- 1School of Textile Science and Engineering, Wuhan Textile University, Wuhan, China
- 2Laboratoire des Sciences du Climat et de I’Environnement (LSCE), IPSL, CEA/CNRS/UVSQ, Université Paris-Saclay, Paris, France
- 3Finance Department, Wuhan Textile University, Wuhan, China
- 4School of Electronic Information Engineering, Wuchang Polytechnic College, Wuhan, China
The study explores into the dynamic change features and technological differences in substitution between factors and energy sources for various types of China’s technological progresses from 1990 to 2020. The measurement for such a study is conducted from the perspective of factor substitution by employing the transcendental logarithmic production function. The results reveal that the sources of contribution to China’s economic development are mainly attributed to non-energy factors such as capital and labor, as capital and labor can effectively substitute energy, and non-fossil energy sources possess certain comparative advantages over fossil energy sources in terms of technology within energy factors. With such an increase in substitution, the trend of clean energy substitution for fossil energy is irreversible. Accordingly, it is proposed that the path for energy conservation and consumption reduction via energy transformation be achieved by increasing input into capital and labor to improve the utilization efficiency of these two factors from the perspective of factor substitution. Meanwhile, preferences should be delivered for the development of non-fossil energy sources in terms of technology bias and input scale.
1 Introduction
China’s economic development has long been marked by inefficient growth with high inputs. However, it now faces pressing constraints such as energy shortage, diminishing demographic dividends and structural adjustment, as well as the environmental problems that affect social stability and sustainable economic development. In this context, energy substitution serves as the key to solving some of these problems, as the consumption of non-clean energy sources continues to accelerate with the increase in global economic development and the higher cost of restoring the destruction caused by traditional energy sources to the external environment over time, making the substitution of non-fossil energy sources for fossil energy sources a must (Cantarero, 2020). Although there is no consensus on such an issue as to when fossil energy will be exhausted, the increasing consumption of fossil energy is bringing this deadline closer. Once the marginal cost of non-fossil energy is lower than that of fossil energy, fossil energy will gradually lose its advantage (Chen et al., 2016). But for now, fossil energy still has a cost advantage and path dependence over non-fossil energy, while the current economic development models and energy utilization patterns are incurring serious global climate problems. We need to achieve cost reductions and reduce such uncertainties by fully taking advantage of technological progress. For China, bringing a change in the current excessively distorted energy consumption composition is imminent, but a large-scale clean energy utilization pathway has not yet been developed.
The underlying cause for motivating energy alternatives might be attributed to the current energy consumption patterns resulting in deterioration of the climate (Zhao et al., 2022), while the excessive utilization of fossil fuels in the share of energy mix is in clear conflicts with the philosophy of ecological conservation and green development. Moreover, the coal-dominated consumption pattern in China’s energy mix is also the main cause for this inefficiency in energy utilization (Han et al., 2018). With the increasing energy demand for China’s economic development, the urge for a green and efficient practice in utilization of its energy mix has been put on agenda. With the constraint of emission reduction target, the government is also obliged to abandon its path dependence on traditional energy sources ahead of time in this context. Definitely, the earlier the deadline comes to embrace this alternative path to bring into function, the lower pressure will be imposed on the whole national economic system of China. As China attaches more emphasis on its idea of green and sustainable development by such intensified policies, its energy consumption just keeps declining, yet the scope of such a decline is shrinking gradually. For government sectors, it poses a tough task to maintain robust economic growth in response to such a requirement for ever declining consumption of energy. Amid the context of optimized factor allocation efficiency and current technological progress, how to attain the goal of economic growth with clean and efficient energy alternatives? How to attain the goal of energy conservation and lowering energy consumption, while exploring a rational combination of factor inputs and optimizing utilizations in the energy mix? All these issues are of crucial concern for the social development of our current days.
2 Review of relevant studies
Fruitful achievements have been made concerning the research on factor substitution and economic growth by scholars both at home and abroad. For example, Zheng (2016) measured the capital-labor substitution elasticity of 71 countries in the world, indicating that the improvement of factor substitution elasticity helps promote economic growth. Han et al. (2013) evaluated the energy conservation potential under the allocation circumstances by different factor inputs. The overall consideration of interaction effects among production factors and rational allocation of factor structure can effectively improve energy utilization efficiency. However, the imperfect allocation of factor structure in China has thwarted the effects of energy conservation. Berndt and Wood (1975) considered energy an important factor of production and incorporated it into the production function model with other factors. Omisakin (2008) analyzed the relationship between energy consumption and economic growth. The results indicate a unidirectional relationship between energy consumption and economic growth. Qi and Wang (2013) pointed out that factor substitution helps to reduce energy consumption, and Shi et al. (2010) further concluded that capital could effectively replace energy input and improve energy utilization efficiency. Yang et al. (2011) measured the dynamic change of elasticity between energy and non-energy factors in the economic system, indicating the existence of bidirectional casualty between economic growth and energy consumption, with impacts on energy consumption caused by capital stock. Liu (2014) took into account the role of human capital in the process of energy substitution, and found that it also plays a core role in this process, which radically changes the combination structure between energy and capital as well as inputs of various energy factors. Meanwhile, the attempt to strengthen the role of capital as a substitute for energy effectively alleviates the inhibition effects of energy constraints on economic growth. Likewise, distinctions in substitution effects resulting from industrial factors in economic activities exist. For heavy industry, the factor inputs are classified as capital and energy-intensive. The increase in capital helps to realize the goal of energy utilization efficiency and energy saving in the heavy industry sector, while for the light industry sector, technological progress yields no significant effects on energy conservation (Lin and Liu, 2017; Lin and Tian, 2017).
