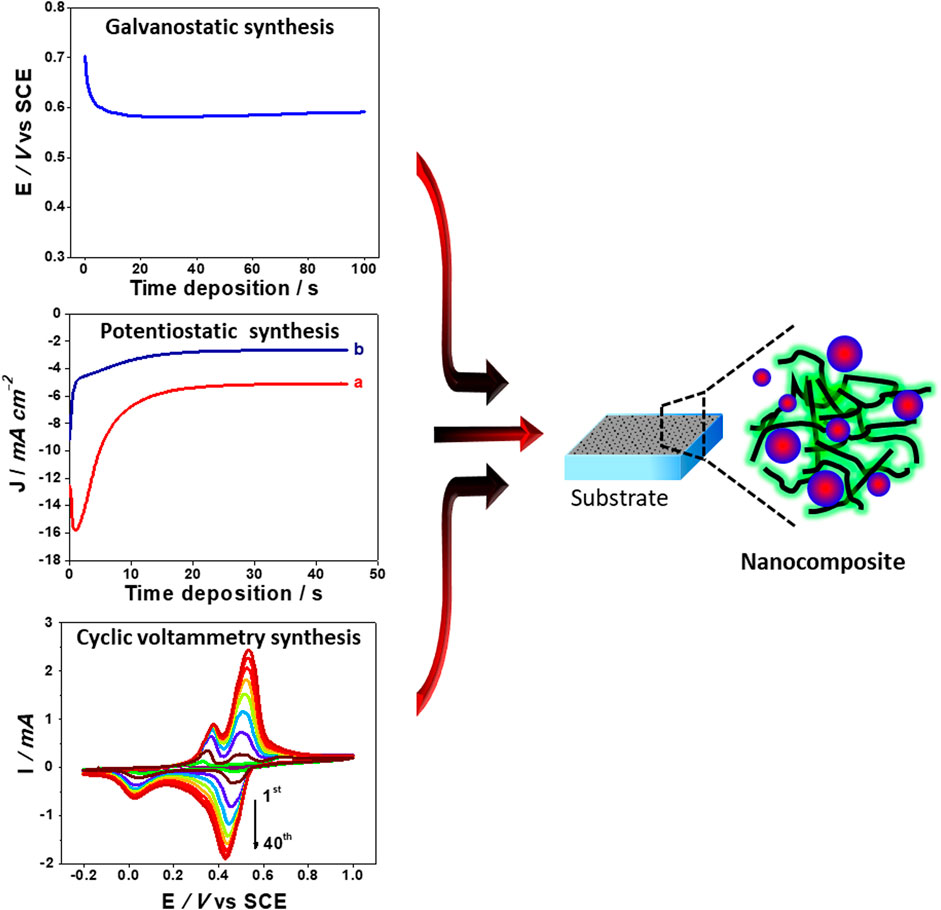
FIGURE 4. Electrochemical routes to synthesis of conducting polymer—metal nanostructures composites.
The material synthesis by both the galvanostatic and potentiostatic methods could be controlled as a function of the reaction time, whereas the synthesis by cyclic voltammetry could be controlled by varying the number of cycles. Yan et al. fabricated raspberry-like PtNps/PANI nanocomposite via the one-step electrochemical method (Yan et al., 2018). The nanocomposite was performed on GCE using cyclic voltammetry from a solution containing aniline monomer and potassium tetrachloroplatinate precursor. SEM and TEM characterizations illustrated the formation of PtNps/PANI nanoparticles, with a 40-60 nm diameter composed of small Pt particles of approximately 2-5 nm distributed in PANI film. During the cyclic voltammetry process, PtNps were attached to PANI film by the interaction of Pt atom with N atom, leading to a marked increase in the quinonoid units in the PANI structure. Lie et al. (2016) developed the PEDOT/AuNps composite on GCE through the potentiostatic deposition of PEDOT in the presence of commercial AuNps. The electropolymerization of EDOT monomer was performed using a potentiostatic mode. The PEDOT/AuNps composite displayed a 3D porous network structure with a highly effective surface area. The presence of AuNps acted as both dopants and templates during the electropolymerization. In fact, PEDOT grew around one or a few nanoparticles to form strong bonding between them because of the effective interaction between sulfur atoms offered by the PEDOT structure and Au atoms (Harish et al., 2008). Figure 5 depicts different nanostructured materials applied as anode catalysts for alcohol oxidation.
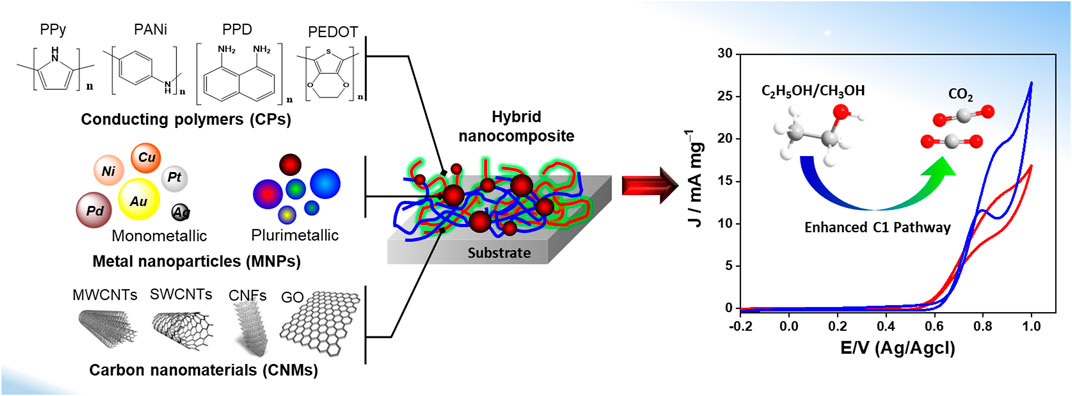
FIGURE 5. Conducting Polymers/ Carbon nanomaterials/ Metal nanoparticles as anode catalysts for alcohol oxidation.
It should be mentioned that the electrochemical synthesis depends on many reaction parameters such as electrode surface: pH, concentration, temperature, and composition of the electrolyte, and the electrodeposition mode. Venkata Jagadeesh and Lakshminarayanan (2019) were interested in studying the impact of the electrode substrate on the morphology and the catalytic properties of Pt-PANI nanocomposite. The nanocomposite was prepared using one-pot synthesis performed by the galvanostatic mode at 10 mA cm−2 on three kinds of graphite electrodes (flexible graphite sheet (GS), exfoliated graphite sheet at positive potential (+IGS), and exfoliated graphite sheet at negative potential (−IGS)). The results showed that the morphology of Pd-PANI nanocomposite was completely different between the three studied electrodes. In fact, on the GS the nanocomposite displayed nanoclusters with cauliflower-shaped domains, whereas the globular structure was observed on the (+) IGS. A cylindrical rod-like structure was formed on (–) IGS. In addition, the exfoliation of the graphite sheet creates a large number of edge planes and defects at the surface, acting thus as heterogeneous sites for the deposition of Pd-PANI, which result in the increase in the loading and the catalytic sites of Pd. The electrochemical synthesis of MNs/CP offers the advantage to be an eco-friendly (the uses of strong reducing agents are reduced), available, and low-cost method. More interestingly, it allows the control over both size and structure of the nanocomposites.
Having discussed in the previous section the common approaches used for the preparation of CP/metal nanocomposites (summarized in Table 1), regardless of their application fields, we will focus, in the next section, on the catalytic performance of CP/metal nanostructures composites for direct alcohol fuel cells applications.
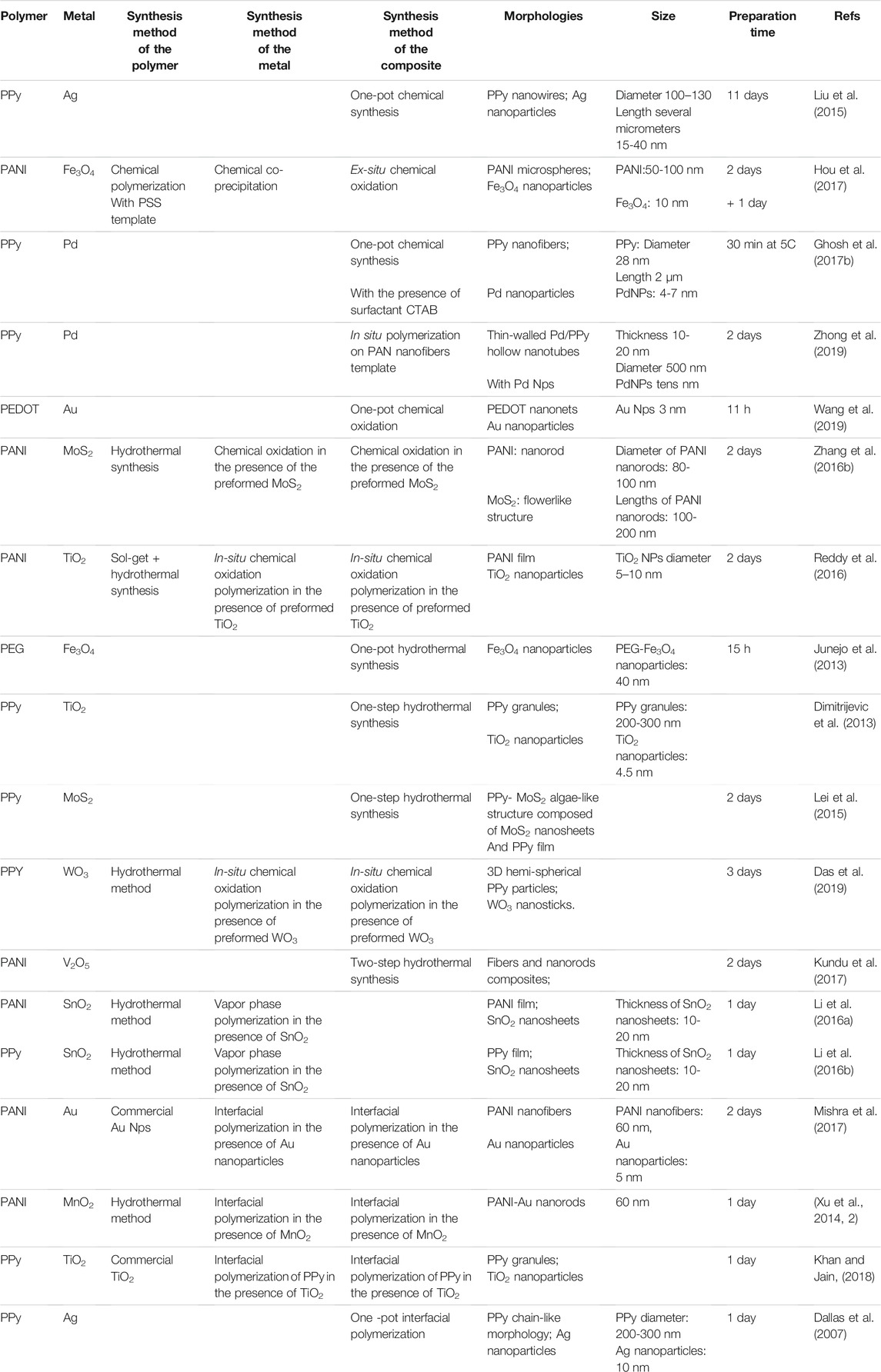
TABLE 1. Comparison of morphological structure and synthesis techniques of monometallic-based conducting polymers catalyst.
Electrooxidation of Ethanol and Methanol on Catalyst Supported on Conducting Polymers
Monometallic Catalyst Supported on Conducting Polymer
Various studies have been undertaken on the oxidation of alcohols using metal nanostructures. Generally, metal nanostructures with small size and low loading present exceptional catalytic properties, in contrast to their bulk forms, for direct alcohol oxidation. However, the main factors affecting the development of a new catalyst are the long-term stability and the cost, which depend on its size, shape, and support materials used. Regarding the effect of the catalyst support material, multiple roles have been discussed, for instance: 1) increasing the stability of the catalyst, 2) offering a high active surface area for the deposited catalyst, 3) contribution in the mass transfer and the oxidation reaction, and 4) reducing the poisoning of the catalyst surface (Huang et al., 2012; Das et al., 2015; Caglar and Kivrak, 2019; Wu et al., 2019). Some initiatives have been taken to address some of these issues such as using metal-based nanostructures supported on CPs. In fact, CPs are extremely important and offer multiple advantages such as good electrical conductivity, high specific area, and good chemical stability, which lead to a uniform distribution of metal-based nanostructures with optimized metal loading and maximize the electrocatalytic sites for effective alcohol oxidation reactions. In this context, platinum-based nanostructures have been extensively studied for various alcohol oxidation reactions, especially methanol and ethanol oxidation reactions (Shah et al., 2016; Yan et al., 2018). Yan et al. (2018) fabricated the raspberry-like Pt NPs/PANI composite (PNPC) for methanol electrooxidation. The electrocatalytic performance of the prepared catalyst was compared to that of the commercial Pt/C catalyst using both cyclic voltammetry and chronoamperometry techniques. The current of the methanol oxidation peak for PNPC was 1.2 times higher than that of the Pt/C, after 1,000 cycles, the mass activity of PNCP and Pt/C remained 80.1 and 61.9% of the initial value, respectively. For the chronoamperometry test, PNPC showed superior catalytic stability and retained the higher current density after 500s. In another work, Ali Shah et al. (Shah et al., 2016) studied the electrooxidation of methanol at the PANI/POAP-Pt and POAP-PT electrodes in both pure methanol and sulfuric acid mixed methanol solutions. It was observed that the PANI/POAL-Pt electrode presents low electrocatalytic activity and less stability in the presence of H2SO4, which was explained by the lesser stability of PANI-coated electrode in sulfuric acid. However, when a pure methanol solution was used, the PANI/POAL-Pt electrode demonstrated lower onset potential along with the highest peak current during the methanol oxidation reaction, which was 5 times higher than that obtained with the POAP-Pt electrode. On the other side, when the alcohol oxidation reaction operates in alkaline media, the reaction kinetic would be significantly improved and non-Pt catalyst could be used. For instance, Palladium catalyst with high catalytic performance enhanced resistance to CO poisoning compared with Pt-based catalysts (Habas et al., 2007; Guo et al., 2015). Pd-CP hybrid nanocomposites have been widely investigated as electrocatalysts for the oxidation of both methanol and ethanol. Pd nanoparticles deposited on hollow PPy nanospheres (h-PPy) were used as anode material for direct ethanol fuel cell (Bai et al., 2015). The catalytic performance of the Pd/h-PPy toward ethanol oxidation was compared with those of Pd deposited on solid PPy and Pd deposited on acid-oxidized Vulcan XC-72 in alkaline media. The Pd/h-PPy catalyst exhibited better catalytic activity and stability, owing to the hollow-sphere structure of PPy and the formation of the active Pd-N sites. Accordingly, controlling the morphology of the CP support could improve the catalyst performance. The catalytic performance of metal nanostructures could be further improved by using copolymer. More recent work has focused on the formation of Pd nanoflowers on the copolymer of aniline and pyrrole (PPCA) (Fard et al., 2017b). The electrocatalytic activity of Pd/PPCA toward the methanol oxidation reaction was 50 and 30% higher than Pd/PANI and Pd/PPy, respectively. The copolymerization provided an enhanced electrochemical active surface area leading to easier charge transfer and higher electrocatalytic properties of Pd nanoflowers. The role of gold particles in promoting the electrooxidation reaction of alcohol has also been explored by several groups due to its superior catalytic activity at high pH. It seems also that the adsorbed poisoning species such as CO can be easily oxidized (Pandey and Lakshminarayanan, 2012; Wang et al., 2019). K. Pandey et al. (Pandey and Lakshminarayanan, 2012) compared the electrocatalytic performance of gold nanoparticles (Au) deposited on various CPs, namely, PANI, PPy, PT, and PEDOT, for the ethanol oxidation reaction. The prepared Au-CP nanocomposites displayed different morphologies depending on the nature of the CP. Indeed, Au-PANI nanocomposite showed a spherical disc-like feature with a diameter of approximately 100–400 nm, and Au-PPy nanocomposite revealed flat triangular and rectangular features of approximately 20-100 nm with sharp edges. Au-PT presented a dendritic structure that is more than 1 μm in length, and composed of side branches with 10-20 nm diameter. On the other hand, Au-PEDOT material showed the presence of cauliflower-like nanostructures with a fractal growth and a varied size from 20 to 100 nm (Pandey and Lakshminarayanan, 2012). Concerning the electrocatalytic performance, these nanocomposites exhibited a high durability for EOR in alkaline media, except for the Au-PEDOT catalyst, which showed a 38% decrease in the peak current of ethanol electrooxidation. The Au-CP nanocomposites showed a quasi-unchanged peak current, after 200 cycles. Indeed, the polymeric support offers high porosity that makes the electrocatalytic centers easily accessible by the reacting species. The best catalytic performance was observed by combining PANI with Au particles (Halim et al., 2019b; Chemchoub et al., 2020). It should be noted that small metallic nanoparticles (less than 5 nm) have emerged as highly useful and active electrocatalyst for many catalytic reactions (Hvolbæk et al., 2007; Zhang et al., 2017). Zhang et al. (2017) studied the electrooxidation of ethanol in alkaline media on Au nanoparticles/poly (3,4-ethylenedioxythiophene): polystyrene sulfonate (AuNPs/PEDOT:PSS) nanocomposite deposited on Pd substrate. Doping PEDOT by PSS could improve its electrical properties as well as its stability (Horii et al., 2018; Kayser and Lipomi, 2019). An enhanced catalytic performance was achieved with respect to the Pd electrode, especially the best catalytic performance was obtained using a small AuNPs size (less than 5 nm). These findings could be explained by the effective transfer electrons and diffusion of the reactive species through the porous polymer network, as well as the synergistic interaction between the AuNPs, conductive polymer, and Pd electrode interfaces (Zhang et al., 2017). In alkaline media, the ethanol electrooxidation is generally initiated by the cleavage of the O-H bond of ethanol on the Au surface, and the formation of gold hydroxide by chemisorption of OH− species on the gold surface, contrary to that in acidic media, resulting in faster kinetics of alcohol oxidation on Au in alkaline media (Dutta et al., 2015; Arjona et al., 2016; Wang et al., 2019). However, Pt, Pd, and Au-based catalysts present some critical drawbacks, especially their high cost and low abundance, which make the cost of fuel cell devices very high and inevitably prohibit their commercialization (Khalafallah et al., 2018; Pattanayak et al., 2018; Chemchoub et al., 2020). In comparison with precious metals, transition metals are more economical, abundant, and have attracted the attention of many researchers due to their promising performance as catalyst for alcohol oxidation reactions in alkaline media. It is in this context that Ni has emerged as one of the most promising low-cost catalysts for the electrooxidation of alcohol (Das et al., 2015, 2016; Ehsani et al., 2015; Ali Shah et al., 2017; Chemchoub et al., 2019; Vyas et al., 2020). In fact, Ni-based catalyst presents high tolerance toward the intermediates formed during electrooxidation reactions, and enhances stability due to the formation of Ni(OH)2 and NiO on the catalyst surface (Ehsani et al., 2015; Ali Shah et al., 2017; Halim et al., 2019b; Chemchoub et al., 2020). Ehsani et al. (2015) reported the synthesis of NiO on polytyramine (PTy) matrix for direct electrooxidation of methanol. The PTy was formed in the absence and the presence of sodium dodecyl sulfate (SDS) and the impact of this latter on electrocatalytic activity toward MOR was investigated. PTy-SDS-NiO catalyst exhibited a larger methanol oxidation response, which was 2 times higher than that of PTy-NiO catalyst. This result was explained by the positive impact of SDS on the surface area of the Pty polymer, leading to both high and good dispersion of Ni nanoparticles. In other approach, Das et al. studied the impact of the incorporation of sulfonic acid (−SO3H) dopant on the properties of PPy (Das et al., 2016) and PANI (Das et al., 2015) supports and on the catalytic properties of Ni. The performance of Ni deposited on partially sulfonated PPy (SPPy) was compared with those of the Ni/PPy and the commercial Pt-Ru/C catalysts. The deposition of Ni nanoparticles catalyst on sulfonated polymer shows a higher deposition, smaller particle sizes, and a better dispersion and distribution compared with non-sulfonated polymer support.
