- 1Shaoxing Power Supply Company, State Grid Zhejiang Electric Power Co., Ltd, Shaoxing, China
- 2College of Electrical and Information Engineering, Hunan University, Changsha, China
This paper proposes an economic benefit evaluation model of distributed energy storage system considering multi-type custom power services. Firstly, based on the four-quadrant operation characteristics of the energy storage converter, the control methods and revenue models of distributed energy storage system to provide reactive power compensation, new energy consumption, peak-valley arbitrage and other customized power services are analyzed. Secondly, an economic benefit evaluation model of custom power services is formulated, considering the life cycle degradation cost, investment payback period, net present value, and internal return rate of energy storage. Finally, demonstrate the effectiveness and superior performance of the proposed methodology through comparative studies on the economic benefits and practical value. The influence of reserve capacity ratio of energy storage converter, additional price for power quality management, peak-valley price difference, battery cost and project cycle on the annual return and internal rate of return is revealed through the sensitivity analysis, which provides the decision-making reference for battery selection and capacity allocation of distributed energy storage system participating in various custom power services.
1 Introduction
Generally, the custom power utilizes the advanced power electronics technology and control theory to provide power supply services that are higher than the national power supply standards for different users with specific demands and diverse requirements for power quality (Tao et al., 2014). Providing custom power services with diverse power quality can flexibly meet the demands of users and improve the economic operation of the power grid (Brenna et al., 2009; Liang et al., 2022). At present, the custom power services for power quality control including voltage sag, harmonics, and voltage deviation, are mostly using the specific power quality control equipment, such as dynamic voltage restorer (Bae et al., 2010; Kumar and Tyagi, 2021), active power filter (Azhagesan et al., 2021), unified power quality regulator (Krishna et al., 2022), and static VAR compensator (Zaidan and Toos, 2021). The energy storage converter has the four-quadrant high-efficiency operation characteristics of bidirectional active and reactive power regulation. In addition to the conventional application scenarios such as renewable energy consumption (Murty and Kumar, 2020) and peak-valley arbitrage (Das et al., 2018), the energy storage converter has significant economic and technical advantages in power quality control including the voltage deviation and voltage sag as well (Alshehri and Khalid, 2019; Guo et al., 2019; LI et al., 2019; Liu et al., 2021; Akdogan and Ahmed, 2022). Brenna et al. (2009), LI et al. (2019), and Akdogan and Ahmed (2022) reviewed the research status and development trend of energy storage system for solving steady-state and dynamic power quality problems of power grid, and analyzed the feasibility of energy storage to solve the voltage deviation, harmonic and three-phase unbalance problems. Liu et al. (2021) proposed a day-ahead optimal scheduling model for integrated energy systems considering the potential economic benefits of energy storage, which can promote the active participation of energy storage in energy market scheduling for improving its profits. Guo et al. (2019) proposed a voltage coordinated control method for the high renewable penetrated distribution network based on the model predictive control, which can improve the voltage deviation of power grid through the four-quadrant regulation ability of active and reactive power of energy storage. Alshehri and Khalid (2019) proposed an optimal active and reactive power decoupling control method for the battery energy storage system to enhance the anti-disturbance ability of distribution network, while the improvement of power quality was not taken into account. Therefore, the energy storage system possesses unique technical advantages in solving the power quality problems, laying a solid theoretical foundation for providing various custom power services for energy storage.
In this paper, an economic benefit evaluation model of distributed energy storage system considering the custom power services is proposed to elevate the economic performance of distributed energy storage system on the commercial application and satisfying manifold custom power demands of different users. The main contributions of this paper are as follows:
1) A revenue model of distributed energy storage system is proposed to provide reactive power compensation, renewable energy consumption and peak-valley arbitrage services. An additional electricity pricing model of distributed energy storage system to provide reactive power compensation for users is formulated. The problems of long investment return period and insufficient utilization caused by the single profit mode of distributed energy storage system are solved.