Some scholars are beginning to consider the impact of substitution relationships between energy sources on energy efficiency and economic growth, and the solution to global warming and energy shortages also relies to a great extent on substituting new energy sources for fossil energy sources and non-energy factors for energy factors (Li and Lin, 2016). Ma and Stern (2016) estimated both the long-term inter- and intra-factor elasticity of substitution, showing that the elasticity of substitution between fossil energy sources is limited, while the elasticity between energy and labor in the long-term process will be greater than the possibility of substitution between energy and capital. Yan et al. (2015) argued that energy restructuring is determined by inter-energy substitutability, and non-fossil energy sources have the strongest possibility of replacing fossil energy sources. But intra-energy substitution requires long-term support in both technological research and development with the promotion of large-scale applications (He et al., 2017). Lin et al. (2016) explored the impact of substitutability between factors and different energy sources on the energy economy, and the results indicate that all factors are substitutable with technological improvements resulting from the resource allocation-oriented cleaner energy sources, which will effectively improve the environment and reduce carbon emissions. Steinbuks and Narayanan (2015) analyzed energy substitution in the industrial sectors of 63 countries. The results show that fossil energy-producing countries can have higher energy substitution elasticity. For China, the massive use of fossil energy sources is both a challenge and a new opportunity for economic growth, provided the factor input structure can be effectively optimized. Sun and Nie (2014) also analyzed the substitution of renewable energy for non-renewable energy from a dynamic perspective, which indicates that the development of non-renewable energy is limited by resource reserves, while renewable energy faces the limitation of technological expertise, and such a limitation can be naturally settled with the increasing accumulation of technology. Expanding the share of clean energy can also bring optimization of the energy utilization structure, which is also an inevitable path towards a sustainable and environment-friendly economy.
Technological progress can shift the structure of energy consumption to new energy sources via the introduction of new energy technologies, thus improving the level of energy utilization (Wang and Fan, 2017), which can be divided into neutral and biased categories in terms of the effects of technological progress on factor allocation. However, most studies are based on Hicks’ assumption of neutral technological progress. Under the assumption of neutral technological progress, Zheng and Liu (2004) calculated the output elasticity of various factor inputs in the economic system and the variability of technological progress; Huang and Hu (2013) also pointed out that technological progress is not necessarily effective in achieving the goals of energy conservation due to the rebound effect, which may also generates such results as substitution effects and lead to an increase rather than decrease for energy demand. Neutral technological progress can ensure the balance of labor and capital and stable economic growth, while the differentiated characteristics of factor inputs lead to non-neutral technology, and the differences in the technological progress of different factors also affect the output effect. Wang and Qi (2014) pointed out that it is not accurate to consider only neutral technological progress and ignore the role of biased technological progress, and they also approve the impact of biased technological progress on energy intensity. Zhang et al. (2017) studied the impact of property rights structure on regional energy efficiency in the framework of biased technological progress, and the result shows that biased technological progress can effectively promote the adjustment of the energy mix for realization of energy substitution. Cai (2014) also pointed out that the depletion of energy will impact the sustainability of economic development, while the energy shortage can be effectively circumvented with the provision of sufficient labor input and energy-biased technological progress in economic system; Dong and Zhao (2017) measured the preference of eight sources of technological progress and their effects on energy intensity, the results indicating that different technological sources have significant differences on energy intensity. Wang et al. (2021) found that technological progress and factor allocation in China’s renewable energy industry shows a bias toward the capital. From the perspective of energy, however, the growth of non-fossil energy inputs, although beneficial to improving the environment, is achieved at the expense of economic growth and likely to conflict with economic growth. To promote energy substitution at a lower economic cost, utilization of neutral technological progress will enable us to fully exploit the scale and learning effects of renewable energy for effective improvement of the situation (Qi and Li, 2017). But the more advanced technology in non-fossil energy allows for relatively lower R&D costs and diminishes the negative economic impacts of the crowding-out effect, so neutral technologies and energy-biased technological advances are manifesting their importance for energy substitution and optimization of the energy mix.