More recently, Chemchoub et al. (2020) deposited Ni nanoparticles on the PPy matrix and studied the effect of the regeneration of nickel nanoparticles (NiNPs-R) on the kinetics of the EOR. The regeneration was performed in acidic solution followed by redeposition of Ni NPs under potentiostatic mode. Then, the electrooxidation of ethanol was studied on both electrodes (regenerated and nonregenerated NiPs). The regenerated electrode showed a major increase in the current, which was 10 times higher than that of the nonregenerated electrode. Accordingly, the regeneration approach of the electrode allows an easier access of ethanol molecules to the catalytic sites.
Additionally, metal oxide has received considerable attention for direct alcohol fuel cells (Scibioh et al., 2008; Hassan and Tammam, 2018; Khalafallah et al., 2018). In fact, they are able to adsorb a large number of OH- species involved in the redox process occurring between different oxidation states of the metal oxide, and improve their catalytic properties (Scibioh et al., 2008; Hassan and Tammam, 2018). Khalafallah et al. (2018) developed a metallic cobalt oxide core wrapped with a PPy nanoshell (PPy/Co3O4) for the electrocatalytic oxidation of methanol. The results show that the PPy/Co3O4 exhibited superior catalytic stability and activity, which was 4 times higher than that on Co3O4. These results highlighted the positive impact of the incorporated PPy shell, improving the number of the electroactive sites, the electrical conductivity, and the surface area. El Attar et al.(2020) prepared cuprous oxide on PPy using a simple electrochemical approach. The resulted Cu2O/PPy nanocomposite showed enhanced catalytic activity and stability for 30 min. The catalytic activity of the Cu2O/PPy electrode was 8 times larger than the response of the Pt electrode. The same researchers developed a nanodendritic cuprous oxide on PPy using the regeneration technique in the acidic medium (El Attar et al., 2021). The obtained results showed that the regeneration of catalyst changes the morphology of copper oxide deposited on PPy from octahedral particle with an average size of 1.2 um to dendritic nanoparticles with an average size of 100 nm. The catalytic activity of Cu2O-NDs/PPy was 1.8 times larger than the response of the Cu2O/PPy with a high stability under 1,000 scans (only a decrease of 7.25% after 1,000 scans).
Overall, it has to be noted that CPs have been emerged as an ideal catalyst support to avoid the aggregation and the poor distribution of metal nanostructures and to promote their catalytic activity. The catalytic performance of different catalysts discussed in this section with their synthesis strategies and morphologies is summarized in Table 2.
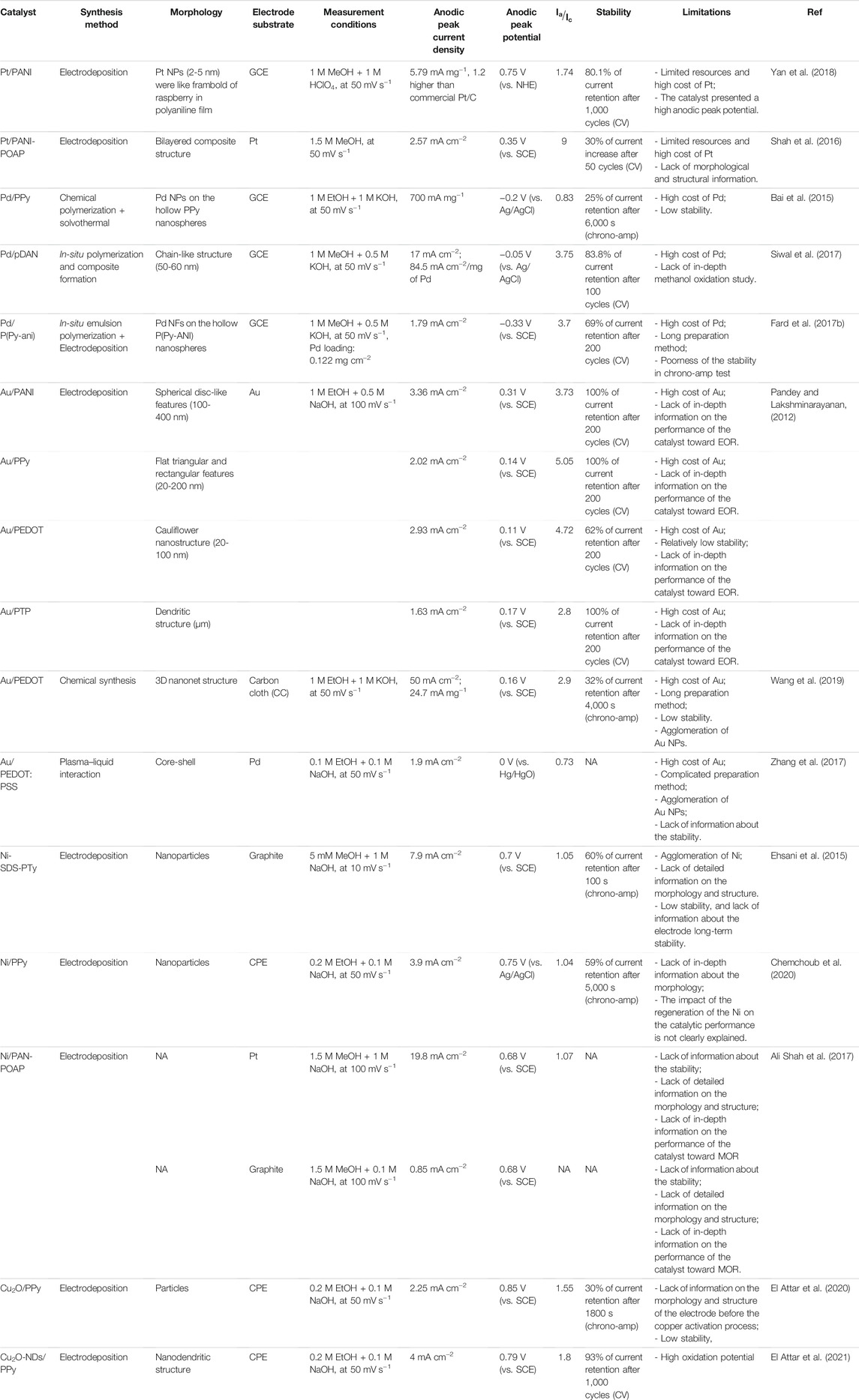
TABLE 2. Electrochemical properties and electrocatalytic performances of monometallic-based conducting polymers catalyst applied for alcohol electrooxidation.
Moreover, extensive efforts have been devoted to the development of binary or ternary alloys supported on CP with promising catalytic properties. In the next section, we will refer to the catalytic performance of alloys supported on CP.
Binary and Ternary Alloys Supported on Conducting Polymers
As explained in the previous section, Pt-based catalysts have been developed as the most mature anode in DAFCs due to their superior activity toward alcohol electro-oxidation. However, the reaction intermediates (e.g., CO and CHx) generated during the catalytic process can cause obvious surface poisoning of Pt-based catalysts, leading to the sluggish kinetics of the alcohol reaction and thus weak catalytic performance (Ong et al., 2017; Xie et al., 2019). One of the most promising strategies to modulate the electronic structure of Pt is to alloy it with other metals to form bimetallic and trimetallic catalysts (Tao et al., 2019). Excellent papers have been devoted to the development of binary or ternary Pt-based catalysts with reduced Pt loading or carefully controlled microstructures as well as the design of non-noble metal-based alternatives (Ghosh et al., 2017a; Jin et al., 2019). Alkaline electrolyte has proven to improve drastically the kinetics of alcohols as far as the electrocatalytic reaction is concerned, and allows the substitution of expensive Pt catalyst by more available, cost-effective, non-noble metals (Yuan et al., 2015; Guo et al., 2018). One of the most advantageous aspects of using bimetallic and trimetallic catalysts is that the additives are usually affordable and act as cocatalysts that reduce the platinum content needed for the oxidation reaction and most importantly promote the C-C bond cleavage in the case of EOR. In fact, bi and tri-metallic alloys lead to a substantial improvement in the catalytic properties of the resulting nanoparticles which is not achievable by using a monometallic catalyst (Ren et al., 2020). Yajun Liu et al. (Liu et al., 2019) elaborated a solvothermal synthesis of trimetallic (PtAuCu) alloy nanowires (NWs) with adjusted and optimized percentages of individual metals. The trimetallic PtAuCu NWs exhibited an excellent electrocatalytic activity toward the methanol oxidation reaction attributed specifically to the synergistic contact of different metals. Indeed, in bi and tri-metallic catalysts, the electronic effect is of great importance since it describes the charge transfer. In other words, the electronic structural modification of the bimetallic nanoparticles is directly induced by alloying the constituting elements. Consequently, preventing the intermediately formed carbonaceous species from reaching the metal catalyst shall eliminate the problem of catalyst poisoning (Ghosh et al., 2017a; Nasrollahzadeh et al., 2019). Shu-He Han et al. (Han et al., 2018) proposed a galvanic reaction to elaborate hollow and porous PtRhCu trimetallic nanoboxes (CNBs) for EOR. The nanocatalyst showed an improved specific mass activity and stability which was ascribed to its elevated geometric structure and synergistic effects.
The previous studies stated that the polymer-supported metal catalysts exhibited an enhanced catalytic performance because of their high chemical stability and superior catalytic activity (Selvaraj et al., 2006). Aside from reducing the cost and providing a uniform dispersion of metal nanoparticles, conducting polymers as catalyst supports help to expand the accessible surface area of the catalyst system (Datta et al., 2012). However, the choice of a suitable support is essential to achieve a better cell performance (Dwivedi et al., 2018). Many studies were carried out on applying conjugated polymers with high conductivity such as PANI (Ivanova et al., 2016), PPy (Ghosh et al., 2017a), poly-para-phenylendiamine (PpPD) [The electrocatalytic oxidation of methanol on a carbon paste electrode modified by poly (para-phenylenediamine) and Nickel particles, 2019], poly-ortho-phenylendiamine (PoPD) (Gajendran and Saraswathi, 2013; Parsa and Amanzadeh-Salout, 2016; Halim et al., 2019a), PEDOT (Nakova et al., 2019) as support of metallic nanoparticles.
Novel synthetic routes have been developed to fabricate various catalysts to improve the catalytic performance of DAFCs by strengthening the contact or interaction between catalysts and supports which can further enhance the electron and mass transfer. For instance, electrodeposition was confirmed to be a quick, reliable, and reproducible method for catalyst metal nanoparticles (NPs) and is found to be an efficient method for electrochemical reactions (Salinas-Quezada et al., 2019). Zahra Nodehi et al. (Nodehi et al., 2018) suggested electrochemical synthesis of bimetallic Ag-Pd synthesis on PANI via the constant current method used as an efficient electrode for the electrooxidation of ethanol in alkaline media. In this electrosynthesis, the catalytic enhancement is attributed to the synergic effect between PANI, Ag, and Pd. PANI was deposited as wire and particle morphology which explained the improvement of the contact surface area, Ag and Pd presented a dendrite shape that provided a larger surface area that increases the catalytic performance toward EOR that reached almost 12 A cm−2 mgPd−1. Nevertheless, its long-term stability and durability was not thoroughly evaluated. Following a similar method, Mohammad Lashkenari et al (Soleimani-Lashkenari et al., 2018) explored an electrochemical approach for Pd nanoparticles supported on the PANI and titanium dioxide (PANI/TiO2) synthesis. This electrocatalyst demonstrated excellent catalytic performance for methanol oxidation and exhibited a higher electrochemical surface area as opposed to pure Pd catalyst which was ascribed to the proper conducting behavior of TiO2 along with the polymeric matrix of PANI. Pure PANI showed a smooth surface and flakes morphology. In contrast, TiO2 contributed to the reformation of PANI/TiO2 morphology with nanoparticles, indicating that TiO2 is thoroughly distributed within the PANI structure. On the elaborated electrocatalyst, the onset potential shifted to negative values by 120 and 140 mV compared with the onset potentials of Pd and PANI/TiO2 for methanol oxidation, respectively. This was attributed to the enhancement of methanol oxidation kinetics resulting from the increased hydroxide ions concentration in the electrolyte. In terms of stability, a decay of approximately 41% of the forward anodic peak during 150 cycles was registered. Using a different method, Das et al. (Das et al., 2018) utilized PANI nanostructure support to deposit Pt-Ru catalyst nanoparticles to be used as an anode for methanol oxidation. It was highlighted that the PANI NWs support showed a better dispersion allowing a higher utilization of the bimetallic Pt-Ru catalyst nanoparticles. The PANI NWs synthesis was carried out at the interface of an aqueous layer containing HCl and the oxidant ammonium peroxodisulfate and an organic layer containing aniline monomer. The obtained NWs, having an average length of 40–45 nm and an average diameter of 10–15 nm, promoting the dispersion and utilization of the deposited Pt-Ru nanoparticles, and leading to an excellent electrocatalytic activity, a higher resistance to catalyst poisoning species, and good stability. The highest current density generated by this electrocatalyst reached 255.6 mA cm−2, which was recorded at the end of the testing period 700 s. This value was much better than that of Pt-Ru/C (180.1 mA cm−2) catalyst systems. The nanoparticles formation mechanism is reviewed by taking into consideration the polymer’s redox potential, its chemical structure, and the position of the side amino groups. This method assures the easy control of the incorporated metal nanoparticles.
