- School of Mechanical and Automotive Engineering, Fujian University of Technology, Fuzhou, China
Solar selective absorbing coatings (SSAC) harvest solar energy in the form of thermal energy. Traditional metal-rich SSACs like cermet-based coatings and semiconductor–metal tandems usually exhibit both a high solar absorptance and a low thermal emittance; however, metal nanoparticles can easily oxidize or diffuse at high temperature. Different from these SSACs, the all-ceramic SSACs can keep the superior optical performance at high temperatures by restraining oxidation and metal element diffusion. Besides, the facile and inexpensive fabrication of the all-ceramic SSACs makes it possible for commercial applications. These SSACs are usually a regular combination of transition-metal carbides and nitrides, which show great thermal stability and optical properties simultaneously. The structure design of the SSACs will affect the element diffusion, element oxidation, phase transition, as well as the spectral selectivity obviously. In this article, we review the structure designs of all-ceramic SSACs, and the optical properties and thermal stability of the all-ceramic SSACs in the latest literature are also compared. The purpose of this review is to identify the optimal structure design of the all-ceramic SSAC, and we also present an outlook for the structure design strategy for all-ceramic SSACs with high photothermal conversion efficiency and thermal stability.
Introduction
Solar selective absorbing coatings (SSACs) harvest solar energy in the form of heat. Due to the abundant solar energy on earth, the application of the SSACs is a promising way to protect the environment by reducing the usage of fossil fuels. Traditionally, the captured solar energy was used in households like water warming and water purification under a low operating temperature of the SSACs (Sharma et al., 2017). To meet the demand of increasing energy consumption, in recent years, increased attention has been focused on the industrial applications of the SSACs at high temperature through Concentrating solar power (CSP) plants, such as central receiver (or “power tower”), solar dishes, solar thermoelectric generators (STEGs), or solar thermophotovoltaics (STPVs) (Cao et al., 2014). In these situations, the SSACs are working under high temperature to obtain high efficiency.
Concentrating solar power (CSP) plants are known as high-temperature solar–thermal systems and are widely used in power supplying. For example, in the “power tower” plant, a collector coated with SSACs is heated to be more than 550°C to melt the salt (usually 60% KNO3 + 40% NaNO3), and then the molten salt is used to produce superheated steam for power generation by heat exchange, as shown in Figure 1A. Theoretically, the maximum efficiency of the “power tower” can reach 85% when the system operates at ∼2,200°C. In fact, the “power tower” falls short of this efficiency for a number of reasons, such as the limitation of the thermal stability of the SSACs (Kraemer et al., 2016).
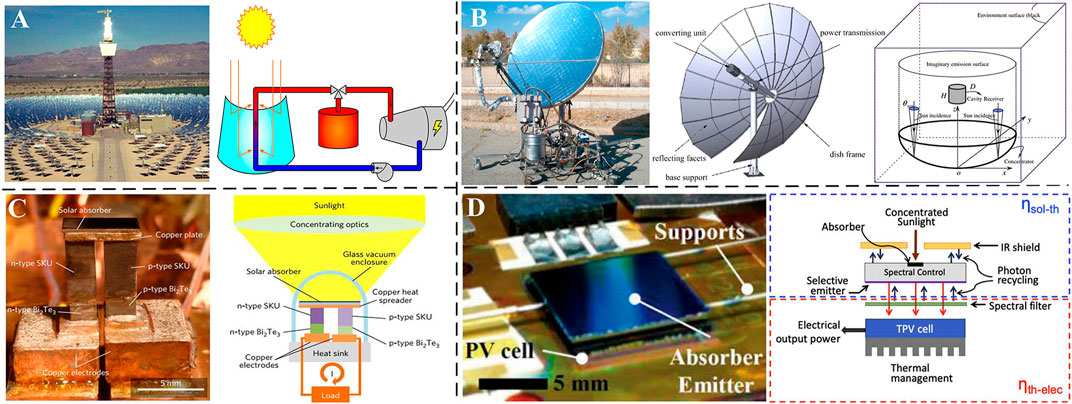
FIGURE 1. An overview of the application of solar selective absorbing coatings (SSACs) at high temperatures. Photographs and illustrations of (A) “Power tower” plant (adapted from Weinstein et al., 2015). (B) Solar dishes (adapted from Mao et al., 2014; Hijazi et al., 2016; Loni et al., 2018). (C) Solar thermoelectric generators (STEGs) (adapted from Kraemer et al., 2016). (D) Solar thermophotovoltaics (STPVs) (adapted from Wang et al., 2019; Bhatt and GUPTA, 2020).
