- 1State Key Laboratory of Petroleum Resources and Prospecting, China University of Petroleum, Beijing, China
- 2MOE Key Laboratory of Petroleum Engineering, China University of Petroleum, Beijing, China
- 3National Engineering Research Center of Oil, Gas and Completion Technology, Beijing, China
Unconventional gas includes tight sandstone gas, shale gas, coalbed methane, and natural gas hydrate. With huge reserves, unconventional gas has become the most important natural gas resource successor after the end of the “Easy Oil era.” The drilling fluid is an indispensable wellbore working fluid for unconventional gas drilling with multiple functions. The polymer drilling fluid (PDF) is the most common, longest developed, and most diverse drilling fluid type. With advantages of easily controlled rheology, convenient on-site performance maintenance, and specifically low cost and weak environment pollution, the PDF is gradually replacing the oil-based drilling fluid as the first choice for unconventional gas drilling. The invention of the non-disperse low-solid-content PDF in the 1960s shows that PDF technology has entered the stage of scientific development, and until now, its development has generally experienced five stages: beginning, developing, improving, re-developing, and re-improving. Dozens of polymer additives and PDF systems have been invented and applied, which have solved severe drilling problems, greatly improved drilling efficiency, and promoted exploration and development in difficult oil and gas resources. This paper first reviews the research progress of PDF technology according to the timeline by introducing the composition, feature, advantages, and disadvantages of some representative polymer additives and PDF systems, emphatically the function and mechanism of stabilizing wellbores, lubricating drilling tools, and protecting reservoirs of the biomimetic wellbore-strengthening PDF and amphiphobic high-efficiency PDF in unconventional gas drilling. Then, combining future global demands, especially China’s strategic needs of oil and gas exploration and development, the development tendency of PDF technology is critically illustrated by introducing several potential research directions including intelligent PDF, ecological PDF, and PDF for natural gas hydrate and deep layer gas resources.
1 Introduction
Drilling is an engineering technology that constructs a channel from the ground to the underground. It is not only the most essential method to obtain underground resources including crude oil, natural gas, water, and terrestrial heat but also a must to acquire geological information to promote geoscience research (Ladd et al., 1967; Hammond, 1975; Eichelberger, 1997; CelsoMorooka et al., 2001; Hanson, 2003; Hou et al., 2018). The drilling fluid is the “blood” of drilling, which is a complex fluid composed of various additives with multiple functions including suspending and carrying rock cuttings, preventing wellbore collapse, balancing formation pressure, protecting oil and gas reservoirs, cooling and lubricating drilling equipment, and transmitting hydraulic horsepower (Browning and Chesser, 1972; Growcock and Harvey, 2005). The drilling fluid affects whether drilling can advance normally and decides directly whether the well could be successfully constructed. Since clean water was used as the drilling fluid in the 1880s, drilling fluid technology has experienced more than a century’s development. Hundreds of drilling fluid additives and dozens of drilling fluid systems have been invented, which plays an irreplaceable role in exploring and developing oil and gas resources.
According to the type of the dispersion medium, the drilling fluid can be classified into the water-based drilling fluid, oil-based drilling fluid, and gas drilling fluid. The water-based drilling fluid is the most widely used type and always occupies the dominating place. The polymer drilling fluid (PDF) is an essential type of water-based drilling fluid. In a broad sense, the water-based drilling fluid using a polymer as the major additive is regarded as the PDF. Owning advantages of low solid content, fast rate of penetration (ROP), easy-to-control rheological property, and low cost, the PDF has become a drilling fluid kind with the fastest and highest development and the widest application (Browning and Chesser, 1972; Growcock and Harvey, 2005). Since the non-dispersed low-solid PDF was invented in the 1960s (Lummus and Anderson, 1964; Lawhon and Simpson, 1967; Field, 1968; Lanman and Willingham, 1970; Duane, 1972; Mondshine, 1973; Foshee et al., 1977; Miller, 1980; Blattel and Rupert, 1982; Steiger, 1982; Hale and Mody, 1993; Parizad et al., 2018), the PDF entered the scientific development stage. Various functional polymer additives and PDF systems have been invented, which solved many drilling problems, strongly supported the development of oil and gas resources, and greatly promoted the development of drilling fluid technology.
In the past few years, with the ending of the “Easy Oil Era” and under the background of complex changes of international climate and drastic fluctuation of oil price, global oil and gas exploration and development have advanced rapidly toward difficult resources, especially unconventional, low permeable, deepwater, and deep layer. In particular, unconventional gas including shale gas, tight sandstone gas, and coalbed methane is highly focused. It is not only because unconventional gas has huge reserves which is estimated to reach 4,000 trillion cubic meters, about 4.5 times than conventional gas reserves, but also because of the successful commercialization of shale gas which proves the feasibility and economic value of developing unconventional gas. Drilling unconventional gas encounters complex wellbore structures, difficult formation conditions, and special well completion methods, bringing new challenges for PDF technology. Higher and more special performances are needed urgently to meet drilling requirements, which greatly promotes the development of PDF technology.
According to the timeline, the development progress could be approximately divided into five stages, namely, “beginning,” “development,” “improvement,” “re-development,” and “re-improvement.” In the following section, the research progress of PDF technology will be introduced based on representative achievements at each stage, emphatically PDF technology for unconventional gas drilling. Furthermore, combining global demands, especially China’s strategic needs of oil and gas exploration and development, the development tendency of PDF technology in the future is critically illustrated.
2 Research progress of PDF technology
2.1 Beginning stage
In the 1960s, scientific and technical personnel began to realize that components of the drilling fluid greatly affect the drilling efficiency. The content of useless solids, especially rock cuttings and non-mud-making soil, is the major influencing factor which decreases the ROP and increases the drilling cost. Even though the low-solid drilling fluid is used at the beginning, with drilling advances, useless solids are mixed-in continuously and the content increases inevitably. Because the particle size of most useless solids is at the submicro level, they are difficult to be eliminated by solid-control equipment (only available for size of tens of microns). As a result, the low-solid drilling fluid still becomes high-solid drilling fluid eventually.
With the development of a polymer additive, it is found that partially hydrolyzed polyacrylamide (PHPA) and the copolymers of vinyl acetate and maleic acid (VAMA) have the ability of optional flocculation (Figure 1) (Duane, 1972; Foshee et al., 1977; Hale and Mody, 1993), i.e., they could flocculate useless solids without flocculating bentonite, barite, and other useful solids. Therefore, the use of these polymers can reduce the content of useless solids effectively by aggregating fine solid particles into thick particles which could be removed by solid-control equipment. Centering on PHPA and VAMA, the non-dispersed low-solid PDF was established (Lummus and Anderson, 1964; Lawhon and Simpson, 1967; Field, 1968; Lanman and Willingham, 1970; Mondshine, 1973; Miller, 1980; Blattel and Rupert, 1982; Steiger, 1982; Parizad et al., 2018). In 1966, the Pan American Petroleum Corporation used non-dispersed low-solid PDF technology first in western Canada, which greatly increased the ROP and reduced the drilling cost by 2.5%. In the 1970s, this technology was used in scale in the Deira Bay Basin in the United States, which increased the ROP by 17%∼28% and reduced the drilling cost by over 20%. In 1973, China’s Shengli Oilfield used this technology, increasing the average ROP by over 20%. So far, the non-dispersed low-solid PDF is still used worldwide for high-speed and low-cost drilling.

FIGURE 1. Molecular structure of partially hydrolyzed polyacrylamide (A) and the copolymer of vinyl acetate and maleic acid (B) (both neutralized by NaOH).
2.2 Development stage
The early-used PHPA and VAMA are simple in function which makes PDF inadequate to meet diverse drilling requirements of more complex strata. Therefore, different new anionic polyacrylamide derivatives such as the 80 A series, PAC series, and SK series were developed subsequently. A polymer additive was then developed into pluralistic products with different metal ions (Na+, K+, and NH4+), different molecular weights (10,000 Da–5,000,000 Da), and different charge densities (10%–60%). The function of the polymer additive was also expanded to a great extent, including flocculation, encapsulation, inhibition, tackifying, gelation, and fluid loss control (Lourenco et al., 2006; Payam et al., 2016; Oseh et al., 2020). Diversified development of the polymer additive had led to a variety of new PDF systems with improved performance which expanded PDF’s availability scope. The typical representative is a polymer/KCl drilling fluid system which is still one of the most widely used PDFs for drilling water-sensitive strata and the basis of high-performance water-based drilling fluid for shale gas drilling (Steiger, 1982; Sharma and Kachari, 2010; Kharitonov et al., 2011).
2.3 Improvement stage
2.3.1 Cationic polymer drilling fluid
The contact between the drilling fluid and wellbore is inevitable. The surface of the wellbore rock soaks in the drilling fluid, while the inside of the wellbore rock could come into contact with the drilling fluid’s filtrate since the filtrate could permeate into the rock inside through pores and cracks. If the wellbore rock contains high content of shale, clay, or other components that are easy to hydrate, the contact with the water-based drilling fluid will decrease the rock’s cohesion and, thus, soften the wellbore, resulting in wellbore instability or even collapse (Yew and Liu, 1992; Bybee, 2004; Zeynali, 2012; Wenjun and Haiyan, 2014; Xiong et al., 2016; Zheng et al., 2016; Hamza et al., 2019). In addition, it is known that cuttings will be mixed-in continuously with the proceeding of drilling. Thus, for drilling in strata where cuttings are made of these easy-to-hydrate components, like a shale gas reservoir, the hydration and dispersion of cuttings will largely increase the drilling fluid’s viscosity and gel strength, which deteriorates the drilling fluid’s rheological property (Bruton and McLaurine, 1993; Walker and Li, 2000; Ahmad et al., 2019). In addition, for reservoirs containing a high content of easy-to-hydrate components, hydration causes swelling and fines migration which will block oil and gas passage, leading to severe reservoir damage (Zhang et al., 2011a; Memon et al., 2017; Ahamed et al., 2019; Ni et al., 2019a; Lei et al., 2019). Obviously, the main cause of these problems is hydration, swelling, and dispersion of water-sensitive solids. If hydration, swelling, and dispersion could be weakened or even avoided, these problems will be alleviated to a great extent. Therefore, inhibition becomes one of the most important properties of the water-based drilling fluid.
The inhibition of the drilling fluid is mainly attributed to an inhibitor (Halliday et al., 1998; Qu et al., 2009; Yang et al., 2013; Xuan et al., 2015; Saikia and Mahto, 2016a; Boul et al., 2016; Saikia and Mahto, 2016b; Ferreira et al., 2016; Mech and Sangwai Jitendra, 2016; Yang et al., 2017a; Huang et al., 2018a; An and Yu, 2018; Jiang et al., 2019a; Li et al., 2019a; Yang et al., 2019a; Li et al., 2019b; Chu et al., 2019; Ma et al., 2019; Aftab et al., 2020). Polymer additives developed in previous stages have certain inhibitive performances. On one hand, they could adsorb onto a solid’s surface and create a polymeric film which alleviates water contact and infiltration. On the other hand, they could weaken solid dispersion by multi-point adsorption. However, inhibition is unsatisfactory because of weak binding between the polymer and solid since these polymers are all anionic, while the solid’s surface is rarely positively charged and mostly negatively charged. Therefore, to improve the PDF’s inhibition, cationic additives were developed and the cationic PDF was established (Retz et al., 1991; Beihoffer et al., 1992; Rosa et al., 2005).
A typical cationic PDF is prepared with quaternary ammonium salt or a low-molecular weight cationic polymer (called small cation since they are either a small molecule or low-molecular weight polymer) as the inhibitor and a high-molecular weight cationic polymer (called large cation) as the encapsulating agent (Retz et al., 1991; Fernandez, 2005; Beg et al., 2018; Jiang et al., 2019a; Li et al., 2019a). The outstanding inhibition of cationic PDF derives from the synergistic effect between the small cation and large cation. The inhibition mechanism could be summarized into three aspects as follows. It is noted that this inhibition mechanism refers in particular to the mechanism of inhibiting montmorillonite which is the main hydration-causing composition of clay, shale, and other easy-to-hydrate components.
1) Surface adsorption of the small cation
The small cation could preferentially adsorb onto montmorillonite by the electrostatic attraction that existed between its cationic group (particularly R3N+) and the negatively charged surface of montmorillonite (particularly AlOH2-) (Shaikh et al., 2018). The other side of the small cation, namely, the hydrophobic group, could gather and form a hydrophobic film on montmorillonite’s surface which prevents water contact.
2) Crystal layer insertion of the small cation
The swelling of montmorillonite is caused by water infiltration into adjacent crystal layers and expansion of layer spacing. The small cation could insert into montmorillonite’s crystal layer, adsorb onto adjacent layers, and occupy the interlayer space. The occupation will squeeze out the water infiltrated between the adjacent layers and further pull close layer spacing, which not only alleviates montmorillonite swelling but also prevents water re-infiltration (Huang et al., 2018a; An and Yu, 2018; Jiang et al., 2019a; Ma et al., 2019).
3) Bridging of the large cation
A high-molecular weight cationic polymer has multiple cationic groups and also a long molecule chain, which could adsorb onto surfaces of different montmorillonite particles. This multi-point adsorption could flocculate and encapsulate dispersed montmorillonite particles since the intermolecular repulsion between particles is screened, which alleviates montmorillonite dispersion (Rosa et al., 2005).