Cobalt copper metal-organic framework (CoCu-MOF)/PANI synthesized by the hydrothermal method followed by in-situ polymerization was investigated for glycerol oxidation (Zhu et al., 2020). The CoCu-MOF/PANI hybrid showed an astonishing electrocatalytic activity, a higher stability, and a better resistance to poisoning species. The superior electrochemical activity was ascribed to the high porosity and the synergistic behavior of bimetallic structure with the highly conductive PANI. From a structural aspect, this catalyst showed a relatively regular smooth petal shape with the thickness of tens of nanometers. The well-controlled microstructures of the catalysts contribute toward enlarging the specific area of the catalyst and creating abundant active sites, which is crucial for a better catalytic activity (Zhu et al., 2020). In contrast, Jayati Datta et al. (Datta et al., 2012) elaborated an in-situ solid-state polymerization of poly-vinyl carbazole (PNVC) cross-linked with V2O5. The alloyed PtPd NPs were grown onto this support by NaBH4 reduction of the precursor salts taken in an appropriate proportion. The synthesized composite produced an improved activity for ethanol electro-oxidation in the alkaline medium. It also exhibited a satisfactory tolerance to CO poisoning leading to a good level of ethanol conversion into the end products. Activation energy for the oxidation reaction is tremendously reduced when the PNVC is used as support. Indeed, the peak power density on the elaborated catalyst reaches a value of 30 mW cm−2, approximately 36% higher than that obtained with PtPd/C and 130% higher than that attained with Pt/C. Following a distinctive perspective, a colloidal radiolytic technique was established to develop trimetallic PdPtAu alloys incorporated on PPy nanofibers by in-situ nucleation (Ghosh et al., 2017a). The polymerization of PPy was carried out in the presence of CTAB and APS in aqueous HCl. On the other hand, metal nanoparticles were deposited on PPy using a steady-state gamma irradiation under N2 flow. The as-prepared catalysts exhibited a substantial enhancement of electrocatalytic performance toward ethanol oxidation in the alkaline medium reaching 32.45 mA cm−2 activity which is ∼5.5 times higher than monometallic. Interestingly, the elaborated nanocatalyst shows a very good stability reflected with 2% loss in forward current density during 1,000 cycles, implying that the electrocatalysts could be efficiently recycled without noticeable loss of activity. Concerning the synthetized catalyst morphology, the average particle size reached ∼5 nm. The ternary alloys demonstrated a higher catalytic activity in comparison with monometallic catalysts thanks to the synergistic electronic effects of multimetallic components and good dispersion of Pd NPs on PPy nanofibers. It can then be concluded from the above researches that the introduction of conducting polymers is essential for the formation of small nanoparticles, and the creation of N species as active sites, which is favorable for electrocatalysis. It should be highlighted that the most important aspects of using conducting polymers as catalyst supporting materials include a better and more uniform dispersion of metal catalyst, a higher surface area, increased reaction rates, a higher interaction with the deposited metal particles, better interfacial properties, and increased conductivity of the generate electrons (Dutta, 2020). An electrochemical method was introduced to Poly (brilliant cresyl blue) supported the Pt-Ru catalyst for MOR (Khalifeh-Soltani et al., 2020). It was concluded that the nitrogen atoms of the conducting polymer provide numerous favorable sites available for nanoparticles deposition by providing the main initial nucleation sites for the creation of nanoparticles. This catalyst provided excellent catalytic currents throughout the experiment with a good stability. In another work, the hydrothermal method was explored to synthetize the Ag-Ni porous coordination polymer followed by an electrodeposition of Pt NPs as an electrocatalyst for methanol oxidation in alkaline media (Khakpour et al., 2019). The anodic current peak of Pt-ANP reached approximately 105 mA/cm2 which is 33% higher than the anodic peak of pure Pt catalyst (70.21 mA/cm2). The morphological structure of Pt-ANP electrocatalyst demonstrates the formation of metallic nanoparticles as clusters in a diameter ranging from 20 to 70 nm. This catalyst exhibited a favorable stability over a total of 100 cycles with a slight decrease from 90 to 87 mA/cm2 preserving 96% of its primary activity; this was explained by the depletion of methanol and electrode poisoning. The polymeric substrate exhibited a sheet-like structure making the deposition of AuPd easier. Yunong Li et al. (Li et al., 2020) investigated an original method to substitute external heating during the catalytic reaction. A bimetallic AuPd nanocatalyst anchored aza-fused p-conjugated microporous polymer (aza-CMP) was prepared by using a simple method for light-driven benzyl alcohol oxidation. This nanocatalyst showed an improved catalytic activity for benzyl alcohol oxidation using simulated light irradiation.
It is noteworthy that total or partial substitution of Pt can promote the creation of oxygenated species at a relatively low potential, which can alleviate the poisoning effect through the reaction between oxygen-containing groups and CO intermediates (Wassel et al., 2020). In summary, bimetallic electrocatalysts-supported conducting polymers are acknowledged to have an excellent activity toward alcohol dehydrogenation, oxygen-containing species growth, CO and OH adsorption, and successful C-C bond cleavage in the case of ethanol (Khammarnia et al., 2020). In addition, and given the fact that the catalytic process is highly dependent on the microstructure, the shape and size control is essential for strengthening the catalytic performance for AOR. To expand surface areas and to improve the catalysts reactions kinetics, the morphology control through the nanostructure is of extreme necessity and has widely been investigated (Pinithchaisakula et al., 2017).
The electrochemical properties and electrocatalytic performances of Bi/Trimetallic-based conducting polymers catalyst applied for alcohol electrooxidation are summarized in Table 3.
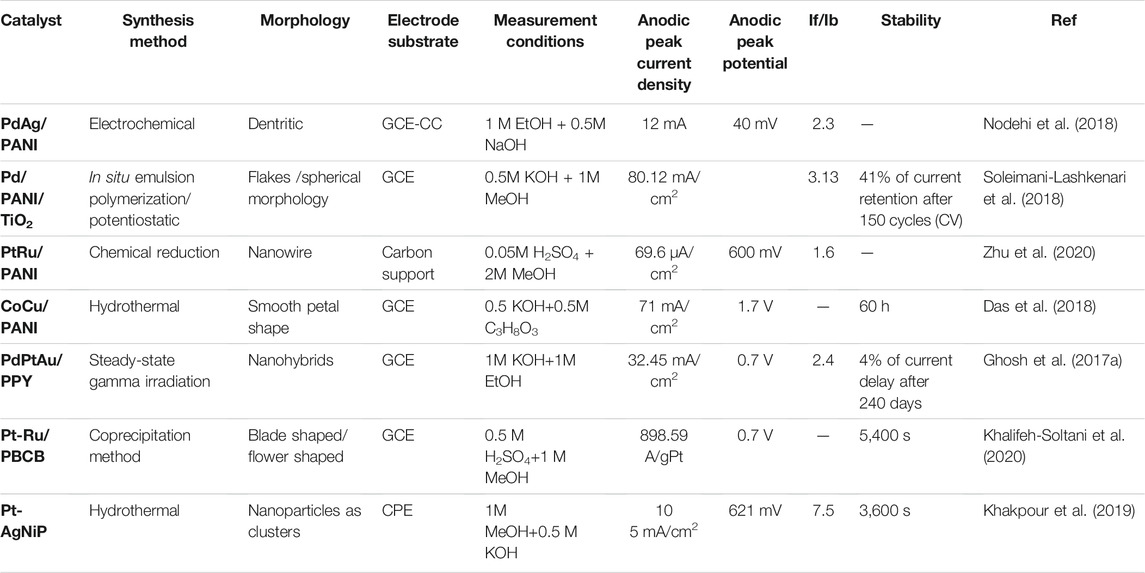
TABLE 3. Electrochemical properties and electrocatalytic performances of bi and trimetallic-based conducting polymers catalyst applied for alcohol electrooxidation.
Metal Catalyst Supported on Conducting Polymer-Carbon Nanomaterials
Since the Nobel prizes rewarding the discovery of carbon nanotubes and graphene, carbon nanomaterials (CNMs) have become an attractive research area owing to their exceptional properties. They are considered the most innovative materials that have attracted enormous interest as catalysts for many fields and in alcohols oxidation (Yang et al., 2016; Çelik et al., 2016; Mao et al., 2019; Wu et al., 2019). CNMs have been extensively used as substrates for metallic electrodeposition thanks to their particular surface area and mesoporous structure that enhance the electrochemical active surface area and ensure a good dispersion. Graphene, with a two-dimensional (2-D) carbon form, possesses a regular atomic-scale chicken wire (hexagonal) pattern, large theoretical specific surface area (Qiu et al., 2011), high electrical conductivity (Marinho et al., 2012), and good thermal properties (Huang et al., 2012; Zhang et al., 2013). The CNTs are one-dimensional (1-D) carbon form and they are hollow tubes composed of sp2-hybridized carbon atoms with a diameter of a few nanometers and a length of approximately 10 μm. The combination between carbon nanomaterials and metal particles was also investigated by many authors in the literature (Liu et al., 2021; Quintero-Ruiz et al., 2021; Yang et al., 2021; Zhang et al., 2021; Ashok et al., 2022; Koçak and Dursun, 2022). It has been proved that the presence of carbon materials ensures a homogeneous dispersion of metal particles leading to an increase in the electrocatalytic performances of the electrocatalyst toward methanol oxidation in terms of current density and onset potential. However, the high synergy between conducting polymers and CNM has prompted further investigation into possible impacts of the combination of CNM/CP and metal particles on alcohol oxidation. In this context, several research groups have studied new catalyst supports based on a combination of CNMs and CPs for alcohols electrooxidation (Elbasri and Rhazi, 2015; Fard et al., 2017a; Halim et al., 2019b; Mozafari and Basiri Parsa, 2020). Polymers have been successfully combined with CNMs and exhibited synergistic properties such as a better stability, high mechanical strength, and high electrical conductivity (Fard et al., 2017a; Oularbi et al., 2017; Halim et al., 2019b). They can also ensure a good dispersion of metal particles for greater catalytic activity. Hence, PANI (Eris et al., 2018; Arukula et al., 2019; Eswaran et al., 2019; Zhang et al., 2020b; Mozafari and Basiri Parsa, 2020), PPy (Ashassi-Sorkhabi et al., 2016; Fard et al., 2017a; Boulaghi et al., 2018; Merati et al., 2018; Pattanayak et al., 2018; Mao et al., 2019; Sarkar et al., 2019), PPD (Halim et al., 2019b; Wu et al., 2019), Poly [2,2’-(2,6-pyridine)-5,5′-bibenzimidazole (PyPBI) (Yang and Nakashima, 2015; Yang et al., 2016), Poly (1,8-Diaminonaphtalene) (P-1,8-DAN) (Elbasri and Rhazi, 2015), combined with carbon nanomaterials, were extensively used as catalyst supports to metals electrocatalyst for alcohol oxidation. It turns out that such a combination is really a good idea to enhance the catalytic surface area and reduce the surface poisoning by the formation of M-OH at lower potential, which leads to obtaining an excellent durability (Sarma et al., 2017; Dai et al., 2018; Fan et al., 2019).
Among different methods used to develop this new kind of catalyst, the chemical and electrochemical methods are the most used ones. The detailed approaches employed for the preparation of CNMs/CPs nanocomposites have been previously reported in excellent papers (Oularbi et al., 2017; Deshmukh et al., 2018; Halim et al., 2019b; Tajik et al., 2020). In summary, the development of the hybrid nanocomposites CNMs/CPs/NPs can be performed in two different ways:
(i) The CNMs dispersion was added to the first step before the polymerization and the deposition of NPs (Boulaghi et al., 2018).
(ii) The CNMs were sonicated in situ along with the polymerization of the monomer and then followed by the preparation of NPs (Eswaran et al., 2019).
In this section, we will give a brief overview of the recent papers that examine the combination of carbon nanomaterials, conducting polymers, and metal nanoparticles as electrocatalysts for alcohol oxidation. Based on analysis in the literature, it seems that PPy and PANI remain the most widely used conducting polymers.
Carbon Nanomaterials/Polypyrrole/Metallic Particles
By taking into account the significant properties of PPy, many approaches have been adopted by many researchers to combine PPy, carbon nanomaterials, and metal particles as a new catalyst for alcohol oxidation (Ashassi-Sorkhabi et al., 2016; Merati et al., 2018; Sarkar et al., 2019; El Attar et al., 2022). Moe et al. developed a hierarchical NiS/Ni (OH)2@polypyrrole/graphene oxide nanosheets using a solvothermal method. The morphological studies underlined the influence of the PPy/GO in the hierarchical micro/nanostructures. They highlighted the role of the PPy chains, particularly the -NH- segments, in the coordination with Ni2+ and the easy formation of NiS. The catalytic performance of the catalyst toward the methanol oxidation reaction was investigated. The results showed a higher current at a more positive potential and a smaller transfer resistance compared with other catalysts, due to a synergetic effect between PPy, GO, and NiS/Ni(OH)2. Despite all these advantages, this catalyst remains unable to completely oxidize methanol due to the formation of acetic acid as a final product leading to 4 electrons at the end of the reaction (Mao et al., 2019). Using another transition metallic NPs, Pattanayak et al. developed a chemical method to synthetize Cu2O/PPy-GO NPs catalyst for methanol oxidation in acidic medium (Pattanayak et al., 2018). Cu2O NPs deposited on PPy/GO support presented a spherical structure dispersed uniformly with an average particle size of 7 nm which is tinier compared with Cu2O NPs/GO (17 nm). The obtained result was explained by the large surface area of PPy/GO, where the functional groups at the GO surface facilitate the interaction between GO and PPy, and the NPs trapping. The catalyst showed a higher current density (300 μA cm−2 at 0.7 V) compared with other catalysts owing to the good dispersion and distribution of the nanoparticles on the support materials. PPy/GO support offers several advantages such as a high conductivity and a large number of nucleation centers. The ratio between forward peak current and backward peak current (If/Ib), an important parameter to define the quantity of poisoning species adsorbed on the catalyst surface, was also studied confirming that Cu2O/PPy/GO reduce the poisoning of the surface. This catalyst shows the capability for a complete oxidation of methanol due to the conversion of acetic acid into CO2 (Pattanayak et al., 2018). In another work, Boulaghi et al. investigated the possibility of using Pt-Pd alloy electrodeposited on PPy and N-doped graphene oxide (NGO) surface for ethanol oxidation in alkaline medium. First, NGO was synthetized by pyrolysis of GO, and then, PPy-NGO hybrid support was formed by in-situ polymerization, followed by electrodeposition of spherical Pt-Pd particles with an average diameter of approximately 40 nm. The catalytic performance of the catalyst showed a high current density (131.58 mA cm−2) and a shift to the negative potential value (−0.6 V). The most important result in this study is the long-term stability and electrochemical activity toward EOR. The Pt-Pd/PPy/GO catalyst exhibited a good stability after 300 scans with a 30% decrease in current density, which is attributed to the consumption of ethanol or the adsorption of intermediate species on the electrode surface (Boulaghi et al., 2018).
Fard et al. examined the performance of PdCo porous nanostructures decorated on PPy@MWCNTs nanocomposite (Fard et al., 2017a). PPy/MWCNTs nanocomposite was prepared via in-situ emulsion polymerization and the PdCo PNS were prepared by chemical reduction. The introduction of MWCNTs in the PPy support allows a better performance for EOR by increasing the electronic transfer and thermal stability, whereas the presence of PdCo PNS leads to the increase in electrocatalytic activity performance and stability by reducing the poisoning of the electrode by the intermediate species.
Carbon Nanomaterials/Polyaniline/Metallic Particles
Polyaniline combined with CNMs has also been investigated as suitable support materials thanks to its promising properties such as good conductivity, structure accessibility, and high surface area which allow a good distribution of metallic nanoparticles (Eris et al., 2018; Ramli and Kamarudin, 2018; Arukula et al., 2019; Bora et al., 2019; Eswaran et al., 2019). Mozafari et al. developed Pd/PANI-MWCNTs-SnO2/Ti electrode for ethanol oxidation using the electrodeposition method (Mozafari and Basiri Parsa, 2020). The introduction of MWCNTs leads to major changes in the morphological structure, generating more surface area for better uniform dispersion of nanoparticles. The electrocatalytic performances of different electrodes were studied using the CV technique in the alkaline medium containing ethanol. An increase of 146% has been observed with a current value of 64.1 mA cm−2 and an onset potential of −0.725 V. It is worth noting that the If/Ib value is higher than other catalysts, indicating that only few species were adsorbed on the catalyst surface during the ethanol oxidation reaction. Moreover, the activity of Pd/PANI-MWCNTs-SnO2/Ti toward EOR showed a small decrease of current of 27% after 700 cycles due to the adsorption of CO on the catalyst surface. In another work, De et al. investigated the formation of Pt NPs on CNT/PANI for the oxidation of ethanol in the acidic medium. The CNT-PANI-Pt catalyst showed an excellent stability. These results were explained by the π-π stacking between the PANI and CNT, and also the efficient electrostatic interactions across the tightly packed CNT-PANI/Pt structure (De et al., 2017). PANI was also combined with electrochemically exfoliated graphene (EEG) using the solvothermal method as support for the Pt catalyst. The electrooxidation of methanol on EEG-PANI-Pt shows a higher current density of approximately 443.7 mA.mgpt−1 at 0.6 V compared with reduced graphene oxide electrodes. The improved catalytic activity of the catalyst was related to the synergistic effect between PANI and EEG, which balances the lack of nucleation sites on EEG that offers more active sites and ensures a well dispersion of Pt. Besides, the combination of EEG and PANI provides more N species for Pt nanocrystals growth, which enhances the electrocatalytic performance of Pt catalyst and increases the CO tolerance (Zhang et al., 2020b). One-step preparation of graphitic carbon nitride (g-C3N4)/PANI/PdNPs electrocatalyst was reported by Eswaran et al. for methanol oxidation in alkaline medium. The g-C3N4/PANI/PdNPs nanohybrid composite was simultaneously electrodeposited on the screen-printed electrode using the cyclic voltammetry technique in the acidic medium. The morphological characterization revealed that PANI exhibited a needle-like nanostructure and the Palladium nanoparticles possessed a spherical shape. The obtained composite showed a good stability and a higher current density of approximately 3.42 mA cm−2 at 0.3 V compared with the commercial catalyst (Eswaran et al., 2019).