Current research on measuring factor elasticity, technological progress, and energy substitution elasticity is mainly conducted by employing transcendental logarithmic function, CES method, multiple elasticity measure, panel vector autoregressive model, and endogenous economic growth model (Solarin and Bello, 2021; Vo, 2021; Zhu et al., 2021), and the most frequently employed are transcendental logarithmic production function and cost function. Compared to other methods, transcendental logarithmic function model is an easy-to-estimate and inclusive variable elasticity production function model, which can effectively study the interaction effects of input factors in the production process and the differences in technical progress of various inputs. Meanwhile, due to the relatively low share of energy in aggregate costs and the low portion of marketization in domestic energy prices, possibly there exists a relatively large derivation in the analysis of the results of the transcendental logarithmic cost function. Hence, this paper employs the transcendental logarithmic production function to measure the dynamic evolution characteristics of factor substitution and inter-energy substitution for economic development. Most existing studies measure the output elasticity between factors and the elasticity of substitution under the assumption of Hicks’ neutral technological progress, part of them also take into account the biased nature of technological progress. Neutral and biased technological progresses can coexist and have simultaneous effects on economic development and factor substitution. Hence, the study is conducted on the basis of whether energy factor inputs need to be subdivided into fossil energy or not, and measures the existence of neutral technological progress as well as biased technological progress to assess the level of substitution of different factors under comprehensive technological progress. On such a basis, it is our goal to measure the elasticity of substitution between factors and energy sources, and discover suitable alternative paths for energy transition with new impetus for economic development from the perspective of factor substitution. The main contributions of the study are: 1) the analysis of the impact of technological advances from different sources in the energy transition and 2) the exploration of the potential of different elements to contribute to a clean energy-oriented energy transition.
3 Research methodology and data acquisition
While capital and labor serve as the core factors in propelling economic development, energy consumption also has an equally important impact on economic development. By measuring the elasticity characteristics of factor inputs and outputs, as well as the internal input environment of energy substitution, we can adjust the technological progress and factor allocation accordingly to achieve a sustainable energy utilization structure for the sake of energy conservation by accomplishing the restructuring from fossil energy to non-fossil energy, so as to realize coordinated development in terms of energy, economy, and society.
The transcendental logarithmic production function can effectively measure the output contribution of factor inputs, the interaction mechanism, and the differentiation in the level of technological development in the economic system. Based on this, the study adopts transcendental logarithmic production function (Wang and Xie, 2013; Hao, 2015) to investigate the dynamic characteristics of the elasticity of substitution of input factors such as capital stock (K), labor (L), and energy (E) in China’s economic system, where energy can be classified as fossil (F) and non-fossil (NF) energy sources. In view of the theory of development economics, long-term economic growth depends entirely on technological progress. In this regard, considering technological progress, there exists both the neutral categories of technological progress that can keep factor inputs constant and the biased types of technology. Both types of technological progress are included in the model, with the time trend variable t (t = 1, 2, ..., 31) introduced to investigate the impact of technological progress on economic growth. Thus, a transcendental logarithmic production function is obtained for the three types of factors, including capital, labor, and energy, under the circumstances of technological progress. The specific form of the function is listed as follows:
In this formula,
The higher output elasticity of the factors indicates that the factors have stronger output capacity and production efficiency, and the process of calculating the output elasticity of the factors is shown in Eq. 2 as follows.
The rate of technological progress, on the other hand, measures the level of aggregate factor productivity of economic development, which is calculated as follows.
The formula
The elasticity of substitution of the factor reflects the response measurement of resource allocation structure concerning the corresponding changes of factor inputs. When the elasticity of substitution is 0, the factors are manifested in a complementary relationship, i.e., the increase of one factor can promote the increase of the marginal output of the corresponding factor; when the elasticity of substitution is greater than 1, the factors are manifested in a substitution relationship, i.e., the increase of one factor is accompanied by the decrease of the marginal output of the other factor. When the elasticity of substitution is between 0 and 1, there is an upper limit of substitution between these two factors, and the elasticity of substitution between factors is calculated as depicted in Eq. 4.
The differentiation in technological progress between the input factors can be obtained from Eq. 5.
Considering the availability of data, the data required in this paper mainly include the aggregate domestic output and the inputs of capital, labor, and the two categories of energy resources, but without considering the intermediate inputs, whereas the research time span is between the year 1990–2020. The aggregate output is indicated in terms of GDP, and GDP at constant prices is obtained by deflating the GNP index to obtain GDP at constant prices for the base period of 1990. While the capital stock is calculated by exploiting capital stock data at constant prices in 1990, the perpetual inventory method is employed to calculate the national capital stock between the years 1990–2020. The specific calculation method is:
Where
4 Model measurement results and analysis
Since there exists a large number of cross terms in the model composition, which are prone to create serious multicollinearity, thus the ordinary least squares regression is prone to create inaccurate parameter estimations or large variances of estimated values. The study employs ridge regression to estimate the parameters of explanatory variables to eliminate this effect. Ridge regression is a biased regression method dedicated to the analysis of covariance data. It is essentially a modified least squares estimation method that obtains more realistic and reliable regression coefficients by abandoning the assumption of unbiased of the least squares method at the expense of losing some information and reducing accuracy (Garcia et al., 2015). The calculated ridge trace map is shown in Figure 1.
