- 1College of Veterinary Medicine and Institute of Veterinary Science, Kangwon National University, Chuncheon, South Korea
- 2Division of Chemical Engineering and Bioengineering, College of Art Culture and Engineering, Kangwon National University, Chuncheon, South Korea
- 3Biohealth-machinery Convergence Engineering, Kangwon National University, Chuncheon, South Korea
- 4Department of Food Science and Bio-Technology, Jeonnam State University, Jeonnam, South Korea
- 5Department of Emergency Medicine and BioMedical Science Graduate Program (BMSGP), Chonnam National University, Gwangju, South Korea
- 6Department of Emergency Medical Rescue, Nambu University, Gwangju, South Korea
- 7Tianjin Key Laboratory of Pulp and Paper, Tianjin University of Science and Technology, Tianjin, China
- 8Department of Forest Biomaterials Engineering, College of Forest and Environmental Sciences, Kangwon National University, Chuncheon, South Korea
This study was aimed to examine the antiapoptotic effect of Ulmus davidiana extracts through regulation of the intracellular cation mobilization in U937 human monocytic cells. To investigate the modulatory effects on lipopolysaccharide-induced apoptosis and the Ca2+ signaling pathway, we measured the levels of intracellular Ca2+ and various protein markers such as Bax, Bcl-2, and PARP. To isolate biopotent molecules, the branches of U. davidiana were processed sequentially using 60% ethanol, supercritical fluid extraction, and ethyl acetate extraction of the remaining samples to obtain single fractions and catechin-glycoside, which is one of the known bioeffector molecules of U. davidiana. Lipopolysaccharide increased intracellular Ca2+ mobilization in U937 cells by inducing transient oscillations and markedly increased Bax and PARP protein expression and decreased Bcl-2 expression. All U. davidiana and catechin-glycoside significantly reduced lipopolysaccharide-induced intracellular Ca2+ mobilization and downregulated apoptosis-related molecules. These results suggest that U. davidiana and catechin-glycoside may be useful for improving immune system function.
Introduction
The branches of Ulmus davidiana var. japonica (ULDA) has been used as a traditional Korean medicine for the treatment of inflammatory disorders (Lee, 1966; Hong et al., 1990; Kim et al., 2010). Previous pharmacological studies have reported that ULDA possesses antioxidant, anti-angiogenic, anticancer, and neuroprotective effects (Kim et al., 2005; Si et al., 2013a).
Recently, ULDA has also been used as a functional food for supplementation of amino acids (Oh et al., 2006), oligosaccharides (Eom et al., 2006), and other unknown metabolites that are involved in many biochemical metabolic processes (Carrillo and Borthakur, 2021).
Many studies have confirmed that ULDA contains several useful ingredients (Shin et al., 2000; Eom et al., 2006; Oh et al., 2006; Lee and Lim, 2007); however, the composition of the extract varies depending on the extraction method. The beneficial effects of ULDA have been recognized worldwide, including Asia, Europe, the United States (Xiu, 1988), and Korea, but its underlying mechanism of action on innate immunity and metabolism is not clear.
Recent studies indicate that ULDA extracts have various ameliorative effects on acute inflammatory responses in rats (Lee et al., 2013a; 2013b; Si et al., 2013b; Park et al., 2020), osteopenia (Zhuang et al., 2016), and in vitro models (Kim et al., 2019). Moreover, the supercritical fluid of ULDA has anti-inflammatory, anti-angiogenic (Jung and Park, 2006; Si et al., 2009b), and anti-melanin effects (Jeon et al., 2020; Xiong et al., 2021a; Xiong et al., 2021b).
Lipopolysaccharide (LPS), an important molecule in the microbial challenge, exerts an effect on intracellular Ca2+ levels [(Ca2+)i], the release of cytokines, and upstream signaling pathways (Azenabor et al., 2009). Intracellular Ca2+-dependent pathways mediated LPS-induced activation of transcriptional factors and iNOS expression in mouse J774 macrophages (Chen et al., 1998).
ADP-ribosyl cyclase(s) modulate concentration of [Ca2+]i by mobilizing intracellular Ca2+ stores or by Ca2+ influx through plasma membrane Ca2+ channels in various cells (Rah et al., 2007; Kim et al., 2009; Park et al., 2011). Ca2+ signaling modulation via ADP-ribose cyclase is involved in signal transduction, including cell growth, differentiation, and death (Kim et al., 1993; Mehta et al., 1996; Liu et al., 2022). In this study, we investigated whether pharmaceutical inhibition of Ca2+ could be effective in protecting cells against programmed cell death. To test this hypothesis, we compared Ca2+ signals and apoptosis markers such as Bcl-2, Bax, and PARP-1 in LPS and supercritical fluid-fractionated ULDA-treated LPS groups of U937 cells.
Therefore, this study was performed to investigate the effects of supercritical fluid-fractionated ULDA, including initial fractions of polyphenols, hydrophobic substances, and flavonoids, on innate immunity modulation and recovery of innate immune function in an in vitro model.
Materials and Methods
Extraction and Isolation of U. davidiana
In this study, extraction equipment was used for supercritical fluid extraction of U. davidiana branch (with bark). U. davidiana branch (with bark) was purchased from the Yangnyeongsi Medicine Market (Seoul, Korea), and impurities were removed, cleaned, and shaded for use as experimental material. One hundred kilograms of Ulmus davidiana branch (with bark) was extracted once with 60% edible ethanol at room temperature. The extract was then concentrated by removing 60% edible ethanol under vacuum to yield a quantity of 4.62 kg (U60E) (Figure 1). The dried sample was pulverized by passing through a 200 mesh screen and maintained at a temperature of 50°C in the pulverization tank. When the temperature stabilized, U. davidiana branch (with bark) samples were kept under CO2 gas at an equilibrium pressure of 400 bar, which was maintained through a control valve controlled by a high-pressure pump. After reaching the set pressure, extraction was performed by injecting 100 L of ethanol (300 ml/min) for 333 min to the bottom of the extraction tank. The high-pressure pump was set at a specific pressure and temperature for 30 min to remove the residual ethanol in the sample, and the extraction was completed by flowing CO2 gas. As described above, after supercritical extraction of 100 kg of U. davidiana branch (with bark) with 60% alcohol at room temperature, the extract was filtered, concentrated under vacuum, and freeze-dried to obtain 4.81 kg of the final product (USCFR) (Jeon et al., 2020). The filtrate (USCFR, 1 kg) was fractionated with ethyl acetate, and the ethyl acetate extract was concentrated under vacuum and freeze-dried to obtain 185.2 g of the final product (USCFREA) (Figure 2), which was dissolved in water and filtered through filter paper no. 20 (Hyundai Micro, Seoul, South Korea). Purification and isolation were performed by liquid column chromatography with TLC monitoring. In addition, 185 g of USCFREA on Disogel (300 g, 3 × 50 cm) with 30% methanol under isocratic conditions in a Prep-LC system (20 ml/min, 280 nm) yielded catechin 7-O-β-D-apiofuranoside (compound 1) (Figure 3).
Cell Culture
U937 cells were obtained from the American Type Culture Collection and maintained in complete RPMI 1640 medium supplemented with 10% fetal bovine serum and 1% antibiotics. After at least 14 days of proliferation, U937 cells were used for in vitro experiments.
