- Pukyong National University, Busan, South Korea
The facades of buildings provide significant potential for photovoltaic panels integration, allowing renewable energy deployment within the built environment. In literature, various options, such as building-integrated photovoltaics, building-integrated photovoltaics-thermal collectors, building-attached photovoltaics, and rooftop photovoltaics, have already been explored. However, this study aimed to develop a new solar photovoltaic collectors’ integration with vertical-green balconies in old high-rise buildings considering the façade reconstruction concepts mainly focusing on the water heating application. The objective of this study is to conduct a preliminary research study investigating such integration possibilities with old buildings considering the façade reconstruction concepts, followed by exploring various benefits. For this, an old high-rise building was rebuilt scientifically and rationally. PHOENICS tool was used to gauge and assess the building’s wind environment first, followed by the solar photovoltaic collector-based facade installation and preliminary assessment. The results include the system design, economic analysis of the solar photovoltaic collector’s application for water heating, calculation of the energy-saving rate, and functional analysis of the solar photovoltaic collectors combined with a vertical green balcony. The outcome of this study suggested that the process-specific rationalization plan can be applied in future urban architecture renovation.
1 Introduction
The International Energy Agency (IEA) estimates that 50% of the world’s population lives in cities, uses 73% of the world’s energy, and emits 70% of its CO2 emissions (Akram et al., 2021; IEA, 2021). The International Energy Outlook study also projects a 56% increase in global energy use between 2010 and 2040 (Akram et al., 2021). Additional forecasts show that the building industry will contribute significantly to overall energy usage when considering sectoral energy consumption assessments. Having said that, 50% of the population lives in urban areas, which is significantly increasing; more and more inner-city green spaces will need to be sacrificed to provide enough living space in urban centers in the future (Akram et al., 2021). As urbanization progresses, more and more high-rise residential structures are built; they typically have 10 to 15 stories. In this context, the quality of city life is affected by these places’ significant impact on the microclimate, which impacts the thermal comfort of cities and their related recreational functions (Shih et al., 2019; Yu et al., 2020; Xue et al., 2017; Liu and Russo, 2021; Puchol-Salort et al., 2020; Piselli et al., 2018). The option of creating green spaces in the city in locations that they previously believed impossible to create is provided by the vertical greening of buildings (Dunnett and Kingsbury, 2008). They provide significant potential for photovoltaic panels integration, allowing renewable energy deployment within the built environment. In literature, various options, such as building-integrated photovoltaics (BIPV), building-attached photovoltaics (BAPV), and rooftop photovoltaics (RTPV), have already been explored, but the most prominent option was BIPV. BIPV is one such popular way of integrating photovoltaics that enables both on-site green energy generation and vertical greening of buildings. Also, there is an increasing trend of BIPV adoption in the built environment to increase the proportion of renewable energy. The facades of buildings provide a significant amount of potential for BIPV deployment. Accurate external convective heat transfer coefficient modeling is necessary to evaluate BIPV facade performance. Moreover, a handful of studies (Ritzen et al., 2016; Yang and Zou, 2016; Goh et al., 2017; Prieto et al., 2017; Tabakovic et al., 2017) emphasize significant obstacles that prevent BIPV from being widely used. They vary from broad product concerns like functionality, aesthetics, and technological complexity (Prieto et al., 2017) to specialized regional concerns like the requirement for in-depth training at the professional and public levels (Ritzen et al., 2016; Goh et al., 2017; Tabakovic et al., 2017). The content of the current BIPV literature is summarized in Table 1, which highlights that there is only a limited amount of knowledge on customization as a possible driver for BIPV adoption. Building energy demand is influenced by several variables, some of which affect building energy usage. The terms “physical environmental elements” and “artificial designing parameters” are used to categorize these variables into two groups. While artificial designing parameters include building form factor and orientation, transparency ratio, optical and thermophysical properties of building material, and the distance between buildings, physical environmental factors include the amount of solar radiation, outdoor temperature, and wind speed, among others (Ekici and Aksoy, 2009).
In Table 2 design related summary, provides the benefits and drawbacks of each of the categories of photovoltaic integration for typical constructed example from literature is given.