2) A custom power services economic benefit evaluation model considering the life cycle cost, investment payback period, net present value and internal return rate of energy storage is formed. The influence of reserve capacity ratio of energy storage converter, additional price for power quality management and project cycle on annual return and internal rate of return is revealed by sensitivity analysis, which provides a decision-making basis for battery selection and capacity allocation of distributed energy storage system so as to participate in custom power services.
2 Revenue model of custom power services
A revenue model for distributed energy storage system to provide custom power services such as power quality management, peak-valley arbitrage, and renewable energy consumption is formulated in this section.
2.1 Power quality management
Since the energy storage converter has the fast power regulation characteristics of active and reactive power four-quadrant operation, making full use of its available reserve capacity to solve the power quality problem of distribution network has great economic benefits and regulation effects. The energy storage converter can implement the voltage deviation and reactive power compensation control of high renewable penetrated distribution network through the PQ control and droop control of active and reactive power coordination (Alshehri and Khalid, 2019). In addition, through the use of series-parallel energy system (LI et al., 2019), the series side can be similar to the dynamic voltage restorer (DVR) operation, and the parallel side can support reactive power adjustment to achieve the function of voltage deviation governance.
Revenues of energy storage for power quality management are from reactive power compensation service charge. Power quality management service charge is acquired by charging additional price for willingness to control according to unit power (Yang et al., 2006; Li et al., 2022; Lu et al., 2022), which is obtained through willingness price consultation with the users. Therefore, the annual revenue of energy storage participating in power quality management
where
2.2 Distributed renewable energy consumption
With the steady growth of the proportion of distributed rooftop photovoltaic installation in the distribution network, the net load of the system will fluctuate rapidly in a short period of time due to the volatility and intermittency of photovoltaic output and anti-peak shaving characteristics of photovoltaic output and system load. At the same time, the renewable energy consumption ability of the distribution network is limited by the transmission capacity, resulting in renewable generation curtailment. Distributed energy storage system can separate power generation and consumption in time and space dimensions. It stores the surplus energy when the renewable energy generation exceeds the load, and releases the stored energy when the renewable energy generation is insufficient, improving the ability of renewable energy accommodation.
The distributed photovoltaic power releasing at the user side generally takes place when the sunlight is sufficient at noon. The energy storage system purchases surplus energy at on-grid price and obtains the corresponding income by selling at the peak price. Therefore, the annual revenue of energy storage participating in distributed renewable energy consumption
where
2.3 Peak-valley arbitrage
The peak-valley arbitrage is the main profit mode of distributed energy storage system at the user side (Zhao et al., 2022). The peak-valley price ratio adopted in domestic and foreign time-of-use electricity price is mostly 3–6 times, and even reach 8–10 times in emergency cases. It is generally believed that when the peak-valley price difference transcends 0.7 CNY/kWh, the energy storage will have the peak-valley arbitrage profit space (Li and Li, 2022). Usually, the energy storage is charged at night when the price is at valley stage, and discharges during the daytime when the power consumption is at peak, so as to achieve peak-valley arbitrage and save cost. Taking the charging/discharging strategy of the general industrial and commercial energy storage as an example, the annual revenue of energy storage participating in peak-valley arbitrage
where
3 Economic benefit evaluation
The economic benefit of distributed energy storage system to provide custom power services considering the cost of energy storage is analyzed and evaluated in this section.