Carbon Nanomaterials/Conducting Polymers/Metallic Particles
In addition to the polymers mentioned above, other works focus on several conducting polymers such as poly-phenylenediamine, poly [2,2’-(2,6-pyridine)-5,5′-bibenzimidazole, poly (1,8-diaminonaphtalene) as support catalysts (Elbasri and Rhazi, 2015; Yang and Nakashima, 2015; Wu et al., 2019; Halim et al., 2021b). Halim et al. synthesized the CPE/CNF/PpPD/NiPs catalyst for methanol oxidation in alkaline medium. First, the CNF was functionalized using the chemical method by thermal treatment in a mixture of acids. After dropping CNF on the CPE surface, pPD was electrodeposited using cyclic voltammetry leading to a very thin layer of the polymer. NiPs were then electrodeposited using a constant potential of −0.3 V for 45 s. The electrodeposition of nickel produced well NiPs with a particle size of 300 nm. The introduction of PpPD increases the catalytic response toward methanol which is probably due to the synergistic effect between the polymer and CNF (Halim et al., 2019b). A simple strategy for the preparation of PtRu nanoparticles on the PPDA-functionalized MWCTS was also reported by Wu et al. The nanoparticles size of PtRu deposited on PPDA-MWCNTs was approximately 3.5 nm. The improvement in the catalytic performance toward methanol oxidation was explained by the small size of nanoparticles, homogenous dispersion, and higher electrochemical surface offered by the developed catalysts (Wu et al., 2019).
Some examples of catalysts reported in the literature, their properties, and electrocatalytic performances are presented in Table 4.
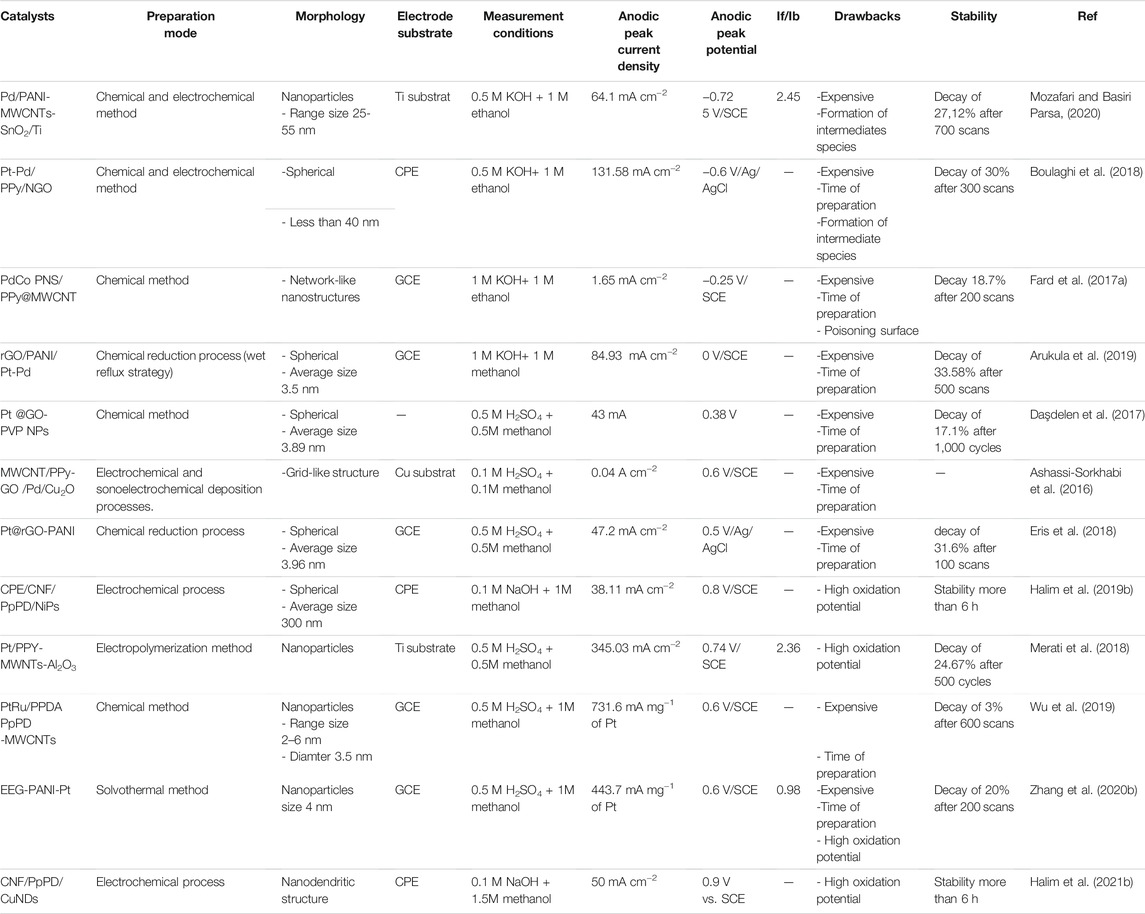
TABLE 4. Electrochemical properties and electrocatalytic performances of metallic-based conducting polymers and carbon materials catalyst applied for alcohol electrooxidation.
Summary and Future Perspectives
There is no doubt that direct alcohol fuel cells constitute a technological approach that has all demands for stable energy conversion with excellent electrical efficiency. As reported in this review, the recent decade has noticed a great discussion about the catalytic performance of anode catalysts, especially platinum, and has witnessed the growth of new catalysts based on inexpensive nanostructured materials. Since one of the most challenges in DAFCs is to prevent the poisoning of the electrode surface due to the slow kinetics of alcohol oxidation reaction, researchers focused their attention on the possible role of CPs as support in the design of new catalysts. CPs offer various advantages such as good electrical properties and high specific area, which lead to a uniform distribution of metal nanostructures with optimized loading and high number of catalytic sites. Various precious metals have been studied for electrocatalytic oxidation of methanol and ethanol, especially Pt, and high catalytic activity has been achieved. However, its high prices hinder the wide-scale commercialization of DAFCs and represent a major reason for studying inexpensive metals that exhibit promising performance. The review has started up with describing the commonly used methods for the synthesis of nanostructured materials. A number of strategies including chemical synthesis, interfacial polymerization method, hydrothermal method, electrochemical method, and the combination of these methods have been widely used to prepare nanostructured materials with a specific structure. The morphology of the prepared material turns out to have a crucial influence on its catalytic properties. Several parameters highly influence the morphology, which should be controlled during the preparation process such as concentration and pH of the precursor’s solution, reaction temperature. The preparation and development of hybrid materials containing CPs and nanostructured metals, with several morphologies, were detailed and explained. It seems that the nature and the mode of synthesis of polymer can affect strongly the morphology of deposited metals leading to an enhancement of catalytic performance. We can conclude that the best response in terms of catalytic activity when using CPs/and metallic particles was obtained with PANI and Pt. Only a decline of 20% was observed after 1,000 cycles (Yan et al., 2018). The combination of PANI with gold catalyst also provides an enhanced catalytic performance in terms of stability and durability (Pandey and Lakshminarayanan, 2012). The substitution of noble metals by transition metals that are more economical and abundant was investigated by many researchers. Recent papers using PPy with Copper or Nickel show that we can reach an excellent stability using electrochemical activation of the surface. It seems that the presence of CP ensures a good dispersion of metallic nanoparticles. The role of amino groups and thiol groups in the dispersion of metallic nanoparticles should be more examined.
Other researches have been devoted to develop bi-metallic and tri-metallic catalysts supported on conducting polymer, to take advantages of the good catalytic performance of noble metal, and reduce its loading. In addition, alloying noble metals (especially Pt) with highly oxophilic metals can oxidize the intermediate species (e.g., CO and CHO) generated during the catalytic process, thus mitigating the catalyst poisoning. As for ternary-supported conducting polymers, and based on the works presented above, the best catalytic performance in terms of catalytic activity and durability was found on Pd-Pt-Au supported on PPy. The structure of the nanohybrid at an average particle size of a few nanometers allowed an excellent mass transfer and demonstrated better tolerance toward intermediate poisoning for alcohol oxidation, highlighting its outstanding durability and recyclability. It is conspicuous that studies involving bi and trimetallic catalysts of varying lengths are of great interest to further understand the effect of a conjugated matrix on the electronic properties to avoid the adsorption of intermediate species.
A combination of conducting polymers and carbon nanomaterials used as substrates for metallic nanoparticles was also investigated and discussed in this review. The carbon nanostructures, varying from one-dimensional to two-dimensional nanofilms, to three-dimensional nanostructures, and even to hierarchical structures, have brought promising results with respect to their functions that are of high importance for their electrocatalytic efficiency. The location of metallic nanoparticles may be supported on surfaces or embedded inside CPs and/or carbon nanomaterials. Their morphology may be tuned from nanoparticles, nanorods, and nanoplates. Their size could be controlled depending on the synthesis technique and the experimental parameters adopted during the process and could reach a few nanometers. Notwithstanding, the synergetic interactions between the structural constituents in nanocomposite are still not disclosed in detail, requiring future research. The most promising findings in terms of catalytic activity and tolerance to intermediate species were found using PANI and PPy combined with GO and CNT. The small size of Pd nanoparticles exhibits a superior catalytic performance toward ethanol oxidation with only 27% current decay after 700 cycles. Conducting polymer–carbon nanomaterials based electrocatalyst demonstrated great performance in terms of stability and durability. Nonetheless, the conducting polymer/carbon nanomaterial nanocomposite support is required to be applied in real fuel cell setups to evaluate the practical capability of these systems.
The application and commercialization are the most challenging issue in the world of Direct Alcohol Fuel cells. In fact, both industrial and academic researchers need to work together closely to create sophisticated approaches to increase the performance of DAFC and foster commercialization (Ong et al., 2017). The estimation of the cost feasibility of the hybrid material reported in this review and the whole operation process should also be considered. This will warrant estimation of the performance-to-cost ratio of these nanocatalysts compared with the commercial Pt/C catalyst system. Hence, commercialization goals could be moved forward. Furthermore, conducting polymers and carbon nanomaterials should be investigated more, as most of the studies are being focused mainly on PANI, PPy, CNT, and CNF. More studies should be conducted on other polymers such as PDA, PT, and PPD. Regarding the catalyst synthesis approach, the electrochemical methods have to be considered in the design of new catalysts to reduce the cost and the time of preparation. Moreover, a drastic investigation should be carried out to explore the efficiency of the elaborated catalyst systems toward the oxidation of other types of fuels within DAFCs and other organic compounds. It is also very important to underline that there is an opportunity to expand the range of applications of this kind of materials to other fields such as reduction of CO2, supercapacitors, or reduction of oxygen.
Author Contributions
All authors were contributed in: Conceptualization, Methodology, Validation, Investigation, Formal analysis, Writing—Review and editing. ME: Conceptualization, Methodology, Validation, Writing—review and editing, Resources, Visualization, Project administration, Funding acquisition.
Funding
This work was supported by MESRSFC (Ministère de l’Enseignement Supérieur et de la Recherche Scientifique et de la Formation des cadres-Morocco) and CNRST (Centre National pour la Recherche Scientifique et Technique-Morocco) (project number PPR/2015/72).
Conflict of Interest
The authors declare that the research was conducted in the absence of any commercial or financial relationships that could be construed as a potential conflict of interest.
Publisher’s Note
All claims expressed in this article are solely those of the authors and do not necessarily represent those of their affiliated organizations, or those of the publisher, the editors and the reviewers. Any product that may be evaluated in this article, or claim that may be made by its manufacturer, is not guaranteed or endorsed by the publisher.
References
Abdolahi, A., Hamzah, E., Ibrahim, Z., and Hashim, S. (2012). Synthesis of Uniform Polyaniline Nanofibers through Interfacial Polymerization. Materials 5, 1487–1494. doi:10.3390/ma5081487
Afzal, A. B., Akhtar, M. J., Nadeem, M., and Hassan, M. M. (2009). Investigation of Structural and Electrical Properties of Polyaniline/Gold Nanocomposites. J. Phys. Chem. C 113, 17560–17565. doi:10.1021/jp902725d
Ahmed, M., and Dincer, I. (2011). A Review on Methanol Crossover in Direct Methanol Fuel Cells: Challenges and Achievements. Int. J. Energ. Res. 35, 1213–1228. doi:10.1002/er.1889
Akbarfallahi, S., Hosseini, S. R., and Ghasemi, S. (2020). Improved Electrocatalytic Activity of Ethanol Oxidation over Pd/TiO2 Nanofibers-Carbon Black Nanocomposite. Mater. Chem. Phys. 252, 123317. doi:10.1016/j.matchemphys.2020.123317
Ali Shah, A.-u. -H., Yasmeen, N., Rahman, G., and Bilal, S. (2017). High Electrocatalytic Behaviour of Ni Impregnated Conducting Polymer Coated Platinum and Graphite Electrodes for Electrooxidation of Methanol. Electrochimica Acta 224, 468–474. doi:10.1016/j.electacta.2016.12.085
Anothumakkool, B., Soni, R., Bhange, S. N., and Kurungot, S. (2015). Novel Scalable Synthesis of Highly Conducting and Robust PEDOT Paper for a High Performance Flexible Solid Supercapacitor. Energy Environ. Sci. 8, 1339–1347. doi:10.1039/C5EE00142K
Antolini, E. (2016). Iron-containing Platinum-Based Catalysts as Cathode and Anode Materials for Low-Temperature Acidic Fuel Cells: a Review. RSC Adv. 6, 3307–3325. doi:10.1039/C5RA22035A
Antolini, E. (2017). Pt-Ni and Pt-M-Ni (M = Ru, Sn) Anode Catalysts for Low-Temperature Acidic Direct Alcohol Fuel Cells: A Review. Energies 10, 42. doi:10.3390/en10010042
Arjona, N., Trejo, G., Ledesma-García, J., Arriaga, L. G. L., and Guerra-Balcázar, M. (2016). An Electrokinetic-Combined Electrochemical Study of the Glucose Electro-Oxidation Reaction: Effect of Gold Surface Energy. RSC Adv. 6, 15630–15638. doi:10.1039/C5RA23780G
Arukula, R., Vinothkannan, M., Kim, A. R., and Yoo, D. J. (2019). Cumulative Effect of Bimetallic alloy, Conductive Polymer and Graphene toward Electrooxidation of Methanol: An Efficient Anode Catalyst for Direct Methanol Fuel Cells. J. Alloys Compd. 771, 477–488. doi:10.1016/j.jallcom.2018.08.303
Ashassi-Sorkhabi, H., Rezaei-Moghadam, B., Asghari, E., Bagheri, R., and Kabiri, R. (2016). Sonoelectrosynthesized Polypyrrole-Graphene Oxide Nanocomposite Modified by Carbon Nanotube and Cu2O Nanoparticles on Copper Electrode for Electrocatalytic Oxidation of Methanol. J. Taiwan Inst. Chem. Eng. 69, 118–130. doi:10.1016/j.jtice.2016.08.026
Ashok, A., Kumar, A., Yuda, A., and Al Ashraf, A. (2022). Highly Efficient Methanol Oxidation Reaction on Durable Co9S8 @N, S-Doped CNT Catalyst for Methanol Fuel Cell Applications. Int. J. Hydrogen Energ. 47, 3346–3357. doi:10.1016/j.ijhydene.2021.07.026
Bagheri, H., and Banihashemi, S. (2014). Silver Nanoparticles-Polyaniline Nanocomposite for Microextraction in Packed Syringe. Chromatographia 77, 397–403. doi:10.1007/s10337-014-2628-6
