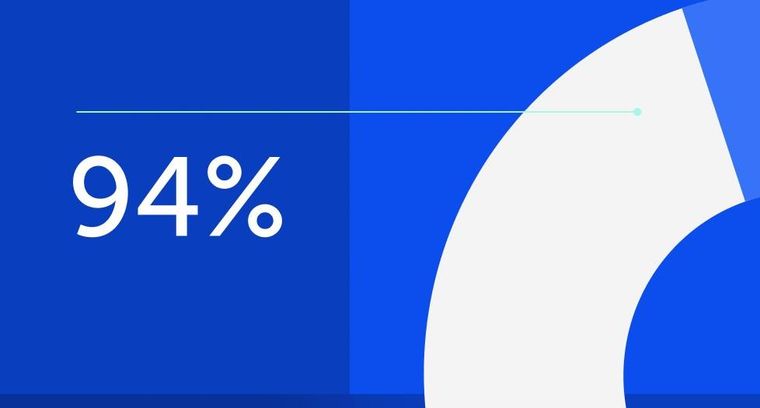
94% of researchers rate our articles as excellent or good
Learn more about the work of our research integrity team to safeguard the quality of each article we publish.
Find out more
BRIEF RESEARCH REPORT article
Front. Energy Res., 15 February 2022
Sec. Smart Grids
Volume 10 - 2022 | https://doi.org/10.3389/fenrg.2022.841415
This article is part of the Research TopicAdvances in Flexible Resource Control and Optimization for High Renewable Penetrated Power SystemView all 9 articles
The impact of the large-scale disconnection of photovoltaic generators on the grid cannot be ignored, so the low-voltage ride-through problem of photovoltaic generators needs to be solved urgently, especially in the stage after fault recovery, there is a problem that the converter is difficult to switch back to the original control strategy. Therefore, a new control strategy of the whole-process low-voltage ride-through control strategy for photovoltaic power generation based on adaptive maximum power tracking is proposed. In the initial stage of failure, an adaptive maximum power control strategy is proposed. This control strategy transforms maximum power point tracking control into adaptive maximum power control by feedback of voltage drop amplitude. It can control the output of photovoltaic power quickly and effectively and realize the zero-voltage crossing of the photovoltaic grid-connected system. In the stage after fault recovery, the switching function is established by comparing the network voltage with the rated voltage. The operating state of the photovoltaic system can be judged by different output of the switching function. It realizes the fast switching of the photovoltaic side control strategy during the fault recovery stage. Simulation results show that the proposed method can switch the control strategy reliably and realize the whole-process low-voltage crossing of photovoltaic power generation quickly.
With the increasing proportion of photovoltaic access capacity in the power system, the safe and reliable operation of the photovoltaic grid-connected system becomes particularly important. When the power grid fails, the power grid voltage will drop temporarily, and the grid-connected current will increase suddenly. At this time, the photovoltaic output current will be limited below the maximum current limit value, and the active power of the photovoltaic output will be limited.(Bighash E et al., 2018; Ding et al., 2015; Li et al., 2021). However, the energy emitted by photovoltaic cells still remains unchanged at the maximum power point, so the excess energy will accumulate on the DC bus capacitor, resulting in the DC bus voltage surge. How to deal with this part of the excess energy becomes the key to achieve low-voltage crossing.
At present, there are literatures on the low-voltage crossing strategy. In reference (Li et al., 2019; Al-Shetwi and Sujod., 2019; Zhang et al., 2020), during a failure, the unloading resistor consumes the excess energy emitted by the photovoltaic cell, to maintain the balance of power on both sides of the inverter. The parallel unloading resistance method at the DC bus proposed in these literatures limits the rise of the DC side voltage and the overcurrent of the AC side grid-connected current, thus meeting the requirements of low-voltage crossing. There are also literatures (Liu et al., 2019; Yin., 2021) that put forward the low-voltage traversal strategy based on supercapacitor energy storage. The control of active power in the low-voltage fault process is realized by using the inherent fast charging and discharging characteristics of the supercapacitor. When the DC bus voltage exceeds the specified value, the supercapacitor absorbs excess energy to balance the power on both sides of the inverter and suppress the dc voltage rise. However, adding ultracapacitors will greatly increase the hardware cost of the device, complicate the control of the system, and increase the uncertainty. In addition, the huge volume of ultracapacitor equipment brings a lot of inconvenience to its installation and maintenance. However, the aforementioned study only considered changing the topology of the system to achieve a good low-voltage crossing. The addition of shunt unloading resistance not only increases the cost, but also generates a large amount of heat, which will affect the safety and stability of the grid-connected system. Therefore, only changing the unloading resistor or adding additional unloading resistance to achieve low-voltage crossing is defective.0.