Similar to the “power tower” plant, solar dishes consist of dishes with an array of mirrors and a receiver as shown in Figure 1B. The cylinder receiver is located at the focal plane of the parabolic dish system. Capacities of parabolic dish plants are in the range of 0.01–0.4 MW, and the operating temperatures are in the range of 250–700°C. To further improve the power of the solar dishes, the operating temperature of the absorbers should be increased.
With the emergence of high-temperature SSACs, the solar selective absorber plants can also be used in power generation by STEGs (Weinstein et al., 2015). In STEGs, the heat generated by SSACs is converted to electricity utilizing a thermoelectric device, as shown in Figure 1C. Thermoelectric devices generate a voltage difference when subjected to a temperature gradient due to the Seebeck effect. When the thermoelectric device is put in a closed circuit, then the electrical power is generated. The efficiency of the thermoelectric device relies on the hot-side temperature, which is associated with the temperature of the SSACs due to the Carnot efficiency. Thus, solar irradiance of more than 200 kWm−2 is needed to support a temperature of more than 600°C for the SSACs.
The heat generated by the SSACs could also be transferred to electrical power by the STPV via a single-junction thermophotovoltaic (TPV) cell as shown in Figure 1D. The utilizing of photon spectrum instead of direct incident sunlight enlarge the usage scale of solar energy. Since electrical power relies on conversion of thermal radiation to electricity, high-efficiency STPV devices require SSACs with high efficiency and thermal stability at high temperatures (>1273 K) (Shimizu et al., 2018).
Compared with solar photovoltaic technology, the solar photothermic technology based on SSACs has higher efficiency (up to 85%) in energy conversion due to a wider utilization of the solar spectrum (Li et al., 2018). As mentioned, the operation temperature of the solar photothermic plants may reach beyond 700°C, approaching the melting point of some metals.
Traditional SSACs like cermets [W-Ni-Al2O3(Cao et al., 2015), WTi-Al2O3(Wang et al., 2017), Ta-SiO2(Bilokur et al., 2020)], semiconductor–metal tandems [Ti/TaC/Al2O3(Kondaiah et al., 2019), Cr2O3/Cr/Cr2O(Khamlich et al., 2020)], multiple metal/ceramic nanofilms [Cu/Si/Al2O3 (El-Mahallawy et al., 2018), Ti/SiO2/Cu (Hu et al., 2018)], and photonic crystals [HfO2/Al2O3(Chou et al., 2014), HfO2/Ta (Rinnerbauer et al., 2015)] have been extensively investigated in the past. However, large-scale industrial applications of these SSACs are impeded by the relatively low solar-to-heat conversion efficiency and their low thermal stability at high temperature. For example, a typical Cu diffusion emerged and caused the performance degradation of Cu-SiO2/Cu tandems above 400°C in a vacuum (Cao et al., 2014). Photonic crystals consist of thicker refractory metals (e.g., W, Ta, Ni, and TiN) and usually show better thermal stability but also suffer from a high IR emittance (Chou et al., 2014; Li et al., 2015). For example, Rinnerbauer et al. designed a Ta-based metallic photonic crystal SSACs by the finite-difference-time-domain method (FDTD) as well as the Fourier modal method. The minimum thermal emittance was 0.256 at 1,000 K and 100 suns (Rinnerbauer et al., 2014). Jiang et al. prepared TiN-based SSACs with TiN nanocavity and SiO2 filled in as dielectric (Jiang and YANG, 2017). The emittance of this encapsulated nanocavity was ∼ 0.25 before annealing and increased to ∼ 0.35 after annealing at 1,273 K for 2 h in Argon. As a common defect, the mismatch in the thermal expansion coefficients of the metal and substrate material, as well as the oxidation of the metal, will result in SSAC fatigue and delamination after many high-temperature thermal cycles (Tian et al., 2020).
Furthermore, cost-effective scaling of the SSACs is another barrier in meeting the large-scale requirements of potential industrial applications. In the process of large-scale production, special attention should be paid to the thermal stability, durability, and production cost. The optophysical properties of coating must remain stable under long-term operation at elevated temperature, repeated thermal cycling, air exposure, UV radiation, etc. Multiple-layer SSACs consisting of all-ceramic intermediate spacer could be a feasible candidate to address these challenges. Thus, in this article, we review the structure designs of all-ceramic SSACs, and the optical properties and thermal stability of the all-ceramic SSACs in the latest literature are also compared.