The cationic PDF has been applied successfully in the Shenyang Oilfield, Beibu Gulf, and Erlian Oilfield (Liu, 1992). Its outstanding inhibition effectively enhances wellbore stability and prevents mud-making of cuttings. Nevertheless, the massive use of the cationic PDF brings severe environmental problems since the cationic polymer is biotoxic. The cationic polymer additive is also incompatible with an anionic polymer additive, easy to adsorb on drilling equipment, and its overpowering inhibition prevents bentonite dispersion, and thus, makes it difficult to control fluid loss. Although the cationic PDF is limited nowadays, it provides the thought and method of using cations to improve PDF’s inhibition. The inhibition mechanism of the small cation and large cation is still the base for developing a high-performance inhibitor today. The schematic illustration of the inhibition mechanism of a newly developed biodegradable inhibitor poly-l-arginine (PArg) on bentonite is shown in Figure 2 as an example (Li et al., 2020a).
2.3.2 Amphoteric PDF
The dispersion of bentonite used in PDFs is very influential on fluid loss. Generally, the more dispersed bentonite is, the higher will be the quality of the filter cake bentonite could form under pressure and the lower the fluid loss. Obviously, it comes to the problem of balancing PDF’s inhibition and fluid loss as indispensable inhibition abates bentonite dispersion. Since the anionic polymer additive is effective in controlling fluid loss but lacks inhibition and the cationic polymer additive has strong inhibition but is unfavorable for controlling fluid loss, an amphoteric polymer additive combining advantages of both was invented and the amphoteric PDF was established around the 1990s (Niu et al., 1995; Niu et al., 1998; Warren et al., 2003; Liu et al., 2005; Cao et al., 2017).
The typical amphoteric PDF takes an amphoteric polymer viscosity reducer (like XY-27) and an amphoteric polymer encapsulator (like FA367) as the core. By using other cooperative additives, systems including the non-dispersed low-solid amphoteric PDF, low-density oil-mixed amphoteric PDF, and high-density amphoteric PDF were invented. Field application showed that the amphoteric PDF resolved the contradiction between inhibition and fluid loss to a great extent.
A significant advantage of the amphoteric polymer additive is good electrolyte tolerance, which enables it to function effectively in brine of a monovalent salt or even divalent salt (Warren et al., 2003; Adnan Hamad et al., 2020; Li et al., 2022a; Liu et al., 2022). For example, the amphoteric terpolymer of acrylamide (AM), 2-acrylamido-2-methylpropane sulfonic acid (AMPS), and diallyldimethylammonium chloride (DMDAAC) could be used as a fluid loss agent against calcium contamination. Research indicates that bentonite and the AM-AMPS-DMDAAC terpolymer could together form a steady net structure by intermolecular electrostatic attraction. The net structure prevents contact between bentonite and Ca2+, which alleviates the compression of Ca2+ on bentonite’s diffusion double-layer and, thus, promotes bentonite dispersion (Figure 3) (Liu et al., 2016).
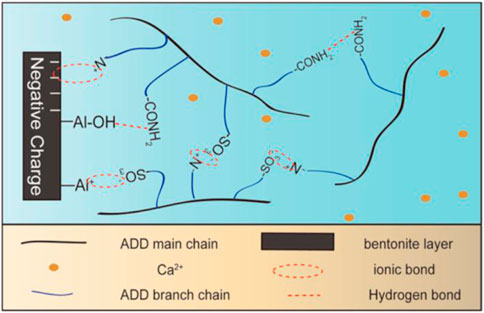
FIGURE 3. Schematic illustration of the net structure formed by bentonite and the AM-AMPS-DMDAAC terpolymer under calcium contamination (ADD represents the terpolymer).
For some time, it was found that a balanced amphoteric polymer (BAP) whose anionic group and cationic group are stoichiometric could function steadily in strong brine and simultaneously under high temperature. Such an advantage of the BAP results from its strong anti-polyelectrolyte behavior, that is, unlike a common polyelectrolyte, BAP’s chain conformation undergoes an absolute extension instead of shrinkage when salinity of the medium is increased. This is because the electrolyte weakens the BAP’s intramolecular electrostatic attraction by a screening effect and then causes the polymer chain to extend osmotically. The extension also happens via temperature modulation as high temperature weakens the ionic bond as well (Figure 4) (Ranka et al., 2015). From this perspective, the BAP is an electrolyte- and temperature-friendly polymer. It is to be noted that sufficient extension of the polymer chain is a prerequisite for the polymer additive to function in the drilling fluid, and it is feasible to develop polymer additives in high-electrolyte content and a high-temperature environment based on the BAP. Until now, the BAP tackifier and BAP fluid loss agent synthesized with the same monomers are developed (Figure 5), and a saturated saltwater (NaCl or KCl) PDF with simple formulation, low polymer additive loading, and no use of any other salt- or temperature-tolerant materials is established. Laboratory research indicates that the saturated saltwater PDF could maintain a stable rheological property and low fluid loss under 150 C for nearly 100 h, showing a promising field application prospect (Guancheng et al., 2019).
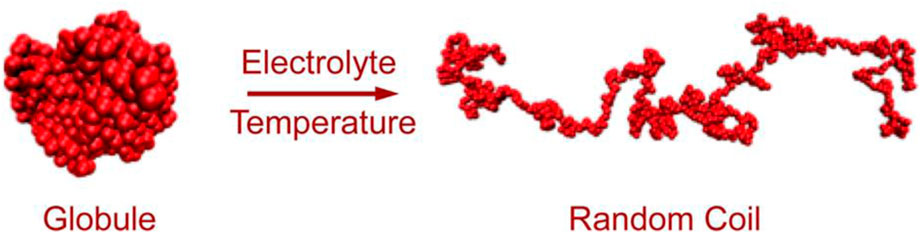
FIGURE 4. Schematic illustration of the chain extension of the BAP via the electrolyte and temperature modulation.
2.4 Re-development stage
Since the 21st century, the worldwide consumption of hydrocarbon fuels soars, averagely reaching 1.8% of annual growth (BP. Statistical Review of World Energy, 2020). Conventional oil and gas resources cannot completely satisfy the huge demand; thus, unconventional resources, especially unconventional natural gas, have gradually become another focus of the energy industry. The most successful representative is shale gas whose commercial development not only alleviates the imbalance between energy supply and demand but also initiates a revolution that has changed the world energy structure (Wakamatsu and Aruga, 2013; Wang et al., 2014).
The horizontal well is the commonest well type for shale gas development. Most shale gas horizontal wells are drilled using the oil-based drilling fluid for safety purposes. For one thing, as discussed previously, using the water-based drilling fluid to drill highly water-sensitive shale always causes wellbore collapse. For another, drilling the curve section and long horizontal section of the horizontal well needs excellent lubricity in case of a sticking accident, and oil just naturally has better lubricity than water. However, two big drawbacks of the oil-based drilling fluid, environment pollution and high cost, have become increasingly influential along with its massive use; thus, the realization of “replacing oil with water” is increasingly excepted. Therefore, scientific and technical personnel focus on developing the high-performance water-based drilling fluid (HPWDF) (Bland et al., 2002; Dye et al., 2005; Morton et al., 2005; Patel et al., 2007).
At the beginning, the HPWDF refers particularly to the “water-based drilling fluid which could simulate performance, mainly inhibition and lubricity, of oil-based drilling fluid and is capable of drilling shale gas horizontal wells.” It uses amino-terminated polyoxypropylene (ATP) as the core additive along with an encapsulating agent, lubricant, rheology modifier, and fluid loss agent. ATP is a low-molecular weight polymer with outstanding inhibitive performance (Figure 6) (DYE et al., 2006). Its inhibition mechanism is similar to that of a small cation; however, its inhibitive effect is much more moderate as the amino group produces a weaker electrolyte effect than the quaternary ammonium group. Satisfactory inhibition could be achieved by large ATP addition while moderate bentonite hydration and colloidal stability of the drilling fluid could be guaranteed at the same time. With the progress of technology, the HPWDF category is quite expanded now that it is neither exclusive for shale gas drilling nor composed of ATP. Its category and composition are multifarious. In the following section, we choose several representative HPWDFs and introduce each composition, performance, feature, and application effect.
1) ULTRADRILL and HydraGlyde
Dedicating to replacing the oil-based drilling fluid by inhibitive water-based drilling fluid early, M-I SWACO reported the UltraDrill system around 2003, which is composed of the inhibitor ULTRAHIB, polymer-encapsulating agent UltraCap, ROP enhancer UltraFree, and other cooperative additives like the polymer fluid loss agent PolyPAC-UL and the biopolymer tackifier MC-VIS. ULTRADRILL not only has excellent inhibition and lubricity but is also non-toxic. The cuttings it produces could be discharged directly into the sea. It has been used in the scale in the Gulf of Mexico, the United States, Brazil, Australia, Libya, and Saudi Arabia (Eia, 2006; Eia and Hernandez, 2006; Hodder et al., 2006; Kong et al., 2006; Burden et al., 2013). China used ULTRADRILL in 2004 for the first time in offshore wells CFD11-2-A5 and CFD11-2-A8 and achieved excellent field application (Huang, 2004). The formulation and the basic performance are shown in Table 1 and Table 2, respectively.
HydraGlyde is another HPWDF system invented by M-I SWACO which is formed mainly with an ROP enhancer HydraSpeed-ROP, inhibitor HydraHib, and a polymer encapsulating agent HydraCap. HydraGlyde has stable rheological property, good inhibition, and particularly outstanding lubricity. Application in Wolfcamp formation in the Midland Basin of West Texas indicates that HydraGlyde increased the ROP by 16% compared with other inhibitive water-based drilling fluids (Table 3) (Schlumberger Service, 2021).
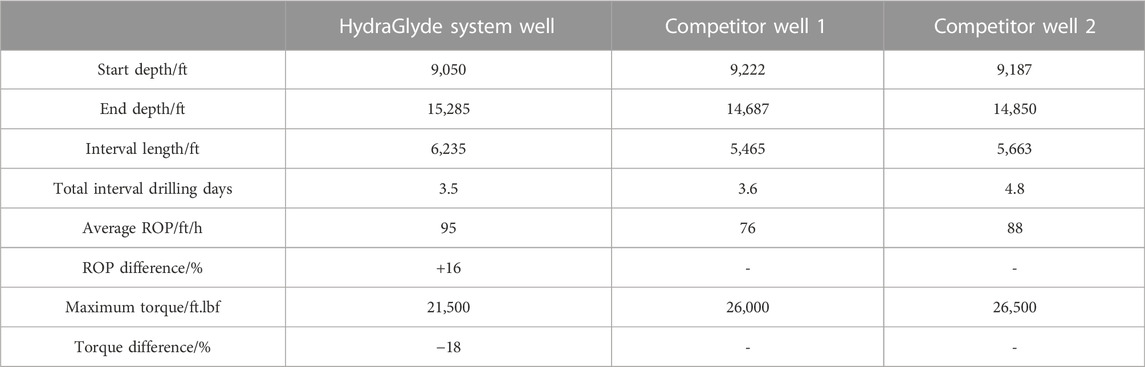
TABLE 3. Comparison of HydraGlyde and other inhibitive water-based drilling fluids applied in offset wells.
2) Pure-Bore
INNOSPEC developed the Pure-Bore system (Clear Solutions Service, 2022). A polymer named Pure-Bore is the core additive with multiple functions, which can control and adjust inhibition, rheological property, fluid loss, and lubricity, as required. Pure-Bore is easy to maintain, non-toxic, biodegradable, and particularly low in additive loading. The whole use of all the additives in a typical Pure-Bore system is lower than 1.7 kg/m3, while a typical polymer/KCl PDF needs higher than 20 kg/m3 in average. Until now, Pure-Bore has been well-applied in North Sea, Norway, and China.
3) PERFORMAX and LATIDRILL
Around 2005, Baker Hughes developed the PERFORMAX system for shale gas reservoirs (Ramirez et al., 2005; Ramirez et al., 2007), which consists of a blocking agent MAX-SHIELD, stabilizer MAX-PLEX, inhibitor MAX-GUARD, polymer-encapsulating agent NEW-DRILL, and lubricant PENETREX. PERFORMAX owns outstanding inhibition by the combined use of polyalcohol and an aluminum-based polymer. Subsequently, Baker Hughes introduced nanotechnology and developed the LATIDRILL system which is the first report of the commercialized application of nanotechnology in the water-based drilling fluid (Bakerhughes Media Center, 2012; Riley et al., 2012; Witthayapanyanon et al., 2013; Yadav et al., 2015). The application result in Cameroon Sea shows that LATIDRILL could improve wellbore stability, prevent bit-balling, decrease friction, and elevate the ROP to a great extent.
4) PERFORMADRIL
Halliburton developed the PERFORMADRIL system with the shale stabilizer PERFORMATROL, bridging agent BARACARB, inhibitor GEM GP, clay PERFORMATROL, and KCl (Halliburton Service, 2012; Halliburton Service, 2013). PERFORMATROL is the key additive which is a low-molecular weight polyamine derivative with the ability to stabilize shale by mitigating its swelling and dispersion tendency. PERFORMADRIL could be used in a wide temperature range and holds the highest environmental rating (GOLD). It has been applied in North Sea and Qatar, saving millions of dollars of drilling cost and more than 10 days of rig time (Table 4).
5) Multi-component synergistic HPWDF
Under the consideration that shale wellbore instability is a complex and difficult problem which cannot be solved properly by using a single additive, researchers developed multi-component synergistic HPWDF systems based on the synergistic effect between polyamine, the aluminum-based polymer, and deformable polymer microsphere (Qiu et al., 2007; Schlumberger Service, 2021). Each of the three polymers has its own function and working mechanism. Polyamine functions as an inhibitor to alleviate shale hydration and wellbore softening. The aluminum-based polymer could yield an aluminum-based precipitate that covers the surface of the wellbore rock, reducing the contact between the drilling fluid and wellbore. The deformable polymer microsphere functions as a sealing agent which could plug into microcracks of shale and stabilize the wellbore rock’s space structure, as well as block water infiltration. The co-action of these polymers could largely improve the stability of the shale wellbore. The multi-component synergistic HPWDF is also very effective in other unstable formations in addition to shale which has been widely used in Saudi Arabia, Egypt, and Africa, as well as in China’s Shengli Oilfield and Xinjiang Oilfield.
