- 1School of Environmental and Municipal Engineering, Qingdao University of Technology, Qingdao, China
- 2School of Energy and Power Engineering, Shandong University, Jinan, China
- 3Key Lab of Industrial Fluid Energy Conservation and Pollution Control (Qingdao University of Technology), Ministry of Education, Qingdao, China
The existence of inert gases such as N2 and CO2 in biogas will reduce the proportion of combustible components in syngas and affect the combustion and NOX formation characteristics. In this study, ANSYS CHEMKIN-PRO software combined with GRI-MECH 3.0 mechanism was used to numerically simulate the effects of different CO2 concentrations (CO2 volume ratio in biogas is 0–41.6%) on flame combustion temperature, flame propagation speed and nitrogen oxide formation of complex biogas with low calorific value. The results showed that when the combustion reaches the chemical equilibrium, the flame combustion temperature and flame propagation speed decrease with the increase of CO2 concentration, and the flame propagation speed decreases even more slowly. Meanwhile, the molar fraction of NO at chemical equilibrium decreases with the increase of CO2 concentration and the decrease is decreasing, which indicates that the effect of CO2 concentration in biogas on NO is simpler. While the molar fraction of NO2 does not change regularly with the change of CO2 concentration, the effect of CO2 concentration in biogas on NO2 is complicated. The highest molar fraction of NO2 was found at chemical equilibrium when the CO2 concentration was 33.6%, when the target was a typical low calorific value biogas.
Introduction
Biomass is a kind of clean and convenient energy with rich reserves, which is a promising green renewable energy (Zhang et al., 2005). Nowadays, direct combustion is a main utilization method for biomass (Yang et al., 2020). However, it has the disadvantages of low utilization ratio, massive content of dust and NOX in flue gas (Zhao and Su, 2019; Wang et al., 2020). To solve above issues, the biogas obtained from pyrolysis or gasification of biomass can be combusted with pulverized coal as boiler fuel, which not only reduces the consumption of coal, but also decreases the NOX emission significantly (Zhang et al., 2017). Therefore, biomass gasification is considered as a more effective, promising and valuable application mode for biomass utilization (Cao et al., 2019). However, the composition of biogas is complex, especially the existence of inert gases such as CO2 and N2 will have a great impact on the combustion characteristics of flame and the formation of NOX (Chu et al., 2021).
In recent years, many scholars have studied the effects of simple component gases on flame characteristics and NOX emission in laminar premixed combustion: Studies by many scholars have shown that the increase of H2 content will lead to higher combustion temperature and laminar flame speed, but the emission of nitrogen oxides will also increase correspondingly (Azimov et al., 2011; Wang et al., 2021). The premixed combustion of syngas under different H2/CO ratio was studied, the results suggested that increasing the H2/CO ratio led to a decrease in the temperature as well as the NO concentration near the flame (Asgari et al., 2017). The combustion characteristics of NH3/H2/air, H2/CO/syngas, propane/hydrogen/air and other mixtures have also been extensively studied (Tang et al., 2008; Nozari and Karabeyoglu, 2015; Chen and Jiang, 2021).
Although many scholars have studied the flame combustion characteristics of biogas, most of them are limited to study some kind of combustible gas, or changing the ratio of two kinds of gas in the combustible gas. A small number of people have studied the effects of inert gas on combustion characteristics and NOX emission: The effects of N2 dilution on laminar burning speed, adiabatic flame temperature, intermediate radicals and NOX emissions of methane were investigated under different equivalence ratio conditions. Results showed that under the same equivalence ratio, the mole fraction of NOX decreased as the N2 doping ratio increased (Resende et al., 2019; Chu et al., 2021). The effect of CO2 and N2 dilution on CH4/air flame by flame temperature distribution measurement and flame calculation was analyzed. At ambient temperature (298K), the specific heat capacity of N2 is 1042 J/ kg·K, H2 is 14,300 J/ kg K and that of CO2 is 839 J/ kg K. Results indicated that CO2 addition shows more significant effects on the thermal properties of flame, except for flame thickness (Zhang et al., 2016). Numerical simulation was used to study the combustion characteristics of laminar premixed biological combustion flames with different equivalence ratios (0.6–1.6) under standard conditions by using ANSYS CHEMKIN-PRO and GRI-MECH 3.0 mechanism. The results showed that the laminar flame speed has a good positive correlation with the adiabatic temperature under different equivalent ratios. The inhibition effect of CO2 is stronger than N2, which may be related to the chemical effect and heat capacity of CO2 (Sun et al., 2021). It can be seen from the above that the change of CO2 concentration has a stronger influence on combustion characteristics than N2, and most scholars have studied the influence of inert gas on combustion characteristics of simple components (methane, methane-air mixture, etc.). The research on combustion characteristics and nitrogen oxide emission of complex biogas which close to actual biogas components by using simulation software is relatively rare, and further research is necessary.