Mechanism of Spectral Selectivity of the Solar Selective Absorbing Coatings
Near 98% of the solar radiation energy concentrated in the range of ultraviolet (UV), visible (VIS), and near-infrared (NIR) spectrum with a wavelength from 0.25 to 2.5 μm. Meanwhile, the wavelength of spontaneous blackbody irradiation is in the range of 2.5–25 μm. The ideal SSACs should have high solar absorptance in the UV-VIS-NIR spectrum range and low emittance in the infrared (IR) spectrum range, thus, leading to preservation of solar energy. Assuming the only loss is radiation from the absorbing surface, and the convective losses go to zero, the performance of the SSACs can be defined as the conversion efficiency η, given by
where
The average solar absorptance (
where θ is the incident angle of sunlight, λ is the wavelength of solar radiation, G(λ) is the incident solar intensity at an atmospheric mass of 1.5 (AM 1.5), and Rλ is the spectral reflectance.
The average thermal emittance (
where C1 and C2 are 3.743 × 10−16 Wm2 and 1.4387 × 10−2 mK, respectively, and Ib(λ, T) is the blackbody intensity given by Plank’s blackbody radiation (Eq. 4).
The “spectral selectivity” of the SSACs guarantees a high solar absorptance together with a low thermal emittance, which warrants an efficient utilization of the solar energy. The “spectral selectivity” of the SSACs benefits their applications in high-temperature circumstances like power generation and thermal energy storage. Conversely, despite high solar absorptance, the ordinary black absorbers usually exhibit high thermal emittance, which leads to energy loss; thus, they are only applicable in low-temperature circumstances like seawater desalination and water heating (Wang et al., 2020a). Some literature revealed that optical properties of the solar absorbers can be tuned by modifying the geometric parameters of the nanostructures (Wang et al., 2020b; Wang et al., 2020c).
Design and Performance of the All-Ceramic Solar Selective Absorbing Coatings
To enable the application of SSACs at high temperature, in recent years, research attention has been focused on improving the efficiency and durability of SSACs in vacuum and in air (Xu et al., 2020). Among all the SSACs, all-ceramic SSACs showed a promising solar–thermal conversion efficiency as well as high thermal stability. The main task of this section is to review the structure design and performance of the all-ceramic SSACs in the latest literature.
Boride, Carbide, Oxide, Nitride, and Oxynitride-Based Solar Selective Absorbing Coatings
Khamlich et al. prepared Cr2O3-based SSACs by the aqueous chemical growth (ACG) method (Khamlich et al., 2013). The structure showed a spectrum absorptance of 0.9 and thermal emittance of 0.28. Despite the thermal stability at 500°C in hydrogen for 2 h, the relatively high emittance restrains it to be potential SSACs. Similar reports were found in the commercial silicone-based Pyromark 2,500 paints, which show a nonlinear thermal emittance from ∼ 0.8 at 100°C to ∼ 0.89 at 1,000°C. Thus, it can be concluded that SSACs with a single-layer absorber have poor solar selectivity due to high thermal losses in the IR. Reflective layers like ZrN and TiN should be employed to reduce thermal emittance.
To further optimize the thermal stability of SSACs, high-temperature stable transition metal boride, carbide, oxide, nitride, and oxynitride-based materials (TM-B/C/O/N/ON, TM=Ti, Hf, Zr, Cr, etc.) with a multiple-layer structure are utilized as the intermediate spacers (Qiu et al., 2019; Kumar and DIXIT, 2020; Xiang et al., 2021). Qiu et al. prepared a novel SS/HfB2-ZrB2/ZrB2/Al2O3 multilayer SSACs by magnetron sputtering method (Qiu et al., 2020). Optical constants of the single layer are optimized by ellipsometric studies and CODE software. The solar absorptance slightly decreased from 0.93 to 0.91 after five cycles of cold (−70°C) and thermal (400°C) alternate experiment in air. It is worth to note that this structure contained a metal substrate, thus, leading to a low thermal emittance of 0.05. Similar SSACs were also prepared by Gao et al., such as SS/TiC/Al2O3, SS/TiN/Al2O3, SS/TiC-WC/Al2O3, etc. (Gao et al., 2016; Gao et al., 2017a; Gao et al., 2017b). Transition metal boride and nitride combined with a special structure design may benefit the solar energy conversion efficiency of the SSACs at high temperature. Dan et al. prepared a TiB2/TiB(N)/Si3N4 structure by DC and RF magnetron sputtering (Dan et al., 2018). This structure indicated a solar absorption of 0.964 and a moderate thermal emittance of 0.18 at 82°C. The attainment of excellent spectral selectivity could be attributed to the systematic variation or gradient in refractive index and extinction coefficient throughout the multilayer stack as shown in Figure 2A.