2.5 Re-improvement stage
The HPWDF successfully replaces the oil-based drilling fluid for drilling shale gas horizontal wells in many cases and elevates PDF performance to an unprecedented level. Nevertheless, in the past decade, higher performance requirements for the drilling fluid are raised by the following aspects.
First, the ending of the “Easy Oil Era” has made global oil and gas exploration and development advance rapidly toward difficult resources. Most newly discovered unconventional gases are buried in strata with increasingly complex and difficult conditions. Second, complex structure wells headed by long horizontal wells are applied increasingly frequently (Stolte et al., 2012; Wang et al., 2020). Third, the environmental protection standard has reached a very strict level. Lastly, oil price fluctuates drastically and international climate changes unpredictably. PDF technology urgently needs re-improvement to better meet the requirements of high performance, low environmental damage, and low cost, and thus, its development enters the “re-improvement” stage.
A marked characteristic of PDF development in this stage is the huge emergence of new drilling fluid additives whose type includes polymeric (Khalil and January 2012; An et al., 2015a; Madkour et al., 2016; Zoveidavianpoor and Samsuri, 2016; Peng et al., 2017; Zhang et al., 2017; Ahmad et al., 2018; Sepehri et al., 2018; Xianmin et al., 2018; Xie et al., 2018; Chang et al., 2020; Ma et al., 2020; Paul et al., 2020; Rana et al., 2020; Shan et al., 2022), nano-based (Kosynkin et al., 2012; An et al., 2015b; Li et al., 2016a; An et al., 2016; Yang et al., 2017b; Li et al., 2018a; Huang et al., 2018b; Li et al., 2018b; AI-Yasiri and Weng, 2019; Ni et al., 2019b; Boyou et al., 2019; Yang et al., 2019b; Verma et al., 2019; Li et al., 2020b; Li et al., 2020c; Rana et al., 2020; Huang et al., 2022), clay-based (Huang et al., 2018a; Wang et al., 2018; Huang et al., 2019), metal-based, and composite additives (Madkour et al., 2016; Swaminathan Ponmanl et al., 2016; Akkouche et al., 2020; Chang et al., 2020; Paul et al., 2020; Li et al., 2022b; Yang et al., 2022). The most representative achievements are biomimetic and amphiphobic additives which solve the drilling problem and improve the drilling fluid’s performance through innovative perspectives. They have formed biomimetic wellbore-strengthening PDFs and amphiphobic high-efficiency PDFs which are introduced in the following section.
2.5.1 Biomimetic wellbore-strengthening PDF
Bionics studies the structure and property of biological systems to obtain new design ideas and working mechanisms for engineering (Guo et al., 2005; Sachsenmeier, 2016). To further improve the PDF’s wellbore-stabilizing effect, bionics is introduced into the field of drilling fluid technology. A biomimetic wellbore-strengthening agent, biomimetic inhibitor, and biomimetic lubricant are invented, and the wellbore-strengthening PDF is thus established.
1) Performance of biomimetic additives
PDFs invented in the previous stages use an amino-based inhibitor, aluminum-based blocking agent, and polymer microsphere sealing agent to improve wellbore stability in water-sensitive strata. However, these additives are all designed to weaken the negative influence of water on the wellbore rock but never to stabilize the wellbore rock’s space structure. Research indicates that mussel could cement rocks by secreting a highly adhesive protein which can bind strongly to virtually all kinds of inorganic and organic substrates in an aqueous environment (Yang et al., 2013). Inspired by this mechanism, the functioning composition in the protein is found and further introduced as the basis to invent a biomimetic wellbore-strengthening agent which is a polymer derivative of amino acid 3,4-dihydroxy-L-phenylalanine. The biomimetic wellbore-strengthening agent could solidify into an adhesive which could glue the wellbore rock and elevate rock strength by improving the rock’s cohesion (Figure 7) (XuanyangJiang et al., 2013; Guancheng et al., 2015; Jiang et al., 2015).
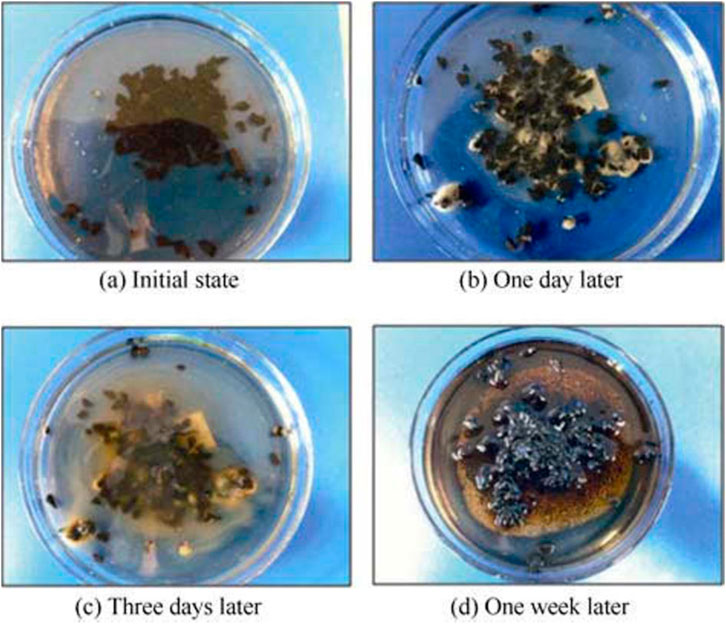
FIGURE 7. Morphology change of shale cuttings immersed in a solution of a biomimetic wellbore-strengthening agent with time. (A) Initial state, (B) one day later, (C) three days later, and (D) one week later.
The biomimetic inhibitor is a polyamino acid derivative also extracted from the adhesive mussel protein (Xuan et al., 2015). Like the small cation, it could embed into clay’s crystal layer, pull close layer spacing, and alleviate clay swelling. Compared with the commonly used polyether amine D230, KCl, and 2,3-epoxypropyltrimethylammonium chloride (EPTAC), the biomimetic inhibitor has the strongest inhibition on montmorillonite which decreases its interlayer spacing from 2.55 nm to only 1.39 nm after soaking treatment (Table 5).
Adding a lubricant to the drilling fluid is an effective way to reduce downhole friction between the drilling fluid and drilling tool and between the drilling tool and rock. The lubricant is also able to prevent bit-balling and elevate the ROP which has become an indispensable additive in the HPWDF. In nature, earthworms adhere to the soil surface by secreting mucus from their body wall, which could form a smooth film between the soil and their body and increase the content of extreme pressure elements such as S and P, and thereby effectively reduce creeping resistance underground. By mimicking this biological behavior, extracting and utilizing the functional composition of mucus, a biomimetic lubricant is invented which is made of a small-molecule cationic phospholipid derivative (Jiang et al., 2018a). It could form steady lubricating films on the surface of the drilling tool and the surface of rock through electrostatic attraction. Evaluation shows that a base with the addition of the biomimetic lubricant has the lowest reduction rate of the friction coefficient, indicating the best lubricity performance compared with other high-performance lubricants (Table 6).
Biomimetic additives have good environmental performance as their compositions are all nature-based. Evaluation by a luminescent bacterial experiment indicates that EC50 of the biomimetic wellbore-strengthening agent, biomimetic inhibitor, and biomimetic lubricant is 1.94 × 105 mg/L, 3.50 × 105 mg/L, and 0.81 × 105 mg/L, respectively, indicating that they are non-toxic.
2) Performance of the biomimetic wellbore-strengthening PDF
The biomimetic wellbore-strengthening PDF consists of a biomimetic wellbore-strengthening agent, biomimetic inhibitor, biomimetic lubricant, cellulose fluid loss agent, and bentonite. A laboratory evaluation indicates that the wellbore-strengthening PDF has rheological property, inhibition, and lubricity similar to a typical oil-based drilling fluid (Table 7), and it is bio-degradable (Table 8).

TABLE 7. Performance comparison between a biomimetic wellbore-strengthening PDF and typical oil-based drilling fluid after ahot-rolling (density is 2.0 g/cm3).
The biomimetic wellbore-strengthening PDF has been used in China’s oilfields such as Changqing, Sichuan, Jilin, Jidong, and Karamay and in countries including Russia, Myanmar, Chad, Brazil, and Iraq. Field application proves that mudstone wellbore stability is effectively improved using this technology. The most successful application is in the Su-53 block of the Sulige tight gas field. Before 2013, when drilling horizontal sections in a mudstone stratum, wellbore collapse would occur without exception. After the introduction of the wellbore-strengthening PDF, wellbore collapse was completely avoided, the ROP was increased by 27%, and the drilling fluid cost was reduced by 35.3% in average.
2.5.2 Amphiphobic high-efficiency PDF
Reservoir damage and wellbore instability caused by capillary force existed in microcracks, and pores remain improperly solved for a long time. This problem has become increasingly influential with the exploration and development of low-permeable shale gas and sandstone gas in recent years. Under the capillary force, the reservoir rock will inevitably soak the drilling fluid into its interior and blocks oil and gas passage. The self-absorption of the drilling fluid also increases the wellbore collapse risk as the wellbore rock will soften after long-time immersion. Actually, although previously invented inhibitors and encapsulators could decrease wellbore rock hydration to a very low level, the wellbore-stabilizing effect is still limited because of the inevitable contact between the drilling fluid and wellbore rock caused by the capillary force. If the rock surface could be hydrophobic or even amphiphobic, the capillary force will be inverted, the drilling fluid’s self-absorption will be avoided, and the hydration of the wellbore rock will be further decreased to a great extent (Sheen et al., 2010; Zhang et al., 2011b; Ensikat et al., 2011; Lee et al., 2013; Li et al., 2016b; Lei et al., 2021a; Lv et al., 2022; Sun et al., 2022). The problem of reservoir damage and wellbore instability will thus be solved more completely. Based on the amphiphobicity theory of the downhole rock surface (Jiang, 2015; Cychosz and Thommes, 2018; Jiang, 2018), a multi-functional super-amphiphobic additive (MSA) with the effect of inhibition, reservoir protection, and lubrication is invented, and the amphiphobic high-efficiency PDF is established.
1) Performance of the MSA
a) Wettability reversal
The MSA is made of nano-SiO2 surface-grafted with fluorooctyl alkane (Figure 8A) (Jiang et al., 2018b; Ni et al., 2018; Jiang et al., 2019b). It could be well-dispersed in water, as shown in Figure 8B, 8C, 8D, without foaming (Chiu et al., 2007; Jin et al., 2017; Yan et al., 2017). After treatment with the MSA, the water-phase contact angle of the rock core, metal, and filter cake is increased from less than 20° to more than 150°, and the oil-phase contact angle of the rock core, metal, and filter cake is increased from 0° to 150° (Figure 9). Obviously, the MSA could prevent water-based and even oil-based drilling fluids from self-absorbing into the rock, thus preventing water-locking, as well as oil-locking, and decreasing the contact between the drilling fluid and rock.
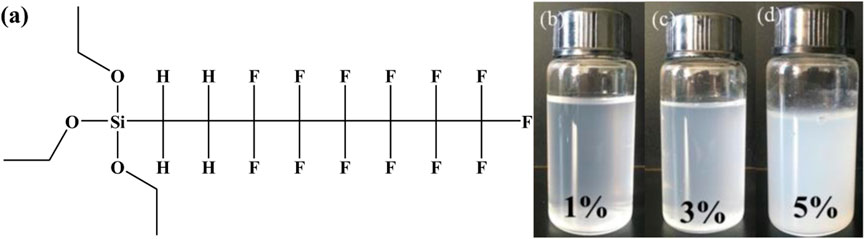
FIGURE 8. Molecular structure of the MSA (A) and MSA aqueous solution at 1% (B), 3% (C), and 5% (D) loading.
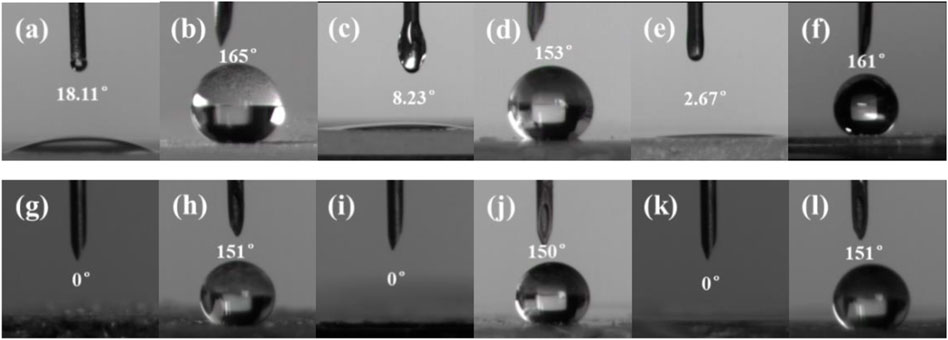
FIGURE 9. Influence of the MSA on the contact angle of the solid surface: the water-phase contact angle of the rock core before (A) and after treatment (B), of the metal before (C) and after treatment (D), and of the filter cake before (E) and after treatment (F); oil-phase (paraffin) contact angle of the rock core before (G) and after treatment (H), of the metal before (I) and after treatment (J), and of the filter cake before (K) and after treatment (L).
b) Inhibition
The MSA has a good inhibitive effect. A soaking experiment indicates that the swelling height of bentonite in 1% MSA solution after 25 h is only 1.59 mm, much lower than that in water and in solutions of KCl, diallyldimethylammonium chloride (DADMAC), polyether amine D230, and EPTAC (Figure 10A). The shale cuttings in MSA solution is also the highest, which is 78.79%, as shown in Figure 10B. It is worth mentioning that the MSA and biomimetic inhibitor have a synergistic effect, so better inhibition would be achieved if they are used together.