Intracellular Ca2+ Measurement
Intracellular Ca2+ mobilization was measured as previously described (Park et al., 2011). Cells were plated manually on poly-L-lysine–coated confocal dishes (#100350; SPL, Pochun, Korea) and loaded with 1 mM Fluo-4 AM (Molecular Probes, CA, United States) at 37°C for 30 min. After washing with Hanks’ balanced salt solution medium containing 0.1% bovine serum albumin, changes in fluorescence were determined at 488 nm excitation/530 nm emission using an air-cooled argon laser system with a TE-2000 inverted microscope (Nikon, Tokyo, Japan) equipped with a temperature-controlled metal stage (Tokai Hit Co., Ltd., Shizuoka-ken, Japan). The emitted fluorescence at 530 nm was measured using a photomultiplier, and time series were acquired with a frame interval of 4 s. For the calculation of [Ca2+]i, the method described by Tsien et al. (1982) was applied with Kd for Fluo-4 using the equation [Ca2+]i = Kd (F − Fmin)/(Fmax − F). Each tracing was calibrated for maximal intensity (Fmax) by adding 8 mM ionomycin and for minimal intensity (Fmin) by adding 50 mM ethylene glycol tetraacetic acid at the end of each measurement.
Western Blot Analysis
Cell protein extracts were prepared immediately before use as follows: cells were solubilized by mixing with ice-cold lysis buffer [20 mM HEPES (pH 7.2), 1% Triton X-100, 10% glycerol, 1 mM EDTA, 1 mM phenylmethylsulfonyl fluoride, 50 mM NaF, 1 mM Na3VO4, leupeptin (10 mg/ml), pepstatin (10 mg/ml), and aprotinin (10 mg/ml)] by repeated trituration using a micropipette. The samples were then incubated for 1 h at 4°C. The supernatants were obtained after centrifugation at 20,000 × g for 10 min. The concentration of the extracted proteins in the supernatant was determined by the Bradford assay using bovine serum albumin as a standard protein. Equivalent micrograms of proteins per lane were resolved on 7–12% SDS-polyacrylamide gel and electrotransferred to a polyvinylidene difluoride membrane (GE Healthcare). Antibodies against Bax, Bcl-2, PARP, and actin were used. Horseradish peroxidase-conjugated secondary antibodies (Santa Cruz Biotechnology) were used and visualized using enhanced chemiluminescence (ECL). All immunoreactive signals were analyzed using densitometric scanning (LAS4000; GE Healthcare, United States).
Quantitative Chromatographic Analysis of U. davidiana Branch (With Bark)
HPLC analysis was conducted using a Waters 2,695 Separation Module (Waters Co., Milford, MA, United States) with a vacuum degasser, a binary pump, a 2,487 dual λ absorbance detector, a column compartment, and Empower software for data acquisition and integration. HPLC-grade reagents (J.T. Baker Co., Ltd., United States) were used for HPLC analysis (Si et al., 2008b, 2009a; An et al., 2019; Liu et al., 2021f). All solvents were filtered and degassed prior to use. The branches (with bark) of U. davidiana were accurately weighed to 2 g and dissolved in 50 ml of methanol. Samples were ultrasonicated for 15 min and filtered through filter paper no. 20 (600 × 600 mm, HYUNDAI Micro, Korea). The filtrate was further filtered through a 0.45-μm syringe filter (PVDF, Gelman, United States). Catechin 7-O-β-D apiofuranoside (1) was separated on a Phenomenex KJ0-4282 guard column and a SkyPakC18 column (5 μm C18 HPLC column, 5 μm, 250 × 4.6 mm; SK Chemical) with a linear gradient of [D.W.MeOH:P2HO4 (940:50:1)]: [MeOH:P2HO4 (990:1)] = 100:0 to 0:100 for 30 min. The column temperature was maintained at room temperature, and the flow rate was maintained at 1.0 ml/min. The sample injection volume was 20 μL. Eluted samples were monitored at 280 nm, and compound 1 was eluted at 14.45 ± 0.14 min. Compound 1 was detected in U. davidiana extracts. For these experiments, compound 1, U60E, USCFR, and USCFREA were prepared at a concentration of 1 mg/ml in methanol; the stock solutions were then diluted to 1,000, 500, 250, 125, and 62.5 ppm.
Statistical Analysis
All data are expressed as the mean ± SEM. One-way analysis of variance followed by Tukey’s multiple range test was used to compare each group (Si et al., 2008a; Du et al., 2019, 2022; Li et al., 2019, 2020; Liu et al., 2020a). Student’s t-test was used for comparison between the groups. Statistical analyses were conducted using SPSS for Windows (version 10.0; Chicago, IL, United States), and data indicated with different superscript letters represent significant difference at p < .05.
Results and Discussion
Identification of Single Compound and Function
Catechin 7-O-β-D-apiofuranoside (Figure 3) from the U60E [12.16 ± 0.15 μg/ml], USCFR [131.45 ± 0.18 ppm (μg/ml)], and USCFREA [350.98 ± 0.16 ppm (μg/ml)] fractions and the catechin-glycoside–rich fraction (Com.1 rich fraction) were determined using a calibration equation (y = 6,857.3x–42,331; R2 = 0.9985) (Figures 2, 3) (Table 1 and Table 2). The chemical structure of purified compound 1 (Figure 3) was determined using ChemDraw (PerkinElmer, MA, United States).
Definition of Compounds
In this study, we established a methodology to produce a high-content extract (Com.1 rich fraction) having a content of Com.1 10–30 times higher than that in general alcohol extracts, which overcame the obstacle to developing pharmaceuticals or functional materials derived from natural products. We believe that this new method will be helpful in obtaining large quantities of active substances (Figure 4).
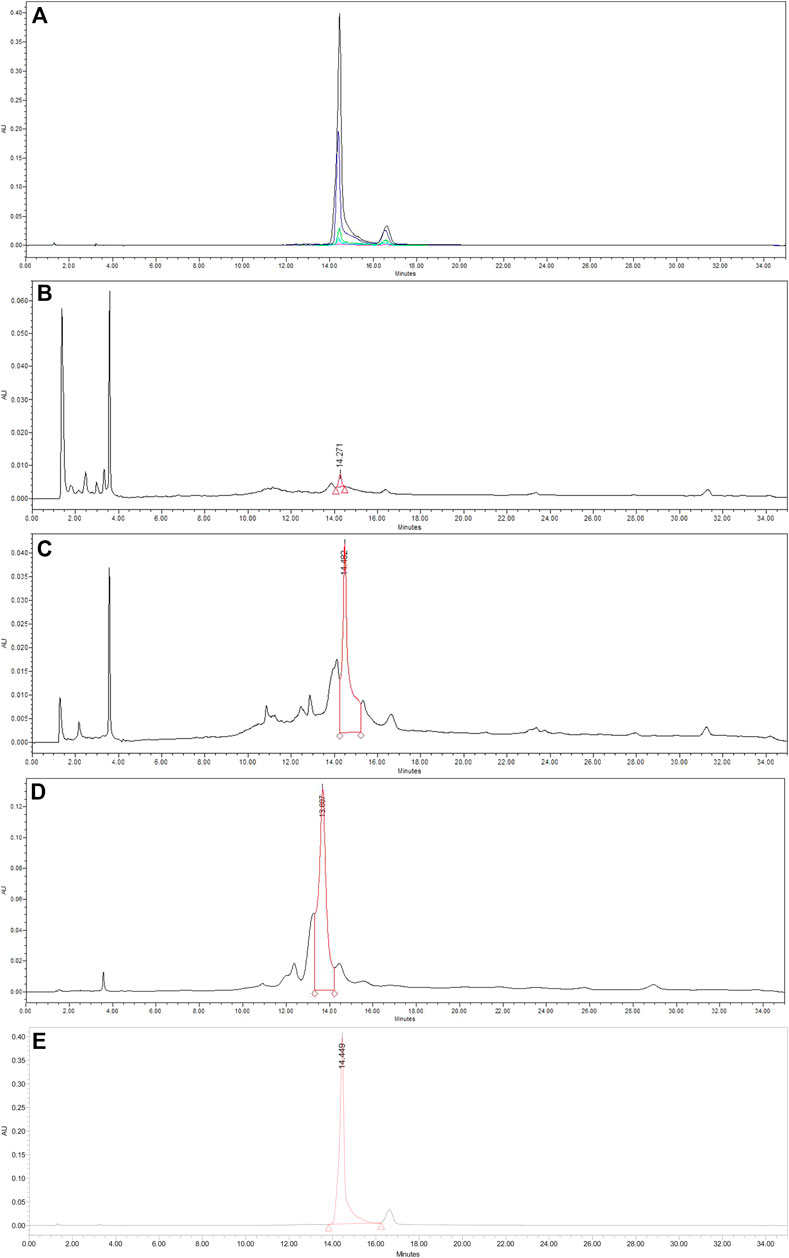
FIGURE 4. Representative HPLC chromatograms of (A) compound standard, (B) U60E [branch (with bark) 60% pre-ethanol A extract], (C) USCFR [branch (with bark) supercritical extraction residue 60% edible ethanolic extract from U. davidiana], (D) USCFREA [ethyl acetate layer extract of USCFR], and (E) catechin-glycoside–rich fraction. U60E = 1,000 ppm, USCFREA = 1,000 ppm, USCFR = 1,000 ppm, and catechin-glycoside–rich fraction = 1,000 ppm.
