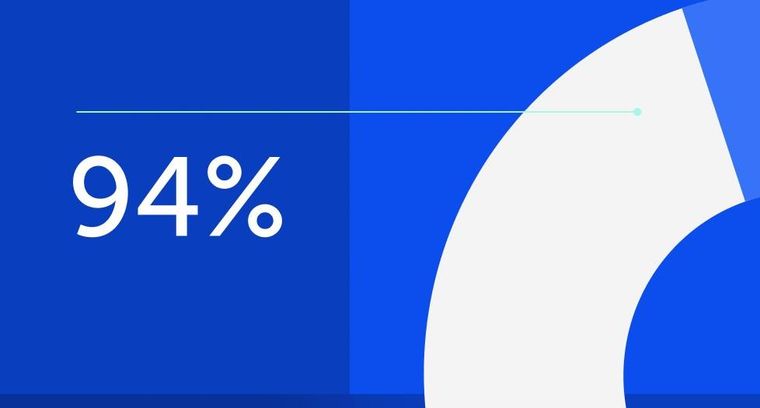
94% of researchers rate our articles as excellent or good
Learn more about the work of our research integrity team to safeguard the quality of each article we publish.
Find out more
ORIGINAL RESEARCH article
Front. Energy Res., 13 January 2023
Sec. Sustainable Energy Systems
Volume 10 - 2022 | https://doi.org/10.3389/fenrg.2022.1005720
This article is part of the Research TopicCritical Energy Minerals: A Material Enabler for Carbon NeutralityView all 17 articles
Based on global cobalt technology patents, this paper uses patent data mining and co-occurrence methods to identify technology application trends, key technology areas, key technology layouts, and technology roadmap. According to the development trend, the development of co-cobalt technology is divided into slow and rapid development stages. The conclusion are: 1) In terms of key technologies, E35-V (Cobalt compound) is the long-term R&D focus in the field of cobalt, and X16-B01F1 (Lithium-based) is the most important key technology area at present. 2) China, Japan, the United States, and South Korea are the main contributors to key cobalt technologies, mainly focusing on the R&D layout of L03-E01B5B (Lithium electrode) technology in the field of X16-B01F1 (Lithium-based). 3) Regarding the cooperation relationship between patent applicants, cooperation between groups is relatively close, cooperation between different countries is less, and transnational cooperation is mainly concentrated in developed countries, among which the United States has the most transnational partners. 4) In the field of cobalt’s most important electrode material technology LG Chemical Co., Ltd. (Korea) and Sumitomo Metal Mining Corporation (Japan) are the two companies with clear technology lines, while Univ Cent South (China) has a late technology development. Finally, we make suggestions for the development of cobalt technology: focus on core technology for R&D and innovation, actively study the technology status and innovation mode of leading companies, and strengthen the cooperation among different R&D institutions.
Cobalt is an important emerging key mineral resource. Several countries such as the United States, China, and Japan have now included cobalt in their national strategic mineral inventories, making it a key mineral for global strategic mineral research and assessment (McCullough and Nassar, 2017). The Democratic Republic of Congo is the world’s largest producer of cobalt ore reserves and production. Cobalt production in the DRC has been a major global supply for decades. At the same time, China is the largest producer of refined cobalt globally, followed by Finland and Canada (Dehaine et al., 2021). Cobalt is widely used in high-tech fields such as aerospace, chemical, electronics, medical and high-temperature magnetic alloys (Hayes and McCullough, 2018). In addition to these applications, cobalt is used to manufacture catalysts, desiccants, dyes, pigments, and pharmaceuticals (Sun et al., 2019). At the same time, cobalt is also an important raw material. Due to the rapid development of new energy electric vehicles, the battery industry has become cobalt’s most important consumption field (Liu et al., 2020). With the rapid development of strategic emerging industries and the transformation of some clean energy sources, fuel vehicles have gradually shifted to electric vehicles, and the economic value, as well as the demand for cobalt, has multiplied (Campbell, 2019). As a frontier material for future development, cobalt has made breakthroughs in related patent technologies, and the number of global patent applications has increased exponentially. Many enterprises worldwide have started early in the patent development of cobalt-related technologies, but most still lack patent layouts of critical technologies. Enterprises involved in cobalt technology R&D in each country must grasp related technologies’ global patent application trends and actively lay out key patent technologies to improve their competitive advantages. Therefore, it is crucial to understand the current global critical technologies of the cobalt industry and their development rules and analyze the technology planning and innovation models of critical technologies in each country to promote the high-quality development and strategic layout of cobalt technology.