3.1 Life cycle cost model of energy storage
The life cycle cost of energy storage is composed of initial investment cost, operation and maintenance cost, replacement cost, and recovery value. The initial investment cost contains the cost of energy storage battery
where
The annual operation and maintenance cost of energy storage
where
The replacement cost refers to the cost that the energy storage equipment is required to be replaced during the operation of the project. In reality, since the energy storage converters and auxiliary facilities can always satisfy the rated operation life, the replacement cost here refers to the replacement cost of the battery
where
Considering the time value of funds, the replacement cost in project cycle
where
The recovery value of energy storage
Considering the time value of funds, the recovery value of battery
In summary, the annual cost of energy storage
where
3.2 Economic evaluation model
The economic benefit evaluation for energy storage is an important part to investigate the feasibility of the project, which offers an essential basis for the scientific decision-making in the early stage of project implementation and provides the technical support for distributed energy storage system project investment. This paper establishes a comprehensive economic benefit evaluation model of custom power services considering the net present value (NPV) of energy storage, investment recovery period and internal rate of return (IRR). Considering the average annual cost of energy storage and the annual revenue of custom power services, the economic benefit evaluation model can be formulated as follows:
where F is the annual profit of energy storage. The value of F is greater than 0 indicating that the investment can be recovered during the operation of energy storage project.
NPV is one of the methods to evaluate the feasibility of investment scheme, which refers to the difference between the discounted cash flow and the initial investment amount brought by project investment, as shown in Eq. 12,
where
Payback period refers to the time required to recover the total investment cost in the project life cycle. Considering the time value of funds, this paper selects the rate of dynamic investment return
IRR refers to the discount rate when the net cash flow accumulation is equal to zero during the project operation period, reflecting the rate of return that the project desires to achieve as shown in Eq. 14. The project is feasible/infeasible if the value of IRR is greater/less than the actual discount rate.
4 Case analysis
4.1 Boundary condition
Adopting an energy storage system with an installed capacity of 500 kW/1,000 kWh built in 10 kV large industrial consumers in east China as a case, the energy storage operators and users share the economic benefits from renewable energy accommodation and peak-valley arbitrage according to the ratio of 8:2. On the basis of the local annual average electricity prices, the valley and peak prices are set to 0.2901 CNY/kWh and 1.0957 CNY/kWh, respectively. The renewable energy on-grid price is 0.4153 CNY/kWh, and the additional electricity charge for reactive power compensation is 0.003 CNY/kWh. The daily renewable energy consumption is 200 kWh and the annual operation time is 350 days. Assuming the length of project cycle is 10 years, the discount rate is set as 8% considering the battery replacement cost, labor cost, maintenance cost, and insurance expense.
Considering the peak-to-valley time division of the time-of-use price, the charge and discharge rates of the battery, the cycle life of the battery and the load characteristics of the consumers, the energy storage system in this paper adopt the strategy of double charge and discharge mode. In this operation mode, the charging periods of the energy storage power station are from 10.00 p.m. to 8.00 a.m. and 11.00 a.m to 1.00 p.m, and the discharging periods are from 9.00 a.m. to 11.00 a.m. and 3.00 p.m. to 5.00 p.m. Note that 1.00 p.m. to 3.00 p.m. in January, July, August, and December are set to the peak discharge periods. During the period from 10.00 p.m. to 8.00 a.m., the charging time is relatively rich and the energy storage can be charged slowly. During the period from 11.00 a.m. to 1.00 p.m., in order to provide energy for the upcoming peak and peak of electricity consumption, it is necessary to charge energy storage with high power and absorb renewable energy during this period. During the period from 9.00 a.m. to 11.00 a.m., in order to give full play to the energy storage capacity, the energy storage can be discharged at high power. During the period from 1.00 p.m. to 5.00 p.m., the dischargeable response time is long, and thus the discharge power can be dynamically and flexibly adjusted.
Lead-carbon battery, sodium-sulfur battery, lithium iron battery and vanadium redox battery are selected as typical distributed energy storage system for research. The specific costs and technical performance parameters are shown in Table 1.