More recently, application-specific design is becoming popular, and among the applications, water heating system is popular. Combining solar water heating technologies with building architecture involves two significant difficulties. One involves a solar water heating system consisting of a water pipeline, a water storage tank, and a solar collector (Ritzen et al., 2016; Prieto et al., 2017; Tabakovic et al., 2017). Evacuated tube Sun collectors are frequently used in buildings in China. However, Europe’s flat plate solar collectors are more typical (Shi et al., 2013). The flat plate collectors are better suited for pressure and secondary circulation systems, are easier to install, have a longer service life, and go better with building aesthetics, even if these two types of solar collectors each have their own technical benefits and limitations. The solar energy system’s performance is the subject of a lot of research. By conducting an experimental examination over a 1-year operating period, Zhai et al. (Wei et al., 2010) confirmed the real-world energy performance of a solar energy system capable of providing a hot water supply, natural ventilation, heating, and cooling in Shanghai. Building-integrated photovoltaic/thermal (BIPV/T) systems that use recovered heat for home heating were evaluated by Pantic et al. (Zhai et al., 2007) for their energy performance in three distinct open-loop air heating applications. Most vertical greening initiatives that have been put in place so far are showcases meant to create an impressive installation with a favorable reputation. The demand for vertical greening is growing, which is justified by the growing need for cities to be climate resilient and by the numerous benefits of green infrastructure in urban settings. This suggests that more and more cities are mandating the greening of buildings to some level, for instance, in the zoning plans.
Despite the greening mandates and various benefits of vertical greening that have been demonstrated, particularly in metropolitan settings, its use has thus far mostly been infrequent. On the one hand, many think installation, maintenance, and care are expensive for this type, but this could be dependent on many parameters (Hollands and Korjenic, 2021). So, the process of deciding on the greening system and choosing the photovoltaic application starts after the choice to design house greenery has been made. But so far, the available literature concerned it is mainly focused on facades and balconies are less explored for photovoltaics integration, nevertheless we see a great potential for photovoltaics integration with vertical green balconies by reconstructing facades in the buildings.
Therefore, this study aimed to develop a new photovoltaic panel’s integration with vertical-green balconies in old high-rise buildings considering the façade reconstruction concepts mainly focusing on the water heating application. The objective of this study is to conduct a preliminary research study investigating such integration possibilities with old buildings considering the façade reconstruction concepts, followed by exploring various benefits.
2 System design and installation
2.1 Proposed integrated design photovoltaics integration with vertical green balconies in high-rise building
The proposed design considers the photovoltaics integration in the vertical green balconies where existing facades are replaced with photovoltaics facades. This arrangement can be seen in Figure 1. Also, in Figure 1, the design plan adopted for façade reconstruction considering a case of schematic representation of high-rise building is shown. While designing the system, it is crucial to consider the ambient temperature, the wind speed, the aperture area of the solar collector, the solar radiation on the collector’s plane, and the amount of water in the water tank while designing a solar heat water system.
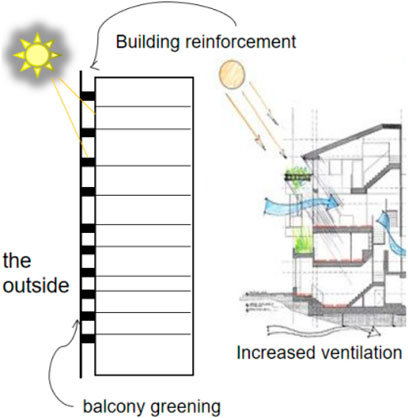
FIGURE 1. Concept design photovoltaics integration with vertical green balconies in high-rise building.
The total energy consumption by the solar water heating system is determined using Eq. 1
where, Qz is the total energy consumption of the solar water heating system in MJ; n is the total number of records; mzi is the hot water flow rate recorded in ith test in m3/s; ρw is the density of hot water in kg/m3; cw is the specific heat capacity of water in J/(kg,K); tdzi is the hot water temperature recorded in ith test in C; tbzi is the cold water temperature recorded in ith test in C; ΔTzi is the time step in s, in this case, ΔTzi = 10 s.