Scholars’ research on cobalt mainly revolves around two aspects: one is the analysis of cobalt ore resources in terms of reserves, supply, and trade, and the other is the development and application of cobalt-related technologies. Horn et al. (2021) studied three deposit types of cobalt and identified 104 deposits in Europe currently being explored for cobalt, 79 of which are located in Finland, Norway, and Sweden. Fu et al. (2020) found by quantifying the material market of cobalt that with the increase in demand for lithium batteries, the demand for cobalt resources is expected to grow by more than 300% in the next decade, and the future faces a significant risk of supply concentration and mining restrictions of cobalt resources. In addition, some scholars have also studied the flow of cobalt using a trade-related material flow model and found that the supply and demand of cobalt have seen rapid growth, with the Democratic Republic of Congo, the United States, China, and Japan being the major powers in the cobalt trade network (Cullen and Allwood, 2013; Sun et al., 2019). Other studies have used a link predictive analysis model, combined with trade networks, to forecast the international trade relationship of cobalt (Liu et al., 2020). The results show reduced trade stability of cobalt and forecast the next three and 5 years for the countries most likely to trade cobalt ore.
Cobalt is currently being studied in various technological fields of technology and applications, such as catalysts, alloy materials, magnetic materials, medicine, and battery materials. Cobalt can be used as a catalyst to improve energy utilization, and Kim et al. (2020) synthesized cobalt-based catalysts with enhancement by inserting traces of palladium. Kim et al. (2018)also prepared cobalt-based catalysts with different base strengths by controlling the amount of yttrium oxide. Metallic cobalt can also be used with other metals to make alloys to generate high-temperature alloys, magnetic alloys, and anticorrosive alloys (Talapatra et al., 2009; Garcia et al., 2016; Yu et al., 2018; Wang et al., 2022). In medical research, cobalt was found to be a trace element in the human body, and its metabolic level was associated with thyroid hormones (Alkjaer et al., 2005). Lin et al. (2020) found that cobalt compounds can act as antimicrobial agents, promote bone cell growth, and reduce inflammatory responses. Cobalt also has many cutting-edge studies in the field of battery materials. Luo et al. (2022) summarized recent nickel-rich and cobalt-as-cathode materials. He et al. (2015) found that cobalt sulfide can be used not only as a lithium-ion battery electrode material but also as a cathode active material in rechargeable magnesium batteries.
Patent data is the world’s largest and most reliable source of technical information and one of the most effective indicators of the current state of technological intellectual property development (Safin and Manteuffel, 2016). Patent analysis methods can be used to identify the state of technological development and current research hotspots and predict future development and competitor innovation trends (Zou et al., 2018). At present, few scholars have conducted an overall overview and analysis of the technology field of cobalt from the perspective of patent technology. Most of the existing patent technology research focuses on the downstream of cobalt, such as lithium-ion battery technology. Lee and Su (2020) used patent analysis methods to analyze lithium-ion battery patent trends using patent metrics and analyzed the technological innovation and similarity of the top six patent assignees. Aaldering and Song (2019) used a data-driven approach combining patent co-classification analysis, link prediction algorithm application, and text mining techniques to highlight the post-lithium-ion battery technology research advances. Malhotra et al. (2021) analyzes the evolution of lithium-ion battery knowledge trajectories over the past 50 years.
From the studies mentioned above, it can be found that previous studies lacked research on the cobalt industry from the perspective of patent analysis, while cobalt belongs to the critical raw materials of the future, and researching key technologies of cobalt is of great significance for countries to carry out technological R&D and strategic layout. As global competition becomes increasingly fierce, science and technology innovation has also become a core indicator of national competitiveness. Improving the level of critical technologies and innovation capability has become a significant task at present. Therefore, assessing the R&D trends and the current situation in the field of cobalt key technologies, determining the national competitive posture of key technologies, and clarifying the future key technology R&D directions are of great practical significance for countries to carry out the layout of cobalt key technology R&D and improve their scientific and technological innovation capabilities. Therefore, in order to effectively evaluate the critical technologies of cobalt, this paper takes the patent data of cobalt as the basis, firstly divides the technological development of cobalt into the slow development stage and rapid development stage according to the development trend, then uses patent analysis and co-occurrence analysis to determine the critical technologies of cobalt in each stage, followed by the analysis of the technology layout and cooperation of patent applicants. Finally, patent applicants in cobalt’s most important electrode material technology field are selected to analyze the patent technology routes. The rest of this report is organized as follows. Section 2 introduces the research framework and methodology, Section 3 presents the research content, and Section 4 concludes and presents the policy comments of this paper.
Based on patent data mining methods such as patent analysis and co-occurrence analysis, this paper first delineates the development trend of cobalt patent technology. The key technologies at different development stages are identified, and on this basis, the patent technology layout and the cooperation relationship between patent applicants and patent application countries are analyzed. Finally, the patent applicants in the most important electrode material technology field of cobalt are selected for the analysis of patent technology routes. The overall analysis framework of this paper is shown in Figure 1, and the whole analysis process is refined into the following six steps.