Regulation of Ca2+ Signal by ULDA Extracts and Catechin-Glycoside
We assessed whether LPS would increase intracellular Ca2+ mobilization in U937 cells, mimicking cell exposure to Gram-negative bacteria, and whether ULDA extracts and catechin-glycoside could protect cells from LPS exposure (Figure 5). Intracellular Ca2+ mobilization in LPS-treated U937 cells was monitored by confocal microscopy. We observed that LPS at micromolar concentration induced transient Ca2+ oscillations with medium amplitude in U937 cells (Figures 5B,E,H,K). Therefore, we determined whether ULDA extracts and catechin-glycoside (each 50 μg/ml) reduced Ca2+ mobilization in response to LPS treatment (Figures 5C,F,I,L). All types of ULDA extracts (U60E: Figures 5A–C panels; USCFR: Figures 5D–F panels; USCFREA: Figures 5G–I panels) and catechin-glycoside (Figures 5J–L panels) reduced LPS-induced Ca2+ mobilization within a few minutes of pretreatment. These results suggest that LPS induces Ca2+ mobilization and that ULDA extracts and catechin-glycoside can reduce LPS-induced damage signals in U937 cells.
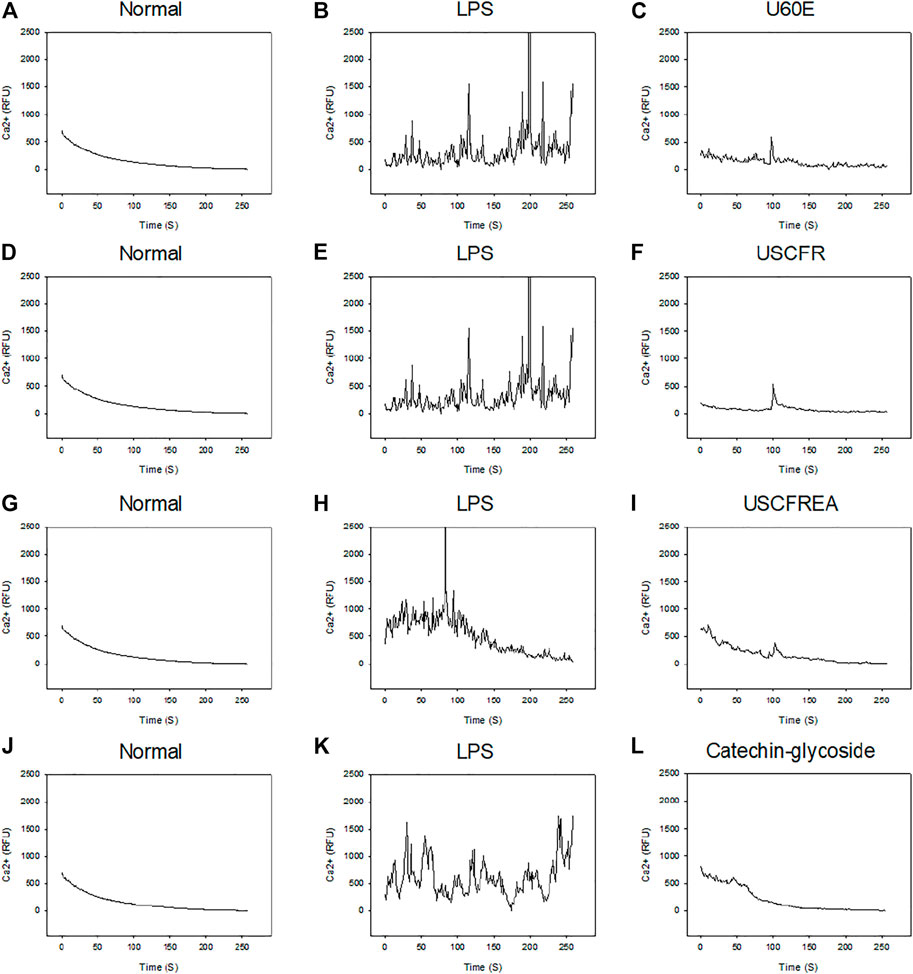
FIGURE 5. LPS-induced Ca2+ signaling and inhibition effects of U60E, USCFR, USCFREA, and catechin-glycoside–rich fraction. Cells were plated on confocal dishes and loaded with Ca2+-specific dye as detailed in Materials and Methods. LPS (1 μg/ml) was added and Ca2+ mobilization was monitored at the indicated time points. Normal (A,D,G,J) and LPS (B,E,H,K). (C) U60E, (F) USCFREA, (I) USCFR, and (L) catechin-glycoside–rich fraction (each 50 μg/ml) were pretreated for 10 min before LPS treatment. Data are representative of three independent experiments and values are expressed.
Antiapoptotic Effects on U937 Cells
Next, we examined apoptotic signaling response in U937 cells treated with 1 μg/ml LPS (Figures 6A–D). Administration of LPS increased the expression of apoptosis markers such as Bax and PARP and reduced the expression of Bcl-2, an antiapoptotic protein. Pretreatment with ULDA extracts (Figures 6A–C) and catechin-glycoside (Figure 6D) markedly reversed Bax and PARP protein expression levels; moreover, Bcl-2 expression was significantly elevated in a dose-dependent manner. Future comprehensive experimental studies might improve our understanding of other molecular pathways activated by ULDA extracts and catechin-glycosides in various disease-related signaling processes and help guide prospective clinical studies evaluating their effects and appropriate usage.