The total heat gain of solar collectors is expressed in Eq. 2.
where Qs is the total heat gain of solar collectors in MJ; msi is the working fluid flow rate in the heat collection system recorded in ith test in m3/s; ρsw is the density of collector fluid in kg/m3; csw is the specific heat capacity of a collector fluid in J/(kg,K); tdsi is the collector fluid temperature at the outlet recorded in ith test in C; tbsi is the collector fluid temperature at the inlet recorded in ith test in C; ΔTsi is the time step in s, in this case, ΔTsi = 10 s.
The solar fraction is expressed in Eq. 3.
where fj is solar fraction measured during short-term testing with certain Sun radiation circumstances, in %.
2.2 Solar photovoltaic collector installation designs
More of the urban buildings are multi-story buildings, whereas the roof heat collection area of urban buildings is generally regarded as sufficient to respond to the needs of the photovoltaic solar hot water systems. Therefore, currently, rooftop installations are the major form of photovoltaic board installation. On the other hand, in actuality, the building area is large, but the roof area is insufficient to meet the total heat demand of the building. To address the issue of insufficient heat collection area, balconies, walls between windows, and other parts of the east, south, and west building facades can be used. This study is aimed to transform the facade of high-rise buildings to achieve the best possible performance.
The quantitative evaluation of ecological function (natural circulation function) can improve the quality level of the target area environment by improving soil function, regulating microclimate and air quality, improving water circulation function, improving animal and plant habitat function, and leading to fundamentally solving urban ecological problems.
The ecological area ratio is given by Eq. 4 and levels are given in Table 3.
2.2.1 Facial installation method
In high-rise buildings, even if the entire roof is used, the problem of insufficient thermal collection area cannot always be solved. Therefore, the facade installation form can be adopted. The facade should be installed to receive as much sunlight as possible to avoid blocking, and safety should be prioritized. A balcony should be set up for older high-rise buildings and outdoor space for photovoltaic boards using thermal imaging analysis. In terms of integration with architecture, the heat collector can be combined with the building shading, and it should serve as shading and heat collector, simultaneously; for this purpose, arrange space can be arranged collector can be directly used as a balcony fence. Furthermore, the heat collector can be installed outside the air conditioner on the south side to serve the purpose of blocking the air conditioner. In Table 4, the business characteristics and local conditions that are a part of three-dimensional city greening plan are given.
2.2.2 Location of the balcony position and photovoltaic board
The exterior wall photovoltaic panel can be mounted directly from the building wall or on the walls of the construction to the south, west, southwest and east of the building. By reserving the tube and a heat collector on the wall, the water storage tank can be fixed to the wall, and the pipeline can be connected. The advantages of this scheme are as follows: i) the installation position of the heat collector is relatively flexible, and the size can be tailored according to the actual situation of the project; ii) the same layer can be used. Further, the pipeline gets shorter, and the heat loss becomes minimal. Also, the installation and maintenance are convenient; iii) a modeling element of architecture can be created when tightly combined with architecture. To achieve the ideal fusion of functionality and aesthetics, different color collecting pipes can be used to create heat collector arrays that have strong rhythmic senses based on the requirements of architectural shapes. The disadvantage of the exterior wall type is that it is typically installed perpendicular to the ground and vertical to the wall surface. The efficiency of the thermal collection is consequently somewhat diminished. The underlying residents might also not be able to use it for other reasons. Therefore, installing solar heat collectors can be thought of as a courteous inclination in order to receive a lot of solar radiation. The association of the two types of window walls could be as follows: i) to fix the heat collector, the triangular frame is directly installed on the lower wall of the window. It is critical to note that it should be used as an oblique tilt collector to avoid obstruction for lower-level users; ii) to rectify the fixed heat collector, the triangle is installed directly on the window’s wall, and used as an oblique tilt collector to avoid obstruction for lower-level users.
3 Results analysis and discussion
3.1 Thermal analysis of the building
It is an established fact that the heat island effect in cities worldwide is becoming increasingly severe, and heat energy in cities is disrupting human development activities. Basically, the heat energy was efficiently utilized by setting photovoltaic panels in the direction of concentrated heat energy and the facade of buildings to achieve the integration of solar energy systems and buildings can be expressed using Eq. 5.
where Uz is the horizontal direction wind speed at the height Z; Uo is the wind speed at the height of Zo; M is the power index determined by the terrain roughness. Referring to the “Code for the load of the Architecture” (GB50009), the M-value was adopted to be .14 in this study.