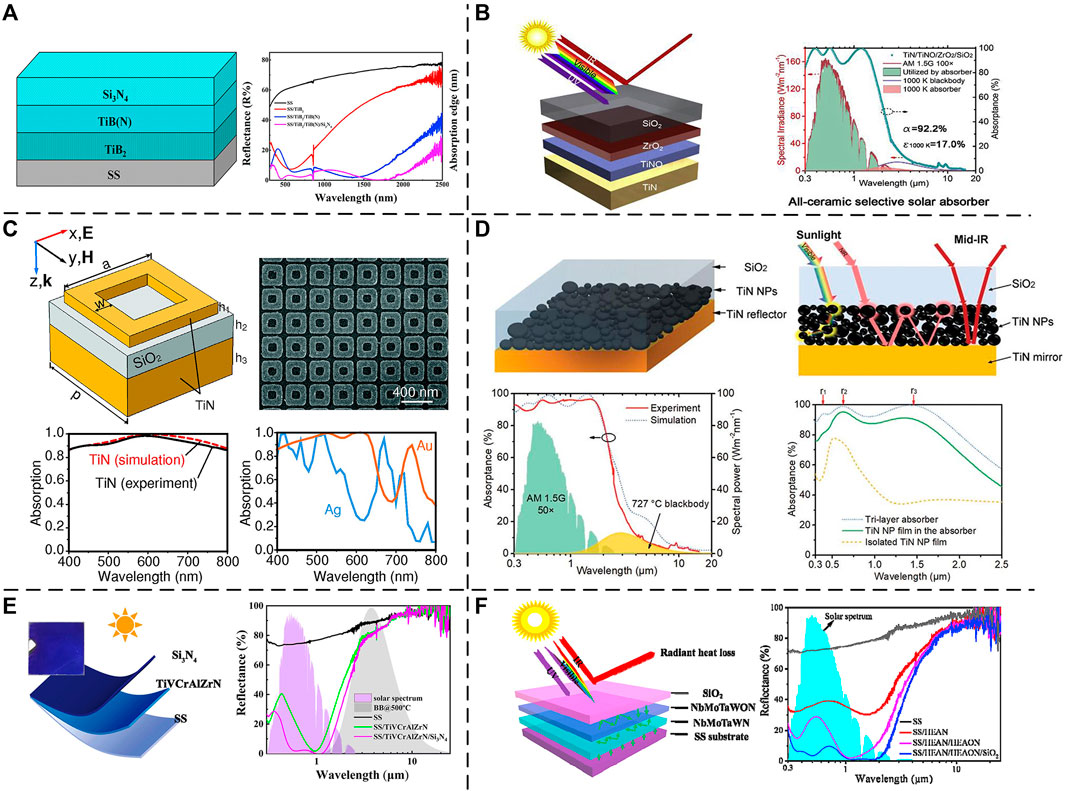
FIGURE 2. Structure design and spectral selective performance of all-ceramic SSACs. (A) Gradient refractive index-designed TiB2/TiB(N)/Si3N4 structure (Adapted from Dan et al., 2018). (B) Multilayer TiN/TiNO/ZrO2/SiO2 structure (adapted from Li et al., 2019). (C) Multilayer TiN-based plasmonic metamaterial structure (adapted from Li et al., 2014). (D) Multilayer TiN/TiN NP/SiO2 absorber (adapted from Li et al., 2021). (E) High-entropy ceramic-based TiVCrAlZrN/Si3N4 absorber (adapted from He et al., 2021a). (F) Multilayer NbMoTaWN/NbMoTaWON/SiO2 structure (adapted from Yu et al., 2021).