Bai, Z., Zhang, Q., Lv, J., Chao, S., Yang, L., and Qiao, J. (2015). A Facile Preparation of Palladium Catalysts Supported on Hollow Polypyrrole Nanospheres for Ethanol Oxidation. Electrochimica Acta 177, 107–112. doi:10.1016/j.electacta.2015.01.126
Bedre, M. D., Basavaraja, S., Deshpande, R., Balaji, D. S., and Venkataraman, A. (2010). Preparation and Characterization of Polypyrrole Silver Nanocomposites via Interfacial Polymerization. Int. J. Polymeric Mater. 59, 531–543. doi:10.1080/00914031003760642
Begum, S., Hassan, Z., Bräse, S., Wöll, C., and Tsotsalas, M. (2019). Metal-Organic Framework-Templated Biomaterials: Recent Progress in Synthesis, Functionalization, and Applications. Acc. Chem. Res. 52, 1598–1610. doi:10.1021/acs.accounts.9b00039
Bogdanović, U., Pašti, I., Ćirić-Marjanović, G., Mitrić, M., Ahrenkiel, S. P., and Vodnik, V. (2015). Interfacial Synthesis of Gold-Polyaniline Nanocomposite and its Electrocatalytic Application. ACS Appl. Mater. Inter. 7, 28393–28403. doi:10.1021/acsami.5b09145
Bogdanović, U., Vodnik, V. V., Ahrenkiel, S. P., Stoiljković, M., Ćirić-Marjanović, G., and Nedeljković, J. M. (2014). Interfacial Synthesis and Characterization of Gold/polyaniline Nanocomposites. Synth. Met. 195, 122–131. doi:10.1016/j.synthmet.2014.05.018
Bora, M. A., Adhav, P. B., Diwate, B. B., Pawar, D. S., Dallavalle, S., and Chabukswar, V. V. (2019). Room Temperature Operating Sensitive and Reproducible Ammonia Sensor Based on PANI/hematite Nanocomposite. Polymer-Plastics Technol. Mater. 58, 1545–1555. doi:10.1080/25740881.2018.1563131
Boulaghi, M., Ghafouri Taleghani, H., Soleimani Lashkenari, M., and Ghorbani, M. (2018). Platinum-palladium Nanoparticles-Loaded on N-Doped Graphene Oxide/polypyrrole Framework as a High Performance Electrode in Ethanol Oxidation Reaction. Int. J. Hydrogen Energ. 43, 15164–15175. doi:10.1016/j.ijhydene.2018.06.092
Caballero-Manrique, G., Garcia-Cardona, J., Brillas, E., Jaén, J. A., Sánchez, J. M., and Cabot, P. L. (2020). Synthesis and Evaluation of PtNi Electrocatalysts for CO and Methanol Oxidation in Low Temperature Fuel Cells. Catalysts 10, 563. doi:10.3390/catal10050563
Çağlar, A., Aldemir, A., and Kivrak, H. (2018). Alcohol Electrooxidation Study on Carbon Nanotube Supported Monometallic, Pt, Bi, and Ru Catalysts. Fullerenes, Nanotubes and Carbon Nanostructures 26, 863–870. doi:10.1080/1536383X.2018.1516641
Caglar, A., and Kivrak, H. (2019). Highly Active Carbon Nanotube Supported PdAu alloy Catalysts for Ethanol Electrooxidation in Alkaline Environment. Int. J. Hydrogen Energ. 44, 11734–11743. doi:10.1016/j.ijhydene.2019.03.118
Çelik, B., Başkaya, G., Sert, H., Karatepe, Ö., Erken, E., and Şen, F. (2016). Monodisperse Pt(0)/DPA@GO Nanoparticles as Highly Active Catalysts for Alcohol Oxidation and Dehydrogenation of DMAB. Int. J. Hydrogen Energ. 41, 5661–5669. doi:10.1016/j.ijhydene.2016.02.061
Chauhan, D., Afreen, S., Talreja, N., and Ashfaq, M. (2020). “Multifunctional Copper Polymer-Based Nanocomposite for Environmental and Agricultural Applications,” in Multifunctional Hybrid Nanomaterials for Sustainable Agri-Food and Ecosystems Micro and Nano Technologies. Editor K. A. Abd-Elsalam (Amsterdam, Netherlands: Elsevier), 189–211. doi:10.1016/B978-0-12-821354-4.00008-X
Chemchoub, S., Elbasri, M., Halim, E. M., and El Rhazi, M. (2019). The Electrocatalytic Oxidation of Methanol on a Carbon Paste Electrode Modified by Poly(para-Phenylenediamine) and Nickel Particles. Mater. Today Proc. 13, 720–729. doi:10.1016/j.matpr.2019.04.033
Chemchoub, S., Oularbi, L., El Attar, A., Younssi, S. A., Bentiss, F., Jama, C., et al. (2020). Cost-effective Non-noble Metal Supported on Conducting Polymer Composite Such as Nickel Nanoparticles/polypyrrole as Efficient Anode Electrocatalyst for Ethanol Oxidation. Mater. Chem. Phys. 250, 123009. doi:10.1016/j.matchemphys.2020.123009
Cho, W., Park, S.-J., and Kim, S. (2011). Effect of Monomer Concentration on Interfacial Synthesis of Platinum Loaded Polyaniline Nanocomplex Using Poly(styrene Sulfonic Acid). Synth. Met. 161, 2446–2450. doi:10.1016/j.synthmet.2011.09.025
Christensen, P. A., Jones, S. W. M., and Hamnett, A. (2012). In SituFTIR Studies of Ethanol Oxidation at Polycrystalline Pt in Alkaline Solution. J. Phys. Chem. C 116, 24681–24689. doi:10.1021/jp308783y
Chu, Y. H., and Shul, Y. G. (2010). Combinatorial Investigation of Pt-Ru-Sn Alloys as an Anode Electrocatalysts for Direct Alcohol Fuel Cells. Int. J. Hydrogen Energ. 35, 11261–11270. doi:10.1016/j.ijhydene.2010.07.062
Corti, H. R., and Gonzalez, E. R. (2014). Direct Alcohol Fuel Cells: Materials, Performance, Durability and Applications. Berlin/Heidelberg, Germany: Springer, 1–32. doi:10.1007/978-94-007-7708-8_1Introduction to Direct Alcohol Fuel Cells
Dai, L.-X., Wang, X.-Y., Yang, S.-S., Zhang, T., Ren, P.-J., Ye, J.-Y., et al. (2018). Intrinsic Composition and Electronic Effects of Multicomponent Platinum Nanocatalysts with High Activity and Selectivity for Ethanol Oxidation Reaction. J. Mater. Chem. A. 6, 11270–11280. doi:10.1039/C8TA03320J
Dallas, P., Niarchos, D., Vrbanic, D., Boukos, N., Pejovnik, S., Trapalis, C., et al. (2007). Interfacial Polymerization of Pyrrole and In Situ Synthesis of Polypyrrole/silver Nanocomposites. Polymer 48, 2007–2013. doi:10.1016/j.polymer.2007.01.058
Darr, J. A., Zhang, J., Makwana, N. M., and Weng, X. (2017). Continuous Hydrothermal Synthesis of Inorganic Nanoparticles: Applications and Future Directions. Chem. Rev. 117, 11125–11238. doi:10.1021/acs.chemrev.6b00417
Das, A. K., Paria, S., Maitra, A., Halder, L., Bera, A., Bera, R., et al. (2019). Highly Rate Capable Nanoflower-like NiSe and WO3@PPy Composite Electrode Materials toward High Energy Density Flexible All-Solid-State Asymmetric Supercapacitor. ACS Appl. Electron. Mater. 1, 977–990. doi:10.1021/acsaelm.9b00164
Das, S., Dutta, K., Kundu, P. P., and Bhattacharya, S. K. (2018). Nanostructured Polyaniline: An Efficient Support Matrix for Platinum-Ruthenium Anode Catalyst in Direct Methanol Fuel Cell. Fuel Cells 18, 369–378. doi:10.1002/fuce.201700201
Das, S., Dutta, K., and Kundu, P. P. (2015). Nickel Nanocatalysts Supported on Sulfonated Polyaniline: Potential toward Methanol Oxidation and as Anode Materials for DMFCs. J. Mater. Chem. A. 3, 11349–11357. doi:10.1039/C5TA01837D
Das, S., Dutta, K., and Kundu, P. P. (2016). Sulfonated Polypyrrole Matrix Induced Enhanced Efficiency of Ni Nanocatalyst for Application as an Anode Material for DMFCs. Mater. Chem. Phys. 176, 143–151. doi:10.1016/j.matchemphys.2016.03.046
Daşdelen, Z., Yıldız, Y., Eriş, S., and Şen, F. (2017). Enhanced Electrocatalytic Activity and Durability of Pt Nanoparticles Decorated on GO-PVP Hybride Material for Methanol Oxidation Reaction. Appl. Catal. B: Environ. 219, 511–516. doi:10.1016/j.apcatb.2017.08.014
Datta, J., Dutta, A., and Biswas, M. (2012). Enhancement of Functional Properties of PtPd Nano Catalyst in Metal-Polymer Composite Matrix: Application in Direct Ethanol Fuel Cell. Electrochemistry Commun. 20, 56–59. doi:10.1016/j.elecom.2012.02.022
De, A., Adhikary, R., and Datta, J. (2017). Proactive Role of Carbon Nanotube-Polyaniline Conjugate Support for Pt Nano-Particles toward Electro-Catalysis of Ethanol in Fuel Cell. Int. J. Hydrogen Energ. 42, 25316–25325. doi:10.1016/j.ijhydene.2017.08.073
Deshmukh, M. A., Shirsat, M. D., Ramanaviciene, A., and Ramanavicius, A. (2018). Composites Based on Conducting Polymers and Carbon Nanomaterials for Heavy Metal Ion Sensing (Review). Crit. Rev. Anal. Chem. 48, 293–304. doi:10.1080/10408347.2017.1422966
Dimitrijevic, N. M., Tepavcevic, S., Liu, Y., Rajh, T., Silver, S. C., and Tiede, D. M. (2013). Nanostructured TiO2/Polypyrrole for Visible Light Photocatalysis. J. Phys. Chem. C 117, 15540–15544. doi:10.1021/jp405562b
Dutta, A., Mondal, A., and Datta, J. (2015). Tuning of Platinum Nano-Particles by Au Usage in Their Binary alloy for Direct Ethanol Fuel Cell: Controlled Synthesis, Electrode Kinetics and Mechanistic Interpretation. J. Power Sourc. 283, 104–114. doi:10.1016/j.jpowsour.2015.01.113
Dutta, K., and Kundu, P. P. (2014). A Review on Aromatic Conducting Polymers-Based Catalyst Supporting Matrices for Application in Microbial Fuel Cells. Polym. Rev. 54, 401–435. doi:10.1080/15583724.2014.881372
Dutta, K. (2020). “Polymeric Nanomaterials in Fuel Cell Applications,” in Nanostructured, Functional, and Flexible Materials for Energy Conversion and Storage Systems. Editors A. Pandikumar, and P. Rameshkumar (Amsterdam, Netherlands: Elsevier), 105–129. doi:10.1016/B978-0-12-819552-9.00004-X
Dwivedi, C., Chaudhary, A., Srinivasan, S., and Nandi, C. K. (2018). Polymer Stabilized Bimetallic Alloy Nanoparticles: Synthesis and Catalytic Application. Colloid Interf. Sci. Commun. 24, 62–67. doi:10.1016/j.colcom.2018.04.001
Ehsani, A., Mahjani, M. G., Babaei, F., and Mostaanzadeh, H. (2015). Physioelectrochemical and DFT Investigation of Metal Oxide/p-type Conductive Polymer Nanoparticles as an Efficient Catalyst for the Electrocatalytic Oxidation of Methanol. RSC Adv. 5, 30394–30404. doi:10.1039/C5RA02297E
El Attar, A., Chemchoub, S., Diallo Kalan, M., Oularbi, L., and El Rhazi, M. (2022). Designing New Material Based on Functionalized Multi-Walled Carbon Nanotubes and Cu(OH)2–Cu2O/Polypyrrole Catalyst for Ethanol Oxidation in Alkaline Medium. Front. Chem. 9, 2296–2646. Available at: https://www.frontiersin.org/article/10.3389/fchem.2021.805654. doi:10.3389/fchem.2021.805654
El Attar, A., Oularbi, L., Chemchoub, S., and El Rhazi, M. (2021). Effect of Electrochemical Activation on the Performance and Stability of Hybrid (PPy/Cu2O Nanodendrites) for Efficient Ethanol Oxidation in Alkaline Medium. J. Electroanalytical Chem. 885, 115042. doi:10.1016/j.jelechem.2021.115042
El Attar, A., Oularbi, L., Chemchoub, S., and El Rhazi, M. (2020). Preparation and Characterization of Copper Oxide Particles/polypyrrole (Cu2O/PPy) via Electrochemical Method: Application in Direct Ethanol Fuel Cell. Int. J. Hydrogen Energ. 45, 8887–8898. doi:10.1016/j.ijhydene.2020.01.008
El Rhazi, M., Majid, S., Elbasri, M., Salih, F. E., Oularbi, L., and Lafdi, K. (2018). Recent Progress in Nanocomposites Based on Conducting Polymer: Application as Electrochemical Sensors. Int. Nano Lett. 8, 79–99. doi:10.1007/s40089-018-0238-2
Elbasri, M., and Rhazi, M. E. (2015). Preparation and Characterization of Carbon Paste Electrode Modified by Poly(1,8-Diaminonaphthalene) and Nickel Ions Particles: Application to Electrocatalytic Oxidation of Methanol. Mater. Today Proc. 2, 4676–4683. doi:10.1016/j.matpr.2015.09.022
Eris, S., Daşdelen, Z., Yıldız, Y., and Sen, F. (2018). Nanostructured Polyaniline-rGO Decorated Platinum Catalyst with Enhanced Activity and Durability for Methanol Oxidation. Int. J. Hydrogen Energ. 43, 1337–1343. doi:10.1016/j.ijhydene.2017.11.051
Eswaran, M., Dhanusuraman, R., Tsai, P.-C., and Ponnusamy, V. K. (2019). One-step Preparation of Graphitic Carbon nitride/Polyaniline/Palladium Nanoparticles Based Nanohybrid Composite Modified Electrode for Efficient Methanol Electro-Oxidation. Fuel 251, 91–97. doi:10.1016/j.fuel.2019.04.040
Fan, L.-Z., and Maier, J. (2006). High-performance Polypyrrole Electrode Materials for Redox Supercapacitors. Electrochemistry Commun. 8, 937–940. doi:10.1016/j.elecom.2006.03.035
Fan, X., Tang, M., Wu, X., Luo, S., Chen, W., Song, X., et al. (2019). SnO2 Patched Ultrathin PtRh Nanowires as Efficient Catalysts for Ethanol Electrooxidation. J. Mater. Chem. A. 7, 27377–27382. doi:10.1039/C9TA10941B
Fang, X., Wang, L., Shen, P. K., Cui, G., and Bianchini, C. (2010). An In Situ Fourier Transform Infrared Spectroelectrochemical Study on Ethanol Electrooxidation on Pd in Alkaline Solution. J. Power Sourc. 195, 1375–1378. doi:10.1016/j.jpowsour.2009.09.025
Fard, L. A., Ojani, R., Raoof, J. B., Zare, E. N., and Lakouraj, M. M. (2017a). PdCo Porous Nanostructures Decorated on Polypyrrole @ MWCNTs Conductive Nanocomposite-Modified Glassy Carbon Electrode as a Powerful Catalyst for Ethanol Electrooxidation. Appl. Surf. Sci. 401, 40–48. doi:10.1016/j.apsusc.2016.12.240
Fard, L. A., Ojani, R., Raoof, J. B., Zare, E. N., and Lakouraj, M. M. (2017b). Poly (Pyrrole- Co -aniline) Hollow Nanosphere Supported Pd Nanoflowers as High-Performance Catalyst for Methanol Electrooxidation in Alkaline media. Energy 127, 419–427. doi:10.1016/j.energy.2017.03.159
Feng, Y., Jiao, T., Yin, J., Zhang, L., Zhang, L., Zhou, J., et al. (2019). Facile Preparation of Carbon Nanotube-Cu2O Nanocomposites as New Catalyst Materials for Reduction of P-Nitrophenol. Nanoscale Res. Lett. 14, 78. doi:10.1186/s11671-019-2914-1
Gajendran, P., and Saraswathi, R. (2013). Electrocatalytic Performance of Poly(o-Phenylenediamine)-Pt-Ru Nanocomposite for Methanol Oxidation. J. Solid State. Electrochem. 17, 2741–2747. doi:10.1007/s10008-013-2155-7
Geng, D., Zhu, S., Chai, M., Zhang, Z., Fan, J., Xu, Q., et al. (2020). PdxFey alloy Nanoparticles Decorated on Carbon Nanofibers with Improved Electrocatalytic Activity for Ethanol Electrooxidation in Alkaline media. New J. Chem. 44, 5023–5032. doi:10.1039/C9NJ06086C
Ghosh, S., and Basu, R. N. (2019). Polymer-based Nanocomposites for Direct Alcohol Fuel Cells. Nanomater. Alcohol. Fuel Cell 49, 271–292.