The airflow around the building generally belongs to non-compressed low-speed turbulence as per the association assumptions. Owing to this, the contact formation restrictions of the airflow and the building have a beneficial effect. In general, the K model has minimal computation requirements and more accurate predictions.
Turbulent kinetic energy model given in Eq. 6.
Dissipation rate model given in Eq. 7.
For thermal analysis results, simulation on the outside natural ventilation of the buildings is conducted for a municipality first using PHOENICS software (PHOENICS, 2021). Figure 2A shows the simulated profile of the municipality. Figure 2B shows the profile of selected area for the computation reflected by the area’s ventilation and heat energy. The outer field size of the model was chosen in PHOENICS software based on airflow at the building complex’s boundary. The positive direction of the model was set to the north along the y-axis based on relevant engineering experience and simulation trial calculation.
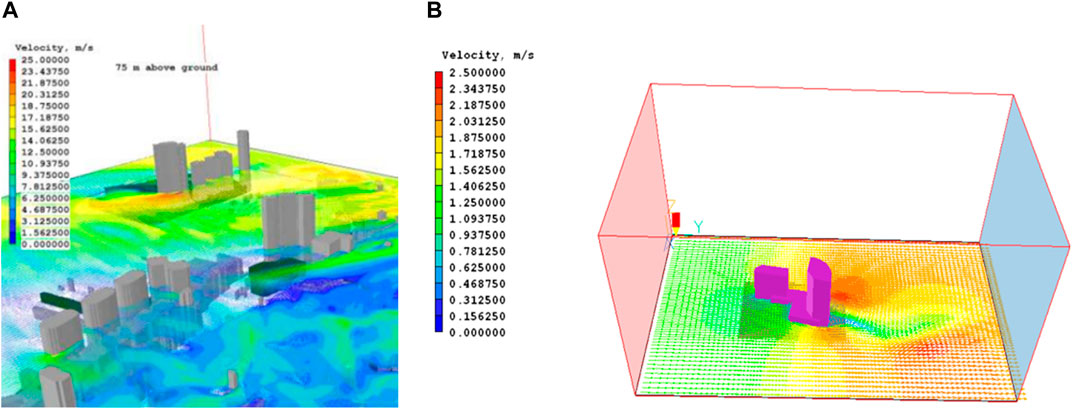
FIGURE 2. The thermal analysis of the buildings (A). Municipality with multiple buildings; (B). Selected areas with lower number of buildings.
3.2 Wind boundaries and wind speed
The non-compressed low-speed turbulence describes the airflow around the building according to the associated assumptions. Because of this, the airflow and building contact formation constraints are helpful. The K model generally requires less processing and produces more precise predictions. Figure 3 explains the wind boundaries and speed for the designed system.
3.3 Understanding the design efficiency by surface model
The following are essential steps to achieve the efficient integrated design of the solar heat water system and building: i) steel structure is set up on the outer facade of the original building to reinforce the building; ii) window is constructed between the floors and balcony space is created to facilitate the management of green plants, improve the ventilation of the building and reduce energy consumption; iii) a curved surface optical board device is set outside the balcony, which is convenient and easy to disassemble and maintain. The analysis shows that a curved surface is more conducive to alleviating urban wind pressure and protecting the buildings in comparison to a square surface, see Figures 4A, B. Further, for the aesthetic purpose, the outer balcony could be set up in a curved shape.
3.4 Annual energy savings potential
It is observed that the designed system can hold a total of 32 tons of hot water per day with a specific heat capacity of 5082880kj/per day. The conversion efficiency of electric heating is generally about 90%, and the total heat required to produce the same heat is Q = 5647644 kJ. While electrical energy used is 3,600 kJ and it needs 1568.7 kWh electricity/day. Table 5 shows the annual energy savings per solar photovoltaic collector technology.