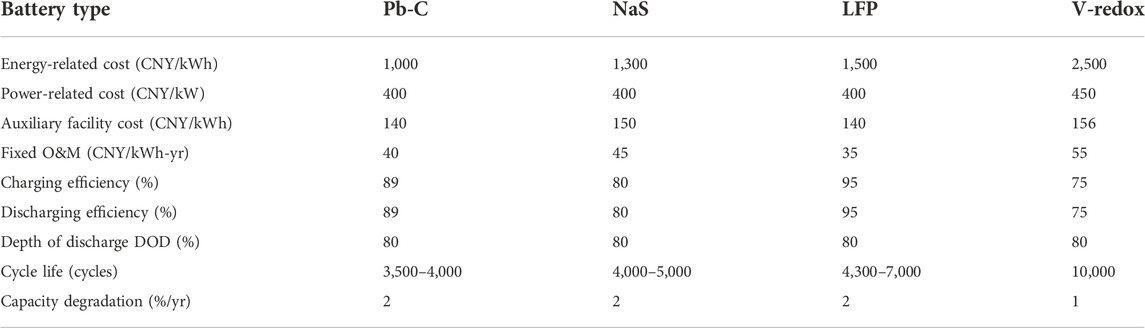
TABLE 1. Costs and technical performance parameters of four kinds of battery energy storage on the user side.
4.2 Comparative analysis
According to the model and parameter settings in Section 4.1, the economic benefits of four battery energy storage systems are calculated and compared. The total investment economic benefits are shown in Table 2.
The calculation results show that the initial investment cost of the lead-carbon battery is lower than that of the other three batteries, and it has the advantage of construction cost. However, its maximum charge and discharge time and battery life are low, and a battery replacement is needed in the project cycle. It can also be observed that the economic performance of lead-carbon battery is worse than that of lithium iron phosphate battery considering the cost of battery replacement. The NPV, IRR and investment payback period of lithium iron battery in the 10-year project cycle are 408.2 thousand CNY, 12.55% and 9.33 years, respectively. The single profit of lithium iron battery in various custom power scenarios is higher than that of the other three batteries due to the characteristics of long life, high safety and easy maintenance. Therefore, when the project cycle is 10 years long, it is of more reference value and engineering application value to use lithium iron battery as the energy storage to provide custom power services considering the economy and battery technical characteristics.
It can be found from Table 2 that the majority of the revenue from energy storage for custom power services comes from the peak-valley price differential arbitrage. The calculation results show that the power quality management, renewable energy photovoltaic consumption and peak-valley arbitrage account for 14.44%, 6.93% and 78.63%, respectively. In the above cases, the proportion of energy storage capacity required for power quality management is only 10%, but the benefits are considerable, indicating that energy storage provides power quality management services with good commercial prospects. Therefore, through the economic calculation of energy storage application in custom power services, it provides a new development direction for energy storage to explore new profit models and improve the income level of energy storage.
4.3 Sensitivity analysis
Figure 1A shows the relationship between the annual return and IRR of the four battery energy storages with the different proportion of reserve capacities. When other parameters remain unchanged, the sensitivity analysis is carried out by setting reserve capacity ratios of 0%, 5%, 10%, 15%, 20%, and 25%, respectively. With the increase of reserve capacity, the annual return and IRR of the four batteries show a trend of increase at the beginning and then decrease. When there is a certain reactive reserve capacity, the energy storage can provide reactive compensation service to obtain benefits. However, when the proportion of reserve capacity continues to increase, the increase of reactive power compensation income is not obvious and the active output of converter is limited, which reduces the income of peak-valley arbitrage and thus the overall income is decreased. Among them, the annual return of lead-carbon battery increases from 121.24 thousand CNY to 149.19 thousand CNY, reaching the maximum when the proportion of reserve capacity is 15%, and finally decreases to 147.61 thousand CNY. The IRR rises from 6.91% to 9.59%, and reaches its maximum at a reserve capacity ratio of 15% and finally falls to 9.44%.