Because CHEMKIN software has relatively perfect mechanism files for solving complex chemical reaction problems, it is often used to study flame combustion characteristics and NOX conversion mechanism. It was proved to be very effective to study the combustion characteristics and NOX emissions by using the PREMIX code of CHEMKIN(Chen et al., 2011; Gong et al., 2018). CHEMKIN and GRI-MECH 3.0 chemical kinetic models were used to simulate combustion process, which has outstanding advantages in predicting combustion chamber pressure, temperature, distribution of main combustion species and formation of nitrogen oxides (Mansha et al., 2010). Chemical dynamics simulation with hedged flame model in CHEMKIN software was carried out, and the influence mechanism of water vapor on combustion flame characteristics was analyzed, the simulated data were in good agreement with the experimental results. (Cui et al., 2020). Wilk, M. studied the combustion characteristics of carbonized biomass by TGA (Thermo Gravimetric Analyzer) analysis and CHEMKIN calculation, and the results showed that the change of gas products calculated by CHEMKIN during combustion was consistent with the results of TGA analysis (Wilk et al., 2017). The above research shows that the simulation of combustion characteristics and nitrogen oxides emission by CHEMKIN software is in good agreement with the actual situation, so it can be used to study the combustion mechanism.
The influence of one or more combustible gases on flame combustion characteristics and NOX formation mechanism has been extensively studied, and the effects of nitrogen and carbon dioxide dilution on combustion characteristics are well explored, studies on combustion characteristics and NOX formation mechanism of syngas flame with complex components under different inert gas concentrations are relatively scarce seldom. Therefore, in this study, typical low calorific value biogas (Li, 2019) which is closer to the real biogas composition (the composition of typical low calorific value biogas is shown in Table 2 of Section 2.1) was selected to study one-dimensional laminar premixed flame, and CHENKIN software was used to simulate the influence of CO2 concentration change on flame combustion characteristics and the formation of nitrogen oxides, aiming at providing help for the design of biomass gas burner and theoretical support for emission reduction of biomass gas boiler.
Methods
Mechanism
In this study, based on CHEMKIN software, the premixed laminar flame characteristics and NOX conversion mechanism of material gas were studied. All calculations in CHEMKIN software are based on mechanism files. This simulation uses two mechanism files. One is the Flame -speed-parameter-study case in CHEMKIN software, which contains C, H, O and N elements. The components include all the components in the biogas, which can well simulate the combustion process of the selected biogas. Since the generation of NOX is not involved in this mechanism document, GRI-MECH3.0 methane mechanism document is selected for the concentration variation law of NO and NO2. The above two mechanism documents can be used to complete the combustion characteristics of the flame and NOX conversion mechanism.
Assuming that the gas inlet is a one-dimensional steady flow, the governing equation can be simplified as follows (Kuo, 2005; Sun et al., 2021):
Continuity
In the above formula,
Energy
where x represents the one-dimensional coordinates, T represents the temperature, λ is the heat conductivity of the gas, Cp represents the heat capacity of the mixed gas at constant pressure, Cpk expresses the kth species heat capacity at constant pressure, Yk denotes the kth species mass fraction, Vk indicates the diffusion rate of the kth gas, hk indicates the specific enthalpy of the kth gas, Wk represents molecular weight of the kth species,
Species
Equation of state
In Eq. 4, P represents the pressure in the x direction, R is the general constant of gas, and
Material
Table 1 shows the main gas components of different types of biomass gasification processes in the references (Wu et al., 2009; Kim et al., 2011; Miao et al., 2013; Wang et al., 2015). It can be noticed that the variation range of CO2 concentration in biogas is 0–30%. In addition, related reference also showed that when the proportion of CO2 is about 40% (Li, 2019) the fuel has better combustion characteristics and low NOX generation. It can be seen that the influence range of CO2 concentration on combustion characteristics and NOX is about 0–40%, so we choose the biogas synthesized in the laboratory, that is, the typical low calorific value biogas (the composition of typical low calorific value biogas is shown in Table 2) as the control group for numerical simulation, and study it in the range of CO2 concentration of 0–41.6%.