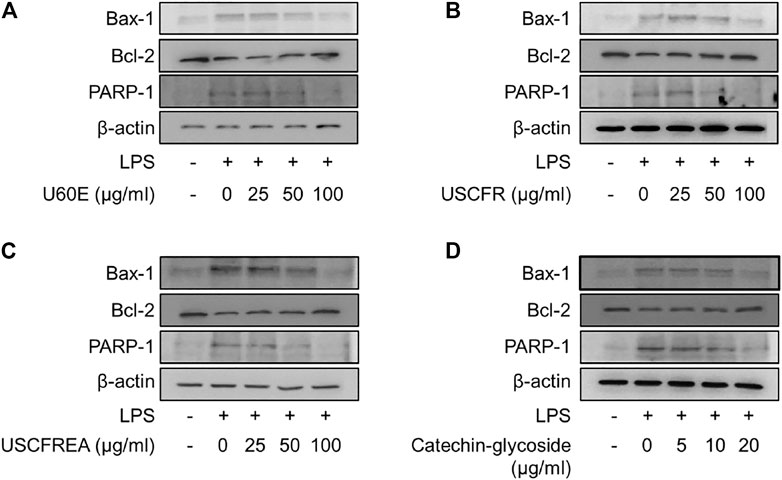
FIGURE 6. Inhibition effects of U60E, USCFREA, USCFR, and catechin-glycoside–rich fraction on LPS-induced apoptosis signaling in U937 cells. Cells were treated with LPS (1 μg/ml) in the presence or absence of the indicated doses of (A) U60E, (B) USCFR, (C) USCFREA, and (D) catechin-glycoside–rich fraction. Lysed cell extracts were analyzed by Western blotting using the indicated antibodies. U60E, USCFREA, USCFR, and catechin-glycoside–rich fraction were pretreated for 10 min before LPS treatment. The Western blot is representative of three independent experiments.
Lipopolysaccharide is a major virulence factor, and previous studies have demonstrated that LPS induces apoptosis in the murine macrophage-like cell line J744.1 (Suzuki et al., 2008; Ude et al., 2020), but not in humans. In this study, we emphasized the important role of the nuclear apoptotic pathway leading to Bax and PARP activation in LPS-induced apoptosis of human macrophages, U937 cells, and suggested that catechin-glycoside is a good candidate for amelioration of inflammatory disorders. However, when using natural materials with excellent physiological activity, it is necessary to review and consider the optimal process for biological manufacturing.
Intracellular Ca2+ mobilization is a universal signaling pathway in which cells respond to a wide range of external stimuli such as hormones, chemicals, and other organic/inorganic compounds (Lee and Zhao, 2019; Dara et al., 2021). Stimulation of Ca2+ mobilization is initiated by the activation of second messenger production in the cytosol, which activates Ca2+ release from stores, such as the endoplasmic reticulum and endolysosomes (Streb et al., 1983). Ca2+ influx played an important role in the [Ca2+]i mobilization pathway in U937 cells and well-defined LPS-induced cytokine production (Wehrhahn et al., 2010) via the transient receptor potential melastatin 2 (TRPM2) pathway. In addition, LPS-induced U937 apoptotic signaling is mediated by the transient receptor potential vanilloid subtype 1 (TRPV1) channel along with the cytosolic ROS signaling pathways (Güzel and Akpınar, 2021). These LPS-induced apoptotic signals are dependent upon mitochondrial dysfunction (Kuwabara and Imajoh-Ohmi, 2004), which is expected to be a parallel mechanism. In this study, we observed the effect of [Ca2+]i mobilization on LPS-induced cell apoptosis and inhibitory effects of ULDA extract fractions and catechin-glycoside in U937 cells, but the critical pathway was not defined clearly and needs to be further investigated.
In many similar studies, an optimal extraction method was developed to obtain large quantities of effective substances from the same amount of natural raw materials while considering cost, space, facilities, and infrastructure (Liu et al., 2017; Hu et al., 2018; Lu et al., 2019; Kumar et al., 2020; Wang et al., 2020; Xu et al., 2020c; Yang et al., 2020; Ha et al., 2021; Huang et al., 2021; Zheng et al., 2021). Accordingly, the most commonly used extraction methods in academia and industry include hot water extraction, ethanol extraction, ultrasonic extraction, pressurized extraction, and supercritical extraction (Si et al., 2011; Chen et al., 2016; Hu et al., 2017; Xie et al., 2018, 2019; Yang et al., 2019; Xu et al., 2020a, Xu et al., 2020b, Xu et al., 2021a, Xu et al., 2021b; Wang et al., 2021a; Liu et al., 2021a, 2021b, 2021c). Each extraction method has its advantages and disadvantages that should be considered in many ways at the laboratory level and future commercialization level (Dai et al., 2020a; Liu et al., 2020b; Liu et al., 2021d; Liu et al., 2021e; Dai et al., 2020b; Chen et al., 2020; Chen et al., 2021; Ma et al., 2021; Zhang et al., 2021). Among various extraction methods, supercritical extraction technology has the advantages of being eco-friendly, use of safe organic solvents, and avoidance of environmental pollution. Thus, we used supercritical extraction technology for obtaining U. davidiana extracts with low cytotoxicity (Mun et al., 2018; Seo et al., 2018). Traditionally, water and alcohols (methanol or ethanol) have been used for the extraction of basic ingredients in local and industrial fields, but the risks and advantages of nonspecific classification between molecules exist (Cho et al., 2017). Therefore, fractionation with various solvents following polarity, ionic strength, hydrophilicity, and hydrophobicity along with purification has been performed worldwide, and techniques for the synthesis of the same molecules have been developed (Rho et al., 2004; Nan et al., 2013; Ravichandiran et al., 2019; Fang et al., 2020; Wang et al., 2021b). In this study, we extracted effective molecules from the residual material by supercritical fluidic extraction (Mun et al., 2018; Seo et al., 2018). Considering that these residues could retain relatively hydrophilic molecules after supercritical fluid extraction, we performed common hydrophilic and hydrophobic extraction methods using 60% edible ethanol and ethyl acetate, respectively.
In this study, to examine the value of the supercritical extraction residue, physiological activity study was performed by extracting the supercritical extract of U. davidiana using 60% alcohol. In addition, ethyl acetate solvent analysis was conducted as a part of the production method to examine the added physiological value of U. davidiana supercritical extract and to verify the possibility of U. davidiana supercritical extraction residue as a new natural material.
Conclusion
The branches of Ulmus davidiana var. japonica (ULDA) has traditionally been used in Korea and other Asian countries. ULDA extracts are complex substances consisting of many components; a few of them have pharmaceutical applications in various diseases, such as inflammation and other chronic problems, and as antimicrobial agents.
In this study, we investigated the effects of supercritical fluid-fractionated ULDA, including initial fractions of polyphenols, hydrophobic substances, and flavonoids, on innate immunity modulation and recovery of innate immune function in an in vitro model. Future experiments are needed to investigate the beneficial effects of these resources in other diseases using in vivo models.
Data Availability Statement
The original contributions presented in the study are included in the article/Supplementary Material; further inquiries can be directed to the corresponding authors.
Author Contributions
Investigation, J-HY, YM, and H-OK; Supervision, CS, K-HP, and S-EC; Writing—original draft, S-EC and K-HP; Writing—review and editing, CS and J-HJ.
Funding
This research was supported by 2021 Universities Leading Lab-Specific Start-Ups through the National Research Foundation of Korea (NRF) grant funded by the Korean government (MSIT), the Key Technology Research and Development Program of Tianjin (19YFZCSN00950), the R and D program for Forest Science Technology (201703B10-1819-BA01, 2019151D10-2023-0301 and 2019151D10-2223-0301) provided by the Korea Forest Service (Korea Forestry Promotion Institute), and partially supported by the Technology Development Program (S3030198) funded by the Ministry of SMEs and Startups (MSS, Korea) to S-EC. This work was also partially supported by the National Research Foundation of Korea grant funded by the Korean government (2019R1I1A1A01057005 to H-OK, 2021R1A2C1091322 to K-HP, and NRF-2020R1I1A3071928 to H-JY).