The global trend of technology applications for cobalt materials is shown in Figure 2. Patent applications for cobalt-related technologies first began around the 1960s, and the number of applications began to show a rapid growth trend after the 21st century. Currently, the countries with a large number of cobalt-related patent applications worldwide are China, the United States, and Japan. The earliest cobalt technology patent was applied for by the United States in 1955, while the earliest patent in China was applied for in 1986, compared with the late start of cobalt industry technology research and development in China. Specifically, the development of global patents related to cobalt technology is divided into two main stages.
The first stage was from 1955 to 1995, a period of slow technological development with few patent applications. In the 1940s, the United States first paid attention to and developed cobalt-based alloys, and at the end of the 1950s, cobalt-based alloys were widely cast and used in the United States. Global patents on cobalt technology were mainly in the hands of Japan and the United States. The second phase, from 1996 to the present, is a period of rapid technological growth, with the total number of patent applications increasing rapidly. Japan and the U.S. still hold many technology patents, but the number of patent applications in China and Korea is also growing rapidly. Due to the global financial crisis in 2008, the growth rate of cobalt industry consumption in many countries was restrained and slowed down. Refined cobalt production and sales in China have surged due to the stagnation of many overseas projects. The rapid growth of cobalt technology patents filed in China has made it the most important country and the main driver of technology development in the cobalt industry. There is an 18-month lag in current patent applications, so the data for the latter period is for reference only.
According to the slow and rapid development stages divided in 3.1, the hot patent technology fields with frequencies greater than 200 times and greater than 3,000 times are extracted for co-occurrence analysis, as shown in Figure 3. The nodes in the graph represent the technical field of cobalt, the node size represents the frequency of occurrence of that technical field, and the node color represents the betweenness centrality of the technical field. The edges between the nodes represent the two technical fields that appear in the patent at the same time, and the weights of the edges represent the number of times the two technical fields appear at the same time. The figure shows that the technology nodes of M26-B08, J04-E04, E35, and E35-V in the slow development stage are darker and larger in color. According to the patent codes, we can find that Nickel or cobalt alloys, Catalysts, Compounds of other metals, and Cobalt compounds are the important and hot technology areas of cobalt. There are more important and hot technology areas in the second stage, and the technology nodes of A10-E05B, X16-B01F1, L03-E01B5B, and E35-W are larger and darker, indicating that Chemical modification by carbonisation, Lithium-based, Lithium electrodes, Nickel compound, etc. are the hot technology industries of cobalt.
In order to better identify the key technology areas, this paper uses the top 10 Derwent Manual Code ranked by the product of frequency and betweenness centrality in the technology areas to represent the top 10 key technology areas (as Table 1 shows). Based on the identification results and the meaning of the entries of the Derwent manual codes, it can be concluded that the first ranking of cobalt key technology patents in the slow development stage is E35-V (Cobalt compound), M26-B08 (Nickel or cobalt alloy), J04-E04 (Catalysts), M13-B (Coating from solution or suspension of metal compounds), etc. also belong to the key technology area of cobalt in this stage. In the rapid development stage, X16-B01F1 (Lithium-based) replaced E35-V (Cobalt compound) as the top-ranked key technology, and L03-H05(Vehicles), L03-E01B5B (Lithium electrodes), and L03-E08B (Production of electrodes) also became important key technology areas for cobalt in this stage. In 1990, Sony invented the lithium-ion battery, and the demand for lithium cobaltate as one of the anode materials began to increase rapidly, and its related technology patents also became the key technology of the cobalt industry. Cobalt-containing batteries are widely used because of their high energy density, en-vironmental safety, and stable performance.
From the trend of cobalt key technologies over time, it can be found that E35-V (Cobalt compound) ranks first and second in the slow development phase and the rapid development phase, respectively, indicating that Cobalt compound has been the focus of technology research and going in the cobalt industry. x16-B01F1 (Lithium-based) has been ranked first in the rapid growth phase. It has been the top-ranked key technology area so far. From the rapid growth stage, the most important use of cobalt is for lithium-ion batteries as the electrode material for ternary batteries. From the vital technology changes in each phase, catalysts such as J04-E04 (Catalysts) and N06-F (Catalyst support) have been necessary critical technologies for the cobalt industry. E08B (Production of electrodes) has become the new key technology for the cobalt industry.
In order to further analyze the characteristics of critical technologies, we created a matrix for each phase of technology topics with the number of patent occurrences as the horizontal axis and the betweenness centrality as the vertical axis. We took the average of the two as the intersection point of the analysis (as Figure 4 shows).