Calculation Conditions
To ensure that the flame is close to the real burning condition, the flame speed calculator simulates a freely propagating flame at 298 K and 1 atm. At the equivalent ratio of 1.0, the laminar flame is the fastest and the flame is stable (Liu et al., 2020), so the simulation is performed at the equivalent ratio of 1.0. The initial flow rate parameter of the biogas was set to 50 cm/ s. In the simulation process, the proportion of other gases remains unchanged, only the concentration of CO2 to be studied will be changed. Table 3 shows the biogas composition under different CO2 volume ratios.
Results and Discussion
Effect of CO2 Concentration on Flame Combustion Temperature
CO2 has a high specific heat capacity and a relatively high heat absorption capacity, which has a certain influence on the flame combustion temperature, and the flame combustion temperature will have an influence on the formation of some temperature-sensitive intermediate products (Zhang, 2020). Reasonable control of the combustion temperature ensures that the fuel is fully burned, and the generation of intermediate products can be controlled to reduce the formation of pollutants while ensuring combustion efficiency.
Figure 1 illustrates the variation curve of flame temperature with distance for different CO2 compositions simulated by CHEMKIN. For typical low calorific value biogas, when the volume ratio of CO2 is 33.60%, it can be used as a control group to compare the influence of CO2 concentration change on flame combustion temperature. It can be seen from Figure 1 that with the increase of the distance and the continuation of the reaction, the temperature of the flame keeps rising. When the combustion reaches chemical equilibrium, the final temperature of the reaction keeps decreasing with the increase of CO2 concentration. As the percentage of CO2 increases from 0 to 41.6%, the flame temperature decreases by about 395 K, which can be explained by the fact that the concentration of small molecular intermediates such as H, O and OH in the flame decreases as the concentration of CO2 increases, and the maximum flame temperature falls with the increase of CO2, while the high temperature area also decreases (Cao and Zhu, 2012). The research results of Park et al. showed that with the increase of CO2 concentration from 0 to 0.4, the flame burning temperature decreases by about 400K (Park et al., 2007), which is highly consistent with our simulation results. The effect of CO2 concentration on combustion temperature provides a theoretical basis for adding CO2 as a diluent to the fuel.
Effect of CO2 Concentration on Flame Propagation Speed
The flame propagation speed of laminar flow can fully reflect the fuel activity, the basic characteristics of heat and mass transfer of combustion reaction, and the tempering characteristics of flame, etc (De Goey et al., 2011). However, the addition of CO2 has a great influence on the flame propagation speed, so studying the influence of CO2 concentration on the flame propagation speed can provide great help to the design of burner. Therefore, the flame propagation speed was simulated numerically using CHEMKIN software, and the variation curve of flame propagation speed with CO2 concentration was obtained as shown in Figure 2. It can be seen that the flame propagation speed decreases with the increase of the volume ratio of CO2, the flame propagation speed at 40% CO2 concentration is 64.2% lower than that at 10%, which is consistent with the results of Hinton and Cohe (Cohe et al., 2009; Hinton and Stone, 2014). In addition, the simulation results are in good agreement with the experimental results of Maria Mitu (Mitu et al., 2017). With the increase of CO2 concentration, the laminar flame combustion velocity decreases from the initial 35 cm/s to about 10 cm/s.
The reason why the existence of CO2 reduces the flame propagation speed is that although CO2 is an inert gas, it also participates in the primitive reaction CO2+H→CO + OH(Glarborg and Bentzen, 2008), which inhibits the oxidation of CO. It will also compete with the branch reaction of the main chain for H element, leading to the reduction of H group and OH group (Huang, 2011), and these two free radicals have a great promotion effect on the flame propagation speed. Consequently, the volume ratio of CO2 in syngas should be controlled to ensure a reasonable combustion rate.