3.5 Thermal efficiency
To illustrate how the thermal efficiency of a solar energy system was calculated, a centralized solar heat collection system with a circulating water pump was considered. Typically, under solar radiation, a solar heat collection system raises the temperature of hot water from its initial temperature to a preset level. In the solar heat collection system, only the water pump that circulates water uses electricity; no other equipment does. Using this system as an example, the circulating water pump’s rated input power was estimated to be around .265 kW. It took .128 h and consumed .034 degrees of electricity to circulate 1000 kg of water having 35° higher temperature. Therefore, the actual calorific value of the centralized solar heat collection system equipped with a circulating water pump is 1,029,411.76 kCal/degrees.
3.6 Wind load standard value detection
When there is no malfunction or damage from repeated pressure detection, the universal value of the wind load should be performed. Detection pressure increased by
3.6.1 Wind load design value detection
3.6.2 Evaluation of the test results
The border support triangular glass surface plate method was calculated using Eq. 8
Where,
Other component face line deflection computation can be done using Eq. 9:
Where
3.6.3 Evaluation of deformed detection
The evaluation of the deformation detection should indicate the deflection of the opposite face line
4 Discussion on functional benefits and conclusion
From the preliminary assessment carried out in this study, it is understood that façade reconstruction for integrating solar photovoltaic collectors with vertical green balconies is possible. The design and analysis, shows they could potentially offer two important functional benefits: 1). Ecological functions: Using their inherent ecological abilities, plants can purify indoor air, regulate temperature and humidity indoors, inhale carbon dioxide and exhale oxygen, maintain a balance of the two gases in the atmosphere, and keep the air fresh. They can also trap and filter airborne dust to clean the air. 2). Spatial function: By placing the balcony on the building’s facade, it is important to organize, embellish, and beautify the interior space. However, in order to achieve the transition of indoor and outdoor space, the green color can be set up in the area where outdoor and indoor spaces meet. The technique of borrowing scenery, through the glass and through the window can also be used so that the inside and outside green scenery is connected into a single piece.
The scientific analysis of building construction could be carried out using the PHOENICS software model about the existing high-rise building facade, steel reinforcement of older buildings, installation of solar energy combined with balconies, installation of photovoltaic panels set out on the steel frame, and solution to the shortage of urban greening and internal user comfort. It also reduces construction costs and encourages the construction of green buildings, in addition to reducing air pollution. The plan discussed in this study contributes to the delivery of services that satisfy customers, the elimination of temporary conditions, the reduction of construction-related annoyance for locals, the opportunity for building owners to receive early rental income, and the creation of a comfortable workplace. Future renovations of urban architecture are predicted to apply the process-specific rationalization plan that was created as a consequence of this study, and numerous experiences will be gathered from this that will be used to carry out more type-specific research.
Data availability statement
The original contributions presented in the study are included in the article/supplementary material further inquiries can be directed to the corresponding author.
Author contributions
ZW is responsible for Conceptualization, Data Curation, Formal Analysis, Funding Acquisition, Investigation, Methodology, Project Administration, Resources, Software, Supervision, Visualization, and Writing. KC-S, as the corresponding author, is responsible for all communication related to the paper, regularly checks the progress of further studies, makes sure the IMRAD section of the manuscript meets the requirement both in content and format before submission, including Introduction, Research Methods, Results, Discussion, and Conclusions, uploads the paper to the relevant database as required, deal with all relevant procedures, and promotes the whole process.
Conflict of interest
The authors declare that the research was conducted in the absence of any commercial or financial relationships that could be construed as a potential conflict of interest.
Publisher’s note
All claims expressed in this article are solely those of the authors and do not necessarily represent those of their affiliated organizations, or those of the publisher, the editors and the reviewers. Any product that may be evaluated in this article, or claim that may be made by its manufacturer, is not guaranteed or endorsed by the publisher.