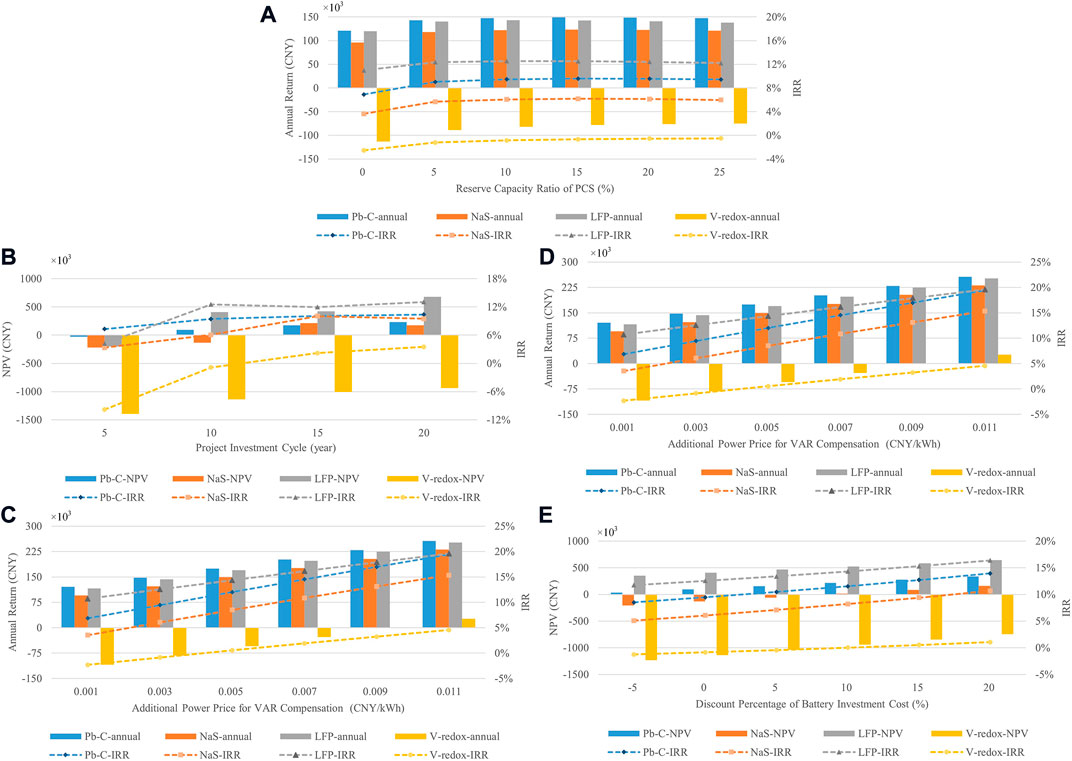
FIGURE 1. Annual return, NPV, and IRR with five sensitive factors: (A) reserve capacity ratio of PCS, (B) project investment cycle, (C) additional power price for VAR compensation, (D) peak-valley price ratio, and (E) discount percentage of battery investment cost.
Figure 1B is the relationship between NPV and IRR of four kinds of battery energy storage with project cycle. In the case of constant parameters, the project cycles are set as 5, 10, 15, and 20 years for sensitivity analysis. Four kinds of battery energy storage NPV and IRR show an increasing trend with the increase of project cycle. When the project cycle is 5 years, the lead-carbon battery has better economic performance than the sodium sulfur battery, lithium iron battery and vanadium redox battery because of its low cost and no replacement cost. When the project cycle is more than 10 years, the IRR of lithium iron battery exceeds 10% due to its good charge and discharge efficiency and cycle life, which is more than other types of batteries. Therefore, the lead-carbon battery can be used as the energy storage system when the project cycle is less than 5 years. Moreover, when the project cycle is more than 10 years, the use of lithium iron battery with the characteristics of safe and easy maintenance as the energy storage is better.