Conflict of Interest
The authors declare that the research was conducted in the absence of any commercial or financial relationships that could be construed as a potential conflict of interest.
Publisher’s Note
All claims expressed in this article are solely those of the authors and do not necessarily represent those of their affiliated organizations, or those of the publisher, the editors, and the reviewers. Any product that may be evaluated in this article, or claim that may be made by its manufacturer, is not guaranteed or endorsed by the publisher.
References
An, L., Si, C., Wang, G., Sui, W., and Tao, Z. (2019). Enhancing the Solubility and Antioxidant Activity of High-Molecular-Weight Lignin by Moderate Depolymerization via In Situ Ethanol/acid Catalysis. Ind. Crops Prod. 128, 177–185. doi:10.1016/j.indcrop.2018.11.009
Azenabor, A. A., Kennedy, P., and York, J. (2009). Free Intracellular Ca2+ Regulates Bacterial Lipopolysaccharide Induction of iNOS in Human Macrophages. Immunobiology 214, 143–152. doi:10.1016/j.imbio.2008.06.001
Carrillo, J. T., and Borthakur, D. (2021). Do Uncommon Plant Phenolic Compounds have Uncommon Properties? A Mini Review on Novel Flavonoids. J. Bioresour. Bioprod. 6 (4), 279–291. doi:10.1016/j.jobab.2021.09.001
Chen, B.-C., Chou, C.-F., and Lin, W.-W. (1998). Pyrimidinoceptor-mediated Potentiation of Inducible Nitric-Oxide Synthase Induction in J774 Macrophages. J. Biol. Chem. 273, 29754–29763. doi:10.1074/jbc.273.45.29754
Chen, S., Wang, G., Sui, W., Parvez, A. M., Dai, L., and Si, C. (2020). Novel Lignin-Based Phenolic Nanosphere Supported Palladium Nanoparticles with Highly Efficient Catalytic Performance and Good Reusability. Ind. Crops Prod. 145, 112164. doi:10.1016/j.indcrop.2020.112164
Chen, T., Liu, H., Liu, J., Li, J., An, Y., Zhu, M., et al. (2021). Carboxymethylation of Polysaccharide Isolated from Alkaline Peroxide Mechanical Pulping (APMP) Waste Liquor and Its Bioactivity. Int. J. Biol. Macromol. 181, 211–220. doi:10.1016/j.ijbiomac.2021.03.125
Chen, X., Yang, Q., Si, C.-L., Wang, Z., Huo, D., Hong, Y., et al. (2016). Recovery of Oligosaccharides from Prehydrolysis Liquors of poplar by Microfiltration/ultrafiltration Membranes and Anion Exchange Resin. ACS Sustainable Chem. Eng. 4, 937–943. doi:10.1021/acssuschemeng.5b01029
Cho, E., Chung, E. Y., Jang, H.-Y., Hong, O.-Y., Chae, H. S., Jeong, Y.-J., et al. (2017). Anti-cancer Effect of Cyanidin-3-Glucoside from Mulberry via Caspase-3 Cleavage and DNA Fragmentation In Vitro and In Vivo. Acamc 17, 1519–1525. doi:10.2174/1871520617666170327152026
Dai, L., Cao, Q., Wang, K., Han, S., Si, C., Liu, D., et al. (2020a). High Efficient Recovery of L-Lactide with Lignin-Based Filler by thermal Degradation. Ind. Crops Prod. 143, 111954. doi:10.1016/j.indcrop.2019.111954
Dai, L., Ma, M., Xu, J., Si, C., Wang, X., Liu, Z., et al. (2020b). All-lignin-based Hydrogel with Fast pH-Stimuli Responsiveness for Mechanical Switching and Actuation. Chem. Mater. 32, 4324–4330. doi:10.1021/acs.chemmater.0c01198
Dara, P. K., Geetha, A., Mohanty, U., Raghavankutty, M., Mathew, S., Nagarajarao, R. C., et al. (2021). Extraction and Characterization of Myofibrillar Proteins from Different Meat Sources: A Comparative Study. J. Bioresour. Bioprod. 6 (4), 367–378. doi:10.1016/j.jobab.2021.04.004
Du, H., Liu, W., Zhang, M., Si, C., Zhang, X., and Li, B. (2019). Cellulose Nanocrystals and Cellulose Nanofibrils Based Hydrogels for Biomedical Applications. Carbohydr. Polym. 209, 130–144. doi:10.1016/j.carbpol.2019.01.020
Du, H., Zhang, M., Liu, K., Parit, M., Jiang, Z., Zhang, X., et al. (2022). Conductive PEDOT:PSS/cellulose Nanofibril Paper Electrodes for Flexible Supercapacitors with superior Areal Capacitance and Cycling Stability. Chem. Eng. J. 428, 131994. doi:10.1016/j.cej.2021.131994
Eom, S. Y., Chung, C. B., Kim, Y. S., Kim, J. H., Kim, K. S., Kim, Y. H., et al. (2006). Cosmeceutical Properties of Polysaccharides from the Root Bark of Ulmus Davidiana Var. Japonica. J. Cosmet. Sci. 57, 355–367.
Fang, X., Zhou, J., Wang, J., Tang, F., and Zhao, L. (2020). Preparation of Flavonoids from Osmanthus Dregs and Enzymatic Modification. J. For. Eng. 5, 99–105. doi:10.13360/j.issn.2096-1359.202006009
Güzel, M., and Akpınar, O. (2021). Hydroxychloroquine Attenuates Acute Inflammation (LPS)-Induced Apoptosis via Inhibiting TRPV1 Channel/ROS Signaling Pathways in Human Monocytes. Biology 10, 967. doi:10.3390/biology10100967
Ha, E. J., Yun, J.-H., Si, C., Bae, Y. S., Jeong, Y.-H., Park, K.-H., et al. (2021). Application of Ethanol Extracts from Alnus Sibirica Fisch. Ex Turcz in Hair Growth Promotion. Front. Bioeng. Biotechnol. 9, 673314. doi:10.3389/fbioe.2021.673314
Hong, N. D., Kim, N. J., No, Y. S., and Kim, J. S. (1990). A Study on Efficacy of Ulmi Cortex. Kor. J. Phamacogn. 21, 217–222.
Hu, L., Du, H., Liu, C., Zhang, Y., Yu, G., Zhang, X., et al. (2018). Comparative Evaluation of the Efficient Conversion of Corn Husk Filament and Corn Husk Powder to Valuable Materials via a Sustainable and Clean Biorefinery Process. ACS Sustainable Chem. Eng. 7, 1327–1336. doi:10.1021/acssuschemeng.8b05017
Hu, L., Wang, K., Li, G., Zhang, R., Luo, Y., Si, C.-L., et al. (2017). Isolation and Structural Elucidation of Heartwood Extractives of Juglans Sigillata. Holzforschung 71, 785–791. doi:10.1515/hf-2017-0036
Huang, L.-Z., Ma, M.-G., Ji, X.-X., Choi, S.-E., and Si, C. (2021). Recent Developments and Applications of Hemicellulose from Wheat Straw: a Review. Front. Bioeng. Biotechnol. 9, 690773. doi:10.3389/fbioe.2021.690773
Jeon, G., Ko, J.-Y., Mun, M.-J., Min, J., Choi, S.-E., and Bang, S. H. (2020). Effect of Melanin Reduction by Extracts from Ulmus Davidiana by Supercritical Fluid Extraction. Toxicol. Environ. Health Sci. 12, 325–329. doi:10.1007/s13530-020-00057-3
Jung, H. J., and Park, E. H. (2006). “Anti-inflanunatory, Anti-angiogenic and Analgesic Activities of Ulmus Davidiana Var. Japonica,” in Proceeding of the Convention of the Pharmaceutical Society of Korea (Busan, Seoul: The Pharmaceutical Society of Korea), 138–139.