Effect of CO2 Concentration on the Formation of NO and NO2
The emission of nitrogen oxides is a problem to be considered in small-capacity boilers, because the nitrogen oxides produced by any fossil fuel combustion will lead to the formation of acid rain and photochemical smog, which are important causes of air pollution (Werle, 2015). Thus, studying the effect of CO2 concentration on NOX formation can reduce the pollutant generation at the source and can provide theoretical support for reducing NOX emissions for biomass gas boilers.
Figure 3A shows the curve of NO mole fraction with distance under different CO2 concentration, and Figure 3B presents the variation curve of NO mole fraction with CO2 concentration in chemical equilibrium. From the above two figures, it can be observed that the molar fraction of NO keeps increasing with the distance and the reaction proceeds continuously. When the biogas is devoid of CO2, the molar fraction of NO increases fastest with distance, and when the combustion reaches chemical equilibrium, the proportion of NO produced is the largest, and the molar fraction of NO produced at equilibrium declines as the volume proportion of CO2 in the fuel increases. This is in agreement with the findings of Tang (Tang et al., 2009). The influence of CO2 concentration on NO generation was studied by using a tube furnace with Li of 1373K and equivalent ratio of 1. The experimental results showed that with the increase of CO2 concentration, NO concentration decreases from 14.8 to 5.9 ppm (Li, 2019), which is consistent with our simulation results. Because of the different gas composition and the influence of experimental operation process, the specific values are different. Liu and Dong et al. demonstrated that CO2+H→CO + OH and CO2+CH→HCO + CO are the main chemical reaction in which CO2 participates, while these two reactions indirectly affect the production of the NO precursor HCN, thus inhibiting the production of NOX (Liu et al., 2001; Dong et al., 2014).
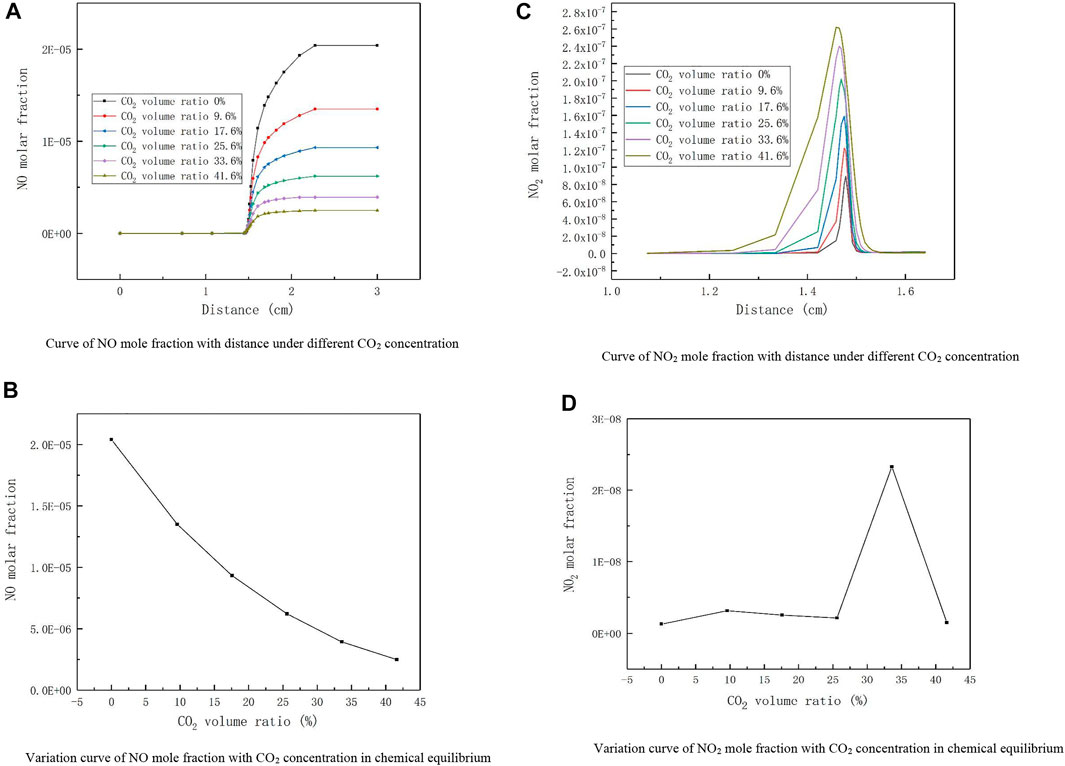
FIGURE 3. Distribution curve of nitrogen oxides under different CO2 concentrations. (A) Curve of NO mole fraction with distance under different CO2 concentration. (B) Variation curve of NO mole fraction with CO2 concentration in chemical equilibrium. (C) Curve of NO2 mole fraction with distance under different CO2 concentration. (D) Variation curve of NO2 mole fraction with CO2 concentration in chemical equilibrium.