Based on the results of the ridge regression, K = 0.03 can be determined and the regression coefficients of the transcendental logarithmic production function can be obtained accordingly, as the results are shown in Table 1. The model test results show that all variables except lnF, tlnF, and (lnF)2 have passed the significance test at the significance level of 0.05, and the model coefficient of determination R2 is 0.998, which means that all these variables better illustrate the level of economic development, and the coefficients of the variables are more consistent with the actual situation. Hence, the results of the model estimation are relatively reasonable. Although fossil energy has long driven China’s economic growth, the results of this paper suggest that the fossil energy-related factor contribution is not significant to the economic development. This may be due to the process of decoupling economic growth and energy consumption, especially fossil energy consumption, continues to be optimized (Wei et al., 2020), while the growth rate of fossil energy has been significantly curbed.
The parameter results (Table 1) show that the contribution of input factors to China’s economic growth during the study period is measured respectively by order of labor, capital, and non-fossil energy, while the contribution of fossil energy and technological progress is significantly lower, indicating that China’s extensive economic development model of relying on factor inputs to propel economic growth since the 1990s has not yet been effectively reversed, which mainly relies on labor and capital inputs. The coefficients of the squared terms of all parameters are positive, which indicates that the factor inputs are at the stage of incremental returns to scale against aggregate output. The positive value of the time trend term indicates the existence and incessant improvement of neutral technological progress in the economic system, while the positive biased technological term also indicates the existence of interplay between technological progress and capital, labor, and energy, which jointly contribute to the promotion of output. Technological progress reduces the level of factor inputs per unit of output, which means that the level of factor efficiency is improved, and this can effectively promote China’s transformation from sloppy growth, which is promoted by the scale of factor inputs, to more intensive growth, which is based on productivity improvement. However, the result also manifests that the progress of biased technology remains at the same level concerning its impacts on various factors.
Further, this paper calculates the output elasticity of each factor input from the year 1990–2020 based on the parameter estimation results of the model (as shown in Figure 2). With the continuous adjustment and optimization of China’s economic and social structure, the allocation structure, as well as the supply and demand structure of each factor, are continuously adjusted simultaneously. In this regard, the output levels of various factor inputs have shown significant differentiations. The overall trend of China’s transition from labor- and capital-intensive development to capital-intensive development is taking shape during this observation period, but the output elasticity of energy is not high throughout this observation period, yet although it has improved, the growth rate is not rapid enough. Meanwhile, China has long been in a state of massively incremental returns to scale of factor inputs, mainly owing to the fact that the output elasticity of capital factor inputs in China remains high, the output elasticity of labor keeps stable for a long time. However, the inefficiency in the output of the energy factor has affected the growth effect. In the early 1990s, the capital output elasticity and labor output elasticity in China were relatively close, both registering around 2. Since then, thanks to the optimization of the capital input structure, the efficiency of capital utilization has been drastically improved, significantly widening the gap with the labor output elasticity, with incessant contribution to bolstering the development of the economic system. Meanwhile, with improvement in educational conditions and acceleration in human capital accumulation, China’s labor force output elasticity has also been gaining momentum slightly.
The demand for energy in China’s economic growth has been continuously escalating and is predicted to decline slightly after reaching the peak around the year 2030, but the energy factor input has been remaining inefficient for a long time. As the situation that fossil energy is still the dominant energy source in China cannot be effectively reversed in the short term, the expensive initial investment of non-fossil energy and its relatively low output elasticity can hardly accomplish a qualitative leap in this aspect. In contrast, the output elasticity of non-fossil energy is relatively higher than that of fossil energy, but the contribution of fossil and non-fossil energy to economic development remains low for a long time. Thus, there is still an ample space and pressure for energy conservation and consumption reduction.
Based on Eq. 3, this study aims to calculate the contribution of aggregate factor productivity to socio-economic development from 1990 to 2020 for various types of technological progress in China, and the results are shown in Figure 3.
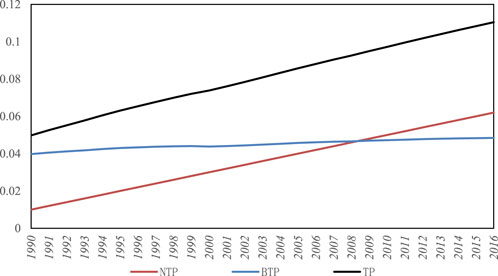
FIGURE 3. Changes in Aggregate Factor Productivity of Technological Progress in China’s Economic Development from 1990 to 2020. NTP represents neutral technological progress, BTP represents biased technologies progress, and TP represents total technological progress.