Kim, E.-J., Jang, M.-K., Yoon, E.-H., Jung, C.-Y., Nam, D.-W., Lee, S.-D., et al. (2010). Efficacy of Pharmacopuncture Using Root Bark of Ulmus Davidiana Planch in Patients with Knee Osteoarthritis: a Double-Blind Randomized Controlled Trial. J. Acupuncture Meridian Stud. 3, 16–23. doi:10.1016/S2005-2901(10)60003-9
Kim, K.-S., Lee, S.-D., Kim, K.-H., Kil, S.-Y., Chung, K.-H., and Kim, C.-H. (2005). Suppressive Effects of a Water Extract of Ulmus Davidiana Planch (Ulmaceae) on Collagen-Induced Arthritis in Mice. J. Ethnopharmacology 97, 65–71. doi:10.1016/j.jep.2004.10.011
Kim, M., Park, K.-H., and Choi, S. E. (2019). Protective Effects of Supercritical Fluid Extracts of Ulmus Daviana Var. Japonica on LPS-Induced Immune Responses. ksbbj 34, 216–220. doi:10.7841/ksbbj.2019.34.3.216
Kim, S.-Y., Park, K.-H., Gul, R., Jang, K. Y., and Kim, U.-H. (2009). Role of Kidney ADP-Ribosyl Cyclase in Diabetic Nephropathy. Am. J. Physiology-Renal Physiol. 296, F291–F297. doi:10.1152/ajprenal.90381.2008
Kim, U.-H., Han, M.-K., Park, B.-H., Kim, H.-R., and An, N.-H. (1993). Function of NAD Glycohydrolase in ADP-Ribose Uptake from NAD by Human Erythrocytes. Biochim. Biophys. Acta (Bba) - Mol. Cell Res. 1178, 121–126. doi:10.1016/0167-4889(93)90001-6
Kumar, A., Sharma, G., Naushad, M., Al-Muhtaseb, A. a. H., García-Peñas, A., Mola, G. T., et al. (2020). Bio-inspired and Biomaterials-Based Hybrid Photocatalysts for Environmental Detoxification: A Review. Chem. Eng. J. 382, 122937. doi:10.1016/j.cej.2019.122937
Kuwabara, T., and Imajoh-Ohmi, S. (2004). LPS-induced Apoptosis Is Dependent upon Mitochondrial Dysfunction. Apoptosis 9, 467–474. doi:10.1023/B:APPT.0000031453.90821.6a
Lee, E.-H., Park, C.-W., and Jung, Y.-J. (2013a). Anti-inflammatory and Immune-Modulating Effect of Ulmus Davidiana Var. Japonica Nakai Extract on a Macrophage Cell Line and Immune Cells in the Mouse Small Intestine. J. Ethnopharmacology 146, 608–613. doi:10.1016/j.jep.2013.01.035
Lee, H.-S., Jang, M. S., Kim, J.-H., Hong, C.-P., Lee, E.-J., Jeun, E. J., et al. (2013b). Ulmus Davidiana Var. Japonica Nakai Upregulates Eosinophils and Suppresses Th1 and Th17 Cells in the Small Intestine. PLoS One 8, e76716. doi:10.1371/journal.pone.0076716
Lee, H. C., and Zhao, Y. J. (2019). Resolving the Topological enigma in Ca2+ Signaling by Cyclic ADP-Ribose and NAADP. J. Biol. Chem. 294, 19831–19843. doi:10.1074/jbc.REV119.009635
Lee, S.-J., and Lim, K.-T. (2007). Inhibitory Effect of Phytoglycoprotein on Tumor Necrosis Factor-α and Interleukin-6 at Initiation Stage of colon Cancer in 1,2-Dimethylhydrazine-Treated ICR Mice. Toxicol. Appl. Pharmacol. 225, 198–205. doi:10.1016/j.taap.2007.07.010
Li, X., Lu, X., Nie, S., Liang, M., Yu, Z., Duan, B., et al. (2020). Efficient Catalytic Production of Biomass-Derived Levulinic Acid over Phosphotungstic Acid in Deep Eutectic Solvent. Ind. Crops Prod. 145, 112154. doi:10.1016/j.indcrop.2020.112154
Li, X., Xu, R., Yang, J., Nie, S., Liu, D., Liu, Y., et al. (2019). Production of 5-hydroxymethylfurfural and Levulinic Acid from Lignocellulosic Biomass and Catalytic Upgradation. Ind. Crops Prod. 130, 184–197. doi:10.1016/j.indcrop.2018.12.082
Liu, C., Wang, G., Sui, W., An, L., and Si, C. (2017). Preparation and Characterization of Chitosan by a Novel Deacetylation Approach Using Glycerol as green Reaction Solvent. ACS Sustainable Chem. Eng. 5, 4690–4698. doi:10.1021/acssuschemeng.7b00050
Liu, H., Du, H., Zheng, T., Liu, K., Ji, X., Xu, T., et al. (2021a). Cellulose Based Composite Foams and Aerogels for Advanced Energy Storage Devices. Chem. Eng. J. 426, 130817. doi:10.1016/j.cej.2021.130817
Liu, H., Xu, T., Cai, C., Liu, K., Liu, W., Zhang, M., et al. (2022). Multifunctional Superelastic, Superhydrophilic, and Ultralight Nanocellulose-Based Composite Carbon Aerogels for Compressive Supercapacitor and Strain Sensor. Adv. Funct. Mater. 32, 2113082. doi:10.1002/adfm.202113082
Liu, H., Xu, T., Liu, K., Zhang, M., Liu, W., Li, H., et al. (2021b). Lignin-based Electrodes for Energy Storage Application. Ind. Crops Prod. 165, 113425. doi:10.1016/j.indcrop.2021.113425
Liu, K., Du, H., Liu, W., Liu, H., Zhang, M., Xu, T., et al. (2021c). Cellulose Nanomaterials for Oil Exploration Applications. Polym. Rev. 0, 1–41. doi:10.1080/15583724.2021.2007121
Liu, K., Du, H., Zheng, T., Liu, H., Zhang, M., Zhang, R., et al. (2021d). Recent Advances in Cellulose and its Derivatives for Oilfield Applications. Carbohydr. Polym. 259, 117740. doi:10.1016/j.carbpol.2021.117740
Liu, K., Du, H., Zheng, T., Liu, W., Zhang, M., Liu, H., et al. (2021e). Lignin-containing Cellulose Nanomaterials: Preparation and Applications. Green. Chem. 23, 9723–9746. doi:10.1039/D1GC02841C
Liu, W., Du, H., Liu, H., Xie, H., Xu, T., Zhao, X., et al. (2020a). Highly Efficient and Sustainable Preparation of Carboxylic and Thermostable Cellulose Nanocrystals via FeCl3-Catalyzed Innocuous Citric Acid Hydrolysis. ACS Sustainable Chem. Eng. 8, 16691–16700. doi:10.1021/acssuschemeng.0c06561
Liu, W., Du, H., Liu, K., Liu, H., Xie, H., Si, C., et al. (2021f). Sustainable Preparation of Cellulose Nanofibrils via Choline Chloride-Citric Acid Deep Eutectic Solvent Pretreatment Combined with High-Pressure Homogenization. Carbohydr. Polym. 267, 118220. doi:10.1016/j.carbpol.2021.118220
Liu, W., Du, H., Zhang, M., Liu, K., Liu, H., Xie, H., et al. (2020b). Bacterial Cellulose-Based Composite Scaffolds for Biomedical Applications: A Review. ACS Sustainable Chem. Eng. 8, 7536–7562. doi:10.1021/acssuschemeng.0c00125
Lu, J., Zhu, W., Dai, L., Si, C., and Ni, Y. (2019). Fabrication of Thermo- and pH-Sensitive Cellulose Nanofibrils-Reinforced Hydrogel with Biomass Nanoparticles. Carbohydr. Polym. 215, 289–295. doi:10.1016/j.carbpol.2019.03.100
Ma, C., Ma, M. G., Si, C., Ji, X. X., and Wan, P. (2021). Flexible MXene‐Based Composites for Wearable Devices. Adv. Funct. Mater. 31, 2009524. doi:10.1002/adfm.202009524
Mehta, K., Shahid, U., and Malavasi, F. (1996). Human CD38, a Cell‐surface Protein with Multiple Functions. FASEB j. 10, 1408–1417. doi:10.1096/fasebj.10.12.8903511
Mun, M.-J., Park, K.-H., and Choi, S. E. (2018). Biological Activity of Supercritical Extraction Residue 60% Ethanolic Extracts from Ulmus Davidiana. J. Converg. Inf. Technol. 8, 29–36. doi:10.22156/CS4SMB.2018.8.5.029
Nan, H., Yoo, D. J., and Kang, S. H. (2013). Fast Parallel Detection of Feline Panleukopenia Virus DNA by Multi-Channel Microchip Electrophoresis with Programmed Step Electric Field Strength. J. Sep. Sci. 36, 350–355. doi:10.1002/jssc.201200721
Oh, P. S., Lee, S. J., and Lim, K. T. (2006). Glycoprotein (116 kD) Isolated from Ulmus Davidiana Nakai Protects from Injury of 12-O-Tetradecanoylphorbol 13-acetate (TPA)-treated BNL CL.2 Cells. Pharmacol. Rep. 58, 67–74.