References
Akram, M. W., Hasannuzaman, M., Cuce, E., and Cuce, P. M. (2021). Global technological advancement and challenges of glazed window, facade system and vertical greenery-based energy savings in buildings: A comprehensive review. Energy Built Environ. 4, 206–226. doi:10.1016/j.enbenv.2021.11.003
Bonomo, P., Chatzipanagi, A., and Frontini, F. (2015). Overview and analysis of current BIPV products: New criteria for supporting the technological transfer in the building sector. Vitr. Int. J. Archit. Technol. Sustain. 2015, 67–85. doi:10.4995/vitruvio-ijats.2015.4476
Cerón, I., Caamaño-Martín, E., and Neila, F. J. (2013). ‘State-of-the-art’ of building integrated photovoltaic products. Renew. Energy 58, 127–133. doi:10.1016/j.renene.2013.02.013
Dunnett, N., and Kingsbury, N. (2008). Planting green roofs and living walls. Portland, OR, USA: Timber Press.
Ekici, B. B., and Aksoy, U. T. (2009). Prediction of building energy consumption by using artificial neural networks. Adv. Eng. Softw. 40 (5), 356–362. doi:10.1016/j.advengsoft.2008.05.003
Goh, K. C., Goh, H. H., Yap, A. B. K., Masrom, M. A. N., and Mohamed, S. (2017). Barriers and drivers of Malaysian BIPV application: Perspective of developers. Procedia Eng. 180, 1585–1595. doi:10.1016/j.proeng.2017.04.321
Heinstein, P., Ballif, C., and Perret-Aebi, L. E. (2013). Building integrated photovoltaics (BIPV): Review, potentials, barriers and myths. Green 3, 125–156. doi:10.1515/green-2013-0020
Hollands, J., and Korjenic, A. (2021). Evaluation and planning decision on facade greening made easy—Integration in BIM and implementation of an automated design process. Sustainability 13 (16), 9387. doi:10.3390/su13169387
IEA (2021). Empowering “Smart Cities” toward net zero emissions. AvaliableAt: https://www.iea.org/news/empowering-smart-cities-toward-net-zero-emissions.
Jelle, B. P., Breivik, C., and Røkenes, H. D. (2012). Building integrated photovoltaic products: A state-of-the-art review and future research opportunities. Sol. Energy Mat. Sol. Cells 100, 69–96. doi:10.1016/j.solmat.2011.12.016
Jelle, B. P., and Breivik, C. (2012). The path to the building integrated photovoltaics of tomorrow. Energy Procedia 20, 78–87. doi:10.1016/j.egypro.2012.03.010
Liu, O. Y., and Russo, A. (2021). Assessing the contribution of urban green spaces in green infrastructure strategy planning for urban ecosystem conditions and services. Sustain. Cities Soc. 68, 102772. doi:10.1016/j.scs.2021.102772
Montoro, D. F., Vanbuggenhout, P., and Ciesielska, J. (2011). “Building Integrated Photovoltaics: An overview of the existing products and their fields of application,” in Report prepared in the framework of the European funded project (Saskatoon, Canada: BIPV).
Munari Probst, M. C., Roecker, C., Frontini, F., Scognamiglio, A., Farkas, K., Maturi, L., et al. (2013). “Solar energy systems in architecture-integration criteria and guidelines,” in International energy agency solar heating and cooling programme. Editors M. Probst, M. Cristina, and C. Roecker (Paris, France: International Energy Agency).
Nagy, Z., Svetozarevic, B., Jayathissa, P., Begle, M., Hofer, J., Lydon, G., et al. (2016). The adaptive solar facade: From concept to prototypes. Front. Archit. Res. 5, 143–156. doi:10.1016/j.foar.2016.03.002
PHOENICS (2021). Software. AvaliableAt: https://www.britannica.com.
Piselli, C., Castaldo, V., Pigliautile, I., Pisello, A., and Cotana, F. (2018). Outdoor comfort conditions in urban areas: On citizens’ perspective about microclimate mitigation of urban transit areas. Sustain. Cities Soc. 39, 16–36. doi:10.1016/j.scs.2018.02.004
Prieto, A., Knaack, U., Auer, T., and Klein, T. (2017). Solar façades-Main barriers for widespread façade integration of solar technologies. J. Façade Des. Eng. 5, 51–62. https://sdgresources.relx.com/articles/urban-planning-sustainability-framework-systems-approach-blue-green-urban-design
Puchol-Salort, P., O’Keeffe, J., van Reeuwijk, M., and Mijic, A. (2020). An urban planning sustainability frame work: Systems approach to blue green urban design. Sustain. Cities Soc. 66, 102677.