In the slow development stage, the first quadrant is the quadrant with the high occurrence and high intermediate centrality. E35-V (Cobalt compound) and M26-B08 (Nickel or cobalt alloy) are located in the first quadrant, where E35-V (Cobalt compound) has the highest occurrence and highest intermediate centrality, indicating that it is the focus of R&D in the slow development phase. M13-B (Coating from solution or suspension of metal compounds) and L03-B01A (Variable resistors) are located in the second quadrant, where key technologies play a mediating role in the technology field but are less studied. The third quadrant is the low incidence and low intermediate centrality quadrant, where the key technologies are less important and less studied than the other key technologies, E35-W (Nickel compound), E35 (Compounds of other metals), N02 [Fe, Co, Ni, Cu, noble metal—element, (hydr)oxide, inorganic salt, carboxylate] and L03-B02B (Magnetic non-metals) are located in this quadrant. The fourth quadrant is the high occurrence and low intermediate centrality quadrant, where J04-E04 (Catalysts) and N02-B [Cobalt—element, (hydr)oxide, inorganic salt, carboxylate catalyst] are located, and the key technology for this phenomenon is a popular research area, but the relationship with other key technologies is weak.
Similarly, the three technology areas in the first quadrant of the rapid development phase are X16-B01F1 (Lithium-based), E35-V (Cobalt compound), and L03-H05 (Vehicles), indicating that these three technologies are the focus of research in the rapid development phase and are in a critical position. Among them, X16-B01F1 (Lithium-based) is the first in the number of occurrences and intermediary centrality, which is the most important key technology in the rapid development stage. There are four technology areas in the second quadrant, namely N02-B01 (Cobalt element or oxide catalyst), N06-F (Catalyst support), J04-E04A (Redox catalysts), and E35-W (Nickel compound), and this quadrant’s key technologies are more closely linked to other technologies but are relatively less studied. In the fourth quadrant, L03-E01B5B (Lithium electrodes), L03-E08B (Production of electrodes), and X16-E01C1 (Oxides, complex oxides) are a hot research area but have less connection with other technologies. Among them, the lithium-ion and electrode field is a hot R&D area for cobalt but relatively less connected with other technology fields.
According to the statistics of patent applications for cobalt key technologies, China, Japan, the United States, and South Korea account for 40%, 23%, 16%, and 7% of the global total patent applications for cobalt key technologies, respectively. Therefore, this paper focuses on the technological layout of cobalt critical technologies in China, Japan, the United States, and South Korea at different stages of development. As shown in Figure 5, the size of the bubbles indicates the number of patents filed by each country on crucial technologies. The horizontal coordinates represent countries, and the vertical coordinates represent different key technologies.
As can be seen from the Figure 5, during the slow growth period, Japan has an absolute advantage in the number of patent applications for crucial technologies of cobalt, especially in the fields of E34 (Compounds of other metals) and N02 [Fe, Co, Ni, Cu, noble metal—element, (hydr)oxide, inorganic salt, carboxylate] in two technical fields. The U.S. technology areas of N02 [Fe, Co, Ni, Cu, noble metal—element, (hydr)oxide, inorganic salt, carboxylate] and N02-B Cobalt—element, (hydr)oxide, inorganic salt, The number of patent applications in N02-B [Cobalt—element, (hydr)oxide, inorganic salt, carboxylate catalyst] is high, while China and Korea are less competitive and have fewer patent applications at this stage. During the rapid growth period, China has an absolute advantage in the number of patent applications for critical technologies and is the most competitive country overall; it is mainly in the patent fields of X16-B01F1 (Lithium-based), L03-E01B5B (Lithium electrodes) and L03-E08B (Production of electrodes). Japan is second only to China in the number of applications for crucial cobalt technologies, but it overtook China as the world’s top filing country in L03-H05 (Vehicles). However, Japan has relatively few applications in catalyst technologies such as N06-F (Catalyst support) and J04-E04A (Redox catalysts). The critical technology applications in the U.S. at this stage are mainly in the fields of X16-B01F1 (Lithium-based) and N02-B01 (Cobalt element or oxide catalyst), and the number of patent applications for N02-B01 (Cobalt element or oxide catalyst) has exceeded that of Japan The number of patent applications for N02-B01 (Cobalt element or oxide catalyst) has surpassed that of Japan and is only surpassed by China. Korea is mainly in X16-B01F1 (Lithium-based) and L03-E01B5B (Lithium electrodes) technologies.
Compared with the slow development stage, the number of applications in China and Korea has increased significantly. Both China and Korea mainly focus on the R&D of X16-B01F1 (Lithium-based) and L03-E01B5B (Lithium electrodes) technology areas. China’s total number of patent applications for key cobalt technologies surpasses Japan’s, making it the most competitive country. However, Japan has the highest number of applications in the world in L03-H05 (Vehicles).