The results in Figure 3 indicate the increasing role of technological progress in China’s economic system, growing from 1% in 1990 to 7% in 2020, with an average annual growth rate of 0.2%. Although neutral technological progress was lower than the sum of the contributions of factor-biased technological progress in the aggregate factor productivity contribution to China’s economic development before 2008, its progress has been growing steadily and exceeded the contribution from biased technological progress for a long time since then. Thus, although biased technological progress could save factor inputs, the results of factor substitution characteristics might also be subject to relatively large errors if neutral technological progress were neglected.
Thanks to the incessant escalation of China’s innovation, new products and industries have been boosting the rapid growth by contributing to neutral technological progress. Since China’s technological progress is largely based on “latecomer advantage”, the escalation in neutral technological progress also confirms this. Yet, with the narrowing gap in technology against those developed countries, the room for employing neutral technological progress to promote economic development is supposed to diminish. However, for the moment, the inefficiency in the growth trend prevails over the effects displayed by biased technology concerning its penetration into factors.
Figure 4 shows the elasticity of substitution among the input factors of China’s economic development from 1990 to 2020. From the factor substitution level, the substitution elasticity among the factors has a relatively obvious tendency to converge towards an elasticity of 1. At present, only the elasticity of substitution for capital-fossil energy and labor-fossil energy is slightly less than 1, while the elasticity of substitution for capital and non-fossil energy, labor-nonfossil energy, and capital-labor are all able to achieve full substitution. This result indicates that capital and labor are perfect substitutes for non-fossil energy sources, but there are substitution limitations for non-fossil energy sources. The degree of substitution between non-fossil and fossil energy sources is greater than 1, indicating that non-fossil and fossil energy sources can also achieve perfect substitution in China’s energy consumption mix, and that adoption of policy guidance is needed to accelerate the clean energy transition strategy, thus accelerating the trend of reducing the share of fossil energy sources. In the future, the scale effect of non-fossil energy development, such as solar energy, nuclear energy, wind energy, etc. should be fully demonstrated, and the increase of non-fossil energy input will also have a catalytic effect for other factors, and the vigorous development of non-fossil energy is of great importance for saving factor inputs.
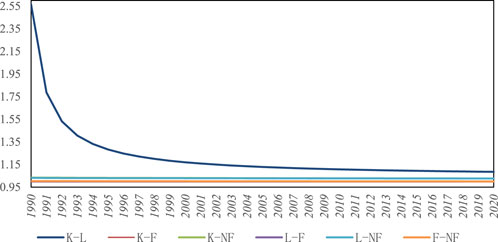
FIGURE 4. Elasticity of substitution among input factors for China’s economic development (1990–2020).
The trend of the differentiation in technological progress (Figure 5) shows a relatively obvious distinction in technological progress among factors, and the distribution of such distinction trend tends to be very uneven. Meanwhile, once a factor has achieved technological advantage in its input, such an advantage is very likely to persist and expand in the long run. The transition from fossil energy to capital kept declining for a long time until 2000, and then it gradually expanded its advantage. But compared to other factors, the technological advantage of fossil energy over capital is extremely insignificant. All factors are at a technological advantage when compared to the capital, the greatest distinction of technological progress lies in its transition process from labor to capital, or from labor to energy, which is also very close to that level. This indicates that with the improvement of China’s education competence and institutional mechanism, the quality of its labor force keeps improving, and the acceleration of human capital accumulation also facilitates the superior status of input in the labor force. But it is difficult for capital factor input to maintain the formation of capital-intensive development with the expansion of technological differentiation.
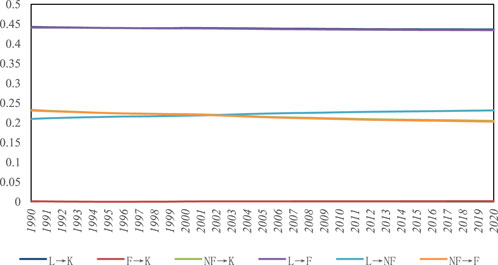
FIGURE 5. Variation in technological progress among factors of economic development in China (1990–2020).
To ease the tension of energy utilization, the Chinese government has been promoting technological investment in the energy sector to improve its energy production capacity and utilization efficiency, enabling non-fossil energy to gain a greater technological advantage over capital. Since non-fossil energy lacks the cost advantage over fossil energy, it will not be easy to get consumers’ favor by developing non-fossil energy without technological progress. The deterioration of the environment and the pressing shortage of fossil energy urgently require technological improvements in non-fossil energy sources. However, for the moment, it seems that non-fossil energy sources do not have much technological advantage over fossil energy sources, which makes it difficult for the energy transition to accomplish the desired goals effectively.