Figure 1C shows the relationship between the annual return and IRR of the four battery energy storages and the additional electricity price of power quality. In the case of constant parameters, the sensitivity analysis was carried out by setting the additional power charges of 0.001, 0.003, 0.005, 0.007, 0.009, and 0.011 CNY/kWh, respectively. The annual profit and IRR of four kinds of battery energy storage show a linear growth trend with the increase of power quality additional charge. Among them, the lead-carbon battery energy storage in the process of increasing the additional power cost of power quality from 0.001 CNY/kWh to 0.011 CNY/kWh, the annual income increases from 120.66 to 256.27 thousand CNY, and the IRR increases from 6.85% to 19.43%. Therefore, the increase of the additional electricity charge has a positive effect on the growth of energy storage income.
Figure 1D shows the relationship between the annual return and IRR of the four battery energy storages with the peak-valley price difference. At present, the peak-valley arbitrage of energy storage is mostly the peak-valley price arbitrage, and the peak price is about four times that of the valley price. In the case of constant parameters, the peak-valley price ratios are set to be 2, 4, 6, and 8 times for sensitivity analysis. With the increase of peak-valley price difference, the annual return and IRR of the four types of battery energy storages increase linearly. Among them, the peak-valley price difference of the lead-carbon battery energy storage increases from 2 times to 8 times, and its annual return and IRR rise from −54.13 to 627.65 thousand CNY and −11.40%–50.93%, respectively, indicating that the peak-valley price difference has a great impact on the energy storage income.
Figure 1E shows the relationship between the NPV and IRR of the four battery energy storages and the decrease of battery cost. In the case of constant parameters, the sensitivity analysis was carried out by setting the battery cost reduction percentages of −5%, 0%, 5%, 10%, 15%, and 20%, respectively. The investment returns of four kinds of battery energy storage increase linearly with the decrease of battery cost. When the battery cost decreases from −5% to 20%, the cumulative NPV of lead-carbon battery energy storage increases from 34.46 to 334.70 thousand CNY, and the IRR increases from 8.51% to 13.95%.
5 Conclusion
In this paper, the economic benefit evaluation model of distributed energy storage system considering custom power services is established. Through the assessment and sensitivity analysis of multi-type distributed energy storage system including the lead-carbon battery, sodium sulfur battery, lithium iron battery and vanadium redox battery, the main conclusions are summarized as follows:
(1) The energy storage converter has the fast power regulation characteristics of active/reactive four-quadrant operation. Participation in reactive power compensation, renewable energy consumption and peak-valley arbitrage can bring great economic benefits to the energy storage project, which provides a novel idea for the transformation of the business model of distributed energy storage system from the price difference-based to comprehensive profit, and is conducive to promoting the commercial promotion and diversified development of distributed energy storage system on the user side.
(2) The economy of distributed energy storage system participating in the custom power services increases at the beginning and then decreases with the increase of the reserve capacities. When there is a certain reactive reserve capacity, the energy storage can benefit by providing reactive power compensation service. With the increase of the reserve capacity, the maximum active power output of energy storage converter decreases, and the benefits of peak-valley arbitrage and renewable energy consumption decrease gradually. However, the increase of reactive power compensation benefits is not obvious, which reduces the overall economy of energy storage. Through sensitivity analysis, it is found that the economic performance is optimal when the proportion of converter reserve capacity is 15%.
(3) The economic benefits of energy storage systems are greatly affected by the length of project cycle. When the project cycle is shorter than 5 years, lead-carbon battery has better economic performance than sodium sulfur battery, lithium iron battery and vanadium redox battery because of its cost advantage. When the project cycle is longer than 10 years, lithium iron phosphate battery performs better in economic benefits due to its charge-discharge efficiency and cycle life advantages. With the development of energy storage material technology in the future, the price cost of lithium battery will gradually decrease, and its cost performance will show obvious economic advantages and market demand.