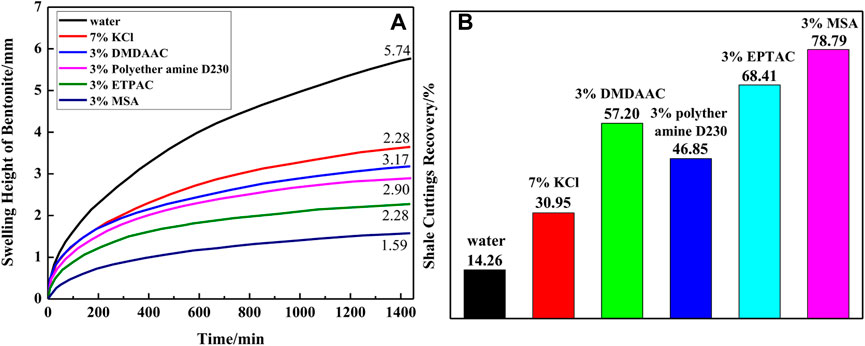
FIGURE 10. Swelling height of bentonite (A) and shale cuttings recovery (B) in different aqueous solutions.
c) Reservoir protection performance and lubricity
A general water-based drilling fluid with a formulation of “3% bentonite+0.5% fluid loss additive+1% blocking agent+1% starch+20% barite” is used as the base for the reservoir protection performance evaluation. As shown in Table 9, the reservoir rock core polluted by the drilling fluid with the MSA has the highest permeability recovery, indicating that the MSA has better reservoir protection performance than the low-permeable agent and film-forming agent.
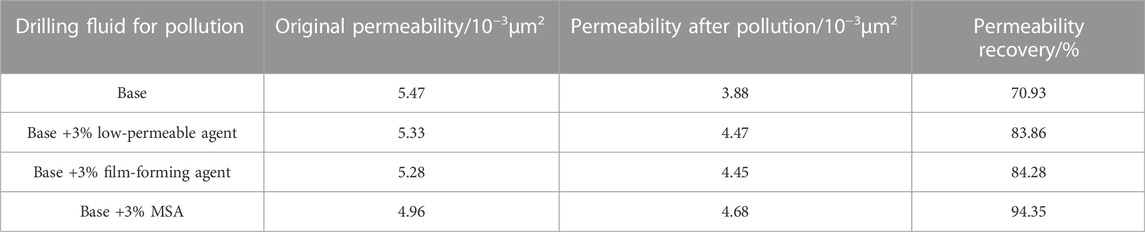
TABLE 9. Permeability recovery of the low-permeable rock core after polluting with different drilling fluids.
As a kind of nanomaterial, the MSA also has a lubricating effect. For one thing, the MSA could act like “microsphere bearing” at the friction interface which decreases the sliding resistance. For another, the nano-size enables the MSA to penetrate into micropits of the drilling tool and rock, thus decreasing the roughness of the friction interface. The synergistic effect between the MSA and biomimetic lubricant makes their co-use very effective in friction reduction. A 0.5% addition of the MSA could decrease the friction coefficient of the base loaded with the biomimetic lubricant from the original 0.12 to 0.08 (Table 6).
2) Performance of the amphiphobic high-efficiency PDF
The amphiphobic high-efficiency PDF is established on the core additive MSA and the three biomimetic additives. Compared with a typical low-density oil-based drilling fluid, the amphiphobic high-efficiency PDF has lower AV and PV, higher YP, and equivalent FLHTHP (Table 10). The cuttings recovery is higher, and the friction coefficient of the filter cake is lower, indicating that inhibition and lubricity of the amphiphobic high-efficiency PDF are better than those of the oil-based drilling fluid. The amphiphobic high-efficiency PDF also maintains good environmental performance with an EC50 of 3.02 × 104 mg/L and BOD5/CODCr of 0.188.

TABLE 10. Performance comparison (after ahot-rolling) between the amphiphobic high-efficiency PDF and typical oil-based drilling fluid (density is 1.4 g/cm3).
The amphiphobic high-efficiency PDF system has been applied in more than 200 complex structure wells in China’s oilfields including Changqing, Xinjiang, Huabei, Sichuan, and Jilin, which solves wellbore collapse, jamming, bit-balling, and reservoir damage problems effectively. In general, this technology has elevated the ROP by 32.8%, saved the drilling fluid cost by 29.3%, and increased single-well yield over 1.5 times on average.
3 Development tendency of PDF technology
By reviewing the development history of PDF technology and combining with global demands, especially China’s strategic demands of oil and gas exploration and development in the future, we illustrate the development tendency of PDF technology by introducing several possible and potential research directions.
3.1 Intelligent PDF
The drilling fluid with different ingredients has different functions, and different strata must match suitable drilling fluids with the required functions so that high-quality drilling could be achieved. In other words, before designing the drilling fluid, it is necessary to obtain the correct information about stratum rock, stratum fluid, stratum pressure, stratum temperature, etc., and to judge the downhole complexities and accidents that may occur. However, uncertainty of the stratum makes it difficult to obtain correct information, especially for newly discovered gas and oil blocks and difficult resources, which causes blind designing of the drilling fluid and increasing occurrence of downhole complexities or accidents. If there is a drilling fluid that can automatically identify the stratum condition and automatically adjust its performance based on the identification to meet the drilling requirement, downhole complexities and accidents could then be reduced fundamentally, and the principle of “the design drilling fluid must strictly depend on the stratum condition” would be changed. Such a drilling fluid with characteristics of “auto-identification, auto-adjustment, and auto-adaption” could be deemed as an intelligent drilling fluid.
The intelligent drilling fluid meets the requirement of the times. As seen from the development process of the petroleum industry, four technology revolutions in 1920∼1930s, 1960∼1970s, 1980∼1990s, and in the beginning of the 21st century all sharply increased oil and gas production in the world. In the near future, under the background of a surprising development and application of big data and artificial intelligence, the fifth revolution centering on “intelligence” may occur very possibly (Fatai, 2011; Abdelgawad et al., 2018; Nunoo, 2018; Bai et al., 2021; Lei et al., 2022a; Zhao et al., 2022). As an indispensable component of intelligent drilling, the intelligent drilling fluid is bound to become a promising technology development trend. Future development of the intelligent PDF should lay emphasis on the stimulus-responsive polymer, especially thermo- (Leblanc et al., 2015; Xie and Liu, 2017; Divers et al., 2018; Lei et al., 2021b; Lei et al., 2022b), salt- (Guancheng et al., 2019; Chang et al., 2020), pressure- (Carpenter, 2017; Mussab et al., 2021), mechano-, and pH-responsive polymers (Al-Anazi and Sharma, 2002; Choi et al., 2010; Li et al., 2018a; Chen et al., 2020), since these kinds of responsiveness are connected tightly with drilling fluid performance.
3.2 Ecological PDF
Protecting the environment is important during the process of changing nature for mankind, and it is also important to ensure the harmonious coexistence between mankind and nature and the sustainable development of the economy and society. The storage, use, and post-disposal of the drilling fluid will potentially cause environmental pollution. Although many previously developed PDF systems are environment-friendly, non-toxic, and capable of biodegrading, wastes they generate are still quite hard to dispose properly. Most harmless treatment technologies are passive, which often cause incomplete treatment and secondary pollution (Jiang et al., 2018c). If the drilling fluid could not reach the level of absolute non-toxic, degrade quickly, and yield environment-friendly degradation products, the degradation products could promote the growth of plants as nutrition or even become a necessary part of native ecological circulation; thus, the passive treatment could be avoided and the environment-friendly drilling fluid could be improved to the ecological drilling fluid. To develop ecological PDF, studies should lay emphasis on the invention of additives made of natural polymers and their derivatives including polysaccharide, poly-amino-acid, protein, and poly-fatty-acid (Mittal et al., 2014; Li et al., 2015; Panda et al., 2017; Jiang et al., 2018c; Clark and Santiso, 2018). Finding inspiration from substances generated in animal and plant metabolism could be an effective way. Additionally, it is necessary to build a new method to evaluate the ecological performance of the drilling fluid except COD and BOD measurements, like investigating the change of the ecological index of specific zones after the introduction of the drilling fluid.
3.3 PDF for natural gas hydrate (NGH)
Natural gas hydrate (NGH) is commonly known as “flammable ice,” which is a clean energy resource producing almost no residues after combustion. It is estimated that the total amount of NGH in the world is about twice the total amount of traditional oil and gas resources, while China has about four billion tons of oil-equivalent controllable NGH buried in deep water (Zhang et al., 2014), which has become an important potential superseding resource. Although NGH has been successfully exploited in trials in China, the United States, Japan, and other countries, there are many scientific and technological problems to be resolved; therefore, the commercial mining of NGH cannot be realized yet.
NGH is usually buried in loose mud and sand sediment 0∼500 m beneath the bottom of the sea. The stratum stability is very poor, which requires the drilling fluid to have good inhibition and excellent wellbore-strengthening performance to elevate the cementation of loose mud and sand. An underwater adhesive, like a polyelectrolyte complex adhesive based on ion bonds and a tannin-based adhesive based on multiple hydrogen bonds (Lee et al., 2020; Vahdati et al., 2020), could strongly adhere onto the substrate’s surface in an aqueous environment. It is promising to utilize an underwater adhesive to solve the inadequate cementation problem, but it is still needed to find practical methods to use in the drilling fluid. In addition, since NGH stores in the deep water, a drilling challenge also arises from a narrow safe density window, temperature difference, and strict environmental protection requirement. The flat-rheology PDF may be the best option for NGA drilling as the flat-rheological property has been proved very effective in weakening the fluctuation of the equivalent circulating density in synthetic drilling fluid cases. The core additive of the flat-rheology PDF should be a polymer flat-rheology modifier which could be a temperature-sensitive polymer capable of changing chain conformation from curled to extended with temperature increase. Polycaprolactam, poly-N-isopropylacrylamide, and their derivatives are promising choices (Xie et al., 2019).
3.4 PDF for deep-layer resources
Oil and gas remained in China are mostly buried in deep layers with high temperature and high pressure (Cai and Brown, 2017). It is estimated that China’s deep and ultra-deep resource storage is 67.1 billion tons (oil-equivalent), about 34% of the total. Of all the deep layer natural gas storage, tight gas and shale gas occupy more than 29%. The drilling fluid for deep-layer resources must have high temperature tolerance and high density simultaneously. For example, the temperature of some reservoirs in the Songliao Basin and Tarim Basin could reach over 250°C, and the drilling fluid density needed to balance formation pressure is 2.6 g/cm3 or even higher. In addition, a thick salt rock layer and salt gypsum layer are often encountered in deep-layer drilling, additionally requiring a drilling fluid with excellent anti-pollution ability. However, PDF technology does not make breakthroughs to overcome these challenges (Guo et al., 2019). The first problem to solve for the deep-layer PDF is to maintain good fluidity and control fluid loss under high temperature and high pressure. Research should emphasize on the development of a high-temperature polymer thinner, high-temperature gelator, and high-temperature fluid loss controller. Particularly, under the consideration that the high-temperature tolerance of a single organic polymer additive is limited, the development of high-temperature polymer additives should lay more focus on the synergistic effect between different polymers and on the use of inorganic polymers.
4 Conclusion
PDF technology development has undergone stages of “beginning,” “development,” “improvement,” “re-development,” and “re-improvement.” Dozens of polymer additives and PDF systems have been invented and applied successfully. HPWDFs, biomimetic wellbore-strengthening PDFs, and amphiphobic high-efficiency PDFs are outstanding representatives which have elevated the PDF’s performance to an unprecedented level, even better than a typical oil-based drilling fluid in some cases. They have avoided downhole complexities and problems, elevated ROP, saved drilling cost, alleviated environmental damage, and provided key technology support for safe, high-efficient, economic, and environmental exploration and development of unconventional gas resources.
The future development of PDF technology should be guided by strategic demands of exploration and development of promising resources. Higher and more special requirements are elevated by more particular and difficult situations, and polymer additives and PDFs should be innovated and improved accordingly. Multi-disciplinary integration of geology, chemistry, engineering, ecology, bionics, nanoscience, etc., and the multi-technology assembly of a stimulus-responsive polymer, intelligent material, natural material, nanomaterial, etc., will become the important dependence and practical method, and thus, revolutionary PDF technologies could be realized soon.
Author contributions
YH designed the paper structure, collected and organized data, and wrote the manuscript. GJ reviewed and revised the manuscript. TD, GW, and JH collected data from different parts. HD and MD proofread the manuscript.
Funding
This study received financial support from the National Natural Science Foundation of China (51991361 and 52004297), the China Postdoctoral Science Foundation (BX20200384), and the Foundation of China University of Petroleum (Beijing) (2462022YJRC005).
Conflict of interest
The authors declare that the research was conducted in the absence of any commercial or financial relationships that could be construed as a potential conflict of interest.
The reviewer GZ declared a shared parent affiliation with the authors to the handling editor at the time of review.
Publisher’s note
All claims expressed in this article are solely those of the authors and do not necessarily represent those of their affiliated organizations, or those of the publisher, the editors, and the reviewers. Any product that may be evaluated in this article, or claim that may be made by its manufacturer, is not guaranteed or endorsed by the publisher.