Some literatures consider to realize low-voltage crossing by changing the control strategy without changing the topological structure of the system. In the literature (Li F. et al., 2021; Jia et al., 2020; Yan et al., 2021), the photovoltaic side detects the feedback of power grid voltage drop and quickly switches the constant power double closed-loop control of the inverter in the normal state, to the constant single closed-loop control of the inverter in the fault state. The aforementioned control strategy can ensure that the grid-connected current does not flow, but the DC bus voltage is not considered in the single loop control, which cannot ensure the stability of DC bus voltage. There are also literatures (Benali et al., 2018; Huka et al., 2018) that put forward the low-voltage crossing strategy based on the PQ theory and PR control method for the single-stage photovoltaic system. Although the effectiveness of the method is verified by experiments, only the slight voltage sag is considered, and the more serious fault is not considered. Also, this method is only applicable to the single-stage photovoltaic system and cannot be applied to the current use of more common two-stage three-phase photovoltaic grid-connected system. Therefore, only changing the control strategy also cannot achieve a good low-voltage crossing of the photovoltaic system too.
In addition, after failure recovery, the photovoltaic system should be restored to the maximum power output mode. However, the traditional control method cannot accurately detect the changes of system operating conditions, and the photovoltaic cell may not be able to recover the MPPT control. This will affect the operating efficiency of the photovoltaic DC grid-connected system, so the control method in the fault recovery stage also needs to be further studied.
Aiming at the aforementioned problems, this article proposes the whole-process low-voltage crossing control strategy for photovoltaic power generation based on adaptive maximum power tracking. In the initial stage of power grid failure, the photovoltaic side adjusts the power tracking method from MPPT to adaptive control strategy, through the feedforward of voltage drop amplitude. The proposed adaptive control strategy controls the terminal voltage of photovoltaic cells, reduces the energy emission, balances the power on both sides of the converter quickly, and keeps the dc bus voltage stable. In addition, combined with the direct current control of the inverter, the power on both sides of the inverter is quickly balanced, so that the DC bus voltage is stable, and the grid-connected output current does not flow. By improving the control strategy of the inverter, reactive power is generated according to the different amplitude of voltage sag to support the voltage recovery of the grid.
In the stage of power grid fault recovery, a switching function control strategy is defined in this article. The power grid voltage is compared with the rated voltage to measure the power grid voltage feedforward amount. Then, the operating state of the photovoltaic system is judged by the positive and negative voltage feedforward quantity, so that the switching function outputs different quantities. It can switch back to MPPT control quickly and reliably without communication. Thus, the whole-process low-voltage crossing of photovoltaic power generation is controlled from fault initial to fault recovery by the proposed strategy in this article. PSCAD simulation results show that the proposed control strategy can ensure the system stability and improve the photovoltaic utilization rate under various voltage drops of the power grid. It realizes the whole process of photovoltaic low-voltage crossing from the initial stage of failure to the period of failure recovery.
As shown in Figure 1, a complete three-phase photovoltaic grid-connected system includes photovoltaic cells, inverters, and control systems. The control system consists of two parts: photovoltaic cells and inverters. The photovoltaic cell side can control the output power and raise the voltage to the appropriate value so that the photovoltaic side can realize the adaptive maximum power control strategy, which can make the inverter work normally. The inverter partially controls the DC bus voltage through the double closed-loop of voltage and current and transmits the required electricity to the power grid side.
Photovoltaic cells convert light energy into electricity using the photoelectric volt effect of the P-N junction. When the light is illuminated, the I-V characteristics of the photovoltaic cell are diode characteristics, and the current changes exponentially with the voltage. If the photogenic current is regarded as a constant current source, the effect of series equivalent resistance
The I-V characteristic equation of photovoltaic cells can be expressed as (Yang et al., 2017; Li Y. et al., 2021):
where A and B are parameters related to P-N junction material properties, q is the charge of the electron K is the Boltzmann constant, and T is the absolute temperature.