Park, K.-H., Chung, E.-Y., Choi, Y.-N., Jang, H.-Y., Kim, J.-S., and Kim, G.-B. (2020). Oral Administration of Ulmus Davidiana Extract Suppresses Interleukin-1β Expression in LPS-Induced Immune Responses and Lung Injury. Genes Genom. 42, 87–95. doi:10.1007/s13258-019-00883-x
Park, K.-H., Kim, B.-J., Kang, J., Nam, T.-S., Lim, J. M., Kim, H. T., et al. (2011). Ca 2+ Signaling Tools Acquired from Prostasomes Are Required for Progesterone-Induced Sperm Motility. Sci. Signal. 4 (173), ra31. doi:10.1126/scisignal.2001595
Rah, S.-Y., Park, K.-H., Nam, T.-S., Kim, S.-J., Kim, H., Im, M.-J., et al. (2007). Association of CD38 with Nonmuscle Myosin Heavy Chain IIA and Lck Is Essential for the Internalization and Activation of CD38. J. Biol. Chem. 282, 5653–5660. doi:10.1074/jbc.m609478200
Ravichandiran, P., Subramaniyan, S. A., Kim, S.-Y., Kim, J.-S., Park, B.-H., Shim, K. S., et al. (2019). Synthesis and Anticancer Evaluation of 1,4-Naphthoquinone Derivatives Containing a Phenylaminosulfanyl Moiety. ChemMedChem 14, 532–544. doi:10.1002/cmdc.201800749
Rho, Y. S., Kim, S. Y., Kim, W. J., Yun, Y. K., Sin, H. S., and Yoo, D. J. (2004). Convenient Syntheses of Daunomycinone‐7‐D‐Glucuronides and Doxorubicinone‐7‐D‐Glucuronides. Synth. Commun. 34, 3497–3511. doi:10.1081/scc-200030978
Seo, J.-H., Lee, Y.-J., Jo, Y.-I., Ko, J.-Y., Mun, M.-J., Park, K.-H., et al. (2018). Anti-fungal, Anti-oxidant, and Anti-inflammatory Effects of Supercritical Fluid Extracts from Ulmus Davidiana. J. Korea Convergence Soc. 9, 225–233. doi:10.15207/JKCS.2018.9.8.225
Shin, D.-Y., Kim, H.-S., Min, K.-H., Hyun, S.-S., Kim, S.-A., Huh, H., et al. (2000). Isolation of a Potent Anti-MRSA Sesquiterpenoid Quinone from Ulmus Davidiana Var. Japonica. Chem. Pharm. Bull. 48, 1805–1806. doi:10.1248/cpb.48.1805
Si, C.-L., Deng, X.-J., Liu, Z., Kim, J.-K., and Bae, Y.-S. (2008a). Studies on the Phenylethanoid Glycosides with Anti-complement Activity fromPaulownia tomentosavar.Tomentosawood. J. Asian Nat. Prod. Res. 10, 1003–1008. doi:10.1080/10286020802242364
Si, C.-L., Jiang, J.-Z., Liu, S.-C., Hu, H.-Y., Ren, X.-D., Yu, G.-J., et al. (2013a). A New Lignan Glycoside and Phenolics from the branch wood of Pinus Banksiana Lambert. Holzforschung 67, 357–363. doi:10.1515/hf-2012-0137
Si, C.-L., Kim, J.-K., Bae, Y.-S., and Li, S.-M. (2009a). Phenolic Compounds in the Leaves ofPopulus Ussuriensisand Their Antioxidant Activities. Planta Med. 75, 1165–1167. doi:10.1055/s-0029-1185476
Si, C.-L., Liu, Z., Kim, J.-K., and Bae, Y.-S. (2008b). Structure Elucidation of Phenylethanoid Glycosides from Paulownia Tomentosa Steud. Var. Tomentosa wood. Holzforschung 62, 197–200. doi:10.1515/hf.2008.047
Si, C.-L., Shen, T., Jiang, Y.-Y., Wu, L., Yu, G.-J., Ren, X.-D., et al. (2013b). Antioxidant Properties and Neuroprotective Effects of Isocampneoside II on Hydrogen Peroxide-Induced Oxidative Injury in PC12 Cells. Food Chem. Toxicol. 59, 145–152. doi:10.1016/j.fct.2013.05.051
Si, C.-L., Wu, L., and Zhu, Z.-Y. (2009b). Phenolic Glycosides from Populus Davidiana Bark. Biochem. Syst. Ecol. 37, 221–224. doi:10.1016/j.bse.2009.01.007
Si, C.-L., Xu, J., Kim, J.-K., Bae, Y.-S., Liu, P.-T., and Liu, Z. (2011). Antioxidant Properties and Structural Analysis of Phenolic Glucosides from Bark of Populus Ussuriensis Kom. Wood Sci. Technol. 45, 5–13. doi:10.1007/s00226-009-0286-x
Streb, H., Irvine, R. F., Berridge, M. J., and Schulz, I. (1983). Release of Ca2+ from a Nonmitochondrial Intracellular Store in Pancreatic Acinar Cells by Inositol-1,4,5-Trisphosphate. Nature 306, 67–69. doi:10.1038/306067a0
Suzuki, C., Kimoto-Nira, H., Kobayashi, M., Nomura, M., Sasaki, K., and Mizumachi, K. (2008). Immunomodulatory and Cytotoxic Effects of Various Lactococcus Strains on the Murine Macrophage Cell Line J774.1. Int. J. Food Microbiol. 123, 159–165. doi:10.1016/j.ijfoodmicro.2007.12.022
Tsien, R. Y., Pozzan, T., and Rink, T. J. (1982). Calcium Homeostasis in Intact Lymphocytes: Cytoplasmic Free Calcium Monitored with a New, Intracellularly Trapped Fluorescent Indicator. J. Cell Biol. 94, 325–334. doi:10.1083/jcb.94.2.325
Ude, M. U., Oluka, I., and Eze, P. C. (2020). Optimization and Kinetics of Glucose Production via Enzymatic Hydrolysis of Mixed Peels. J. Bioresour. Bioprod. 5, 283–290. doi:10.1016/j.jobab.2020.10.007
Wang, H., Du, H., Liu, K., Liu, H., Xu, T., Zhang, S., et al. (2021a). Sustainable Preparation of Bifunctional Cellulose Nanocrystals via Mixed H2SO4/formic Acid Hydrolysis. Carbohydr. Polym. 266, 118107. doi:10.1016/j.carbpol.2021.118107
Wang, H., Xie, H., Du, H., Wang, X., Liu, W., Duan, Y., et al. (2020). Highly Efficient Preparation of Functional and Thermostable Cellulose Nanocrystals via H2SO4 Intensified Acetic Acid Hydrolysis. Carbohydr. Polym. 239, 116233. doi:10.1016/j.carbpol.2020.116233