References
Abdelgawad, K., Elkatatny, S., Moussa, T., Mahmoud, M., and Patil, S. (2018). “Real time determination of rheological properties of spud drilling fluids using a hybrid artificial intelligence technique[C],” in SPE Kingdom of Saudi Arabia Annual Technical Symposium and Exhibition, Dammam, Saudi Arabia, 23-26 April.
Adnan Hamad, Bahati, He, Miao, Xu, Mingbiao, Liu, W., Mpelwa, M., Tang, S., et al. (2020). A novel amphoteric polymer as a rheology enhancer and fluid-loss control agent for water-based drilling muds at elevated temperatures. ACS Omega 5 (15), 8483–8495. doi:10.1021/acsomega.9b03774
Aftab, A., Ali, M., Sahito, M. F., Mohanty, U. S., Jha, N. K., Akhondzadeh, H., et al. (2020). Environmental friendliness and high performance of multifunctional tween 80/ZnO-Nanoparticles-Added water-based drilling fluid: An experimental approach. ACS Sustain. Chem. Eng. 8 (30), 11224–11243. doi:10.1021/acssuschemeng.0c02661
Ahamed, M. A. A., Perera, M. S. A., Dong-Yin, L., Ranjith, P., and Matthai, S. (2019). Proppant damage mechanisms in coal seam reservoirs during the hydraulic fracturing process: A review. Fuel 253, 615–629. doi:10.1016/j.fuel.2019.04.166
Ahmad, H. M., Kamal, M. S., Murtaza, M., Khan, S., and Al-Harthi, M. A. (2018). High molecular weight copolymers as rheology modifier and fluid loss additive for water-based drilling fluids. J. Mol. Liq. 252, 133–143. doi:10.1016/j.molliq.2017.12.135
Ahmad, H. M., Kamal, M. S., Murtaza, M., Khan, S., and Al-Harthi, M. (2019). “Alteration of wettability and hydration properties of shale using ionic liquids in water-based drilling fluids [C],” in International Petroleum Exhibition & Conference, Abu Dhabi, UAE, 11-14 November.
Ai-Yasiri, Mortatha, and Weng, Dongsheng (2019). Gr-Al2O3 nanoparticles-based multifunctional drilling fluid[J]. Ind. Eng. Chem. Res. 58 (23), 10084–10091. doi:10.1021/acs.iecr.9b00896
Akkouche, A., Benmounah, A., Gueciouer, A., and Chalah, K. (2020). Valorization of mixed metal hydroxide on Algerian Na-Bentonite suspensions: Application to water-based drilling fluid. Egypt. J. Petroleum 29 (2), 127–131. doi:10.1016/j.ejpe.2019.12.005
Al-Anazi, H. A., and Sharma, M. M. (2002). in Use of a pH Sensitive Polymer for Conformance Control[C]International Symposium and Exhibition on Formation Damage Control, Lafayette, Louisiana, 20-21 February.
An, Y., Jiang, G., Qi, Y., Ge, Q., Zhang, L., and Ren, Y. (2015). Synthesis of nano-plugging agent based on AM/AMPS/NVP terpolymer. J. Petroleum Sci. Eng. 135, 505–514. doi:10.1016/j.petrol.2015.10.014
An, Y., Jiang, G., Ren, Y., Zhang, L., Qi, Y., and Ge, Q. (2015). An environmental friendly and biodegradable shale inhibitor based on chitosan quaternary ammonium salt. J. Petroleum Sci. Eng. 135, 253–260. doi:10.1016/j.petrol.2015.09.005
An, Y., and Yu, P. (2018). A strong inhibition of polyethyleneimine as shale inhibitor in drilling fluid. J. Petroleum Sci. Eng. 161, 1–8. doi:10.1016/j.petrol.2017.11.029
An, Yuxiu, Jiang, Guancheng, Qi, Yourong, Xianbin, H., and He, S. (2016). High-performance shale plugging agent based on chemically modified graphene. J. Nat. Gas Sci. Eng. 32, 347–355. doi:10.1016/j.jngse.2016.04.048
Bai, Y., Zhang, Q., Sun, J., Lv, K., Shang, X., Liu, C., et al. (2021). Self-healing hydrogels and their action mechanism in oil–gas drilling and development engineering: A systematic review and prospect. J. Nat. Gas Sci. Eng. 96, 104250. doi:10.1016/j.jngse.2021.104250
Bakerhughes Media Center (2012). LATIDRILL water-based fluid system improves shale drilling[EB/OL]. Available at http://www.bakerhughes.com/news-and-media/media-center/product-announce-ments/houston-texas-august-7-2012-latidrill.
Beg, M., Sharma, S., and Ojha, U. (2018). Effect of cationic copolyelectrolyte additives on drilling fluids for shales. J. Petroleum Sci. Eng. 161, 506–514. doi:10.1016/j.petrol.2017.12.009
Beihoffer, T. W., Growcock, F. B., Deem, C. K., Dorrough, D. S., Bray, R. P., Munro, R., et al. (1992). “Field testing of a cationic polymer/brine drilling fluid in the North Sea[C],” in SPE Annual Technical Conference and Exhibition, Washington, D.C, 4-7 October.
Bland, R. G., Waughman, R. R., Tomkins, P. G., Halliday, W. S., Pessier, R. C., Isbell, M. R., et al. (2002). “Water-based alternatives to oil-based muds: Do they actually exist?[C],” in IADC/SPE Drilling Conference, Dallas, Texas, 26-28 February.
Blattel, Steve R., and Rupert, J. P. (1982). “The effect of weight material type on rate of penetration using dispersed and non-dispersed water-base muds[C],” in SPE Annual Technical Conference and Exhibition, New Orleans, Louisiana, 26-29 September.
Boul, P. J., Reddy, B. R., Zhang, J., Thaemlitz, C., et al. (2016). “Functionalized nanosilicas as shale inhibitors in water-based drilling fluids[C],” in Offshore Technology Conference, Houston, Texas, 2-5 May.
Boyou, N. V., Ismail, I., Wan Sulaiman, W. R., Sharifi Haddad, A., Husein, N., Hui, H. T., et al. (2019). Experimental investigation of hole cleaning in directional drilling by using nano-enhanced water-based drilling fluids. J. Petroleum Sci. Eng. 176, 220–231. doi:10.1016/j.petrol.2019.01.063
BP. Statistical Review of World Energy (2020). Global corporate energy-economics statistical-review-of-world-energy. Available at https://www.bp.com/en/global/corporate/energy-economics/statistical-review-of-world-energy.html.
Browning, W. C., and Chesser, B. G. (1972). Polymer-polyelectrolyte drilling fluid systems. J. Petroleum Technol. 24 (10), 1255–1263. doi:10.2118/3063-pa
Bruton, J. R., and McLaurine, H. C. (1993). “Modified poly-amino acid hydration suppressant proves successful in controlling reactive shales [C],” in SPE Annual Technical Conference and Exhibition, Houston, Texas, 3-6 October.
Burden, P., Dimitriadis, K., and Clements, K. (2013). “Drilling fluid selection methodology for environmentally sensitive areas[C],” in SPE/IADC Drilling Conference, Amsterdam, The Netherlands, 5-7 March.
Bybee, K. (2004). Drilling fluid strengthens wellbore. J. Petroleum Technol. 56 (11), 59–60. doi:10.2118/1104-0059-jpt
Cai, M. F., and Brown, E. T. (2017). Challenges in the mining and utilization of deep mineral resources. Engineering 3 (4), 432–433. doi:10.1016/j.eng.2017.04.027
Cao, Jie, Meng, Lingwei, Yang, Yuping, Zhu, Y., Wang, X., Yao, C., et al. (2017). Novel acrylamide/2-acrylamide-2-methylpropanesulfonic acid/4-vinylpyridine terpolymer as an anti-calcium contamination fluid-loss additive for water-based drilling fluids. Energy fuels. 31 (11), 11963–11970. doi:10.1021/acs.energyfuels.7b02354
Carpenter, Chris (2017). Advancement in openhole sand-control applications with shape-memory polymer. J. Petroleum Technol. 69 (10), 102–104. doi:10.2118/1017-0102-jpt
CelsoMorooka, K., IvanMendes, R. Guilhermem José R. P., and Mendes, J. R. (2001). Development of intelligent systems for well drilling and petroleum production. J. Petroleum Sci. Eng. 32 (2-4), 191–199. doi:10.1016/s0920-4105(01)00161-9
Chang, X., Sun, J., Zhang, F., Bai, Y., Lv, K., Wang, J., et al. (2020). Salt-responsive zwitterionic polymer brush based on modified silica nanoparticles as a fluid-loss additive in water-based drilling fluids. Energy fuels. 34 (2), 1669–1679. doi:10.1021/acs.energyfuels.9b04109
Chen, Jingsi, Peng, Qiongyao, Peng, Xuwen, Han, L., Wang, X., Wang, J., et al. (2020). Recent advances in mechano-responsive hydrogels for biomedical applications. ACS Appl. Polym. Mat. 2 (3), 1092–1107. doi:10.1021/acsapm.0c00019
Chiu, C. W., Cheng, W. T., Wang, Y. P., and Lin, J. J. (2007). Fine dispersion of hydrophobic silicate platelets in anhydride-cured epoxy nanocomposites. Ind. Eng. Chem. Res. 46 (22), 7384–7388. doi:10.1021/ie070629b
Choi, S. K., Sharma, M. M., Bryant, S. L., and Huh, C. (2010). pH-sensitive polymers for novel conformance-control and polymer-flood applications. SPE Reserv. Eval. Eng. 13 (6), 926–939. doi:10.2118/121686-pa
Chu, Q., Lin, L., and Zhao, Y. (2019). Hyperbranched polyethylenimine modified with silane coupling agent as shale inhibitor for water-based drilling fluids. J. Petroleum Sci. Eng. 182, 106333. doi:10.1016/j.petrol.2019.106333
Clark, J. A., and Santiso, E. E. (2018). Carbon sequestration through CO2 foam-enhanced oil recovery: A green chemistry perspective[J]. Engineering 4 (3), 336–342. doi:10.1016/j.eng.2018.05.006
Clear Solutions Service (2022). Pure-Bore[EB/OL]. Available at https://www.drilling-products.com/wp-content.
Cychosz, K. A., and Thommes, M. (2018). Progress in the physisorption characterization of nanoporous gas storage materials. Engineering 4 (4), 559–566. doi:10.1016/j.eng.2018.06.001
Divers, T., Al-Hashmi, A. R., Al-Maamari, R. S., Favero, C., et al. (2018). “Development of thermo-responsive polymers for CEOR in extreme conditions: Applicability to Oman oil fields[C],” in SPE EOR Conference at Oil and Gas West Asia, Muscat, Oman, 26-28 March.
Duane, B. (1972). “Non-dispersed weighted muds[C],” in Fall Meeting of the Society of Petroleum Engineers of AIME, San Antonio, Texas, 8-11 October.
Dye, W., Daugereau, K., Hansen, N., Otto, M., Shoults, L., Leaper, R., et al. (2005). “New water-based mud balances high-performance drilling and environmental compliance[C],” in SPE/IADC Drilling Conference, Amsterdam, Netherlands, 23-25 February.
Dye, W., Daugereau, K., Hansen, N., Otto, M., Shoults, L., Leaper, R., et al. (2006). New water-based mud balances high-performance drilling and environmental compliance. SPE Drill. Complet. 21 (4), 255–267. doi:10.2118/92367-pa
Eia, J. T., and Hernandez, E. (2006). “Environmental advances in drilling fluids and waste operations applying novel technology for fluid recovery and recycling (Russian)[C],” in SPE Russian Oil and Gas Technical Conference and Exhibition, Moscow, Russia, 3-6 October.
Eia, J. T. (2006). “Environmental advances in drilling fluids, completion fluid and waste operations applying novel technology for fluid recovery and recycling,” in SPE Russian Oil and Gas Technical Conference and Exhibition, Moscow, Russia, 3-6 October.
Eichelberger, J. C. (1997). Drilling volcanoes. Science 278 (5340), 1084–1085. doi:10.1126/science.278.5340.1084
Ensikat, H. J., Ditschekuru, P., Neinhuis, C., and Barthlott, W. (2011). Superhydrophobicity in perfection: The outstanding properties of the lotus leaf. Beilstein J. Nanotechnol. 2 (1), 152–161. doi:10.3762/bjnano.2.19
Fatai, A. A. (2011). “Artificial intelligence application in reservoir characterization and modeling: Whitening the black box[C],” in SPE Saudi Arabia section Young Professionals Technical Symposium, Dhahran, Saudi Arabia, 14-16 March.
Fernandez, I. J. (2005). “Evaluation of cationic water-soluble polymers with improved thermal stability[C],” in SPE International Symposium on Oilfield Chemistry, The Woodlands, Texas, 2-4 February.
Ferreira, C. C., Teixeira, G. T., Lachter, E. R., and Nascimento, R. S. V. (2016). Partially hydrophobized hyperbranched polyglycerols as non-ionic reactive shale inhibitors for water-based drilling fluids. Appl. Clay Sci. 132-133, 122–132. doi:10.1016/j.clay.2016.05.025
Field, L. J. (1968). “Low-solids non-dispersed mud usage in western Canada[C],” in Drilling and Production Practice, New York, 1 January.
Foshee, W. C., Jennings, R. R., and West, T. J. (1977). “Preparation and testing of partially hydrolyzed polyacrylamide solutions[C],” in SPE International Symposium on Oilfield Chemistry Annual Technical Meeting, San Antonio, Texas, 14-17 February.