On the premise of ensuring certain accuracy, the output current of the simplified photovoltaic cell is shown in Eq. 2 (Mei et al., 2021).
where
According to the aforementioned mathematical model of photovoltaic cells, the changes of photovoltaic characteristics under different environmental influences can be obtained. Under normal circumstances, the output power curve of photovoltaic cells is a single-peak curve, which with maximum power point calculated as the extreme value. Under different light intensity and temperature conditions, the voltage of the photovoltaic cell is controlled so that it operates at its maximum power point at different light levels and temperatures (Sabir and Ibrir., 2020; He et al., 2019; Li et al., 2020).
In the dq-rotating coordinate system, the mathematical model of the grid-connected inverter can be expressed as (Abidi et al., 2020):
where “
The low-voltage crossing strategy of proposed adaptive maximum power changes the terminal voltage of photovoltaic cells by adjusting photovoltaic cells during failures. It reduces the generation of excess energy from the source, quickly and effectively realizes the power balance on both sides of the inverter, and avoids DC bus overvoltage and grid-connected current overcurrent. Under the premise of not changing the topological structure and not adding the unloading resistor, it can realize low-voltage crossing well and greatly reduce the cost of the system.
Under normal circumstances, the photovoltaic system finds the terminal voltage corresponding to the MPPT. It keeps the photovoltaic cell terminal voltage at this value and makes it emit as much energy as possible. When low-voltage faults occur on the grid side, in order to achieve energy balance, the terminal voltage of photovoltaic cells cannot remain unchanged at the original value. The terminal voltage should be changed by adding an additional value which is related to the depth of voltage drop. The additional value here is adjusted by the PI_LVRT regulator, whose input value is the difference voltage U between the actual grid voltage and 90% of the rated voltage. The transfer function of PI_LVRT is
The adaptive MPPT control structure diagram of low-voltage crossing in photovoltaic power generation is shown in Figure 3. Define the given value of the photovoltaic cell terminal voltage as
When the power grid is in normal operation or the power grid voltage fluctuation is less than 10% of the rated voltage, the system is in normal operation, and the influence of the power grid voltage is not considered. Photovoltaic cells operate at the maximum power point. In this situation,
The Chinese standard (GB/T 19964-2012) “Technical Provisions for Photovoltaic Power Station Access to Power System” points out the requirements for low-voltage crossing that photovoltaic power stations should meet: when the voltage of the connection point drops to 0, the photovoltaic power station should be able to operate continuously for 0.15s without off-grid. In addition, in the failure stage, the photovoltaic power station needs to support the voltage in the grid. Therefore, the adaptive variable power controller needs to provide dynamic reactive power support to the receiving network in case of failure. The reactive power value injected by the adaptive variable power controller and the voltage at the connection point should meet the following requirements:
where
In normal operation, the constant DC voltage control of the adaptive variable power controller makes the DC bus voltage run stably at the rated value and keeps the balance of the power transmission of the system. When the power grid fails, the proposed adaptive variable power control will provide reactive power support for the power grid and switch to constant power control. In order to prevent overcurrent of the adaptive variable power controller in the process of system failure, the reference value of active power of the adaptive variable power controller is set at
In order to transmit as much active power as possible in case of failure, Eq. 5 is substituted into the aforementioned equation, and the relationship between the maximum active power output of the adaptive variable power controller and the voltage of the connection point is:
In the stage of power grid fault recovery, the photovoltaic needs to switch back to MPPT control to improve the efficiency of photovoltaic power generation. However, the traditional control method may have the situation that the DC voltage fluctuation is not obvious and the adaptive variable power control is difficult to switch back to MPPT control. Therefore, the control strategy of the post fault recovery stage needs to be studied.
In general, when the low-voltage crossing process is over, the photovoltaic cell terminal voltage is at a low value. The photovoltaic system needs to spend some time to re-track the maximum power point to make the battery run at the maximum power, even though the voltage dip only lasts for a very small period of time. In order to solve these problems, a switching function module is added to the adaptive variable power control system in the stage of fault recovery.