(4) Energy storage income is greatly affected by market mechanism. The energy storage economy increases linearly with the increase of peak-valley price difference and high-quality electricity additional price. Besides, the change of market mechanisms such as peak-valley price pricing mechanism will affect the energy storage benefits. Under the market-oriented electricity sale mode, the price fluctuates up and down according to the market supply and demand, providing a wide profit space for the peak-valley arbitrage of energy storage. The establishment of auxiliary service market can provide a broad space for the development of energy storage to offer power quality services. The increase of users’ demands for power quality will increase the service cost of reactive power compensation and provide profitable space for the power quality management of energy storage.
Data availability statement
The original contributions presented in the study are included in the article/Supplementary Material, further inquiries can be directed to the corresponding author.
Author contributions
Writing the original draft and editing, JF; Conceptualization, ZGP and TC; Formal analysis, ZHP and SK; Visualization and contributed to the discussion of the topic, JC and SH.
Conflict of interest
Authors JF, ZGP, TC, SK and JC were employed by Shaoxing Power Supply Company State Grid Zhejiang Electric Power Co., Ltd.
The remaining authors declare that the research was conducted in the absence of any commercial or financial relationships that could be construed as a potential conflict of interest.
Publisher’s note
All claims expressed in this article are solely those of the authors and do not necessarily represent those of their affiliated organizations, or those of the publisher, the editors and the reviewers. Any product that may be evaluated in this article, or claim that may be made by its manufacturer, is not guaranteed or endorsed by the publisher.
References
Akdogan, M. E., and Ahmed, S. (2022). Novel compensation methods using energy storage system (ESS) in islanded unbalanced single-/three-phase multi-microgrids. IEEE Trans. Ind. Appl. 58 (4), 5457–5468. doi:10.1109/TIA.2022.3175763
Alshehri, E. J., and Khalid, M. (2019). Power quality improvement in microgrids under critical disturbances using an intelligent decoupled control strategy based on battery energy storage system. IEEE Access 7, 147314–147326. doi:10.1109/ACCESS.2019.2946265
Azhagesan, D., Periyasamy, M., Manickavasagam Parvathy, S., Sridharan, M., and Baladhandautham, C. B. (2021). Predictive current control of FL-shunt active power filter for dynamic and heterogeneous load compensation. Electr. Eng. 103, 2147–2160. doi:10.1007/s00202-021-01224-6
Bae, B., Jeong, J., Lee, J., and Han, B. (2010). Novel sag detection method for line-interactive dynamic voltage restorer. IEEE Trans. Power Deliv. 25 (2), 1210–1211. doi:10.1109/TPWRD.2009.2037520
Brenna, M., Faranda, R., and Tironi, E. (2009). A new proposal for power quality and custom power improvement: Open upqc. IEEE Trans. Power Deliv. 24 (4), 2107–2116. doi:10.1109/TPWRD.2009.2028791
Das, C. K., Bass, O., Kothapalli, G., Mahmoud, T. S., and Habibi, D. (2018). Overview of energy storage systems in distribution networks: Placement, sizing, operation, and power quality. Renew. Sustain. Energy Rev. 91, 1205–1230. doi:10.1016/j.rser.2018.03.068
Guo, Y., Wu, Q., Gao, H., Chen, X., Østergaard, J., and Xin, H. (2019). MPC-based coordinated voltage regulation for distribution networks with distributed generation and energy storage system. IEEE Trans. Sustain. Energy 10 (4), 1731–1739. doi:10.1109/TSTE.2018.2869932
Krishna, D., Sasikala, M., and Kiranmayi, R. (2022). FOPI and FOFL controller based UPQC for mitigation of power quality problems in distribution power system. J. Electr. Eng. Technol. 17, 1543–1554. doi:10.1007/s42835-022-00996-6