Ritzen, M., Reijenga, T., El Gammal, A., Warneryd, M., Sprenger, W., Rose-Wilson, H., et al. (2016). “IEA-PVPS task 15: Enabling framework for BIPV acceleration. (IEA-PVPS),” in Proceedings of the 48th IEA PVPS executive commitee meeting (Vienna, Austria: IEA).
Scognamiglio, A., Farkas, K., Frontini, F., and Maturi, L. (2012). “Architectural quality and photovoltaic products,” in Proceedings of the 27th European photovoltaic solar energy conference and exhibition (Frankfurt, Germany: EUPVSEC), 24–28.
Shi, J., Su, W., Zhu, M., Chen, H., Pan, Y., Wan, S., et al. (2013). Solar water heating system integrated design in high-rise apartment in China. Energy Build. 58, 19–26. doi:10.1016/j.enbuild.2012.10.018
Shih, W. Y., Ahmad, S., Chen, Y. C., Lin, T. P., and Mabon, L. (2019). Spatial relationship between land development pattern and intra-urban thermal variations in Taipei. Sustain. Cities Soc. 62, 102415. doi:10.1016/j.scs.2020.102415
Shukla, A. K., Sudhakar, K., and Baredar, P. (2016). A comprehensive review on design of building integrated photovoltaic system. Energy Build. 128, 99–110. doi:10.1016/j.enbuild.2016.06.077
Tabakovic, M., Fechner, H., Van Sark, W., Louwen, A., Georghiou, G., Makrides, G., et al. (2017). Status and outlook for building integrated photovoltaics (BIPV) in relation to educational needs in the BIPV sector. Energy Procedia 111, 993–999. doi:10.1016/j.egypro.2017.03.262
Wei, X., Ruicheng, Z., and Bin, L. (2010). China Solar PV Industry Research Report. Doudin.com 2010, 12–27. https://www.docin.com/p-111802404.html
Xue, F., Gou, Z., and Lau, S. S. Y. (2017). Green open space in high-dense Asian cities: Site configurations, microclimates and users’ perceptions. Sustain. Cities Soc. 34, 114–125. doi:10.1016/j.scs.2017.06.014
Yang, R. J., and Zou, P. X. (2016). Building integrated photovoltaics (BIPV): Costs, benefits, risks, barriers and improvement strategy. Int. J. Constr. Manag. 16, 39–53. doi:10.1080/15623599.2015.1117709
Yu, Z., Chen, S., Wong, N. H., Ignatius, M., Deng, J., He, Y., et al. (2020). Dependence between urban morphology and outdoor air temperature: A tropical campus study using random forests algorithm. Sustain. Cities Soc. 61, 102200. doi:10.1016/j.scs.2020.102200
Keywords: building integrated photovoltaic (BIPV), photovoltaic panels, vertical greening, building facades, building retrofitting, wind environment, urban heat island effect
Citation: Wu Z and Chul-Soo K (2023) A preliminary study understanding the possibility and benefits of solar photovoltaic collector integration with vertical green balconies in building facade reconstruction. Front. Energy Res. 10:1025564. doi: 10.3389/fenrg.2022.1025564
Received: 23 August 2022; Accepted: 30 December 2022;
Published: 18 January 2023.
Edited by:
Anshu Priya, City University of Hong Kong, Hong Kong SAR, ChinaReviewed by:
Sandhya Thotakura, Gandhi Institute of Technology and Management (GITAM), IndiaAlpesh Desai, Pandit Deendayal Petroleum University, India
Copyright © 2023 Wu and Chul-Soo. This is an open-access article distributed under the terms of the Creative Commons Attribution License (CC BY). The use, distribution or reproduction in other forums is permitted, provided the original author(s) and the copyright owner(s) are credited and that the original publication in this journal is cited, in accordance with accepted academic practice. No use, distribution or reproduction is permitted which does not comply with these terms.
*Correspondence: Kim Chul-Soo, a2FuanJAbmF2ZXIuY29t