The working principle of the switching function is as follows in Figure 4: compare the network voltage with 90% of the rated voltage, and get the positive and negative of the network voltage feedforward regulation. Then, the fault state of the power grid is judged, and different switching function values are obtained to realize the switching of the control strategy. When the power grid voltage is lower than 90% of the rated voltage, the power grid fails, the power grid voltage feedforward regulation
Based on PSCAD simulation software, a simulation model of 9-kw three-phase photovoltaic grid-connected system was established. A total of five key observations from the whole process, grid-connected voltage U, grid-connected current I, active power output P, reactive power output Q, and DC bus voltage Udc, are shown in Figures 5, 6. By observing these important parameters, the effectiveness of the whole process control strategy in the initial stage of fault occurrence and the stage after fault recovery is verified. The main parameters are as follows: maximum power
1) Simulation of low-voltage crossing when the voltage drops to 70% of the rated voltage.
It can be seen from Figure 5 that the voltage drop reaches 70% of the rated voltage within 0.2∼0.7 s, and the voltage drop lasts for 0.5 s.
First, the change of the grid-connected current is observed, and it can be seen that the grid-connected current only fluctuates at the beginning and end of the fall. In the initial stage of the fault, its amplitude does not exceed 1.2 times of the limited current; in the fault recovery stage, its amplitude does not exceed 1.0-1.2 times of the limited current. The grid-connected current in the whole process of failure is within the standard range. It will not cause the grid current overcurrent protection to start and damage the device or trigger protection to shut off the circuit breaker.
Second, the changes of DC bus voltage were observed. It was found that the DC bus voltage basically remained near the reference voltage of 800 V during the whole fault process. Finally, the changes of active power and reactive power during the period of fault voltage drop are observed. It can be seen that the active power emitted decreases from the rated power of 9000 W to about 6800 W, and the reactive power increases from 0 Var to 2,500 Var. It is basically the same as the power calculated by using the reactive current value required in the Chinese standard.
Under the simulation condition of the grid voltage dropping to 70% of the rated voltage, it is found that in the initial stage of the fault and the recovery stage, the grid current is within the standard range of 1.0–1.2 times, which well verifies the effectiveness of the whole-process low-voltage crossing control strategy of the adaptive variable power tracking trajectory.
2) Simulation of low-voltage crossing when the voltage drops to zero.
It can be seen from Figure 6 that within 0.2∼0.35, power grid voltage drops to zero and lasts for 150 ms, which is the most serious case of power grid failure. The photovoltaic system can keep up on the grid for 150 ms when the adaptive variable power tracking strategy is adopted in this article.
First, the change of the grid-connected current is observed, and it can be found that the grid-connected current basically remains at the rated value except that it fluctuates during the initial stage of failure.
Second, the changes of DC bus voltage were observed. It can be seen that during the failure period, the DC bus voltage did not exceed the limit, and after the fault recovery, the adaptive variable power tracking also quickly switched to the maximum power output, and the DC bus voltage also returned to normal.
Finally, the changes of active power and reactive power during the fault voltage drop are observed. According to the requirements for reactive current stipulated in China standard low-voltage crossing requirements, when the grid voltage is less than 40% of the rated value, the grid-connected current should be all reactive current, and the active current is 0. Therefore, under the simulation condition that the voltage drops to 0, the grid-connected current is 100% reactive current, and the active current drops to 0. As shown in Figure 6, both active power and reactive power drop to 0.
To sum up, when the grid voltage drops to zero, it is found that the DC bus voltage does not exceed the limit in the whole process of the fault, and zero-voltage crossing is realized. It is further demonstrated that the whole-process low-voltage through control strategy for photovoltaic power generation based on adaptive maximum power tracking can be well applied to low-voltage crossing of photovoltaic power generation.
The simulation results verify the feasibility of the adaptive variable power strategy under different voltage drops. The low-voltage strategy proposed in this article can realize the whole process of low-voltage traversal of the photovoltaic system, even the whole process of zero-voltage traversal without additional equipment.
In this article, the whole-process low-voltage through control strategy for photovoltaic power generation based on adaptive maximum power tracking is proposed, and the following conclusions are drawn:
1) In the initial stage of failure, an adaptive variable power control strategy is proposed. This control strategy transforms MPPT control into adaptive maximum power control by feedback of voltage drop amplitude. It changes the photovoltaic cell terminal voltage, and then quickly and effectively controls the photovoltaic cell power output, to ensure the balance of power on both sides of the inverter. Finally, the zero-voltage crossing of the photovoltaic grid-connected system is realized.