Kumar, M., and Tyagi, B. (2021). A robust adaptive decentralized inverter voltage control approach for solar PV and storage-based islanded microgrid. IEEE Trans. Ind. Appl. 57 (5), 5356–5371. doi:10.1109/TIA.2021.3094453
Li, J., Xu, D., Wang, J., Zhou, B., Wang, M., and Zhu, L. (2022). “P2P multi-grade energy trading for heterogeneous distributed energy resources and flexible demand,” in IEEE Trans. Smart Grid (IEEE), 1. doi:10.1109/TSG.2022.3181703
Li, J., Yuan, X., and Yu, Z. L. G. E. (2019). Comments on power quality enhancement research for power grid by energy storage system. Automation Electr. Power Syst. 0 (8), 15–25. doi:10.7500/AEPS20180826002
Li, X., and Li, P. (2022). Analysis of economics and economic boundaries of large-scale Application of power batteries in cascade utilization. Energy Storage Sci. Technol. 11 (2), 717–725. doi:10.19799/j.cnki.20954239.2021.0487
Liang, Y., Ding, Z., Zhao, T., and Lee, W. -J. (2022). “Real-time operation management for battery swapping-charging system via multi-agent deep reinforcement learning,” in IEEE Trans. Smart Grid (IEEE), 1. doi:10.1109/TSG.2022.3186931
Liu, S., Zhou, C., Guo, H., Shi, Q., Song, T. E., Schomer, I., et al. (2021). Operational optimization of a building-level integrated energy system considering additional potential benefits of energy storage. Prot. Control Mod. Power Syst. 6, 4. doi:10.1186/s41601-021-00184-0
Lu, Y., Liang, Y., Ding, Z., Wu, Q., Ding, T., and Lee, W. -J. (2022). Deep reinforcement learning-based charging pricing for autonomous mobility-on-demand system. IEEE Trans. Smart Grid 13 (2), 1412–1426. doi:10.1109/TSG.2021.3131804
Murty, V. V. S. N., and Kumar, A. (2020). Retracted article: Multi-objective energy management in microgrids with hybrid energy sources and battery energy storage systems. Prot. Control Mod. Power Syst. 5 (1), 2–20. doi:10.1186/s41601-019-0147-z
Tao, S., Li, K., and Xu, Y. (2014). The coordination analysis on power supply and custom power devices for premium power park. Mod. Electr. Power 0 (6), 1–9. doi:10.19725/j.cnki.1007-2322.2014.06.001
Yang, J., Li, G., and Zhou, M. (2006). Power quality service pricing method based on differential pricing. Automation Electr. Power Syst. 30 (8), 41–46. doi:10.3321/j.issn:1000-1026.2006.08.009
Zaidan, M. R., and Toos, S. I. (2021). Optimal location of static var compensator to regulate voltage in power system. IETE J. Res. 1, 9. doi:10.1080/03772063.2021.1886877
Keywords: custom power services, power quality, peak-valley arbitrage, distributed energy storage system, economic benefit evaluation
Citation: Fang J, Pei Z, Chen T, Peng Z, Kong S, Chen J and Huang S (2023) Economic benefit evaluation model of distributed energy storage system considering custom power services. Front. Energy Res. 10:1029479. doi: 10.3389/fenrg.2022.1029479
Received: 27 August 2022; Accepted: 16 September 2022;
Published: 05 January 2023.
Edited by:
Liansong Xiong, Xi’an Jiaotong University, ChinaReviewed by:
Ning Li, Xi’an University of Technology, ChinaQin Wang, Electric Power Research Institute (EPRI), United States
Bo Yang, Kunming University of Science and Technology, China
Copyright © 2023 Fang, Pei, Chen, Peng, Kong, Chen and Huang. This is an open-access article distributed under the terms of the Creative Commons Attribution License (CC BY). The use, distribution or reproduction in other forums is permitted, provided the original author(s) and the copyright owner(s) are credited and that the original publication in this journal is cited, in accordance with accepted academic practice. No use, distribution or reproduction is permitted which does not comply with these terms.
*Correspondence: Zhihui Peng, MTY5NTQyOTQ2MUBxcS5jb20=