Figure 3C shows the curve of NO2 mole fraction with distance under different CO2 concentration, and Figure 3D presents the variation curve of NO2 mole fraction with CO2 concentration in chemical equilibrium. From Figure 3C, it can be found that the molar fraction of NO2 at the peak is highest when the volumetric proportion of CO2 in the biogas is 41.6%, with a value of 2.06E-07. As the volumetric proportion of CO2 in the biogas decreases, the molar fraction of NO2 at the peak also reduces. As can be noticed from Figure 3D, the molar fraction of NO2 produced at equilibrium is maximum for the selected typical low calorific value biogas whose CO2 concentration is around 33.6%, and the molar fraction of NO2 produced is significantly lower in other cases. Thus, it can be seen that the effect of the variation of CO2 concentration on NO2 is very complex. This may be due to the fact that the concentration of H, O, and HO2 changes with different CO2 concentrations, leading to unstable formation of NO2(Glarborg and Bentzen, 2008). Since the combustion mechanism is very complex the exact cause needs to be further verified.
Conclusions
In this paper, the influence of CO2 concentration on flame combustion characteristics and NOX formation was studied by using CHEMKIN software. The main research results are as follows.
(1) With the increase of CO2 concentration, the flame combustion temperature and flame propagation speed gradually decrease, and the flame propagation speed decreases even more slowly. Compared with syngas without CO2, when the CO2 concentration in biogas is 41.6%, the flame temperature is 395 K lower and the flame propagation speed decreases by 64.2%. It can be seen that the existence of CO2 in biogas not only reduces the combustion temperature of the flame, but also greatly reduces the propagation speed of the laminar flame.
(2) After the combustion reaches the chemical equilibrium, the molar fraction of NO in the product decreases with the increase of CO2, while the molar fraction of NO2 does not show regularity with the change of CO2 concentration.When the concentration of carbon dioxide is 33.6%, that is, when the research object is typical low calorific value biogas, the mole fraction of nitrogen dioxide in chemical equilibrium is the highest.
Data Availability Statement
The original contributions presented in the study are included in the article/supplementary material, further inquiries can be directed to the corresponding author.
Author Contributions
JM performed the data analysis and wrote the manuscript. CQ performed the simulations using software. SL provided ideas and directed the analysis of the data. ZZ provided constructive comments on revisions to the manuscript.
Funding
This work supported by the National Key Research and Development of China (2020YFD1100302), 2020 science and technology project of Qingdao West Coast New Area (Science and technology benefiting the people) (2020–99).
Conflict of Interest
The authors declare that the research was conducted in the absence of any commercial or financial relationships that could be construed as a potential conflict of interest.
Publisher’s Note
All claims expressed in this article are solely those of the authors and do not necessarily represent those of their affiliated organizations, or those of the publisher, the editors and the reviewers. Any product that may be evaluated in this article, or claim that may be made by its manufacturer, is not guaranteed or endorsed by the publisher.