2) In the stage after fault recovery, a control method of the switching function is proposed. By comparing the grid voltage with the rated voltage, the positive and negative UPV_L of the grid voltage feedforward regulation is judged. Then, different values are output according to the switching function, so that the photovoltaic side can be switched to MPPT control in time. It reliably improves the efficiency of photovoltaic power generation.
3) In this article, the whole-process low-voltage ride-through control strategy for photovoltaic power generation based on adaptive maximum power tracking is verified by simulation under different voltage drops. It can realize the whole process of low-voltage traversal, even the whole process of zero-voltage traversal of the photovoltaic system, and effectively improve the economy of photovoltaic power generation.
The original contributions presented in the study are included in the article/supplementary material; further inquiries can be directed to the corresponding author.
JY: data curation, writing, and software.
This work is supported by the Key Scientific Research Projects of Colleges and Universities in Henan Province (19A470003).
The authors declare that the research was conducted in the absence of any commercial or financial relationships that could be construed as a potential conflict of interest.
All claims expressed in this article are solely those of the authors and do not necessarily represent those of their affiliated organizations, or those of the publisher, the editors, and the reviewers. Any product that may be evaluated in this article, or claim that may be made by its manufacturer, is not guaranteed or endorsed by the publisher.
Abidi, M., Ben Rhouma, A., and Belhadj, J. (2020). Water-Energy System toward the Meeting of an Improved Low Voltage Ride through Capability of Grid-Connected Photovoltaic Generator: Power-Sharing and Control Issues. Energy Sourc. A: Recovery, Utilization, Environ. Effects 42, 1–28. doi:10.1080/15567036.2020.1841857
Al-Shetwi, A. Q., and Sujod, M. Z. (2019). Voltage Sag Detection in Grid-Connected Photovoltaic Power Plant for Low Voltage Ride-Through Control. Eeeng 12 (4), 384–392. doi:10.2174/2352096511666180626151223
Benali, A., Khiat, M., Allaoui, T., and Denai, M. (2018). Power Quality Improvement and Low Voltage Ride through Capability in Hybrid Wind-PV Farms Grid-Connected Using Dynamic Voltage Restorer. IEEE Access 6, 68634–68648. doi:10.1109/access.2018.2878493
Ding, G., Gao, F., and Tian, H., (2015). Adaptive DC-link Voltage Control of Two-Stage Photovoltaic Inverter during Low Voltage Ride-Through Operation. IEEE Trans. Power Electro. 31 (6), 4182–4194.
He, Y., Wang, M., and Xu, Z., (2019). Coordinative Low-Voltage-Ride-Through Control for the Wind-Photovoltaic Hybrid Generation System. IEEE J. Emerging Selected Top. Power Electro. 8 (2), 1503–1514.
Huka, G. B., Li, W., Chao, P., and Peng, S. (2018). A Comprehensive LVRT Strategy of Two-Stage Photovoltaic Systems under Balanced and Unbalanced Faults. Int. J. Electr. Power Energ. Syst. 103, 288–301. doi:10.1016/j.ijepes.2018.06.014
Jia, J., Yan, X., Wang, Y., Aslam, W., and Liu, W. (2020). Parameter Identification and Modelling of Photovoltaic Power Generation Systems Based on LVRT Tests. IET Generation, Transm. Distribution 14 (15), 3089–3098. doi:10.1049/iet-gtd.2019.1730
Li, F., Liu, M., Wang, Y., and Zhang, X. (2021). Research on HIL-Based HVRT and LVRT Automated Test System for Photovoltaic Inverters. Energ. Rep. 7, 405–412. doi:10.1016/j.egyr.2021.08.026
Li, Y., Han, M., and Yang, Z., (2021). Coordinating Flexible Demand Response and Renewable Uncertainties for Scheduling of Community Integrated Energy Systems with an Electric Vehicle Charging Station: A Bi-level Approach. IEEE Trans. Sust. Energ. 12, 2321–2331. doi:10.1109/tste.2021.3090463
Li, Y., Lei, Y., and Lin, X., (2019). Research on the Application of an SMES Based on Sliding Mode Control to Enhance the LVRT Capability of a Grid-Connected PV System. Electric Power Components Syst. 47 (9-10), 914–926. doi:10.1080/15325008.2019.1627612
Li, Y., Li, J., and Wang, Y. (2021). Privacy-preserving Spatiotemporal Scenario Generation of Renewable Energies: A Federated Deep Generative Learning Approach. IEEE Trans. Ind. Inform.