In order to analyze the layout of different companies in the field of cobalt key technologies, this paper selects the major patent owners whose total patent applications in key technologies in the slow development stage and the rapid development stage exceed 50 and 200, respectively, for comparison, and their patent technology layout is shown in Figure 6. Among them, gray nodes represent key technologies, yellow, orange, green, blue, and red nodes indicate Japanese, American, German, Korean and Chinese patentees, respectively, and the thickness of connecting lines between nodes represent the number of patent applications for key technologies. As seen from Figure 6A, the key technology companies applying for cobalt in the slow development stage mainly originate from Japan, such as HITACHI LTD., MATSUSHITA ELEC IND CO. LTD., TDK ELECTRONICS CO. LTD., etc. The main layout is E35-V (Cobalt compound) and M26-B08 (Nickel or cobalt alloy). PHILLIPS PETROLEUM CO and TDK CORP in the United States are also an essential part of cobalt key technology. Secondly, BASF AG in Germany also has more patents in N02-B [Cobalt-element, (hydr)oxide, inorganic salt, carboxylate catalyst] and E35-V (Cobalt compound).
It can be seen in the rapid development stage that the main origins of companies applying for cobalt key technology are starting to become diversified, mainly China, Korea, Japan, and Germany. Chinese CAS DALIAN CHEM & PHYSICAL INST, CHINA PETROLEUM & CHEM CORP, and UNIV ZHEJIANG are the main contributors to the vital cobalt technology in China, mainly in N02-B01 (Cobalt element or oxide catalyst) and N06-F (Catalyst support). Chinese universities and research institutes are an important part of the global cobalt key technology patentees and have strongly promoted cobalt key technology innovation. In addition, Japan’s SANYO ELECTRIC CO. LTD., TOYOTA JIDOSHA KK, and SONY CORP have strong R&D strength in key cobalt technologies, mainly in the field of X16-B01F1 (lithium-based) technology. LG CHEM LTD. and SAMSUNG SDI CO. LTD. of Korea have focused on X16-B01F1 (Lithium-based) and X16-E01C1 (Oxides, complex oxides), and these two companies have the largest number of patents in X16-B01F1 (Lithium-based) technology, so Korea has a certain technological advantage in Lithium-based. BASF SE of Germany has more patents in X16-B01F1 (Lithium-based) and N02-B01 (Cobalt element or oxide catalyst).
In order to study the cooperative relationship of key technology patent applicants of cobalt, this paper uses Gephi software for the co-occurrence diagram of patent applicants, as shown in Figure 7. In order to understand the evolutionary characteristics of the overall cooperative network, the parameters of the overall network characteristics of the cooperative network at different stages are compiled in Table 2 in this paper. It can be seen from the figure that from the slow growth stage to the rapid development stage, the network size expands, and the number of cooperative relationships increases rapidly. The average clustering coefficient and the average shortest path length in Table 2 represent the degree of close cooperation among patent applicants. It can be found that the average clustering coefficient becomes larger and the average path value becomes smaller, indicating that the cooperative relationships among patent applicants are getting closer and closer. The cooperation network shows the characteristics of large dispersion and small aggregation, with close cooperation among institutions in the same country but less cooperation among institutions in different countries.
In order to deeply analyze the cross-border cooperation relationship of patent applicants, the patent applicants with a high number of cross-border cooperation in both phases are visualized in Figure 8. Different colors in the figure represent the countries from which the organizations come, with yellow representing Japan, orange representing the US, purple representing the Netherlands, green representing Germany, red representing China, blue representing Korea, gray representing the UK, pink representing Canada, rose pink representing Italy, and brown representing France. As seen from the figure, in the slow development stage, US firms engage in more cross-border cooperation, with more frequent cooperation between institutions in the US and the Netherlands. The number of cross-border collaborations by global institutions in the slow development stage is relatively small. In the rapid development stage, cross-border cooperation among patent applicants increased, with the most frequent cooperation between Italy and France. Italy’s Eni, Germany’s Infineon, and France’s Inst Francais collaborate more frequently with other countries. Overall, cross-border cooperation in key cobalt technologies is mainly concentrated in developed countries such as the US, Italy, and the UK, with the US having the most cross-border partners.
The technology roadmap is an important tool for strategic planning and decision-making, as it can clearly show the pulse and frontier of technology development to relevant organizations through visualization. In this paper, we have selected the critical technology area of cobalt in electrodes and created a patent roadmap based on the patent citation relationship based on the three organizations from different countries with the largest number of related patents, LG Chem Ltd. (Korea), Sumitomo Metal Mining Co. (Japan) and Univ Cent South (China). The critical patents in the patent roadmap are determined based on the frequency of patent citations, key technology areas, and the number of family patents. According to the statistical analysis, in the field of cobalt electrode technology, LG Chem Ltd. (Korea) has 993 patent records, Sumitomo Metal Mining Co. (Japan) has 419 records, and Univ Cent South (China) has 424 records. The patent technology roadmap of the three organizations in the field of electrodes is shown in Figure 9, with the gray part of the figure showing the patents of other organizations and the green part showing the patents of their organizations.