Growcock, F., and Harvey, T. (2005). “Chapter 2 - drilling fluids,” in Drilling fluids processing handbook (Burlington: Gulf Professional Publishing).
Guancheng, J., Fan, L., Jinxi, W., Yinbo, H., Zhengqiang, D., Kai, W., et al. (2018). Bionic lubricant and its preparation method and application.
Guancheng, J., Deli, G., Guangchang, M., Jinsheng, S., Wang, Xi, Pu, Xiaolin, et al. (2018). Use of dual-cationg fluorocarbon surfactant as amphiphobic wettability reversal agent in drilling fluid.
Guancheng, J., Yinbo, H. E., Wuge, C., Yang, L., and Ye, C. (2019). A saturated saltwater drilling fluid based on salt-responsive polyampholytes. Petroleum Explor. Dev. 46 (2), 401–406. doi:10.1016/s1876-3804(19)60020-0
Guancheng, J., Yang, X., Xianzhu, W., Junbin, C., Wei, O., Taotao, L., et al. (2015). Method for preparation of biomimetic polymer for stabilizing wellbore and drilling fluid.
Guo, X. S., Hu, D. F., Li, Y. P., Duan, J., Zhang, X., Fan, X., et al. (2019). Theoretical progress and key technologies of onshore ultra-deep oil/gas exploration. Engineering 5 (3), 458–470. doi:10.1016/j.eng.2019.01.012
Guo, Z., Zhou, F., Hao, J., and Liu, W. (2005). Stable biomimetic super-hydrophobic engineering materials. J. Am. Chem. Soc. 127 (45), 15670–15671. doi:10.1021/ja0547836
Hale, A. H., and Mody, F. K. (1993). “Partially hydrolyzed polyacrylamide (PHPA) mud systems for Gulf of Mexico deepwater prospects[C],” in SPE International Symposium on Oilfield Chemistry, New Orleans, Louisiana, Texas, 2-5 March.
Halliburton Service (2012). Drilling fluids solutions[EB/OL]. https://www.halliburton.com/en-US/ps/baroid/fluid-services/drilling-fluids-solutions/water-based/performadrill.
Halliburton Service (2013). Drilling fluids solutions[EB/OL]. https://www.halliburton.com/content/dam/ps/public/bar/contents/Case-Histories/web/PERFORMADRIL-Angola.pdf.
Halliday, W., Clapper, D. K., and Smalling, M. (1998). “New gas hydrate inhibitors for deepwater drilling fluids[C],” in IADC/SPE Drilling Conference, Dallas, Texas, 3-6 March.
Hammond, A. L. (1975). Exploring the continent by drilling: A new proposal. Science 189 (4196), 35. doi:10.1126/science.189.4196.35
Hamza, A., Shamlooh, M., Hussein, I. A., Nasser, M., and Salehi, S. (2019). Polymeric formulations used for loss circulation materials and wellbore strengthening applications in oil and gas wells: A review. J. Petroleum Sci. Eng. 180, 197–214. doi:10.1016/j.petrol.2019.05.022
Hodder, M., Ross, N., Gibb, S., and Redgrave, S. (2006). “Successful Drilling of a High Step-Out Ratio Well With Water-Based Mud in Morecambe Bay[C],” in SPE Annual Technical Conference and Exhibition, San Antonio, Texas, USA, 24-27 September.
Hou, H-S., Wang, C-S., Zhang, J-D., Ma, F., Fu, W., Wang, P-J, et al. (2018). Deep Continental Scientific Drilling Engineering Project in Songliao Basin: progress in Earth Science research. China Geol. 1 (2), 173–186. doi:10.31035/cg2018036
Huang, Haoqing (2004). An environmentally safe water-base drilling fluid ULTRADRIL[J]. Drill. Fluid & Complet. Fluid 21 (6), 4–7.
Huang, X., Lv, K., Sun, J., Lu, Z., Bai, Y., Shen, H., et al. (2019). Enhancement of thermal stability of drilling fluid using laponite nanoparticles under extreme temperature conditions. Mater. Lett. 248, 146–149. doi:10.1016/j.matlet.2019.04.005
Huang, Xianbin, Shen, Haokun, Sun, Jinsheng, Lv, K., Liu, J., Dong, X., et al. (2018). Nanoscale Laponite as a Potential Shale Inhibitor in Water-Based Drilling Fluid for Stabilization of Wellbore Stability and Mechanism Study. ACS Appl. Mat. Interfaces 10 (39), 33252–33259. doi:10.1021/acsami.8b11419
Huang, Xianbin, Sun, Jinsheng, He, Li, Wang, R., and Lv, K. (2022). Fabrication of a Hydrophobic Hierarchical Surface on Shale Using Modified Nano-SiO2 for Strengthening the Wellbore Wall in Drilling Engineering. Engineering 11, 101–110. doi:10.1016/j.eng.2021.05.021
Huang, Xianbin, Sun, Jinsheng, Lv, Kaihe, Liu, J., Shen, H., and Zhang, F. (2018). Application of core-shell structural acrylic resin/nano-SiO2 composite in water based drilling fluid to plug shale pores. J. Nat. Gas Sci. Eng. 55, 418–425. doi:10.1016/j.jngse.2018.05.023
Jiang, G., Li, X., Zhu, H., Yang, L., Li, Y., Wang, T., et al. (2019). Improved shale hydration inhibition with combination of gelatin and KCl or EPTAC, an environmentally friendly inhibitor for water-based drilling fluids. J. Appl. Polym. Sci. 136 (22), 47585. doi:10.1002/app.47585
Jiang, Guancheng (2018). Gas wettability of reservoir rock surfaces with porous media. Beijing: Gulf Professional Publishing.
Jiang, Guancheng, Ni, Xiaoxiao, Yang, Lili, Ma, Guangchang, Peng, Chunyao, Gao, Deli, et al. (2019). Super-amphiphobic composite material and use of the same as inhibitor, lubricant, reservoir protectant, and accelerator in water-based drilling fluids. USA.
Jiang, Guancheng, Peng, Shuanglei, Li, Xinliang, Yang, L., and Soares, J. B. (2018). Preparation of amphoteric starch-based flocculants by reactive extrusion for removing useless solids from water-based drilling fluids. Colloids Surfaces A Physicochem. Eng. Aspects 558, 343–350. doi:10.1016/j.colsurfa.2018.08.077
Jiang, Guancheng (2015). Theoretical basis and application of gas wettability of rock surface in porous media reservoirs. Dongying: China University of Petroleum Press.
Jiang, Guancheng, Wu, Xianzhu, Chen, Junbin, et al. (2015). Biomimetic polymer for stabilizing wellbore and method for preparation of the same and drilling fluid.
Jin, J., Wang, Y., Nguyen, T., Nguyen, A. V., Wei, M., and Bai, B. (2017). The effect of gas-wetting nano-particle on the fluid flowing behavior in porous media. Fuel 196, 431–441. doi:10.1016/j.fuel.2017.01.083
Khalil, M., and Jan, B. M. (2012). Viscoplastic Modeling of a Novel Lightweight Biopolymer Drilling Fluid for Underbalanced Drilling. Ind. Eng. Chem. Res. 51 (10), 4056–4068. doi:10.1021/ie200811z
Kharitonov, A., Zapotylok, D. A., and Chertenkov, M. (2011). “High Performance KCl-Based Mud Provides Outstanding Drilling Performance in Kyrtael, Komi Republic, Russia[C],” in SPE Arctic and Extreme Environments Conference and Exhibition, Moscow, Russia, 18-20 October.
Kong, Qing-ming, Chang, Feng, Sun, Cheng-chun, Sha, Dong, Chen, Shao-liang, Liu, Chuan-du, et al. (2006). Application of ULTRADRILTM water-based drilling fluid in Zhanghai 502FH well[J]. Drill. Fluid & Complet. Fluid 23 (6), 71–76.
Kosynkin, D. V., Ceriotti, G., Wilson, K. C., Lomeda, J. R., Scorsone, J. T., Patel, A. D., et al. (2012). Graphene Oxide as a High-Performance Fluid-Loss-Control Additive in Water-Based Drilling Fluids. ACS Appl. Mat. Interfaces 4 (1), 222–227. doi:10.1021/am2012799
Ladd, H. S., Tracey, J. I., and Gross, M. G. (1967). Drilling on Midway Atoll, Hawaii. Science 156 (3778), 1088–1094. doi:10.1126/science.156.3778.1088
Lanman, D. E., and Willingham, R. W. (1970). “Use of Non-Beneficiating Selectively Flocculated, Low- Solids, Non- Dispersed Mud Systems In Wyoming's Big Horn Basin[C],” in Drilling and Production Practice, Washington, D.C, 1 January.
Lawhon, Charles P., and Simpson, Jay P. (1967). Laboratory Drilling Rate and Filtration Studies of Clay And Polymer Drilling Fluids. J. Petroleum Technol. 19 (5), 688–694. doi:10.2118/1521-pa
Leblanc, T., Braun, O., Thomas, A., Divers, T., Gaillard, N., Favero, C., et al. (2015). “Rheological Properties of Stimuli-Responsive Polymers in Solution to Improve the Salinity and Temperature Performances of Polymer-Based Chemical Enhanced Oil Recovery Technologies[C],” in SPE Asia Pacific Enhanced Oil Recovery Conference, Kuala Lumpur, Malaysia, 11-13 August.
Lee, Daiheon, Hwang, Honggu, Kim, Jun-sung, Park, J., Youn, D., Kim, D., et al. (2020). Vata: A Poly(vinyl alcohol)- and Tannic Acid-Based Nontoxic Underwater Adhesive. ACS Appl. Mat. Interfaces 12 (18), 20933–20941. doi:10.1021/acsami.0c02037
Lee, S. E., Kim, H. J., Lee, S. H., and Choi, D. G. (2013). Superamphiphobic Surface by Nanotransfer Molding and Isotropic Etching. Langmuir 29 (25), 8070–8075. doi:10.1021/la4011086
Lei, M., Huang, W., Sun, J., et al. (2022). The Utilization of Self-Crosslinkable Nanoparticles as High-Temperature Plugging Agent in Water-Based Drilling Fluid[J]. SPE J., 1–14.
Lei, M., Huang, W., Sun, J., Shao, Z., Ouyang, Y., and Han, Q. (2021). Synthesis of hydrophobically modified polyampholyte based on epoxidized soybean oil as shale inhibitor in water-based drilling fluid. Colloids Surfaces A Physicochem. Eng. Aspects 622, 126664. doi:10.1016/j.colsurfa.2021.126664
Lei, M., Huang, W., Sun, J., Shao, Z., Zhao, L., Zheng, K., et al. (2021). Synthesis and characterization of thermo-responsive polymer based on carboxymethyl chitosan and its potential application in water-based drilling fluid. Colloids Surfaces A Physicochem. Eng. Aspects 629, 127478. doi:10.1016/j.colsurfa.2021.127478
Lei, M., Huang, W., Tong, Y., Sun, J., Jin, Z., Li, X., et al. (2022). Synthesis and characterization of chitosan-based graft copolymers with temperature-switchable and self-adaptive plugging performance for drilling in microporous formation. J. Mol. Liq. 366, 120246. doi:10.1016/j.molliq.2022.120246
Lei, W., Hui, Z., Xiaodong, P., Wang, P., Zhao, N., Chu, S., et al. (2019). Water-sensitive damage mechanism and the injection water source optimization of low permeability sandy conglomerate reservoirs. Petroleum Explor. Dev. 46 (6), 1218–1230. doi:10.1016/s1876-3804(19)60275-2
Li, F., Du, M., and Zheng, Q. (2016). Dopamine/Silica Nanoparticle Assembled, Microscale Porous Structure for Versatile Superamphiphobic Coating. Acs Nano 10 (2), 2910–2921. doi:10.1021/acsnano.6b00036
Li, J., Sun, J., Lv, K., Ji, Y., Ji, J., and Liu, J. (2022). Nano-Modified Polymer Gels as Temperature- and Salt-Resistant Fluid-Loss Additive for Water-Based Drilling Fluids. Gels 8 (9), 547. doi:10.3390/gels8090547
Li, J., Sun, J., Lv, K., Ji, Y., Liu, J., Huang, X., et al. (2022). Temperature- and Salt-Resistant Micro-Crosslinked Polyampholyte Gel as Fluid-Loss Additive for Water-Based Drilling Fluids. Gels 8 (5), 289. doi:10.3390/gels8050289
Li, M. C., Ren, S., Zhang, X., Dong, L., Lei, T., Lee, S., et al. (2018). Surface-Chemistry-Tuned Cellulose Nanocrystals in a Bentonite Suspension for Water-Based Drilling Fluids. ACS Appl. Nano Mat. 1 (12), 7039–7051. doi:10.1021/acsanm.8b01830
Li, M-C, Wu, Q., Han, J., Mei, C., Lei, T., Lee, S-y., et al. (2020). Overcoming Salt Contamination of Bentonite Water-Based Drilling Fluids with Blended Dual-Functionalized Cellulose Nanocrystals. ACS Sustain. Chem. Eng. 8 (31), 11569–11578. doi:10.1021/acssuschemeng.0c02774
Li, M., Wu, Q., Lei, T., Mei, C., Xu, X., Lee, S., et al. (2020). Thermothickening Drilling Fluids Containing Bentonite and Dual-Functionalized Cellulose Nanocrystals. Energy fuels. 34 (7), 8206–8215. doi:10.1021/acs.energyfuels.0c01192
Li, M. C., Wu, Q., Song, K., French, A. D., Mei, C., and Lei, T. (2018). pH-Responsive Water-Based Drilling Fluids Containing Bentonite and Chitin Nanocrystals. ACS Sustain. Chem. Eng. 6 (3), 3783–3795. doi:10.1021/acssuschemeng.7b04156
Li, Mei-Chun, Wu, Qinglin, Song, Kunlin, De Hoop, C. F., Lee, S., Qing, Y., et al. (2016). Cellulose Nanocrystals and Polyanionic Cellulose as Additives in Bentonite Water-Based Drilling Fluids: Rheological Modeling and Filtration Mechanisms. Ind. Eng. Chem. Res. 55 (1), 133–143. doi:10.1021/acs.iecr.5b03510
Li, Mei-chun, Wu, Qingling, Song, Kunlin, Lee, S., Jin, C., Ren, S., et al. (2015). Soy Protein Isolate As Fluid Loss Additive in Bentonite–Water-Based Drilling Fluids. ACS Appl. Mat. Interfaces 7 (44), 24799–24809. doi:10.1021/acsami.5b07883
Li, X., Jiang, G., Yang, L., Wang, K., Shi, H., Li, G., et al. (2019). Application of Gelatin Quaternary Ammonium Salt as an Environmentally Friendly Shale Inhibitor for Water-Based Drilling Fluids. Energy fuels. 33 (9), 9342–9350. doi:10.1021/acs.energyfuels.9b01798
Li, Xinliang, Jiang, Guancheng, Shen, Xiulun, et al. (2020). Poly-l-arginine as a High-Performance and Biodegradable Shale Inhibitor in Water-Based Drilling Fluids for Stabilizing Wellbore. ACS Sustain. Chem. Eng. 8 (4), 1899–1907. doi:10.1021/acssuschemeng.9b06220
Li, Z., Li, Q., Yang, G., Zhang, F., and Ma, T. (2019). “The Synthesis and Application of a New Plugging Inhibitor PAS-5 in Water-Based Drilling Fluid [C],” in SPE Middle East Oil and Gas Show and Conference, Manama, Bahrain, 18-21 March.