Li, Z., Lu, J., Liu, J., Zhu, Y., and He, Q. (2020). Resistance Selection and Control Strategy Considering the Coordination of Protection Performance and Low Voltage Ride through of a Large-Scale Photovoltaic Power Station. IEEE Access 8, 143746–143758. doi:10.1109/access.2020.3014232
Liu, H., Xu, K., Zhang, Z., Liu, W., and Ao, J. (2019). Research on Theoretical Calculation Methods of Photovoltaic Power Short-Circuit Current and Influencing Factors of its Fault Characteristics. Energies 12 (2), 316. doi:10.3390/en12020316
Mei, H., Jia, C., Fu, J., and Luan, X. (2021). Low Voltage Ride through Control Strategy for MMC Photovoltaic System Based on Model Predictive Control. Int. J. Electr. Power Energ. Syst. 125, 106530. doi:10.1016/j.ijepes.2020.106530
Sabir, A., and Ibrir, S. (2020). A Robust Control Scheme for Grid-Connected Photovoltaic Converters with Low-Voltage Ride-Through Ability without Phase-Locked Loop. ISA Trans. 96, 287–298. doi:10.1016/j.isatra.2019.05.027
Yan, X., Liang, B., Jia, J., Aslam, W., Wang, C., Zhang, S., et al. (2021). Strategies to Increase the Transient Active Power of Photovoltaic Units during Low Voltage Ride through. Energies 14 (17), 5236. doi:10.3390/en14175236
Yang, W., Deng, C., and Zheng, F. (2017). Low Voltage Ride-Through Capability Improvement of Photovoltaic Systems Using a Novel Hybrid Control. J. Renew. Sust. Energ. 9 (5), 055301. doi:10.1063/1.5005552
Yin, J. (2021). Research on Short-Circuit Current Calculation Method of Doubly-Fed Wind Turbines Considering Rotor Dynamic Process. Front. Energ. Res. 9, 204. doi:10.3389/fenrg.2021.686146
Zangeneh Bighash, E., Sadeghzadeh, S. M., Ebrahimzadeh, E., and Blaabjerg, F. (2018). Improving Performance of LVRT Capability in Single-phase Grid-Tied PV Inverters by a Model-Predictive Controller. Int. J. Electr. Power Energ. Syst. 98, 176–188. doi:10.1016/j.ijepes.2017.11.034
Keywords: photovoltaic power generation, whole process, low voltage ride through, control strategy, adaptive maximum power tracking
Citation: Yin J (2022) The Whole-Process Low-Voltage Ride-Through Control Strategy for Photovoltaic Power Generation Based on Adaptive Maximum Power Tracking. Front. Energy Res. 10:841415. doi: 10.3389/fenrg.2022.841415
Received: 22 December 2021; Accepted: 21 January 2022;
Published: 15 February 2022.
Edited by:
Wei Wang, North China Electric Power University, ChinaReviewed by:
Xingshuo Li, Nanjing Normal University, ChinaCopyright © 2022 Yin. This is an open-access article distributed under the terms of the Creative Commons Attribution License (CC BY). The use, distribution or reproduction in other forums is permitted, provided the original author(s) and the copyright owner(s) are credited and that the original publication in this journal is cited, in accordance with accepted academic practice. No use, distribution or reproduction is permitted which does not comply with these terms.
*Correspondence: Jun Yin, eWluanVuQG5jd3UuZWR1LmNu
Disclaimer: All claims expressed in this article are solely those of the authors and do not necessarily represent those of their affiliated organizations, or those of the publisher, the editors and the reviewers. Any product that may be evaluated in this article or claim that may be made by its manufacturer is not guaranteed or endorsed by the publisher.
Research integrity at Frontiers
Learn more about the work of our research integrity team to safeguard the quality of each article we publish.