Ghosh, S., Bera, S., Bysakh, S., and Basu, R. N. (2017a). Highly Active Multimetallic Palladium Nanoalloys Embedded in Conducting Polymer as Anode Catalyst for Electrooxidation of Ethanol. ACS Appl. Mater. Inter. 9, 33775–33790. doi:10.1021/acsami.7b08327
Ghosh, S., Bhandary, N., Basu, S., and Basu, R. N. (2017b). Synergistic Effects of Polypyrrole Nanofibers and Pd Nanoparticles for Improved Electrocatalytic Performance of Pd/PPy Nanocomposites for Ethanol Oxidation. Electrocatalysis 8, 329–339. doi:10.1007/s12678-017-0374-x
Gorgojo, P., Jimenez-Solomon, M. F., and Livingston, A. G. (2014). Polyamide Thin Film Composite Membranes on Cross-Linked Polyimide Supports: Improvement of RO Performance via Activating Solvent. Desalination 344, 181–188. doi:10.1016/j.desal.2014.02.009
Guan, H., Fan, L.-Z., Zhang, H., and Qu, X. (2010). Polyaniline Nanofibers Obtained by Interfacial Polymerization for High-Rate Supercapacitors. Electrochimica Acta 56, 964–968. doi:10.1016/j.electacta.2010.09.078
Guo, J., Chen, R., Zhu, F.-C., Sun, S.-G., and Villullas, H. M. (2018). New Understandings of Ethanol Oxidation Reaction Mechanism on Pd/C and Pd2Ru/C Catalysts in Alkaline Direct Ethanol Fuel Cells. Appl. Catal. B: Environ. 224, 602–611. doi:10.1016/j.apcatb.2017.10.037
Guo, Y., Xu, Y.-T., Gao, G.-H., Wang, T., Zhao, B., Fu, X.-Z., et al. (2015). Electro-oxidation of Formaldehyde and Methanol over Hollow Porous Palladium Nanoparticles with Enhanced Catalytic Activity. Catal. Commun. 58, 40–45. doi:10.1016/j.catcom.2014.08.034
Habas, S. E., Lee, H., Radmilovic, V., Somorjai, G. A., and Yang, P. (2007). Shaping Binary Metal Nanocrystals through Epitaxial Seeded Growth. Nat. Mater 6, 692–697. doi:10.1038/nmat1957
Halim, E. M., Demir-Cakan, R., Debiemme-Chouvy, C., Perrot, H., El Rhazi, M., and Sel, O. (2021a). Poly(ortho-phenylenediamine) Overlaid Fibrous Carbon Networks Exhibiting a Synergistic Effect for Enhanced Performance in Hybrid Micro Energy Storage Devices. J. Mater. Chem. A. 9, 10487–10496. doi:10.1039/D1TA00763G
Halim, E. M., Demir-Cakan, R., Perrot, H., El Rhazi, M., and Sel, O. (2019a). Correlation between the Interfacial Ion Dynamics and Charge Storage Properties of Poly(ortho-Phenylenediamine) Electrodes Exhibiting High Cycling Stability. J. Power Sourc. 438, 227032. doi:10.1016/j.jpowsour.2019.227032
Halim, E. M., Elbasri, M., Perrot, H., Sel, O., Lafdi, K., and El Rhazi, M. (2019b). Synthesis of Carbon Nanofibers/poly(para-Phenylenediamine)/nickel Particles Nanocomposite for Enhanced Methanol Electrooxidation. Int. J. Hydrogen Energ. 44, 24534–24545. doi:10.1016/j.ijhydene.2019.07.141
Halim, E. M., Perrot, H., Sel, O., Debiemme-Chouvy, C., Lafdi, K., and El Rhazi, M. (2021b). Electrosynthesis of Hierarchical Cu2O-Cu(OH)2 Nanodendrites Supported on Carbon Nanofibers/poly(para-Phenylenediamine) Nanocomposite as High-Efficiency Catalysts for Methanol Electrooxidation. Int. J. Hydrogen Energ. 46, 19926–19938. doi:10.1016/j.ijhydene.2021.03.119
Han, J., Wang, M., Hu, Y., Zhou, C., and Guo, R. (2017). Conducting Polymer-noble Metal Nanoparticle Hybrids: Synthesis Mechanism Application. Prog. Polym. Sci. 70, 52–91. doi:10.1016/j.progpolymsci.2017.04.002
Han, S. H., Liu, H. M., Chen, P., Jiang, J. X., and Chen, Y. (2018). Porous Trimetallic PtRhCu Cubic Nanoboxes for Ethanol Electrooxidation. Adv. Energ. Mater. 8, 1801326. doi:10.1002/aenm.201801326
Harish, S., Mathiyarasu, J., Phani, K. L. N., and Yegnaraman, V. (2008). PEDOT/Palladium Composite Material: Synthesis, Characterization and Application to Simultaneous Determination of Dopamine and Uric Acid. J. Appl. Electrochem. 38, 1583–1588. doi:10.1007/s10800-008-9609-0
Hassan, H. B., and Tammam, R. H. (2018). Preparation of Ni-Metal Oxide Nanocomposites and Their Role in Enhancing the Electro-Catalytic Activity towards Methanol and Ethanol. Solid State Ionics 320, 325–338. doi:10.1016/j.ssi.2018.03.020
Horii, T., Hikawa, H., Katsunuma, M., and Okuzaki, H. (2018). Synthesis of Highly Conductive PEDOT:PSS and Correlation with Hierarchical Structure. Polymer 140, 33–38. doi:10.1016/j.polymer.2018.02.034
Hou, J., Zhang, L., Qiu, H., Duan, W., Wang, X., Wan, X., et al. (2017). Fabrication and Microwave Absorption Performances of Hollow-Structure Fe3O4/PANI Microspheres. J. Mater. Sci. Mater. Electron. 28, 9279–9288. doi:10.1007/s10854-017-6664-5
Huang, H., Sun, D., and Wang, X. (2012). PtCo alloy Nanoparticles Supported on Graphene Nanosheets with High Performance for Methanol Oxidation. Chin. Sci. Bull. 57, 3071–3079. doi:10.1007/s11434-012-5327-4
Huang, L.-M., Liao, W.-H., Ling, H.-C., and Wen, T.-C. (2009). Simultaneous Synthesis of Polyaniline Nanofibers and Metal (Ag and Pt) Nanoparticles. Mater. Chem. Phys. 116, 474–478. doi:10.1016/j.matchemphys.2009.04.035
Huang, M., Dong, G., Wang, N., Xu, J., and Guan, L. (2011). Highly Dispersive Pt Atoms on the Surface of RuNi Nanoparticles with Remarkably Enhanced Catalytic Performance for Ethanol Oxidation. Energ. Environ. Sci. 4, 4513–4516. doi:10.1039/C1EE02044G
Hvolbæk, B., Janssens, T. V. W., Clausen, B. S., Falsig, H., Christensen, C. H., and Nørskov, J. K. (2007). Catalytic Activity of Au Nanoparticles. Nano Today 2, 14–18. doi:10.1016/S1748-0132(07)70113-5
Inagaki, C. S., Oliveira, M. M., and Zarbin, A. J. G. (2018). Direct and One-step Synthesis of Polythiophene/gold Nanoparticles Thin Films through Liquid/liquid Interfacial Polymerization. J. Colloid Interf. Sci. 516, 498–510. doi:10.1016/j.jcis.2018.01.076
Ivanova, N. M., Soboleva, E. A., and Visurkhanova, Y. A. (2016). Bimetallic Co-cu Polyaniline Composites: Structure and Electrocatalytic Activity. Russ. J. Appl. Chem. 89, 1072–1081. doi:10.1134/S1070427216070053
Jain, M., and Annapoorni, S. (2010). Raman Study of Polyaniline Nanofibers Prepared by Interfacial Polymerization. Synth. Met. 160, 1727–1732. doi:10.1016/j.synthmet.2010.06.008
Jin, L., Xu, H., Chen, C., Shang, H., Wang, Y., Wang, C., et al. (2019). Three-dimensional PdCuM (M = Ru, Rh, Ir) Trimetallic Alloy Nanosheets for Enhancing Methanol Oxidation Electrocatalysis. ACS Appl. Mater. Inter. 11, 42123–42130. doi:10.1021/acsami.9b13557
Jin, W., Han, L., Han, X., Zhang, B., and Xu, P. (2016). Interfacial Synthesis of Lollipop-like Au-Polyaniline Nanocomposites for Catalytic Applications. RSC Adv. 6, 81983–81988. doi:10.1039/C6RA15446H
Junejo, Y., Baykal, A., and Sözeri, H. (2013). Simple Hydrothermal Synthesis of Fe3O4-PEG Nanocomposite. Cent. Eur. J. Chem. 11, 1527–1532. doi:10.2478/s11532-013-0281-9
Kamarudin, M. Z. F., Kamarudin, S. K., Masdar, M. S., and Daud, W. R. W. (2013). Review: Direct Ethanol Fuel Cells. Int. J. Hydrogen Energ. 38, 9438–9453. doi:10.1016/j.ijhydene.2012.07.059
Kamarudin, S. K., Daud, W. R. W., Ho, S. L., and Hasran, U. A. (2007). Overview on the Challenges and Developments of Micro-direct Methanol Fuel Cells (DMFC). J. Power Sourc. 163, 743–754. doi:10.1016/j.jpowsour.2006.09.081
Kanaujia, P. K., Adnan, M., Dehury, K. M., and Prakash, G. V. (2020). Facile Growth and Re-crystallization of Polymer-Based Inorganic-Organic 2D Hybrid Composites and Their Applications. J. Alloys Compd. 829, 154550. doi:10.1016/j.jallcom.2020.154550
Karim, N. A., and Kamarudin, S. K. (2021). “Introduction to Direct Alcohol Fuel Cells (DAFCs),” in Direct Liquid Fuel Cells. Editors R. G. Akay, and A. B. Yurtcan (Cambridge, Massachusetts, United States: Academic Press), 49–70. doi:10.1016/B978-0-12-818624-4.00002-9
Kayser, L. V., and Lipomi, D. J. (2019). Stretchable Conductive Polymers and Composites Based on PEDOT and PEDOT:PSS. Adv. Mater. 31, 1806133. doi:10.1002/adma.201806133
Khakpour, R., Peyravi, M., Jahanshahi, M., and Lashkenari, M. S. (2019). A New Durable and High Performance Platinum Supported on Ag-Ni-Porous Coordination Polymer as an Anodic DMFC Nano-Electrocatalyst: DFT and Experimental Investigation. Int. J. Hydrogen Energ. 44, 26530–26544. doi:10.1016/j.ijhydene.2019.08.101
Khalafallah, D., Alothman, O. Y., Fouad, H., and Abdelrazek Khalil, K. (2018). Hierarchical Co3O4 Decorated PPy Nanocasting Core-Shell Nanospheres as a High Performance Electrocatalysts for Methanol Oxidation. Int. J. Hydrogen Energ. 43, 2742–2753. doi:10.1016/j.ijhydene.2017.12.091
Khalifeh-Soltani, M. S., Shams, E., and Sharifi, E. (2020). Pt-Ru Nanoparticles Anchored on Poly(brilliant Cresyl Blue) as a New Polymeric Support: Application as an Efficient Electrocatalyst in Methanol Oxidation Reaction. Int. J. Hydrogen Energ. 45, 849–860. doi:10.1016/j.ijhydene.2019.10.071
Khammarnia, S., Akbari, A., Ekrami-Kakhki, M.-S., and Saffari, J. (2020). Enhanced Catalytic Activity of Pt-NdFeO3 Nanoparticles Supported on Polyaniline-Chitosan Composite towards Methanol Electro-Oxidation Reaction. J. Nanostructures 10, 239–257. doi:10.22052/JNS.2020.02.005
Khan, A. L., and Jain, R. (2018). Polypyrrole/titanium Dioxide Nanocomposite Sensor for the Electrocatalytic Quantification of Sulfamoxole. Ionics 24, 2473–2488. doi:10.1007/s11581-017-2365-6
Koçak, Ç. C., and Dursun, Z. (2022). Enhanced Electrocatalytic Oxidation of Methanol on Au-Pt Bimetallic Particles Modified Copper Phthalocyanine-Carbon Nanotube Composite Electrode. Mater. Sci. Eng. B 275, 115485. doi:10.1016/j.mseb.2021.115485
Kumar, A., Sharma, K., and Dixit, A. R. (2019). A Review of the Mechanical and thermal Properties of Graphene and its Hybrid Polymer Nanocomposites for Structural Applications. J. Mater. Sci. 54, 5992–6026. doi:10.1007/s10853-018-03244-3
Kundu, S., Satpati, B., Mukherjee, M., Kar, T., and Pradhan, S. K. (2017). Hydrothermal Synthesis of Polyaniline Intercalated Vanadium Oxide Xerogel Hybrid Nanocomposites: Effective Control of Morphology and Structural Characterization. New J. Chem. 41, 3634–3645. doi:10.1039/C7NJ00372B
Lai, S. C. S., and Koper, M. T. M. (2009). Ethanol Electro-Oxidation on Platinum in Alkaline media. Phys. Chem. Chem. Phys. 11, 10446–10456. doi:10.1039/B913170A
Lamy, C., Lima, A., LeRhun, V., Delime, F., Coutanceau, C., and Léger, J.-M. (2002). Recent Advances in the Development of Direct Alcohol Fuel Cells (DAFC). J. Power Sourc. 105, 283–296. doi:10.1016/s0378-7753(01)00954-5
Lee, J., Hill, A., and Kentish, S. (2013). Formation of a Thick Aromatic Polyamide Membrane by Interfacial Polymerisation. Separat. Purif. Technol. 104, 276–283. doi:10.1016/j.seppur.2012.11.015
Lee, K. P., Zheng, J., Bargeman, G., Kemperman, A. J. B., and Benes, N. E. (2015). pH Stable Thin Film Composite Polyamine Nanofiltration Membranes by Interfacial Polymerisation. J. Membr. Sci. 478, 75–84. doi:10.1016/j.memsci.2014.12.045
Lei, J., Lu, X., Nie, G., Jiang, Z., and Wang, C. (2015). One-Pot Synthesis of Algae-like MoS2 /PPy Nanocomposite: A Synergistic Catalyst with Superior Peroxidase-like Catalytic Activity for H2 O2 Detection. Part. Part. Syst. Charact. 32, 886–892. doi:10.1002/ppsc.201500043
Li, K., Guo, D., Chen, J., Kong, Y., and Xue, H. (2015). Oil-water Interfacial Synthesis of Graphene-Polyaniline-MnO2 Hybrids Using Binary Oxidant for High Performance Supercapacitor. Synth. Met. 209, 555–560. doi:10.1016/j.synthmet.2015.09.017
Li, Y., Ban, H., Jiao, M., and Yang, M. (2016a). In Situ growth of SnO2 Nanosheets on a Substrate via Hydrothermal Synthesis Assisted by Electrospinning and the Gas Sensing Properties of SnO2/polyaniline Nanocomposites. RSC Adv. 6, 74944–74956. doi:10.1039/C6RA10280H
Li, Y., Ban, H., and Yang, M. (2016b). Highly Sensitive NH3 Gas Sensors Based on Novel Polypyrrole-Coated SnO2 Nanosheet Nanocomposites. Sensors Actuators B: Chem. 224, 449–457. doi:10.1016/j.snb.2015.10.078
Li, Y., Wang, L., Low, J., Wu, D., Hu, C., Jiang, W., et al. (2020). Integrating Bimetallic AuPd Nanocatalysts with a 2D Aza-Fused π-conjugated Microporous Polymer for Light-Driven Benzyl Alcohol Oxidation. Chin. Chem. Lett. 31, 231–234. doi:10.1016/j.cclet.2019.04.022
Lin, P., Chai, F., Zhang, R., Xu, G., Fan, X., and Luo, X. (2016). Electrochemical Synthesis of Poly(3,4-Ethylenedioxythiophene) Doped with Gold Nanoparticles, and its Application to Nitrite Sensing. Microchim. Acta 183, 1235–1241. doi:10.1007/s00604-016-1751-5
Liu, B.-C., Chen, S.-L., Ling, X.-Y., Li, Q.-X., Xu, C.-W., and Liu, Z.-L. (2020). High Activity of NiCo2O4 Promoted Pt on Three-Dimensional Graphene-like Carbon for Glycerol Electrooxidation in an Alkaline Medium. RSC Adv. 10, 24705–24711. doi:10.1039/C9RA09896H
Liu, F., Yuan, Y., Li, L., Shang, S., Yu, X., Zhang, Q., et al. (2015). Synthesis of Polypyrrole Nanocomposites Decorated with Silver Nanoparticles with Electrocatalysis and Antibacterial Property. Composites B: Eng. 69, 232–236. doi:10.1016/j.compositesb.2014.09.030
Liu, P., and Nørskov, J. K. (2001). Ligand and Ensemble Effects in Adsorption on alloy Surfaces. Phys. Chem. Chem. Phys. 3, 3814–3818. doi:10.1039/B103525H
Liu, S., Dong, F., Tang, Z., and Wang, Q. (2021). The Formation of Wrapping Type Pt-Ni alloy on Three-Dimensional Carbon Nanosheet for Electrocatalytic Oxidation of Methanol. Int. J. Hydrogen Energ. 46, 15431–15441. doi:10.1016/j.ijhydene.2021.02.050
Liu, Y., Ren, G., Wang, M., Zhang, Z., Liang, Y., Wu, S., et al. (2019). Facile Synthesis of Trimetallic PtAuCu alloy Nanowires as High−Performance Electrocatalysts for Methanol Oxidation Reaction. J. Alloys Compd. 780, 504–511. doi:10.1016/j.jallcom.2018.12.016
Long, Z., Zhang, G., Wei, T., Niu, L., Zhu, J., and Li, J. (2020). Tuning of BixOyCl Formation with Sonication Time during Ultrasound-Hydrothermal Preparation. J. Ind. Eng. Chem. 84, 322–331. doi:10.1016/j.jiec.2020.01.014
M, P. R., and Berchmans, S. (2019). Poly (3, 4-ethylene Dioxythiophene) Supported Palladium Catalyst Prepared by Galvanic Replacement Reaction for Methanol Tolerant Oxygen Reduction. Sci. Rep. 9, 19184. doi:10.1038/s41598-019-55688-5
Mahmoudi-Badiki, T., Afghahi, S. S. S., Arsalani, N., Jafarian, M., and Stergiou, C. A. (2019). Effect of Synthesis Approaches and Morphological Properties on Dielectric Enhancement and Microwave Absorption of Fe3O4/PANI Nanocomposites. J. Supercond. Nov. Magn. 32, 1705–1714. doi:10.1007/s10948-018-4866-6
Mao, H., Cao, Z., Guo, X., Liu, M., Sun, D., Sun, Z., et al. (2019). Enhanced Electrocatalytic Performance for the Oxidation of Methanol by Hierarchical NiS/Ni(OH)2@polypyrrole/graphene Oxide Nanosheets. Appl. Surf. Sci. 471, 355–367. doi:10.1016/j.apsusc.2018.11.188
Marinho, B., Ghislandi, M., Tkalya, E., Koning, C. E., and de With, G. (2012). Electrical Conductivity of Compacts of Graphene, Multi-wall Carbon Nanotubes, Carbon Black, and Graphite Powder. Powder Technol. 221, 351–358. doi:10.1016/j.powtec.2012.01.024
Marinkovic, N. S., Li, M., and Adzic, R. R. (2019). Pt-Based Catalysts for Electrochemical Oxidation of Ethanol. Top. Curr. Chem. (Z) 377, 11. doi:10.1007/s41061-019-0236-5