References
Asgari, N., Ahmed, S. F., Farouk, T. I., and Padak, B. (2017). NOx Formation in post-flame Gases from Syngas/air Combustion at Atmospheric Pressure. Int. J. Hydrogen Energ. 42 (38), 24569–24579. doi:10.1016/j.ijhydene.2017.08.017
Azimov, U., Tomita, E., Kawahara, N., and Harada, Y. (2011). Effect of Syngas Composition on Combustion and Exhaust Emission Characteristics in a Pilot-Ignited Dual-Fuel Engine Operated in PREMIER Combustion Mode. Int. J. Hydrogen Energ. 36 (18), 11985–11996. doi:10.1016/j.ijhydene.2011.04.192
Cao, Y., Wang, Q., Du, J., and Chen, J. (2019). Oxygen-enriched Air Gasification of Biomass Materials for High-Quality Syngas Production. Energ. Convers. Manage. 199, 111628. doi:10.1016/j.enconman.2019.05.054
Cao, Z., and Zhu, T. (2012). Effects of CO2 Dilution on Methane Ignition in Moderate or Intense Low-Oxygen Dilution (MILD) Combustion: A Numerical Study. Chin. J. Chem. Eng. 20 (4), 701–709. doi:10.1016/S1004-9541(11)60238-3
Chen, G.-B., Li, Y.-H., Cheng, T.-S., Hsu, H.-W., and Chao, Y.-C. (2011). Effects of Hydrogen Peroxide on Combustion Enhancement of Premixed Methane/air Flames. Int. J. Hydrogen Energ. 36 (23), 15414–15426. doi:10.1016/j.ijhydene.2011.07.074
Chen, Z., and Jiang, Y. (2021). Numerical Investigation of the Effects of H2/CO/syngas Additions on Laminar Premixed Combustion Characteristics of NH3/air Flame. Int. J. Hydrogen Energ. 46 (21), 12016–12030. doi:10.1016/j.ijhydene.2021.01.054
Chu, H., Xiang, L., Meng, S., Dong, W., Gu, M., and Li, Z. (2021). Effects of N2 Dilution on Laminar Burning Velocity, Combustion Characteristics and NOx Emissions of Rich CH4-Air Premixed Flames. Fuel 284, 119017. doi:10.1016/j.fuel.2020.119017
Cohé, C., Chauveau, C., Gökalp, I., and Kurtuluş, D. F. (2009). CO2 Addition and Pressure Effects on Laminar and Turbulent Lean Premixed CH4 Air Flames. Proc. Combustion Inst. 32, 1803–1810. doi:10.1016/j.proci.2008.06.181
Cui, G., Dong, Z., Wang, S., Xing, X., Shan, T., and Li, Z. (2020). Effect of the Water on the Flame Characteristics of Methane Hydrate Combustion. Appl. Energ. 259, 114205. doi:10.1016/j.apenergy.2019.114205
De Goey, L. P. H., Van Oijen, J. A., Kornilov, V. N., and ten Thije Boonkkamp, J. H. M. (2011). Propagation, Dynamics and Control of Laminar Premixed Flames. Proc. Combustion Inst. 33 (1), 863–886. doi:10.1016/j.proci.2010.09.006
Dong, H., Zhang, Y., and Gu, Z. (2014). Effects of Diluents on NO X Formation in Coflow CH4/air Diffusion Flames. Korean J. Chem. Eng. 31 (6), 1002–1007. doi:10.1007/s11814-014-0035-1
Glarborg, P., and Bentzen, L. L. B. (2008). Chemical Effects of a High CO2 Concentration in Oxy-Fuel Combustion of Methane. Energy Fuels 22 (1), 291–296. doi:10.1021/ef7005854
Gong, C., Li, Z., Li, D., Liu, J., Si, X., Yu, J., et al. (2018). Numerical Investigation of Hydrogen Addition Effects on Methanol-Air Mixtures Combustion in Premixed Laminar Flames under Lean Burn Conditions. Renew. Energ. 127, 56–63. doi:10.1016/j.renene.2018.04.047
Hinton, N., and Stone, R. (2014). Laminar Burning Velocity Measurements of Methane and Carbon Dioxide Mixtures (Biogas) over Wide Ranging Temperatures and Pressures. Fuel 116, 743–750. doi:10.1016/j.fuel.2013.08.069
Huang, M. (2011). Research on Biomass Gasification and Co-combustion Processmaster's Thesis. Zhengzhou, Henan: North China University of Water Resources and Electric Power.