LG Chem Ltd. filed a patent WO2004040677A1 on optimized cathode materials for cobalt-containing lithium batteries in 2004, which was cited 71 times. As a result of this technology, the patent WO2016175597-A1 on cathode active material for cobalt-containing lithium-ion secondary batteries was filed in 2016 and was cited 21 times, with 12 family patents. 2005 LG Chem Ltd. filed a patent WO2005011030A1 on anode active material for cobalt-containing lithium-ion secondary batteries, with 22 family patents, and was cited 66 times. On this basis, LG Chem Ltd. and other organizations applied for several patents related to cobalt-containing electrode materials and became the country with the largest number of patents for cobalt electrode materials in the world.
In 2011, Sumitomo Metal Mining Co. applied for WO2011067935A1, “Manufacture of nickel-cobalt-manganese composite hydroxide particles for manufacturing cathode active materials,” which has 16 patents in the family and has been cited 76 times. In addition, WO2014119165A1, “Manufacture of nickel-cobalt composite hydroxide for active cathode materials,” was filed in 2014 with 29 citations and 16 family patents. Sumitomo Metal Mining Co. Based on this, more related patents have been filed.
In 2008, Univ Cent South applied for patent CN101320821A on the technology of integrated energy storage components for capacitors and lithium-ion batteries, which was cited 32 times, and two family patents. 2017, Univ Cent South applied for CN106784525A on the technology of lithium-sulfur battery diaphragm and graphene preparation, which was cited 47 times.
In general, the core technology of the most popular electrode material for cobalt is receiving attention from various companies. Among them, LG Chem Ltd. (Korea) and Sumitomo Metal Mining Co. (Japan) are two companies with clear technology lines, while Univ Cent South (China) is late in developing its technology. Univ Cent South (China) has more patents for critical technologies, but the quality of core technologies is poorer than the other two organizations.
The current research lacks research on cobalt key technologies from the perspective of patent analysis, while cobalt belongs to the key raw materials of the future. There are gaps in the research on the development trend, patent owners, key technology layout, and technology development route of cobalt key technology, etc. Assessing the R&D trend and current situation in the field of cobalt key technology is of great practical significance for countries to carry out the R&D layout of cobalt key technology and improve their scientific and technological innovation capability. Therefore, this paper divides the development trend into slow development stages and rapid development stages and uses patent analysis and symbiosis analysis to identify key technologies according to the current status of technology development in the global cobalt industry. On this basis, the layout of key technologies in the cobalt industry is analyzed from the perspective of the key technology patent applicants and the countries to which the applicants belong, and the cooperative relationship among the patent applicants is analyzed. Finally, by analyzing the technical routes of the main patent owners in the most critical core technology field of cobalt-electrode material technology, the main conclusions drawn are as follows:
(1) Patent co-occurrence analysis is used to identify critical technologies and important cobalt features at different stages. E35-V (Cobalt compound) belongs to the key technologies that have been the focus of R&D in the cobalt industry, while X16-B01F1 (Lithium-based) is the most important key technology area at present.
(2) The patent layout of cobalt key technologies is analyzed according to the patent applicants and the countries to which they belong. China, Japan, the United States, and South Korea are the main contributors to cobalt key technologies. Each country concentrates on the layout of R&D in the X16-B01F1 (lithium-based) fields L03-E01B5B (lithium electrode) technologies.
(3) Based on the cooperation network of cobalt key technology patent applicants, the cooperation relationship is analyzed. Collaboration networks have become closer over time between patent applicants. Most collaborative relationships are domestic, with fewer patent applicants cooperating across borders. Transnational cooperation in cobalt key technologies is mainly concentrated in developed countries such as the United States, Canada, and the United Kingdom, among which the United States has the most transnational partners.
(4) The three organizations with the highest number of relevant patents in different countries were identified according to the key technology areas of electrode materials for cobalt, and a patent roadmap was established based on the patent citation relationship. LG Chem Ltd. (Korea) and Sumitomo Metal Mining Co. (Japan) are the two companies with clear technology lines, while Univ Cent South (China) has a later technology development.
Identify the key technology areas of cobalt, and analyze the critical technology layout of applicants and applicant countries as well as the cooperation relationship to provide certain reference values for the R&D, innovation, and strategic layout of relevant technologies in this field. Combining the above research findings, the following recommendations are made.
(1) We should strengthen the R&D and innovation of core technologies such as X16-B01F1 (lithium-based) and L03-E01B5B (lithium electrode) around the cobalt industry chain and pay close attention to the new developments in the field of cobalt in recent years. In recent years, cobalt, an important raw material for new energy power batteries, has had a broad market prospect. In the future, we should increase our technological research and development efforts and innovation to expand our technological fields.
(2) Countries should increase support for critical enterprises to promote their research and development of key technologies to gain advantages for international competition. For enterprises with strong international competitiveness, other enterprises can actively learn from the technology status and innovation models of leading enterprises, such as Sony Corporation of Japan and Lg Chemical Co. of Korea. Actively promoting the cooperation of different R&D institutions and building industrial R&D technology innovation alliances, not only limited to the cooperation within the country but also strengthening the transnational partnership, is conducive to accelerating the R&D process to improve the efficiency of technology achievement transformation.