Wang, S., Liu, F., Zhou, Q., Xu, S., Huang, S., and Luo, J. (2021b). Preparation of Cedrol and Cedryl Acetate and Their Antifungal Activities. J. For. Eng. 6, 74–80. doi:10.13360/j.issn.2096-1359.202007036
Wehrhahn, J., Kraft, R., Harteneck, C., and Hauschildt, S. (2010). Transient Receptor Potential Melastatin 2 Is Required for Lipopolysaccharide-Induced Cytokine Production in Human Monocytes. J.I. 184, 2386–2393. doi:10.4049/jimmunol.0902474
Xie, H., Du, H., Yang, X., and Si, C. (2018). Recent Strategies of Cellulose Nanocrystals and Cellulose Nanofibrils Derived from Raw Cellulose Materials. Int. J. Polym. Sci. 2018, 7923068. doi:10.1155/2018/7923068,7923068
Xie, H., Zou, Z., Du, H., Zhang, X., Wang, X., Yang, X., et al. (2019). Preparation of Thermally Stable and Surface-Functionalized Cellulose Nanocrystals via Mixed H2SO4/Oxalic Acid Hydrolysis. Carbohydr. Polym. 223, 115116. doi:10.1016/j.carbpol.2019.115116
Xiong, R., Hua, D., Hoeck, J. V., Berdecka, D., Léger, M., Munter, S. D., et al. (2021a). Photothermal Nanofibres Enable Safe Engineering of Therapeutic Cells. Nat. Nanotechnol. 16, 1281–1291. doi:10.1038/s41565-021-00976-3
Xiong, R., Xu, R. X., Huang, C., De Smedt, S., and Braeckmans, K. (2021b). Stimuli-responsive Nanobubbles for Biomedical Applications. Chem. Soc. Rev. 50, 5746–5776. doi:10.1039/C9CS00839J
Xiu, R. J. (1988). Microcirculation and Traditional Chinese Medicine. JAMA 260, 1755–1757. doi:10.1001/jama.260.12.1755
Xu, J., Li, C., Dai, L., Xu, C., Zhong, Y., Yu, F., et al. (2020a). Biomass Fractionation and Lignin Fractionation towards Lignin Valorization. ChemSusChem 13, 4284–4295. doi:10.1002/cssc.202001491
Xu, R., Du, H., Liu, C., Liu, H., Wu, M., Zhang, X., et al. (2021a). An Efficient and Magnetic Adsorbent Prepared in a Dry Process with Enzymatic Hydrolysis Residues for Wastewater Treatment. J. Clean. Prod. 313, 127834. doi:10.1016/j.jclepro.2021.127834
Xu, R., Liu, K., Du, H., Liu, H., Cao, X., Zhao, X., et al. (2020c). Falling Leaves Return to Their Roots: A Review on the Preparation of γ-Valerolactone from Lignocellulose and Its Application in the Conversion of Lignocellulose. ChemSusChem 13, 6461–6476. doi:10.1002/cssc.202002008
Xu, R., Si, C., Kong, F., and Li, X. (2020b). Synthesis of γ-valerolactone and its Application in Biomass Conversion. J. For. Eng. 5, 20–28. doi:10.13360/j.issn.2096-1359.201904004
Xu, T., Du, H., Liu, H., Liu, W., Zhang, X., Si, C., et al. (2021b). Advanced Nanocellulose‐Based Composites for Flexible Functional Energy Storage Devices. Adv. Mater. 33, 2101368. doi:10.1002/adma.202101368
Yang, J., Si, C., Liu, K., Liu, H., Li, X., and Liang, M. (2020). Production of Levulinic Acid from Lignocellulosic Biomass and Application. J. For. Eng. 5, 21–27. doi:10.13360/j.issn.2096-1359.201905013
Yang, X., Xie, H., Du, H., Zhang, X., Zou, Z., Zou, Y., et al. (2019). Facile Extraction of Thermally Stable and Dispersible Cellulose Nanocrystals with High Yield via a Green and Recyclable FeCl3-Catalyzed Deep Eutectic Solvent System. ACS Sustainable Chem. Eng. 7, 7200–7208. doi:10.1021/acssuschemeng.9b00209
Zhang, M., Du, H., Liu, K., Nie, S., Xu, T., Zhang, X., et al. (2021). Fabrication and Applications of Cellulose-Based Nanogenerators. Adv. Compos. Hybrid Mater. 4, 865–884. doi:10.1007/s42114-021-00312-2
Zheng, X., Zhao, Y., Pan, B., and Wang, X. (2021). Extraction, Distribution and Composition of Tannin in Picea Sitchensis. J. For. Eng. 6, 108–113. doi:10.13360/j.issn.2096-1359.202007026
Keywords: Ulmus davidiana extract, supercritical fluid extraction, intracellular Ca2+ signaling, LPS-induced apoptosis, catechin-glycoside
Citation: Yun J-H, Kim H-O, Jeong J-H, Min Y, Park K-H, Si C and Choi S-E (2022) Ulmus davidiana var. japonica Extracts Suppress Lipopolysaccharide-Induced Apoptosis Through Intracellular Calcium Modulation in U937 Macrophages. Front. Energy Res. 10:820330. doi: 10.3389/fenrg.2022.820330
Received: 22 November 2021; Accepted: 17 January 2022;
Published: 02 March 2022.
Edited by:
Chen Huang, Chinese Academy of Forestry, ChinaCopyright © 2022 Yun, Kim, Jeong, Min, Park, Si and Choi. This is an open-access article distributed under the terms of the Creative Commons Attribution License (CC BY). The use, distribution or reproduction in other forums is permitted, provided the original author(s) and the copyright owner(s) are credited and that the original publication in this journal is cited, in accordance with accepted academic practice. No use, distribution or reproduction is permitted which does not comply with these terms.
*Correspondence: Kwang-Hyun Park, a2hwYXJrQG5hbWJ1LmFjLmty; Chuanling Si, c2ljaGxpQHR1c3QuZWR1LmNu; Sun-Eun Choi, b3JlZ29uaW5Aa2FuZ3dvbi5hYy5rcg==
†These authors have contributed equally to this work and share first authorship