Kim, J.-W., Mun, T.-Y., Kim, J.-O., and Kim, J.-S. (2011). Air Gasification of Mixed Plastic Wastes Using a Two-Stage Gasifier for the Production of Producer Gas with Low Tar and a High Caloric Value. Fuel 90 (6), 2266–2272. doi:10.1016/j.fuel.2011.02.021
Li, Y. (2019). “Study on Combustion Characteristics and Numerical Simulation of Biomass Gasification Gas,” (Zhejiang: Zhejiang University). master's thesis.
Liu, F., Guo, H., Smallwood, G. J., and Gülder, Ö. L. (2001). The Chemical Effects of Carbon Dioxide as an Additive in an Ethylene Diffusion Flame: Implications for Soot and NOx Formation. Combustion and Flame 125 (1), 778–787. doi:10.1016/S0010-2180(00)00241-8
Liu, S., Pei, H., Wang, Z., Li, Y., and Yao, H. X. (2020). Laminar Combustion Characteristics of Premixed Shale Gas and Air Flames. J. Energ. Eng. 146 (3), 04020014. doi:10.1061/(Asce)Ey.1943-7897.0000669
Mansha, M., Saleemi, A. R., and Ghauri, B. M. (2010). Kinetic Models of Natural Gas Combustion in an Internal Combustion Engine. J. Nat. Gas Chem. 19 (1), 6–14. doi:10.1016/S1003-9953(09)60024-4
Miao, Q., Zhu, J., Barghi, S., Wu, C., Yin, X., and Zhou, Z. (2013). Modeling Biomass Gasification in Circulating Fluidized Beds. Renew. Energ. 50, 655–661. doi:10.1016/j.renene.2012.08.020
Mitu, M., Giurcan, V., Razus, D., and Oancea, D. (2017). Inert Gas Influence on the Laminar Burning Velocity of Methane-Air Mixtures. J. Hazard. Mater. 321, 440–448. doi:10.1016/j.jhazmat.2016.09.033
Nozari, H., and Karabeyoğlu, A. (2015). Numerical Study of Combustion Characteristics of Ammonia as a Renewable Fuel and Establishment of Reduced Reaction Mechanisms. Fuel 159, 223–233. doi:10.1016/j.fuel.2015.06.075
Park, J., Park, J. S., Kim, H. P., Kim, J. S., Kim, S. C., Choi, J. G., et al. (2007). NO Emission Behavior in Oxy-Fuel Combustion Recirculated with Carbon Dioxide. Energy Fuels 21, 121–129. doi:10.1021/ef060309p
Resende, P. R., Afonso, A., Pinho, C., and Ayoobi, M. (2019). Impacts of Dilution on Hydrogen Combustion Characteristics and NOx Emissions. J. Heat Transfer-Transactions Asme 141 (1), 012003. doi:10.1115/1.4041623
Sun, H., Li, R., Huang, M., Li, Z., and Xu, J. (2021). Numerical Simulations of the Influence of Inert Gases (N2/CO2) on Combustion Characteristics of Laminar-Premixed Biosyngas Flame. ACS Omega 6 (22), 14585–14597. doi:10.1021/acsomega.1c01729
Tang, C., Huang, Z., Jin, C., He, J., Wang, J., Wang, X., et al. (2008). Laminar Burning Velocities and Combustion Characteristics of Propane-Hydrogen-Air Premixed Flames. Int. J. Hydrogen Energ. 33 (18), 4906–4914. doi:10.1016/j.ijhydene.2008.06.063
Tang, G. T., Lv, J. F., Yue, G. X., and Zhang, H. (2009). Effects of CO2 Dilution on NOX Formation Characteristics in Syngas Diffusion Flames. J. Combustion Sci. Tech. 15 (03), 226–231. 1006-8740(2009)03-0226-06.