Memioğlu, F., Bayrakçeken, A., Öznülüer, T., and Ak, M. (2014). Conducting Carbon/polymer Composites as a Catalyst Support for Proton Exchange Membrane Fuel Cells. Int. J. Energ. Res. 38, 1278–1287. doi:10.1002/er.3126
Meng, L.-Y., Wang, B., Ma, M.-G., and Lin, K.-L. (2016). The Progress of Microwave-Assisted Hydrothermal Method in the Synthesis of Functional Nanomaterials. Mater. Today Chem. 1-2, 63–83. doi:10.1016/j.mtchem.2016.11.003
Menshchikov, V., Alekseenko, A., Guterman, V., Nechitailov, A., Glebova, N., Tomasov, A., et al. (2020). Effective Platinum-Copper Catalysts for Methanol Oxidation and Oxygen Reduction in Proton-Exchange Membrane Fuel Cell. Nanomaterials 10, 742. doi:10.3390/nano10040742
Merati, Z., Parsa, J. B., and Babaei-Sati, R. (2018). Electrochemically Synthesized polypyrrole/MWCNTs-Al2O3 Ternary Nanocomposites Supported Pt Nanoparticles toward Methanol Oxidation. Int. J. Hydrogen Energ. 43, 20993–21005. doi:10.1016/j.ijhydene.2018.09.110
Milikić, J., Stamenović, U., Vodnik, V., Ahrenkiel, S. P., and Šljukić, B. (2019). Gold Nanorod-Polyaniline Composites: Synthesis and Evaluation as Anode Electrocatalysts for Direct Borohydride Fuel Cells. Electrochimica Acta 328, 135115. doi:10.1016/j.electacta.2019.135115
Mishra, P., Lakshmi, G. B. V. S., Mishra, S., Avasthi, D. K., Swart, H. C., Turner, A. P. F., et al. (2017). Electrocatalytic Biofuel Cell Based on Highly Efficient Metal-Polymer Nano-Architectured Bioelectrodes. Nano Energy 39, 601–607. doi:10.1016/j.nanoen.2017.06.023
Monyoncho, E. A., Steinmann, S. N., Michel, C., Baranova, E. A., Woo, T. K., and Sautet, P. (2016). Ethanol Electro-Oxidation on Palladium Revisited Using Polarization Modulation Infrared Reflection Absorption Spectroscopy (PM-IRRAS) and Density Functional Theory (DFT): Why Is it Difficult to Break the C-C Bond? ACS Catal. 6, 4894–4906. doi:10.1021/acscatal.6b00289
Mozafari, V., and Basiri Parsa, J. (2020). Electrochemical Synthesis of Pd Supported on PANI-MWCNTs-SnO2 Nanocomposite as a Novel Catalyst towards Ethanol Oxidation in Alkaline media. Synth. Met. 259, 116214. doi:10.1016/j.synthmet.2019.116214
Nakova, A., Ilieva, M., Boijadjieva-Scherzer, T., and Tsakova, V. (2019). Glycerol Oxidation on Pd Nanocatalysts Obtained on PEDOT-Coated Graphite Supports. Electrochimica Acta 306, 643–650. doi:10.1016/j.electacta.2019.03.151
Nasrollahzadeh, M., Sajjadi, M., Shokouhimehr, M., and Varma, R. S. (2019). Recent Developments in Palladium (Nano)catalysts Supported on Polymers for Selective and Sustainable Oxidation Processes. Coord. Chem. Rev. 397, 54–75. doi:10.1016/j.ccr.2019.06.010
Nath, D., Mandal, S. K., and Nath, A. (2019). Polymer Based LaFeO3-Poly(vinylidene Fluoride) Hybrid Nanocomposites: Enhanced Magneto-Electric Coupling, Magnetoimpedance and Dielectric Response. J. Alloys Compd. 806, 968–975. doi:10.1016/j.jallcom.2019.07.299
Nodehi, Z., Rafati, A. A., and Ghaffarinejad, A. (2018). Palladium-silver Polyaniline Composite as an Efficient Catalyst for Ethanol Oxidation. Appl. Catal. A: Gen. 554, 24–34. doi:10.1016/j.apcata.2018.01.018
Nosheen, F., Anwar, T., Siddique, A., and Hussain, N. (2019). Noble Metal Based Alloy Nanoframes: Syntheses and Applications in Fuel Cells. Front. Chem. 7. doi:10.3389/fchem.2019.00456
Ong, B. C., Kamarudin, S. K., and Basri, S. (2017). Direct Liquid Fuel Cells: A Review. Int. J. Hydrogen Energ. 42, 10142–10157. doi:10.1016/j.ijhydene.2017.01.117
Oularbi, L. (2018). Étude de nanocomposites polypyrrole/nanoparticule de carbone par impédance électrochimique et ac-électrogravimétrie: application aux capteurs électrochimiques. Casablanca, Morocco: Sorbonne and Hassan II Casablanca Universities. Ph.D. ThesisJune 29, 2018. ⟨NNT : 2018SORUS110⟩. ⟨tel-02922320⟩.
Oularbi, L., Turmine, M., and El Rhazi, M. (2017). Electrochemical Determination of Traces lead Ions Using a New Nanocomposite of Polypyrrole/carbon Nanofibers. J. Solid State. Electrochem. 21, 3289–3300. doi:10.1007/s10008-017-3676-2
Oularbi, L., Turmine, M., and El Rhazi, M. (2019). Preparation of Novel Nanocomposite Consisting of Bismuth Particles, Polypyrrole and Multi-Walled Carbon Nanotubes for Simultaneous Voltammetric Determination of Cadmium(II) and Lead(II). Synth. Met. 253, 1–8. doi:10.1016/j.synthmet.2019.04.011
Oularbi, L., Turmine, M., Salih, F. E., and El Rhazi, M. (2020). Ionic Liquid/carbon Nanofibers/bismuth Particles Novel Hybrid Nanocomposite for Voltammetric Sensing of Heavy Metals. J. Environ. Chem. Eng. 8, 103774. doi:10.1016/j.jece.2020.103774
Ozoemena, K. I. (2016). Nanostructured Platinum-free Electrocatalysts in Alkaline Direct Alcohol Fuel Cells: Catalyst Design, Principles and Applications. RSC Adv. 6, 89523–89550. doi:10.1039/C6RA15057H
Pandey, R. K., and Lakshminarayanan, V. (2012). Ethanol Electrocatalysis on Gold and Conducting Polymer Nanocomposites: A Study of the Kinetic Parameters. Appl. Catal. B: Environ. 125, 271–281. doi:10.1016/j.apcatb.2012.06.002
Park, K.-W., Choi, J.-H., Kwon, B.-K., Lee, S.-A., Sung, Y.-E., Ha, H.-Y., et al. (2002). Chemical and Electronic Effects of Ni in Pt/Ni and Pt/Ru/Ni Alloy Nanoparticles in Methanol Electrooxidation. J. Phys. Chem. B 106, 1869–1877. doi:10.1021/jp013168v
Park, K.-W., Choi, J.-H., and Sung, Y.-E. (2003). Structural, Chemical, and Electronic Properties of Pt/Ni Thin Film Electrodes for Methanol Electrooxidation. J. Phys. Chem. B 107, 5851–5856. doi:10.1021/jp0340966
Parsa, A., and Amanzadeh-Salout, S. (2016). Electrocatalytic Activity and Electrochemical Impedance Spectroscopy of Poly(Aniline-Co-Ortho-Phenylenediamine) Modified Electrode on Ascorbic Acid. Orient. J. Chem. 32, 2051–2058. doi:10.13005/ojc/320432
Pattanayak, P., Pramanik, N., Kumar, P., and Kundu, P. P. (2018). Fabrication of Cost-Effective Non-noble Metal Supported on Conducting Polymer Composite Such as Copper/polypyrrole Graphene Oxide (Cu 2 O/PPy-GO) as an Anode Catalyst for Methanol Oxidation in DMFC. Int. J. Hydrogen Energ. 43, 11505–11519. doi:10.1016/j.ijhydene.2017.04.300
Paulraj, P., Janaki, N., Sandhya, S., and Pandian, K. (2011). Single Pot Synthesis of Polyaniline Protected Silver Nanoparticles by Interfacial Polymerization and Study its Application on Electrochemical Oxidation of Hydrazine. Colloids Surf. A: Physicochemical Eng. Aspects 377, 28–34. doi:10.1016/j.colsurfa.2010.12.001
Pinithchaisakula, A., Ounnunkad, K., Themsirimongkon, S., Promsawan, N., Waenkaew, P., and Saipanya, S. (2017). Efficiency of Bimetallic PtPd on Polydopamine Modified on Various Carbon Supports for Alcohol Oxidations. Chem. Phys. 483-484, 56–67. doi:10.1016/j.chemphys.2016.11.010
Pinto, A. M. F. R., Oliveira, V. B., and Falcão, D. S. (2018). “Introduction to Direct Alcohol Fuel Cells,” in Direct Alcohol Fuel Cells for Portable Applications. Editors A. M. F. R. Pinto, V. B. Oliveira, and D. S. Falcão (Cambridge, Massachusetts, United States: Academic Press), 1–15. doi:10.1016/B978-0-12-811849-8.00001-2
Pushkarev, A., Pushkareva, I., Ivanova, N., du Preez, S., Bessarabov, D., Chumakov, R., et al. (2019). Pt/C and Pt/SnOx/C Catalysts for Ethanol Electrooxidation: Rotating Disk Electrode Study. Catalysts 9, 271. doi:10.3390/catal9030271
Qi, G., Huang, L., and Wang, H. (2012). Highly Conductive Free Standing Polypyrrole Films Prepared by Freezing Interfacial Polymerization. Chem. Commun. 48, 8246–8248. doi:10.1039/C2CC33889K
Qi, G., Wu, Z., and Wang, H. (2013). Highly Conductive and Semitransparent Free-Standing Polypyrrole Films Prepared by Chemical Interfacial Polymerization. J. Mater. Chem. C 1, 7102–7110. doi:10.1039/C3TC31340A
Qiu, J.-D., Wang, G.-C., Liang, R.-P., Xia, X.-H., and Yu, H.-W. (2011). Controllable Deposition of Platinum Nanoparticles on Graphene as an Electrocatalyst for Direct Methanol Fuel Cells. J. Phys. Chem. C 115, 15639–15645. doi:10.1021/jp200580u
Quintero-Ruiz, J., Ruiz-Rosas, R., Quílez-Bermejo, J., Salinas-Torres, D., Cazorla-Amorós, D., and Morallón, E. (2021). Preparation of Pt/CNT Thin-Film Electrodes by Electrochemical Potential Pulse Deposition for Methanol Oxidation. C 7, 32. doi:10.3390/c7020032
Ramli, Z. A. C., and Kamarudin, S. K. (2018). Platinum-Based Catalysts on Various Carbon Supports and Conducting Polymers for Direct Methanol Fuel Cell Applications: a Review. Nanoscale Res. Lett. 13, 410. doi:10.1186/s11671-018-2799-4
Ramli, Z. A. C., and Kamarudin, S. K. (2018). Platinum-Based Catalysts on Various Carbon Supports and Conducting Polymers for Direct Methanol Fuel Cell Applications: a Review. Nanoscale Res. Lett. 13, 410. doi:10.1186/s11671-018-2799-4
Raskó, J., Dömök, M., Baán, K., and Erdőhelyi, A. (2006). FTIR and Mass Spectrometric Study of the Interaction of Ethanol and Ethanol-Water with Oxide-Supported Platinum Catalysts. Appl. Catal. A: Gen. 299, 202–211. doi:10.1016/j.apcata.2005.10.027
Razzaghi, F., Debiemme-Chouvy, C., Pillier, F., Perrot, H., and Sel, O. (2015). Ion Intercalation Dynamics of Electrosynthesized Mesoporous WO3 Thin Films Studied by Multi-Scale Coupled Electrogravimetric Methods. Phys. Chem. Chem. Phys. 17, 14773–14787. doi:10.1039/c5cp00336a
Reddy, K. R., Karthik, K. V., Prasad, S. B. B., Soni, S. K., Jeong, H. M., and Raghu, A. V. (2016). Enhanced Photocatalytic Activity of Nanostructured Titanium Dioxide/polyaniline Hybrid Photocatalysts. Polyhedron 120, 169–174. doi:10.1016/j.poly.2016.08.029
Ren, X., Lv, Q., Liu, L., Liu, B., Wang, Y., Liu, A., et al. (2020). Current Progress of Pt and Pt-Based Electrocatalysts Used for Fuel Cells. Sustain. Energ. Fuels 4, 15–30. doi:10.1039/C9SE00460B
Ryu, K. S., Kim, K. M., Park, N.-G., Park, Y. J., and Chang, S. H. (2002). Symmetric Redox Supercapacitor with Conducting Polyaniline Electrodes. J. Power Sourc. 103, 305–309. doi:10.1016/S0378-7753(01)00862-X
Sahu, S. N., Sahoo, N. K., Naik, S. N., and Mahapatra, D. M. (2020). “Advancements in Hydrothermal Liquefaction Reactors: Overview and Prospects,” in Bioreactors. Editors L. Singh, A. Yousuf, and D. M. Mahapatra (Amsterdam, Netherlands: Elsevier), 195–213. doi:10.1016/B978-0-12-821264-6.00012-7
Salih, F. E., Ouarzane, A., and El Rhazi, M. (2017). Electrochemical Detection of lead (II) at bismuth/Poly(1,8-Diaminonaphthalene) Modified Carbon Paste Electrode. Arabian J. Chem. 10, 596–603. doi:10.1016/j.arabjc.2015.08.021
Salih, F. E., Oularbi, L., Halim, E., Elbasri, M., Ouarzane, A., and El Rhazi, M. (2018). Conducting Polymer/Ionic Liquid Composite Modified Carbon Paste Electrode for the Determination of Carbaryl in Real Samples. Electroanalysis 30, 1855–1864. doi:10.1002/elan.201800152
Salinas‐Quezada, M. P., Crespo‐Yapur, D. A., Cano‐Marquez, A., and Videa, M. (2019). Electrocatalytic Activity of Galvanostatically Deposited Ni Thin Films for Methanol Electrooxidation. Fuel Cells 19, 587–593. doi:10.1002/fuce.201900022
Sarkar, C., Nath, J., Bhuyan, S., and Dolui, S. K. (2019). Multifunctional Ternary Nanocomposites of Ni/Polypyrrole/Reduced Graphene Oxide as Supercapacitor and Electrocatalyst in Methanol Oxidation. ChemistrySelect 4, 2529–2537. doi:10.1002/slct.201803386
Sarma, S. C., Subbarao, U., Khulbe, Y., Jana, R., and Peter, S. C. (2017). Are We Underrating Rare Earths as an Electrocatalyst? the Effect of Their Substitution in Palladium Nanoparticles Enhances the Activity towards Ethanol Oxidation Reaction. J. Mater. Chem. A. 5, 23369–23381. doi:10.1039/C7TA07945A
Sarmoor, S. S., Hoseini, S. J., Hashemi Fath, R., Roushani, M., and Bahrami, M. (2018). Facile Synthesis of PtSnZn Nanosheet Thin Film at Oil-Water Interface by Use of Organometallic Complexes: An Efficient Catalyst for Methanol Oxidation and P -nitrophenol Reduction Reactions. Appl. Organometal Chem. 32, e3979. doi:10.1002/aoc.3979
Scibioh, M. A., Kim, S.-K., Cho, E. A., Lim, T.-H., Hong, S.-A., and Ha, H. Y. (2008). Pt-CeO2/C Anode Catalyst for Direct Methanol Fuel Cells. Appl. Catal. B: Environ. 84, 773–782. doi:10.1016/j.apcatb.2008.06.017
Selvaraj, V., Alagar, M., and Hamerton, I. (2006). Electrocatalytic Properties of Monometallic and Bimetallic Nanoparticles-Incorporated Polypyrrole Films for Electro-Oxidation of Methanol. J. Power Sourc. 160, 940–948. doi:10.1016/j.jpowsour.2006.02.055
Shah, A.-u. -H. A., Yasmeen, N., Rahman, G., Mehmood, M., and Bilal, S. (2016). Electrooxidation of Methanol at PANI/POAP Bilayered Structure Modified Platinum and Graphite Electrodes. Electrochimica Acta 188, 367–377. doi:10.1016/j.electacta.2015.11.140
Sharma, R. K., Rastogi, A. C., and Desu, S. B. (2008). Pulse Polymerized Polypyrrole Electrodes for High Energy Density Electrochemical Supercapacitor. Electrochemistry Commun. 10, 268–272. doi:10.1016/j.elecom.2007.12.004
Siwal, S., Matseke, S., Mpelane, S., Hooda, N., Nandi, D., and Mallick, K. (2017). Palladium-polymer Nanocomposite: An Anode Catalyst for the Electrochemical Oxidation of Methanol. Int. J. Hydrogen Energ. 42, 23599–23605. doi:10.1016/j.ijhydene.2017.03.033
Soleimani-Lashkenari, M., Rezaei, S., Fallah, J., and Rostami, H. (2018). Electrocatalytic Performance of Pd/PANI/TiO 2 Nanocomposites for Methanol Electrooxidation in Alkaline media. Synth. Met. 235, 71–79. doi:10.1016/j.synthmet.2017.12.001
Song, Y., Fan, J.-B., and Wang, S. (2017). Recent Progress in Interfacial Polymerization. Mater. Chem. Front. 1, 1028–1040. doi:10.1039/C6QM00325G
Surmenev, R. A., Orlova, T., Chernozem, R. V., Ivanova, A. A., Bartasyte, A., Mathur, S., et al. (2019). Hybrid lead-free Polymer-Based Nanocomposites with Improved Piezoelectric Response for Biomedical Energy-Harvesting Applications: A Review. Nano Energy 62, 475–506. doi:10.1016/j.nanoen.2019.04.090
Svietkina, O., Bas, K., Haddad, J., Ziborov, K., and Olishevska, V. (2020). Mechanochemical Activation of Polymetallic Ore and Further Selective Floatation. Kem 844, 65–76. doi:10.4028/www.scientific.net/KEM.844.65
Tajik, S., Beitollahi, H., Nejad, F. G., Shoaie, I. S., Khalilzadeh, M. A. M., Asl, M. S., et al. (2020). Recent Developments in Conducting Polymers: Applications for Electrochemistry. RSC Adv. 10, 37834–37856. doi:10.1039/D0RA06160C
Tao, Z., Chen, W., Yang, J., Wang, X., Tan, Z., Ye, J., et al. (2019). Ultrathin yet Transferrable Pt- or PtRu-Decorated Graphene Films as Efficient Electrocatalyst for Methanol Oxidation Reaction. Sci. China Mater. 62, 273–282. doi:10.1007/s40843-018-9366-x
Townley, B., Luca, R., López, F., Muñoz, M., and Castillo, P. (2019). “Multi-element Geochemistry for Bulk mineral Characterization of Hydrothermal Alteration Associations in Porphyry Copper Deposits and Applications to Predictive Geo-Metallurgical Modeling.”in Proceedings of the IMPC 2018 - 29th International Mineral Processing Congress, Moscow, Russia, 17-21 September 2018 (Montreal, Canada: Canadian Institute of Mining, Metallurgy and Petroleum). 2887–2894. Available at: https://researchers.unab.cl/es/publications/multi-element-geochemistry-for-bulk-mineral-characterization-of-h (Accessed June 21, 2020).