Meanwhile, to verify the reliability of the research results, robustness tests have been conducted in this paper: 1) replacement of the dependent variable. GDP per capita is also a common indicator used by scholars to characterize the level of economic development, so the dependent variable is replaced with GDP per capita in this paper. 2) Replacement of the independent variable. In this paper, the employed population is mainly adjusted to the labor force population, which changes the portrayal dimension of human capital. Since the labor force population is the aggregate sum of labor resources in a country, which comprises both the employed and the unemployed population, such an indicator can be used to portray the degree of labor force participation in social labor. After calculating the modified model by ridge regression, the results are shown in 1) and 2) of Table 2, respectively.
The results in Table 2 show that the model has an excellent imitative effect by adjusting the independent and dependent variables of interest. In addition, all indicators show positive regression coefficients. The distinction lies in the regression coefficients of individual variables and alternation of significance level without affecting the empirical results. Therefore, the results of this paper are credible.
5 Conclusion and recommendations of the research
The study employs a translog production function, using an input-output model with four factors: Capital, labor, energy, and technology. Meanwhile, energy is divided into fossil and non-fossil sources, dividing technological progress into neutral and biased technological progress. We aim to study the output and substitution of capital, labor, and energy in China from 1990 to 2020 under the influence of different technological progress, as well as survey the dynamic characteristics of substitution and inter-energy substitution in China. The dynamic characteristics of factor substitution and inter-energy substitution in China and their effects on its economic development are also investigated. The results show that, in general, non-energy factors are significantly substitutable for energy factors. Moreover, the elasticity of substitution between factors tends to converge to 1, indicating that capital and labor are perfect substitutes for fossil energy as technological progress leads to a shrink in factor differentiation, showing an upper limit to the substitution of capital for non-fossil energy.
The total factor productivity of technological progress in China is relatively low compared to economic growth. Neutral and overall technological progress evolved in the same trend, with biased technological progress making a higher contribution to economic development in the early period. During the sample period, the differentiation in technological progress between factors manifests a labor-saving type, and this trend becomes more distinctive with economic and social development, while the technological progress in energy sources manifests a non-fossil energy-saving type. Evidently, the higher contribution made by the non-fossil energy sources in contrast to fossil energy sources, it will be more likely to effectively promote the replacement of fossil energy sources by non-fossil energy sources, which spurs accomplishing China’s national strategy of the clean energy transition.
Therefore, to achieve a sustainable energy substitution adjustment and smooth economic development from the perspective of factor distribution, it is necessary to increase the input of capital and labor on the one hand, while maintaining a high level of neutral technological progress by shifting our concerns back to the development of biased technological progress, focusing on the insufficiencies to expand effective investment and improve the efficiency of capital utilization. With such efforts to enhance constraints on energy use and reduce energy demand, we aim to propel the adjustments on the composition of energy utilization by optimization of factor input structure so as to boost the realization of the energy consumption peak. This will enable a rational transformation of China’s economic development model, while contributing to reducing energy consumption and improving the quality of economic development, effectively supporting China’s long-term and steady economic growth. On the other hand, however, it is also necessary to encourage the development of a green and low-carbon modern energy utilization system, accelerate the development of non-fossil energy, improve the innovative technology and innovation capacity of non-fossil energy, and simultaneously formulate relevant policies to optimize the efforts and compositions of energy subsidies so as to improve the environment for the utilization of non-fossil energy. All these will help to promote the technological progress of non-fossil energy and accelerate the accumulation of advantages of non-fossil energy. Concerning fossil energy, it is also necessary to improve the utilization efficiency and energy-conservation technology to accomplish the goal of clean and efficient adjustment in energy consumption composition, ensure the sustainable development of energy consumption by alleviating the pressure of fossil energy depletion and negative externalities incurred by high pollution costs to economic development. All these approaches spur to effectively promote the shift of energy alternative paths towards constructing a modern energy system for energy conservation and high-efficiency performance in accordance with the requirements of the energy revolution.
Data availability statement
The raw data supporting the conclusion of this article will be made available by the authors, without undue reservation.
Author contributions
“Conceptualization and methodology, LL; software, YX; validation, WX; formal analysis and investigation, WD; writing—original draft preparation, LL; writing—review and editing, LL; supervision, WX; funding acquisition, WX All authors have read and agreed to the published version of the manuscript.”
Conflict of interest
The authors declare that the research was conducted in the absence of any commercial or financial relationships that could be construed as a potential conflict of interest.
Publisher’s note
All claims expressed in this article are solely those of the authors and do not necessarily represent those of their affiliated organizations, or those of the publisher, the editors and the reviewers. Any product that may be evaluated in this article, or claim that may be made by its manufacturer, is not guaranteed or endorsed by the publisher.