Wang, X., Zhu, Y., Hu, Z., Zhang, L., Yang, S., Ruan, R., et al. (2020). Characteristics of Ash and Slag from Four Biomass-Fired Power Plants: Ash/slag Ratio, Unburned Carbon, Leaching of Major and Trace Elements. Energ. Convers. Manage. 214, 112897. doi:10.1016/j.enconman.2020.112897
Wang, Y., Zhou, X., and Liu, L. (2021). Theoretical Investigation of the Combustion Performance of Ammonia/hydrogen Mixtures on a marine Diesel Engine. Int. J. Hydrogen Energ. 46 (28), 14805–14812. doi:10.1016/j.ijhydene.2021.01.233
Wang, Z., He, T., Qin, J., Wu, J., Li, J., Zi, Z., et al. (2015). Gasification of Biomass with Oxygen-Enriched Air in a Pilot Scale Two-Stage Gasifier. Fuel 150, 386–393. doi:10.1016/j.fuel.2015.02.056
Werle, S. (2015). Nitrogen Oxides Emission Reduction Using Sewage Sludge Gasification Gas Reburning Process/Obniżenie Emisji Tlenków Azotu Przy Użyciu Procesu Reburningu Gazem Ze Zgazowania Osadów Ściekowych. Ecol. Chem. Eng. S-Chemia Inzynieria Ekologiczna S 22 (1), 83–94. doi:10.1515/eces-2015-0005
Wilk, M., Magdziarz, A., Gajek, M., Zajemska, M., Jayaraman, K., and Gokalp, I. (2017). Combustion and Kinetic Parameters Estimation of Torrefied pine, acacia and Miscanthus Giganteus Using Experimental and Modelling Techniques. Bioresour. Tech. 243, 304–314. doi:10.1016/j.biortech.2017.06.116
Wu, C.-z., Yin, X.-l., Ma, L.-l., Zhou, Z.-q., and Chen, H.-p. (2009). Operational Characteristics of a 1.2-MW Biomass Gasification and Power Generation Plant. Biotechnol. Adv. 27 (5), 588–592. doi:10.1016/j.biotechadv.2009.04.020
Yang, S., Wang, H. Y., Zhang, J., Song, C. D., Ding, K., and Ma, Y. H. (2020). General Situation and prospect of Biomass Energy Development and Utilization. Technology Wind (22), 193–194. doi:10.19392/j.cnki.1671-7341.202022158
Zhang, C., Hu, G., Liao, S., Cheng, Q., Xiang, C., and Yuan, C. (2016). Comparative Study on the Effects of Nitrogen and Carbon Dioxide on Methane/air Flames. Energy 106 (Jul.1), 431–442. doi:10.1016/j.energy.2016.03.087
Zhang, L. (2020).Effect of CO2 Addition on Natural Gas Combustion Process and NO Formation. Heilongjiang: Harbin Institute of Technology. doctoral thesis.
Zhang, M., Yuan, Y., and Liu, Y. (2005). Research on Biomass Waste Combustion Technologies. Energ. Res. Inf. 21, 15–20. doi:10.13259/j.cnki.eri.2005.01.003
Zhang, X., Wen, M., Ding, Q., and Liu, Y. (2017). Numerical Simulation Study on the Boiler Combustion Performance for Biomass Gas Co-firing with Pulverized Coal. J. Eng. Therm. Energ. Power 32, 82–87. doi:10.16146/j.cnki.rndlgc.2017.03.013
Keywords: simulation, biogas, CO2 concentration, combustion characteristics, NOX
Citation: Ma J, Qi C, Luo S and Zuo Z (2022) Numerical Simulation of the Influence of CO2 on the Combustion Characteristics and NOX of Biogas. Front. Energy Res. 9:811037. doi: 10.3389/fenrg.2021.811037
Received: 08 November 2021; Accepted: 14 December 2021;
Published: 06 January 2022.
Edited by:
Chuang Wen, University of Exeter, United KingdomReviewed by:
Pengfei Li, Huazhong University of Science and Technology, ChinaJo-Han Ng, University of Southampton Malaysia, Malaysia
Grzegorz Przybyła, Silesian University of Technology, Poland
Copyright © 2022 Ma, Qi, Luo and Zuo. This is an open-access article distributed under the terms of the Creative Commons Attribution License (CC BY). The use, distribution or reproduction in other forums is permitted, provided the original author(s) and the copyright owner(s) are credited and that the original publication in this journal is cited, in accordance with accepted academic practice. No use, distribution or reproduction is permitted which does not comply with these terms.
*Correspondence: Siyi Luo, bHVvc2l5aTY2NkAxMjYuY29t