The original contributions presented in the study are included in the article/Supplementary Materials, further inquiries can be directed to the corresponding author.
ZH contributed to the study conception, data collection, methodological application, and paper writing. TD was involved in the improvement, revision and refinement of the study.
This research is supported by grants from the National Natural Science Foundation of China (Grant No. 71991485, and No. 71991480), and Basic Science Center Project for National Natural Science Foundation of China (No. 72088101, the Theory and Application of Resource and Environment Management in the Digital Economy Era).
The authors declare that the research was conducted in the absence of any commercial or financial relationships that could be construed as a potential conflict of interest.
All claims expressed in this article are solely those of the authors and do not necessarily represent those of their affiliated organizations, or those of the publisher, the editors and the reviewers. Any product that may be evaluated in this article, or claim that may be made by its manufacturer, is not guaranteed or endorsed by the publisher.
Aaldering, L. J., and Song, C. H. (2019). Tracing the technological development trajectory in post-lithium-ion battery technologies: A patent-based approach. J. Clean. Prod. 241, 118343. doi:10.1016/j.jclepro.2019.118343
Alkjaer, T., Pilegaard, M., Bakke, M., and Jensen, B. R. (2005). Effect of aging on performance, muscle activation and perceived stress during mentally demanding computer tasks. Scand. J. Work Environ. Health 31 (2), 152–159. doi:10.5271/sjweh.862
Altuntas, F., and Gök, M. Ş. (2020). Technological evolution of wind energy with social network analysis. Kybernetes 50 (5), 1180–1211. doi:10.1108/k-11-2019-0761
Bai, Y., Chou, L., and Zhang, W. (2021). Industrial innovation characteristics and spatial differentiation of smart grid technology in China based on patent mining. J. Energy Storage 43, 103289. doi:10.1016/j.est.2021.103289
Campbell, G. A. (2019). The cobalt market revisited. Min. Econ. 33 (1-2), 21–28. doi:10.1007/s13563-019-00173-8
Cullen, J. M., and Allwood, J. M. (2013). Mapping the global flow of aluminum: From liquid aluminum to end-use goods. Environ. Sci. Technol. 47 (7), 3057–3064. doi:10.1021/es304256s
Dehaine, Q., Tijsseling, L. T., Glass, H. J., Törmänen, T., and Butcher, A. R. (2021). Geometallurgy of cobalt ores: A review. Miner. Eng. 160, 106656. doi:10.1016/j.mineng.2020.106656
Freeman, L. C. (1978). Centrality in social networks conceptual clarification. Soc. Netw. 1 (3), 215–239. doi:10.1016/0378-8733(78)90021-7
Fu, X., Beatty, D. N., Gaustad, G. G., Ceder, G., Roth, R., Kirchain, R. E., et al. (2020). Perspectives on cobalt supply through 2030 in the face of changing demand. Environ. Sci. Technol. 54 (5), 2985–2993. doi:10.1021/acs.est.9b04975
Garcia, J. R., do Lago, D. C. B., Cesar, D. V., and Senna, L. F. (2016). Pulsed cobalt-rich Zn–Co alloy coatings produced from citrate baths. Surf. Coatings Technol. 306, 462–472. doi:10.1016/j.surfcoat.2016.01.044
Hayes, S. M., and McCullough, E. A. (2018). Critical minerals: A review of elemental trends in comprehensive criticality studies. Resour. Policy 59, 192–199. doi:10.1016/j.resourpol.2018.06.015
He, D., Wu, D., Gao, J., Wu, X., Zeng, X., and Ding, W. (2015). Flower-like CoS with nanostructures as a new cathode-active material for rechargeable magnesium batteries. J. Power Sources 294, 643–649. doi:10.1016/j.jpowsour.2015.06.127
Horn, S., Gunn, A. G., Petavratzi, E., Shaw, R. A., Eilu, P., Törmänen, T., et al. (2021). Cobalt resources in Europe and the potential for new discoveries. Ore Geol. Rev. 130, 103915. doi:10.1016/j.oregeorev.2020.103915
Kim, K., Choi, Y., Lee, H., and Lee, J. W. (2018). Y2O3-Inserted Co-Pd/zeolite catalysts for reductive amination of polypropylene glycol. Appl. Catal. A General 568, 114–122. doi:10.1016/j.apcata.2018.09.029
Kim, K., Kang, D. W., Choi, Y., Kim, W., Lee, H., and Lee, J. W. (2020). Improved H2 utilization by Pd doping in cobalt catalysts for reductive amination of polypropylene glycol. RSC Adv. 10 (73), 45159–45169. doi:10.1039/d0ra10033a
Lee, M.-T., and Su, W.-N. (2020). Search for the developing trends by patent analysis: A case study of lithium-ion battery electrolytes. Appl. Sci. 10 (3), 952. doi:10.3390/app10030952