References
Berndt, E. R., and Wood, D. O. (1975). Technology, prices, and the derived demand for energy. Rev. Econ. Statistics 57, 259–268. doi:10.2307/1923910
Cai, H. X. (2014). Energy constraints, technological progress, and the sustainability of China’s economic growth. Resour. Sci. 36 (5), 0946–0953.
Cantarero, M. M. V. (2020). Of renewable energy, energy democracy, and sustainable development: A roadmap to accelerate the energy transition in developing countries. Energy Res. Soc. Sci. 70, 101716. doi:10.1016/j.erss.2020.101716
Chen, K. H., Yang, H. Y., Lee, J. M., and Chi, C. F. (2016). The impact of energy prices on energy consumption and energy efficiency: Evidence from taiwan. Energy Effic. 9 (6), 1329–1349. doi:10.1007/s12053-016-9426-y
Dong, Z. Q., and Zhao, J. (2017). Biased technical progress, ownership structure and energy efficiency. J. Southeast Univ. Philosophy Soc. Sci. 19 (5), 102–112.
Garcia, C. B., Garcia, J., Martin, M. M. L., and Salmeron, R. (2015). Collinearity: Revisiting the variance inflation factor in ridge regression. J. Appl. Statistics 42 (3), 648–661. doi:10.1080/02664763.2014.980789
Han, Z. H., Liu, M. H., and Wu, Z. Q. (2013). Research on measuring energy saving potential based on factor substitution elasticity. China Popul. Resour. Environ. 23 (9), 42–47. doi:10.3969/j.issn.1002-2104.2013.09.007
Han, Z. H., Qi, C., and Liu, M. H. (2018). The route for realizing the 13th Five-Year Plan’s objectives of energy saving and emission reduction. J. Arid Land Resour. Environ. 32 (3), 23–27. doi:10.13448/j.cnki.jalre.2018.036
Hao, F. (2015). Formula correction and estimation methods comparison on elasticity within translog functions. J. Quantitative Technol. Econ. 4, 88–105. doi:10.13653/j.cnki.jqte.2015.04.006
He, Y. D., Liu, Z. C., and Sun, W. (2017). Effects of substituting energy with capital on China’s energy conservation and emission reduction. Industrial Econ. Res. 1, 114–126. doi:10.13269/j.cnki.ier.2017.01.010
Huang, C. C., and Hu, R. D. (2013). Technological progress, energy efficiency and rebound effect. Macroeconomics 4, 44–52. doi:10.16304/j.cnki.11-3952/f.2013.04.004
Jiang, Z. M., and Wang, W. (2017). How will different depreciation rate affect the estimation of China’s capital stock. Statistics Inf. Forum 32 (19), 14. doi:10.3969/j.issn.1007-3116.2017.01.002
Li, J., and Lin, B. (2016). Inter-factor/inter-fuel substitution, carbon intensity, and energy-related CO2 reduction: Empirical evidence from China. Energy Econ. 56, 483–494. doi:10.1016/j.eneco.2016.04.001
Lin, B., Atsagli, P., and Dogah, K. E. (2016). Ghanaian energy economy: Inter-production factors and energy substitution. Renew. Sustain. Energy Rev. 57, 1260–1269. doi:10.1016/j.rser.2015.12.160
Lin, B., and Liu, K. (2017). Energy substitution effect on China’s heavy industry: Perspectives of a translog production function and Ridge Regression. Sustainability 9 (11), 1892. doi:10.3390/su9111892
Lin, B., and Tian, P. (2017). Energy conservation in China’s light industry sector: Evidence from inter-factor and inter-fuel substitution. J. Clean. Prod. 152, 125–133. doi:10.1016/j.jclepro.2017.03.099
Liu, P. Y. (2014). “The core of energy conservation and emission reduction lies in human capital -- Analysis of energy substitution based on China’s industrial secto,” in Proceedings of the 2014 Academic Annual Meeting of Chinese Academy of Environmental Sciences (Chapter III), August 22–23, 2014, Sichuan,China.
Ma, C., and Stern, D. I. (2016). Long-run estimates of interfuel and interfactor elasticities. Resour. Energy Econ. 46, 114–130. doi:10.1016/j.reseneeco.2016.09.003
Omisakin, D. (2008). Energy consumption and economic growth in Nigeria: A bounds testing cointegration approach. J. Econ. Theory 2 (4), 118–123.