Liu, F., Jiang, G., Peng, S., He, Y., and Wang, J. (2016). Amphoteric Polymer as an Anti-calcium Contamination Fluid-Loss Additive in Water-Based Drilling Fluids. Energy fuels. 30 (9), 7221–7228. doi:10.1021/acs.energyfuels.6b01567
Liu, L., Sun, J., Wang, R., Qu, Y., Liu, F., Yang, J., et al. (2022). Synthesis of a new high temperature and salt resistant zwitterionic filtrate reducer and its application in water-based drilling fluid. Colloids Surfaces A Physicochem. Eng. Aspects 651, 129730. doi:10.1016/j.colsurfa.2022.129730
Liu, Pingde, Niu, Yabin, and Zhang, Mei (2005). Development of PL for Zwitterionic Polymer Drilling Fluid System[J]. Nat. Gas. Ind. 25 (3), 71–73.
Liu, Yuqing (1992). Research and Application of Cationic Polymer Drilling Fluid[J]. Nat. Gas. Ind. 12 (3), 46–52.
Lourenco, A. M. F., Nakagawa, E. Y., Martins, A. L., et al. (2006). “Investigating Solids-Carrying Capacity for an Optimized Hydraulics Program in Aerated Polymer-Based-Fluid Drilling[C],” in IADC/SPE Drilling Conference, Miami, Florida, USA, 21-23 February.
Lummus, J. L., and Anderson, D. B. (1964). “Low-solids Salt-water Drilling Fluid[C],” in Drilling and Production Practice, New York, 1 January.
Lv, K., Liu, J., Jin, J., Sun, J., Huang, X., Liu, J., et al. (2022). Synthesis of a novel cationic hydrophobic shale inhibitor with preferable wellbore stability. Colloids Surfaces A Physicochem. Eng. Aspects 637, 128274. doi:10.1016/j.colsurfa.2022.128274
Ma, J., Xia, B., Yu, P., and An, Y. (2020). Comparison of an Emulsion- and Solution-Prepared Acrylamide/AMPS Copolymer for a Fluid Loss Agent in Drilling Fluid. ACS Omega 5 (22), 12892–12904. doi:10.1021/acsomega.0c00665
Ma, Jingyuan, Yu, Peizhi, Xia, Boru, and Wang, Z. (2019). Synthesis of a Biodegradable and Environmentally Friendly Shale Inhibitor Based on Chitosan-Grafted l-Arginine for Wellbore Stability and the Mechanism Study. ACS Appl. Bio Mat. 2 (10), 4303–4315. doi:10.1021/acsabm.9b00566
Madkour, T. M., Fadl, S., Dardir, M. M., and Mekewi, M. A. (2016). High performance nature of biodegradable polymeric nanocomposites for oil-well drilling fluids. Egypt. J. Petroleum 25 (2), 281–291. doi:10.1016/j.ejpe.2015.09.004
Mech, Deepjyoti, and Sangwai Jitendra, S. (2016). Effect of molecular weight of polyethylene glycol (PEG), a hydrate inhibitive water-based drilling fluid additive, on the formation and dissociation kinetics of methane hydrate. J. Nat. Gas Sci. Eng. 35, 1441–1452. doi:10.1016/j.jngse.2016.06.020
Memon, A., Borman, C., Mohammadzadeh, O., Garcia, M., Tristancho, D. J. R., and Ratulowski, J. (2017). Systematic evaluation of asphaltene formation damage of black oil reservoir fluid from Lake Maracaibo, Venezuela. Fuel 206, 258–275. doi:10.1016/j.fuel.2017.05.024
Miller, James D. (1980). “Application Of Low Solids Mud In Cotton Valley Drilling[C],” in SPE Cotton Valley Symposium, Tyler, Texas, 21 May.
Mittal, H., Jindal, R., Kaith, B. S., Maity, A., and Ray, S. S. (2014). Synthesis and flocculation properties of gum ghatti and poly(acrylamide-co-acrylonitrile) based biodegradable hydrogels. Carbohydr. Polym. 114, 321–329. doi:10.1016/j.carbpol.2014.08.029
Mondshine, T. C. (1973). “A New Potassium Based Mud System[C],” in Fall Meeting of the Society of Petroleum Engineers of AIME, Las Vegas, Nevada, 30 September-3 October.
Morton, K., Bomar, B., Schiller, M., et al. (2005). “Selection and evaluation criteria for high performance drilling fluids[C],” in SPE Annual Technical Conference and Exhibition, Dallas, Texas, 9-12 October.
Mussab, M., Saeed, S., Guoqiang, L., Fan, J., and Teodoriu, C. (2021). Loss circulation prevention in geothermal drilling by shape memory polymer. Geothermics 89, 101943. doi:10.1016/j.geothermics.2020.101943
Ni, Xiaoming, Liu, Zedong, and Wei, Jianping (2019). Quantitative evaluation of the impacts of drilling mud on the damage degree to the permeability of fractures at different scales in coal reservoirs. Fuel 236, 382–393. doi:10.1016/j.fuel.2018.08.130
Ni, Xiaoxiao, Jiang, Guancheng, Fan, Liu, and Deng, Z. (2018). Synthesis of an Amphiphobic Nanofluid with a Novel Structure and Its Wettability Alteration on Low-Permeability Sandstone Reservoirs. Energy fuels. 32 (4), 4747–4753. doi:10.1021/acs.energyfuels.7b03931
Ni, Xiaoxiao, Jiang, Guancheng, Li, Yiying, Yang, L., Li, W., Wang, K., et al. (2019). Synthesis of superhydrophobic nanofluids as shale inhibitor and study of the inhibition mechanism. Appl. Surf. Sci. 484, 957–965. doi:10.1016/j.apsusc.2019.04.167
Niu Yabin, Zhang Daming, Xiao Hongzhang, Zheng Wumuxi, and Li Jian (1995). “Research and Application of Amphoteric Polymers for Drilling Fluid[C],” in SPE International Symposium on Oilfield Chemistry, San Antonio, Texas, 14-17 February.
Niu Yabin, Zhang Daming, Xiao Hongzhang, Zheng Wumuxi, and Li Jian (1998). “Research and Application of Metal Complex - Amphoteric Polymer Drilling Fluid[C],” in SPE International Oil and Gas Conference and Exhibition in China, Beijing, China, 2-6 November.
Nunoo, N. A. (2018). Guest Editorial: How Artificial Intelligence Will Benefit Drilling. J. Petroleum Technol. 70 (5), 14–15. doi:10.2118/0518-0014-jpt
Oseh, J. O., Norddin, M. N. A. M., Ismail, I., Gbadamosi, A. O., and Agi, A. (2020). Effect of the surface charge of entrapped polypropylene at nanosilica-composite on cuttings transport capacity of water-based muds. Appl. Nanosci. 10, 61–82. doi:10.1007/s13204-019-01063-9
Panda, A., Varshney, P., Mohapatra, S. S., and Kumar, A. (2017). Development of liquid repellent coating on cotton fabric by simple binary silanization with excellent self-cleaning and oil-water separation properties. Carbohydr. Polym. 181, 1052–1060. doi:10.1016/j.carbpol.2017.11.044
Parizad, A., Shahbazi, K., and Tanha, A. A. (2018). SiO2 Nanoparticle and KCl Salt Effects on Filtration and Thixotropical Behavior of Polymeric Water Based Drilling Fluid: With Zeta Potential and Size Analysis[J]. Results Phys. 9, 1656–1665. doi:10.1016/j.rinp.2018.04.037
Patel, A., Stamatakis, E., Young, S., et al. (2007). “Advances in inhibitive water based drilling fluids-Can they replace oil-based muds?[C],” in International Symposium on Oilfield Chemistry, Houston, Texas, 28 February-2 March.
Paul, Balding, Li, Mei-chun, Wu, Qinglin, Volkovinsky, R., and Russo, P. (2020). Cellulose Nanocrystal–Polyelectrolyte Hybrids for Bentonite Water-Based Drilling Fluids. ACS Appl. Bio Mat. 3 (5), 3015–3027. doi:10.1021/acsabm.0c00071
Payam, A., Ergun, K., and Mahmut, P. (2016). Experimental investigation of solids transport in horizontal concentric annuli using water and drag reducing polymer-based fluids. J. Nat. Gas Sci. Eng. 35, 1070–1078. doi:10.1016/j.jngse.2016.09.052
Peng, S., Jiang, G., Li, X., Yang, L., Liu, F., and He, Y. (2017). Flocculation of submicron particles in water-based drilling fluids by CMC-g-DMDAAC. J. Petroleum Sci. Eng. 162, 55–62. doi:10.1016/j.petrol.2017.12.036
Qiu, Zhengsong, Xu, Jiafang, Lv, Kaihe, et al. (2007). A Multivariate cooperation principle for well-bore stabilization[J]. Acta Pet. Sin. 28 (2), 117–119.
Qu, Yuanzhi, Lai, Xiaoqing, Zou, Laifang, and Su, Y. (2009). Polyoxyalkyleneamine as shale inhibitor in water-based drilling fluids. Appl. Clay Sci. 44 (3-4), 265–268. doi:10.1016/j.clay.2009.03.003
Ramirez, M. A., Clapper, D. K., Sanchez, G., et al. (2005). “Aluminum-based HPWBM successfully replaces oil-based mud to drill exploratory wells in an environmentally sensitive area[C],” in SPE Latin American and Caribbean Petroleum Engineering Conference, Rio de Janeiro, Brazil, 20-23 June.
Ramirez, M. A., Moura, E., Luna, E., Aragao, A., and Taira, H. (2007). “HPWBM as a Technical Alternative To Drill Challenging Wells Project: Lessons Learned in Deepwater Brazil[C],” in Latin American & Caribbean Petroleum Engineering Conference, Buenos Aires, Argentina, 15-18 April.
Rana, A., Khan, I., Ali, S., Saleh, T. A., and Khan, S. A. (2020). Controlling Shale Swelling and Fluid Loss Properties of Water-Based Drilling Mud via Ultrasonic Impregnated SWCNTs/PVP Nanocomposites. Energy fuels. 34 (8), 9515–9523. doi:10.1021/acs.energyfuels.0c01718
Ranka, M., Brown, P., and Hatton, T. A. (2015). Responsive Stabilization of Nanoparticles for Extreme Salinity and High-Temperature Reservoir Applications. ACS Appl. Mat. Interfaces 7 (35), 19651–19658. doi:10.1021/acsami.5b04200
Retz, R. H., Friedheim, J., Lee, L. J., and Welch, O.O. (1991). “An Environmentally Acceptable and Field-Practical, Cationic Polymer Mud System[C],” in Offshore Europe, Aberdeen, United Kingdom, 3-6 September.
Riley, M., Young, S., Stamatakis, E.et al. (2012). “Wellbore Stability in Unconventional Shales-The Design of a Nano-Particle Fluid[C],” in SPE Oil and Gas India Conference and Exhibition, Mumbai, India, 28-30 March.
Rosa, R. S., Rosa, A., and Farias, S. B. (2005). “A New Inhibitive Water-Based Fluid: A Completely Cationic System[C],” in SPE Latin American and Caribbean Petroleum Engineering Conference, Rio de Janeiro, Brazil, 20-23 June.
Sachsenmeier, P. (2016). Industry 5.0—The Relevance and Implications of Bionics and Synthetic Biology. Engineering 2 (2), 225–229. doi:10.1016/j.eng.2016.02.015
Saikia, Tinku, and Mahto, V. (2016). Experimental investigations of clathrate hydrate inhibition in water based drilling fluid using green inhibitor. J. Petroleum Sci. Eng. 147, 647–653. doi:10.1016/j.petrol.2016.09.022
Saikia, T., and Mahto, V. (2016). Evaluation of 1-Decyl-3-Methylimidazolium Tetrafluoroborate as clathrate hydrate crystal inhibitor in drilling fluid[J]. J. Nat. Gas Sci. Eng. 36, 906–915.