Ulas, B., Caglar, A., Kivrak, A., and Kivrak, H. (2019). Atomic Molar Ratio Optimization of Carbon Nanotube Supported PdAuCo Catalysts for Ethylene Glycol and Methanol Electrooxidation in Alkaline media. Chem. Pap. 73, 425–434. doi:10.1007/s11696-018-0601-9
Venkata Jagadeesh, R., and Lakshminarayanan, V. (2019). Enhanced Electrocatalytic Activity of Pd and Pd-Polyaniline Nanoparticles on Electrochemically Exfoliated Graphite Sheets. Appl. Catal. B: Environ. 251, 25–36. doi:10.1016/j.apcatb.2019.03.030
Vigier, F., Coutanceau, C., Hahn, F., Belgsir, E. M., and Lamy, C. (2004). On the Mechanism of Ethanol Electro-Oxidation on Pt and PtSn Catalysts: Electrochemical and In Situ IR Reflectance Spectroscopy Studies. J. Electroanalytical Chem. 563, 81–89. doi:10.1016/j.jelechem.2003.08.019
Vyas, A. N., Saratale, G. D., and Sartale, S. D. (2020). Recent Developments in Nickel Based Electrocatalysts for Ethanol Electrooxidation. Int. J. Hydrogen Energ. 45, 5928–5947. doi:10.1016/j.ijhydene.2019.08.218
Wang, C., Zhang, K., Xu, H., Du, Y., and Goh, M. C. (2019). Anchoring Gold Nanoparticles on Poly(3,4-Ethylenedioxythiophene) (PEDOT) Nanonet as Three-Dimensional Electrocatalysts toward Ethanol and 2-propanol Oxidation. J. Colloid Interf. Sci. 541, 258–268. doi:10.1016/j.jcis.2019.01.055
Wang, J.-G., Yang, Y., Huang, Z.-H., and Kang, F. (2012). Interfacial Synthesis of Mesoporous MnO2/polyaniline Hollow Spheres and Their Application in Electrochemical Capacitors. J. Power Sourc. 204, 236–243. doi:10.1016/j.jpowsour.2011.12.057
Wang, J., Neoh, K. G., and Kang, E. T. (2001). Preparation of Nanosized Metallic Particles in Polyaniline. J. Colloid Interf. Sci. 239, 78–86. doi:10.1006/jcis.2001.7576
Wang, Y., Zou, S., and Cai, W.-B. (2015). Recent Advances on Electro-Oxidation of Ethanol on Pt- and Pd-Based Catalysts: From Reaction Mechanisms to Catalytic Materials. Catalysts 5, 1507–1534. doi:10.3390/catal5031507
Wassel, A. R., El-Naggar, M. E., and Shoueir, K. (2020). Recent Advances in Polymer/metal/metal Oxide Hybrid Nanostructures for Catalytic Applications: a Review. J. Environ. Chem. Eng. 8, 104175. doi:10.1016/j.jece.2020.104175
White, A. M., and Slade, R. C. T. (2003). Vapour Transport Growth of Thin Films of Polypyrrole and poly(N-Methylpyrrole) Oxidatively Polymerised with Heteropolyacids. J. Mater. Chem. 13, 1345–1350. doi:10.1039/B300445G
Wu, B., Zhu, J., Li, X., Wang, X., Chu, J., and Xiong, S. (2019). PtRu Nanoparticles Supported on P-Phenylenediamine-Functionalized Multiwalled Carbon Nanotubes: Enhanced Activity and Stability for Methanol Oxidation. Ionics 25, 181–189. doi:10.1007/s11581-018-2590-7
Xie, F., Ma, L., Gan, M., He, H., Hu, L., Jiang, M., et al. (2019). One-pot Construction of the Carbon Spheres Embellished by Layered Double Hydroxide with Abundant Hydroxyl Groups for Pt-Based Catalyst Support in Methanol Electrooxidation. J. Power Sourc. 420, 73–81. doi:10.1016/j.jpowsour.2019.02.088
Xu, H., Miao, B., Zhang, M., Chen, Y., and Wang, L. (2017). Mechanism of C-C and C-H Bond Cleavage in Ethanol Oxidation Reaction on Cu2O(111): a DFT-D and DFT+U Study. Phys. Chem. Chem. Phys. 19, 26210–26220. doi:10.1039/C7CP04630H
Xu, H., Zhang, J., Chen, Y., Lu, H., Zhuang, J., and Li, J. (2014). Synthesis of Polyaniline-Modified MnO2 Composite Nanorods and Their Photocatalytic Application. Mater. Lett. 117, 21–23. doi:10.1016/j.matlet.2013.11.089
Xu, Y., Ruban, A. V., and Mavrikakis, M. (2004). Adsorption and Dissociation of O2 on Pt−Co and Pt−Fe Alloys. J. Am. Chem. Soc. 126, 4717–4725. doi:10.1021/ja031701+
Yan, R., Jin, B., Li, D., Zheng, J., Li, Y., and Qian, C. (2018). One-step Electrochemically Co-deposited Pt Nanoparticles/polyaniline Composites with Raspberry Structures for Methanol Electro-Oxidation. Synth. Met. 235, 110–114. doi:10.1016/j.synthmet.2017.11.014
Yang, H., Li, S., Shen, S., Jin, Z., Jin, J., and Ma, J. (2019). Unraveling the Cooperative Synergy of Palladium/Tin Oxide/Aniline-Functionalized Carbon Nanotubes Enabled by Layer-By-Layer Synthetic Strategy for Ethanol Electrooxidation. ACS Sustain. Chem. Eng. 7, 10008–10015. doi:10.1021/acssuschemeng.9b01197
Yang, L., Li, G., Ma, R., Hou, S., Chang, J., Ruan, M., et al. (2021). Nanocluster PtNiP Supported on Graphene as an Efficient Electrocatalyst for Methanol Oxidation Reaction. Nano Res. 14, 2853–2860. doi:10.1007/s12274-021-3300-8
Yang, S., Zhen, H., and Su, B. (2017). Polyimide Thin Film Composite (TFC) Membranes via Interfacial Polymerization on Hydrolyzed Polyacrylonitrile Support for Solvent Resistant Nanofiltration. RSC Adv. 7, 42800–42810. doi:10.1039/C7RA08133B
Yang, Y.-Y., Ren, J., Li, Q.-X., Zhou, Z.-Y., Sun, S.-G., and Cai, W.-B. (2014). Electrocatalysis of Ethanol on a Pd Electrode in Alkaline Media: An In Situ Attenuated Total Reflection Surface-Enhanced Infrared Absorption Spectroscopy Study. ACS Catal. 4, 798–803. doi:10.1021/cs401198t
Yang, Z., Nagashima, A., Fujigaya, T., and Nakashima, N. (2016). Electrocatalyst Composed of Platinum Nanoparticles Deposited on Doubly Polymer-Coated Carbon Nanotubes Shows a High CO-tolerance in Methanol Oxidation Reaction. Int. J. Hydrogen Energ. 41, 19182–19190. doi:10.1016/j.ijhydene.2016.08.198
Yang, Z., and Nakashima, N. (2015). A Simple Preparation of Very High Methanol Tolerant Cathode Electrocatalyst for Direct Methanol Fuel Cell Based on Polymer-Coated Carbon Nanotube/platinum. Sci. Rep. 5, 12236. doi:10.1038/srep12236
Yin, C., Zhou, H., and Li, J. (2019). Facile One-step Hydrothermal Synthesis of PEDOT:PSS/MnO2 Nanorod Hybrids for High-Rate Supercapacitor Electrode Materials. Ionics 25, 685–695. doi:10.1007/s11581-018-2680-6
Yoshimura, H. N., de Lima, M. B., Santos, S. F., and Ortega, F. d. S. (2017). Evaluation of Mechanochemical and Hydrothermal Transformations in a Wet-Milled Alumina by Transmission Electron Microscopy and Thermal Analysis. Msf 881, 46–51. doi:10.4028/www.scientific.net/MSF.881.46
Yuan, L.-S., Zheng, Y.-X., Jia, M.-L., Zhang, S.-J., Wang, X.-L., and Peng, C. (2015). Nanoporous Nickel-Copper-Phosphorus Amorphous alloy Film for Methanol Electro-Oxidation in Alkaline Medium. Electrochimica Acta 154, 54–62. doi:10.1016/j.electacta.2014.12.055
Zakaria, Z., Kamarudin, S. K., and Timmiati, S. N. (2016). Membranes for Direct Ethanol Fuel Cells: An Overview. Appl. Energ. 163, 334–342. doi:10.1016/j.apenergy.2015.10.124
Zhang, B., Zhao, B., Huang, S., Zhang, R., Xu, P., and Wang, H.-L. (2012). One-pot Interfacial Synthesis of Au Nanoparticles and Au-Polyaniline Nanocomposites for Catalytic Applications. CrystEngComm 14, 1542–1544. doi:10.1039/C2CE06396D
Zhang, J., Lu, S., Xiang, Y., and Jiang, S. P. (2020a). Intrinsic Effect of Carbon Supports on the Activity and Stability of Precious Metal Based Catalysts for Electrocatalytic Alcohol Oxidation in Fuel Cells: A Review. ChemSusChem 13, 2484–2502. doi:10.1002/cssc.202000048
Zhang, J., Nan, L., Yue, W., and Chen, X. (2020b). Enhanced Methanol Electro-Oxidation Activity of Electrochemically Exfoliated Graphene-Pt through Polyaniline Modification. J. Electroanalytical Chem. 858, 113821. doi:10.1016/j.jelechem.2020.113821
Zhang, K., Wang, H., Qiu, J., Wu, J., Wang, H., Shao, J., et al. (2021). Multi-dimensional Pt/Ni(OH)2/nitrogen-doped Graphene Nanocomposites with Low Platinum Content for Methanol Oxidation Reaction with Highly Catalytic Performance. Chem. Eng. J. 421, 127786. doi:10.1016/j.cej.2020.127786
Zhang, L., Du, W., Nautiyal, A., Liu, Z., and Zhang, X. (2018). Recent Progress on Nanostructured Conducting Polymers and Composites: Synthesis, Application and Future Aspects. Sci. China Mater. 61, 303–352. doi:10.1007/s40843-017-9206-4
Zhang, L., Liu, P., Ju, L., Wang, L., and Zhao, S. (2010). Polypyrrole Nanocapsules via Interfacial Polymerization. Macromol. Res. 18, 648–652. doi:10.1007/s13233-010-0713-8
Zhang, M., Li, Y., Pan, D., Yan, Z., Meng, S., and Xie, J. (2016a). Nickel Core-Palladium Shell Nanoparticles Grown on Nitrogen-Doped Graphene with Enhanced Electrocatalytic Performance for Ethanol Oxidation. RSC Adv. 6, 33231–33239. doi:10.1039/C6RA06416G
Zhang, M., Xie, J., Sun, Q., Yan, Z., Chen, M., and Jing, J. (2013). Enhanced Electrocatalytic Activity of High Pt-Loadings on Surface Functionalized Graphene Nanosheets for Methanol Oxidation. Int. J. Hydrogen Energ. 38, 16402–16409. doi:10.1016/j.ijhydene.2013.09.108
Zhang, Q., Chen, S., Tan, Z., Zhang, T., and Mclellan, B. (2019a). Investment Strategy of Hydrothermal Geothermal Heating in China under Policy, Technology and Geology Uncertainties. J. Clean. Prod. 207, 17–29. doi:10.1016/j.jclepro.2018.09.240
Zhang, R.-C., Sun, D., Zhang, R., Lin, W.-F., Macias-Montero, M., Patel, J., et al. (2017). Gold Nanoparticle-Polymer Nanocomposites Synthesized by Room Temperature Atmospheric Pressure Plasma and Their Potential for Fuel Cell Electrocatalytic Application. Sci. Rep. 7, 46682. doi:10.1038/srep46682
Zhang, S., Liu, L., Yang, J., Zhang, Y., Wan, Z., and Zhou, L. (2019b). Pd-Ru-Bi Nanoalloys Modified Three-Dimensional Reduced Graphene oxide/MOF-199 Composites as a Highly Efficient Electrocatalyst for Ethylene Glycol Electrooxidation. Appl. Surf. Sci. 492, 617–625. doi:10.1016/j.apsusc.2019.06.228
Zhang, Y., Chen, P., Wen, F., Huang, C., and Wang, H. (2016b). Construction of Polyaniline/molybdenum Sulfide Nanocomposite: Characterization and its Electrocatalytic Performance on Nitrite. Ionics 22, 1095–1102. doi:10.1007/s11581-015-1634-5
Zhang, Z., Xin, L., Sun, K., and Li, W. (2011). Pd-Ni Electrocatalysts for Efficient Ethanol Oxidation Reaction in Alkaline Electrolyte. Int. J. Hydrogen Energ. 36, 12686–12697. doi:10.1016/j.ijhydene.2011.06.141
Zhao, Y., Li, Y., Pang, H., Yang, C., and Ngai, T. (2019). Controlled Synthesis of Metal-Organic Frameworks Coated with noble Metal Nanoparticles and Conducting Polymer for Enhanced Catalysis. J. Colloid Interf. Sci. 537, 262–268. doi:10.1016/j.jcis.2018.11.031
Zhong, M., Chi, M., Zhu, Y., Wang, C., and Lu, X. (2019). An Efficient Thin-Walled Pd/polypyrrole Hybrid Nanotube Biocatalyst for Sensitive Detection of Ascorbic Acid. Analytica Chim. Acta 1056, 125–134. doi:10.1016/j.aca.2018.12.052
Zhou, Q., and Shi, G. (2016). Conducting Polymer-Based Catalysts. J. Am. Chem. Soc. 138, 2868–2876. doi:10.1021/jacs.5b12474
Keywords: metal catalyst, fuel cell, methanol oxidation, ethanol oxidation, conducting polymers, carbon nanostructures
Citation: Halim EM, Chemchoub S, El Attar A, Salih FE, Oularbi L and EL RHAZI M (2022) Recent Advances in Anode Metallic Catalysts Supported on Conducting Polymer-Based Materials for Direct Alcohol Fuel Cells. Front. Energy Res. 10:843736. doi: 10.3389/fenrg.2022.843736
Received: 26 December 2021; Accepted: 28 February 2022;
Published: 23 March 2022.
Edited by:
José Joaquín Linares León, University of Brasilia, BrazilReviewed by:
Anchu Ashok, Texas A&M University at Qatar, QatarLu Wei, Jiangsu Normal University, China
Copyright © 2022 Halim, Chemchoub, El Attar, Salih, Oularbi and EL RHAZI. This is an open-access article distributed under the terms of the Creative Commons Attribution License (CC BY). The use, distribution or reproduction in other forums is permitted, provided the original author(s) and the copyright owner(s) are credited and that the original publication in this journal is cited, in accordance with accepted academic practice. No use, distribution or reproduction is permitted which does not comply with these terms.
*Correspondence: Mama EL RHAZI, ZWxyaGF6aW1AaG90bWFpbC5jb20=