Qi, S. Z., and Li, Y. (2017). Does renewable energy consumption affect economic growth? : Empirical evidence from European union. World Econ. Stud. 4, 106–119. doi:10.13516/j.cnki.wes.2017.04.010
Qi, S. Z., and Wang, B. B. (2013). Technical progress, factor substitution and China’s energy decomposition under open economy condition. World Econ. Stud. 4, 3–9+87. doi:10.13516/j.cnki.wes.2013.09.001
Shi, H. L., Chen, K., and Yan, B. (2010). Substitution elasticity among energy, capital and labor inputs in Chinese steel sector. Based on a translog production function. J. Industrial Technol. Econ. 11, 110–116. doi:10.3969/j.issn.1002-980X.2010.09.012
Solarin, S. A., and Bello, M. O. (2021). Output and substitution elasticity estimates between renewable and non-renewable energy: Implications for economic growth and sustainability in India. Environ. Sci. Pollut. Res. 28 (46), 65313–65332. doi:10.1007/s11356-021-15113-9
Steinbuks, J., and Narayanan, B. G. (2015). Fossil fuel producing economies have greater potential for industrial interfuel substitution. Energy Econ. 47, 168–177. doi:10.1016/j.eneco.2014.11.001
Sun, P., and Nie, P. Y. (2014). The substitution of renewable energy for depletable energy under dynamic perspective. Syst. Eng. 3, 92–98.
Vo, D. H., and Vo, A. T. (2021). Renewable energy and population growth for sustainable development in the Southeast Asian countries. Energy sustain. Soc. 11 (1), 30–15. doi:10.1186/s13705-021-00304-6
Wang, B., and Qi, S. Z. (2014). Biased technological progress, factor substitution and China’s industrial energy intensity. Econ. Res. J. 2, 115–127.
Wang, C. X., and Xie, Z. Z. (2013). Discussing the logical errors with regard to substitution elasticity of essential factor about translog production function. Statistics&Information Forum 28 (10), 13–16. doi:10.3969/j.issn.1007-3116.2013.10.003
Wang, X., and Fan, Z. Q. (2017). Dynamic relationship among technical progress, energy structure and energy efficiency: Empirical analysis based on VAR model. Arid. Land Geogr. 3, 700–704. doi:10.13826/j.cnki.cn65-1103/x.2017.03.026
Wang, Z., Zhao, X. G., and Zhou, Y. (2021). Biased technological progress and total factor productivity growth: From the perspective of China’s renewable energy industry. Renew. Sustain. Energy Rev. 146, 111136. doi:10.1016/j.rser.2021.111136
Wei, W. D., Cai, W. Q., Guo, Y., Bai, C. Q., and Yang, L. Z. (2020). Decoupling relationship between energy consumption and economic growth in China’s provinces from the perspective of resource security. Resour. Policy 68, 101693. doi:10.1016/j.resourpol.2020.101693
Yan, X. X., Zhang, J. S., and Zou, S. H. (2015). Energy substitution elasticity prediction under the new era - with GA-SA Method. Chin. J. Manag. Sci. 23 (S1), 755–762.
Yang, F. X., Yang, M., and Nie, H. L. (2011). An investigation of the elasticity of energy-nonenergy factor substitution: An empirical analysis based on the translog production function. Resour. Sci. 33 (3), 460–467.
Zhang, Y. X., Cheng, J. H., and Tang, S. Y. (2017). Directed technology change, property right structure and the Chinese regional energy efficiency. J. Quantitative Technol. Econ. 8, 72–88. doi:10.13653/j.cnki.jqte.2017.08.005
Zhao, X., Ma, X., Chen, B., Shang, Y., and Song, M. (2022). Challenges toward carbon neutrality in China: Strategies and countermeasures. Resour. Conservation Recycl. 176, 105959. doi:10.1016/j.resconrec.2021.105959
Zheng, M. (2016). Growth effect research of factor substitution based on biased technology progress. J. Quantitative Technol. Econ. 11, 94–110. doi:10.13653/j.cnki.jqte.2016.11.006
Zheng, Z., and Liu, D. (2004). “Translog production function and its application in China,” in Proceedings of the 6th China Youth Operations Research and Management Scholars Conference, Juky 16–20, 2004, Hebei,China, 250–253.
Keywords: factor substitution, energy substitution, production function, energy conservation, consumption reduction
Citation: Li L, Xiong W, Duan W and Xiong Y (2023) Evaluation on substitution of energy transition—An empirical analysis based on factor elasticity. Front. Energy Res. 10:1068936. doi: 10.3389/fenrg.2022.1068936
Received: 13 October 2022; Accepted: 11 November 2022;
Published: 18 January 2023.
Edited by:
Shiwei Yu, China University of Geosciences Wuhan, ChinaReviewed by:
Xing Hu, China University of Geosciences Wuhan, ChinaFeng Dong, China University of Mining and Technology, China
Copyright © 2023 Li, Xiong, Duan and Xiong. This is an open-access article distributed under the terms of the Creative Commons Attribution License (CC BY). The use, distribution or reproduction in other forums is permitted, provided the original author(s) and the copyright owner(s) are credited and that the original publication in this journal is cited, in accordance with accepted academic practice. No use, distribution or reproduction is permitted which does not comply with these terms.
*Correspondence: Weiwei Xiong, xww08012115@hotmail.com