Schlumberger Service (2021). Water-base drilling fluid systems[EB/OL]. Available at https://www.slb.com/resource-library/case-study/mi/hydraglyde.
Sepehri, S., Soleyman, R., Varamesh, A., Valizadeh, M., and Nasiri, A. (2018). Effect of synthetic water-soluble polymers on the properties of the heavy water-based drilling fluid at high pressure-high temperature (HPHT) conditions. J. Petroleum Sci. Eng. 166, 850–856. doi:10.1016/j.petrol.2018.03.055
Shaikh, S. M. R., Nasser, M. S., Magzoub, M., Benamor, A., Hussein, I. A., El-Naas, M. H., et al. (2018). Effect of electrolytes on electrokinetics and flocculation behavior of bentonite-polyacrylamide dispersions. Appl. Clay Sci. 158, 46–54. doi:10.1016/j.clay.2018.03.017
Shan, Wenjun, Ma, Jingyuan, Jiang, Guancheng, and Sun, J. (2022). An Inverse Emulsion Polymer as a Highly Effective Salt- and Calcium-Resistant Fluid Loss Reducer in Water-Based Drilling Fluids. ACS Omega 7 (18), 16141–16151. doi:10.1021/acsomega.2c01476
Sharma, S. K., and Kachari, J. (2010). “Use of KCl-Polymer Clouding Out Polyol Drilling Fluid in combating high pressure in deep exploratory wells of Assam Field: A case study [C],” in SPE Oil and Gas India Conference and Exhibition, Mumbai, India, 20-22 January.
Sheen, Y., Huang, Y., Liao, C., Chou, H. Y., and Chang, F. C. (2010). New approach to fabricate an extremely super-amphiphobic surface based on fluorinated silica nanoparticles. J. Polym. Sci. B. Polym. Phys. 46 (18), 1984–1990. doi:10.1002/polb.21535
Steiger, R. P. (1982). Fundamentals and Use of Potassium/Polymer Drilling Fluids To Minimize Drilling and Completion Problems Associated With Hydratable Clays. J. Petroleum Technol. 34 (8), 1661–1670. doi:10.2118/10100-pa
Stolte, C. J., Wu, C. S., Carroll, D. C., et al. (2012). “The Path from Vertical to Horizontal Shale Gas Wells[C],” in IADC/SPE Asia Pacific Drilling Technology Conference and Exhibition, Tianjin, China, 9-11 July.
Sun, J., Zhang, X., Lv, K., Liu, J., Xiu, Z., Wang, Z., et al. (2022). Synthesis of hydrophobic associative polymers to improve the rheological and filtration performance of drilling fluids under high temperature and high salinity conditions. J. Petroleum Sci. Eng. 209, 109808. doi:10.1016/j.petrol.2021.109808
Swaminathan Ponmanl, R. Nagarajan, Jitendra, S. Sangwai, and Sangwai, J. S. (2016). Effect of Nanofluids of CuO and ZnO in Polyethylene Glycol and Polyvinylpyrrolidone on the Thermal, Electrical, and Filtration-Loss Properties of Water-Based Drilling Fluids. SPE J. 21 (2), 405–415. doi:10.2118/178919-pa
Vahdati, M., Cedano-Serrano,, , Francisco, J., Creton, C., et al. (2020). Coacervate-based underwater adhesives in physiological conditions[J]. ACS Appl. Mater. Interfaces 2 (8), 3397–3410.
Verma, Amit, Chauhan, Geetanjali, Ojha, Keka, and Padmanabhan, E. (2019). Characterization of Nano-Fe2O3-Stabilized Polymer-Free Foam Fracturing Fluids for Unconventional Gas Reservoirs[J]. Energy fuels. 33 (11), 10570–10582. doi:10.1021/acs.energyfuels.9b02195
Wakamatsu, H., and Aruga, K. (2013). The impact of the shale gas revolution on the U.S. and Japanese natural gas markets. Energy Policy 62, 1002–1009. doi:10.1016/j.enpol.2013.07.122
Walker, S., and Li, J. (2000). “The Effects of Particle Size, Fluid Rheology, and Pipe Eccentricity on Cuttings Transport [C],” in SPE/ICoTA Coiled Tubing Roundtable, Houston, Texas, 5-6 April.
Wang, K., Jiang, G., Liu, F., Yang, L., Ni, X., and Wang, J. (2018). Magnesium aluminum silicate nanoparticles as a high-performance rheological modifier in water-based drilling fluids. Appl. Clay Sci. 161, 427–435. doi:10.1016/j.clay.2018.05.012
Wang, Qiang, Chen, Xi, Jha, Awadhesh N., and Rogers, H. (2014). Natural gas from shale formation – The evolution, evidences and challenges of shale gas revolution in United States. Renew. Sustain. Energy Rev. 30, 1–28. doi:10.1016/j.rser.2013.08.065
Wang, Zhiyuan, Yang, Li, Zhang, Yingchun, et al. (2020). Productivity evaluation for long horizontal well test in deep water faulted sandstone reservoir[J]. Geofluids.
Warren, B., van der Horst, P., and Stewart, W. (2003). “Application of Amphoteric Cellulose Ethers in Drilling Fluids[C],” in International Symposium on Oilfield Chemistry, Houston, Texas, 5-7 February.
Wenjun, S., and Haiyan, D. (2014). Development and Application of Anti-collapse & Anti-drag Agent for Drilling Fluid. Procedia Eng. 73, 55–62. doi:10.1016/j.proeng.2014.06.170
Witthayapanyanon A., Leleux J., Vuillemet J.et al. (2013). “High performance water-based drilling fluids-An environmentally friendly fluid system achieving superior shale stabilization while meeting discharge requirement offshore Cameroon[C],” in SPE/IADC Drilling Conference, Amsterdam, The Netherlands, 5-7 March.
Xianmin Z., Guancheng J., Tengfei D.et al. (2018). An amphoteric polymer as a shale borehole stabilizer in water-based drilling fluids[J]. J. Petroleum Sci. Eng. 170, 112–120.
Xie, B., and Liu, X. (2017). Thermo-thickening behavior of LCST-based copolymer viscosifier for water-based drilling fluids. J. Petroleum Sci. Eng. 154, 244–251. doi:10.1016/j.petrol.2017.04.037
Xie, B., Ting, L., Zhang, Y., and Liu, C. (2018). Rheological properties of bentonite-free water-based drilling fluids with novel polymer viscosifier. J. Petroleum Sci. Eng. 164, 302–310. doi:10.1016/j.petrol.2018.01.074
Xie, Binqiang, Zhang, Xianbin, Li, Yagang, Liu, W., and Luo, M. (2019). Application a novel thermo-sensitive copolymer as a potential rheological modifier for deepwater water-based drilling fluids. Colloids Surfaces A Physicochem. Eng. Aspects 581, 123848. doi:10.1016/j.colsurfa.2019.123848
Xiong, Zhengqiang, Tao, S., Li, X., Fu, F., and Li, Y. (2016). Preparation of fluidized catalytic cracking slurry oil-in-water emulsion as anti-collapse agent for drilling fluids. Petroleum 2 (4), 361–368. doi:10.1016/j.petlm.2016.08.009
Xuan, Yang., Jiang, Guancheng, Li, Yingying, Yang, L., and Zhang, X. (2015). Biodegradable oligo (poly-l-lysine) as a high-performance hydration inhibitor for shale. RSC Adv. 5, 84947–84958. doi:10.1039/c5ra16003k
Xuan, Y., Jiang, G., Li, Y., Geng, H., and Wang, J. (2013). A biomimetic drilling fluid for wellbore strengthening[J]. Petroleum Explor. Dev. 40 (4), 497–501.
Yadav, P., Kosandar, B. A., Jadhav, P. B.et al. (2015). “Customized high-performance water-based mud for unconventional reservoir drilling[C],” in SPE Middle East Oil & Gas Show and Conference, Manama, Bahrain, 8-11 March.
Yan, Y. L., Cui, M. Y., Jiang, W. D., He, A. L., and Liang, C. (2017). Drag reduction in reservoir rock surface: Hydrophobic modification by SiO2 nanofluids. Appl. Surf. Sci. 396, 1556–1561. doi:10.1016/j.apsusc.2016.11.209
Yang, J., Sun, J., Wang, R., Liu, F., Qu, Y.et al. (2022). Laponite-polymer composite as a rheology modifier and filtration loss reducer for water-based drilling fluids at high temperature. Colloids Surfaces A Physicochem. Eng. Aspects 655, 130261. doi:10.1016/j.colsurfa.2022.130261
Yang, L., Jiang, G., Shi, Y., and Yang, X. (2017). Application of Ionic Liquid and Polymeric Ionic Liquid as Shale Hydration Inhibitors. Energy fuels. 31 (4), 4308–4317. doi:10.1021/acs.energyfuels.7b00272
Yang, L., Wang, T., Yang, X., Jiang, G., Luckham, P. F., Xu, J., et al. (2019). Highly Stabilized Foam by Adding Amphiphilic Janus Particles for Drilling a High-Temperature and High-Calcium Geothermal Well. Ind. Eng. Chem. Res. 58 (23), 9795–9805. doi:10.1021/acs.iecr.9b01714
Yang, L., Yang, X., Wang, T., Jiang, G., Luckham, P. F., Li, X., et al. (2019). Effect of Alkyl Chain Length on Shale Hydration Inhibitive Performance of Vinylimidazolium-Based Ionic Liquids. Ind. Eng. Chem. Res. 58 (20), 8565–8577. doi:10.1021/acs.iecr.9b01016
Yang, Xianyu, Shang, Zhenxiao, Liu, Hengwei, Cai, J., and Jiang, G. (2017). Environmental-friendly salt water mud with nano-SiO2 in horizontal drilling for shale gas[J]. J. Petroleum Sci. Eng. 156, 408–418. doi:10.1016/j.petrol.2017.06.022
Yang, Xuan, Jiang, Guancheng, Li, Yingying, Wang, J., and Geng, H. (2013). Inhibiting effect of dopamine adsorption and polymerization on hydrated swelling of montmorillonite. Colloids Surfaces A Physicochem. Eng. Aspects 422, 50–60. doi:10.1016/j.colsurfa.2013.01.038
Yew, C. H., and Liu, G. (1992). “Pore Fluid and Wellbore Stabilities [C],” in International Meeting on Petroleum Engineering, 24-27 March.
Zeynali, M. E. (2012). Mechanical and physico-chemical aspects of wellbore stability during drilling operations. J. Petroleum Sci. Eng. 82-83, 120–124. doi:10.1016/j.petrol.2012.01.006
Zhang, Hongtao, Zhang, Haiqi, Zhu, Youhai, Liu, W., Yang, M., Li, Y., et al. (2014). The status of natural gas hydrate research in China: A review. Renew. Sustain. Energy Rev. 31, 778–791. doi:10.1016/j.rser.2013.12.025
Zhang, W., Chen, S., Hu, W., Zhou, B., Yang, Z., Yin, N.et al. (2011). Facile fabrication of flexible magnetic nanohybrid membrane with amphiphobic surface based on bacterial cellulose. Carbohydr. Polym. 86 (4), 1760–1767. doi:10.1016/j.carbpol.2011.07.015
Zhang, X., Jiang, G., Xuan, Y., Wang, L., and Huang, X. (2017). Associating Copolymer Acrylamide/Diallyldimethylammonium Chloride/Butyl Acrylate/2-Acrylamido-2-methylpropanesulfonic Acid as a Tackifier in Clay-Free and Water-Based Drilling Fluids. Energy fuels. 31 (5), 4655–4662. doi:10.1021/acs.energyfuels.6b02599
Zhang, Yan, Xiang, Xingjin, Yan, Jienian, et al. (2011). Low Free Water Drilling Fluid System[J]. Petroleum Explor. Dev. 38 (4), 27–28.
Zhao, Z., Sun, J., Liu, F., Bai, Y., and Wang, R. (2022). A laboratory study of self-healing hydrophobic association gels used as lost circulation material. Colloids Surfaces A Physicochem. Eng. Aspects 646, 128964. doi:10.1016/j.colsurfa.2022.128964
Zheng, Lihui, Chen, Biwu, Zhang, Zheng, Tang, J., and Sun, H. (2016). Anti-collapse mechanism of CBM fuzzy-ball drilling fluid. Nat. Gas. Ind. B 3 (2), 152–157. doi:10.1016/j.ngib.2016.03.011
Keywords: water-based drilling fluid, polymer drilling fluid, polymer additive, wellbore-strengthening, amphiphobicity
Citation: He Y, Jiang G, Dong T, Wang G, He J, Dou H and Du M (2023) Research progress and development tendency of polymer drilling fluid technology for unconventional gas drilling. Front. Energy Res. 10:1058412. doi: 10.3389/fenrg.2022.1058412
Received: 30 September 2022; Accepted: 05 December 2022;
Published: 23 January 2023.
Edited by:
Wai Li, University of Western Australia, AustraliaReviewed by:
Guang Zhao, China University of Petroleum, ChinaJinze Xu, University of Calgary, Canada
Copyright © 2023 He, Jiang, Dong, Wang, He, Dou and Du. This is an open-access article distributed under the terms of the Creative Commons Attribution License (CC BY). The use, distribution or reproduction in other forums is permitted, provided the original author(s) and the copyright owner(s) are credited and that the original publication in this journal is cited, in accordance with accepted academic practice. No use, distribution or reproduction is permitted which does not comply with these terms.
*Correspondence: Guancheng Jiang, bTE1NjAwMjYzMTAwXzFAMTYzLmNvbQ==