Lin, W. C., Chuang, C. C., Yao, C., and Tang, C. M. (2020). Effect of cobalt precursors on cobalt-hydroxyapatite used in bone regeneration and MRI. J. Dent. Res. 99 (3), 277–284. doi:10.1177/0022034519897006
Liu, S., Dong, Z., Ding, C., Wang, T., and Zhang, Y. (2020). Do you need cobalt ore? Estimating potential trade relations through link prediction. Resour. Policy 66, 101632. doi:10.1016/j.resourpol.2020.101632
Luo, Y.-h., Wei, H.-x., Tang, L.-b., Huang, Y.-d., Wang, Z.-y., He, Z.-j., et al. (2022). Nickel-rich and cobalt-free layered oxide cathode materials for lithium ion batteries. Energy Storage Mater. 50, 274–307. doi:10.1016/j.ensm.2022.05.019
Malhotra, A., Zhang, H., Beuse, M., and Schmidt, T. (2021). How do new use environments influence a technology's knowledge trajectory? A patent citation network analysis of lithium-ion battery technology. Res. Policy 50 (9), 104318. doi:10.1016/j.respol.2021.104318
McCullough, E., and Nassar, N. T. (2017). Assessment of critical minerals: Updated application of an early-warning screening methodology. Min. Econ. 30 (3), 257–272. doi:10.1007/s13563-017-0119-6
Robinson, D. K. R., Huang, L., Guo, Y., and Porter, A. L. (2013). Forecasting Innovation Pathways (FIP) for new and emerging science and technologies. Technol. Forecast. Soc. Change 80 (2), 267–285. doi:10.1016/j.techfore.2011.06.004
Safin, E., and Manteuffel, D. (2016). Advanced eigenvalue tracking of characteristic modes. IEEE Trans. Antennas Propag. 64 (7), 2628–2636. doi:10.1109/tap.2016.2556698
Saramäki, J., Onnela, J. P., Kertész, J., and Kaski, K. (2005). “Characterizing motifs in weighted complex networks,” in AIP conference proceedings (American Institute of Physics), 108–117.
Sun, X., Hao, H., Liu, Z., Zhao, F., and Song, J. (2019). Tracing global cobalt flow: 1995–2015. Resour. Conservation Recycl. 149, 45–55. doi:10.1016/j.resconrec.2019.05.009
Talapatra, S., Tang, X., Padi, M., Kim, T., Vajtai, R., Sastry, G. V. S., et al. (2009). Synthesis and characterization of cobalt–nickel alloy nanowires. J. Mat. Sci. 44 (9), 2271–2275. doi:10.1007/s10853-008-3015-1
Wang, C., Chen, X., Chen, Y., Yu, J., Cai, W., Chen, Z., et al. (2022). Accelerated design of high γ′ solvus temperature and yield strength cobalt-based superalloy based on machine learning and phase diagram. Front. Mat. 9. doi:10.3389/fmats.2022.882955
Watts, D. J., and Strogatz, S. H. (1998). Collective dynamics of ‘small-world’networks. nature 393 (6684), 440–442. doi:10.1038/30918
Yu, B., Li, Y., Nie, Y., and Mei, H. (2018). High temperature oxidation behavior of a novel cobalt-nickel-base superalloy. J. Alloys Compd. 765, 1148–1157. doi:10.1016/j.jallcom.2018.06.275
Yuan, X., and Li, X. (2020). A network analytic method for measuring patent thickets: A case of fcev technology. Technol. Forecast. Soc. Change 156, 120038. doi:10.1016/j.techfore.2020.120038
Keywords: cobalt, patent layout, key technologies, patent analysis, co-occurrence
Citation: Hua Z and Dai T (2023) Research on key cobalt technologies based on patent analysis. Front. Energy Res. 10:1005720. doi: 10.3389/fenrg.2022.1005720
Received: 28 July 2022; Accepted: 31 October 2022;
Published: 13 January 2023.
Edited by:
Xiaoqi Sun, Shenzhen University, ChinaReviewed by:
Sida Feng, Beijing University of Chemical Technology, ChinaCopyright © 2023 Hua and Dai. This is an open-access article distributed under the terms of the Creative Commons Attribution License (CC BY). The use, distribution or reproduction in other forums is permitted, provided the original author(s) and the copyright owner(s) are credited and that the original publication in this journal is cited, in accordance with accepted academic practice. No use, distribution or reproduction is permitted which does not comply with these terms.
*Correspondence: Tao Dai, ZWFnbGVkYWlAMTI2LmNvbQ==
Disclaimer: All claims expressed in this article are solely those of the authors and do not necessarily represent those of their affiliated organizations, or those of the publisher, the editors and the reviewers. Any product that may be evaluated in this article or claim that may be made by its manufacturer is not guaranteed or endorsed by the publisher.
Research integrity at Frontiers
Learn more about the work of our research integrity team to safeguard the quality of each article we publish.