To prevent the metal atoms from diffusion, oxidation, and detachment at high temperature, SSACs consisting of all ceramic materials are further developed. Recently, Li et al. carried out some work on all-ceramic SSACs based on transition metal nitride/oxide multilayer coatings (Li et al., 2019). An all-ceramic TiN/TiNO/ZrO2/SiO2 absorber with TiN as reflector, TiNO as absorptive layer, ZrO2 and SiO2 as antireflection layer was prepared by magnetron sputtering and DC sputtering, as shown in Figure 2B. High solar absorptance of 92.2% and ultralow thermal emittance of 17.0% at 1,000 K were obtained by this structure, showing a conversion efficiency of 82.6% under the irradiation of 100 suns, which surpasses the Au-based, WTi-based and Ta-based coatings. What is more, annealing tests demonstrated that the spectral selectivity of TiN/TiNO/ZrO2/SiO2 degrade only 0.5% structure after 50 h annealing at 1,000 K in argon atmosphere. The all-ceramic structure boosted the operating temperature of conventional multilayer absorbers by at least 227 K. The excellent performance was attributed to the rational design of the nanofilm structure.
All-Ceramic Plasmonic Metamaterial Solar Selective Absorbing Coatings
Micro- and nanopatterning of surfaces are usually used to manipulate the broadband response, polarization independence, and angular independence via the use of resonances (Wang and WANG, 2013; Khodasevych et al., 2015). Plasmonic metamaterial absorbers (PMA), consisting of ceramic intermediate layer and ceramic metamaterials, usually exhibit an acceptable conversion efficiency in high solar concentration. For example, TiN/SiO2/TiN, prepared via DC magnetron sputtering and electron beam lithography was suggested by Li et al. (2014) as shown in Figure 2C. TiN with a melting point of 2,930°C was used as metamaterial on TiN/SiO2 substrate. As a result, TiN showed an efficient plasmonic resonance in the VIS-NIR range and improved thermal stability at the illumination of 15.5 W/cm2. The TiN/SiO2/TiN sample retains its shape and optical performance at 800°C in vacuum for 8 h. Near-unity absorption is achieved at around 650 nm, and the average absorption is approximately 95% in this spectral range. The results demonstrate that TiN/SiO2/TiN multiple structure was a promising candidate for high-temperature optical applications.
Mandal et al. prepared Cu nanoparticles/Zn PMAs via a simple “dip and-dry” technique (Mandal et al., 2017). However, due to the nonceramic intermediate and lack of antireflection coatings, the coating exhibited a solar absorptance of 0.94, but reduced to 0.91 at 200°C in argon for 96 h due to oxidation. The operating temperature of the Cu/Zn PMAs may be limited to ∼200°C. In contrast, PMAs comprising all-ceramic material usually exhibited promising thermal stability at high temperatures. Li et al. prepared all-ceramic PMAs via assembling an ultrathin TiN nanoparticle film on a TiN mirror with a dense SiO2 layer as antireflection coating (Li et al., 2021) as shown in Figure 2D. The PMAs showed a high absorptance of 0.95 and a low emittance of 0.03 over the full sunlight spectrum. With the help of an SiO2 antireflection layer, the absorber demonstrated a high thermal stability (efficiency drop <5%) up to 727°C for 150 h in vacuum. It is worth noting that the TiN nanoparticle layer was prepared by spin coating from colloidal solution, and the SiO2 layer was also spin coated from the perhydropolysilazane (PHPS) solutions. The total procedure only includes spin coating and follow-up baking, which seems to be a cost-effective way to prepare the all-ceramic SSACs. Besides, the facile solution-based processes promoted the versatility and scalability of the fabrication of the PMAs.
High-Entropy Ceramic-Based Solar Selective Absorbing Coatings
High-entropy alloys (HEA) consisting of more than five equimolar or near-equimolar alloying elements possess high-phase stability and great mechanical strength. Strong interests have been inspired to develop a new class of selective absorbers/emitters based on high-entropy ceramic for high-temperature applications.
Zhao et al. fabricated a nanometer-thick high-entropy alloy nitride Al0.4Hf0.6NbTaTiZrN-based SSAC onto 306 SS via reactive RF magnetron sputtering (Zhao et al., 2021). The as-deposited coating exhibits a high absorptance of 0.931 and a low emittance of 0.064. There was a slight change in the absorptance and emittance after annealing at 873 K for 2 h in a low-vacuum atmosphere, which resulted in a drop in the solar absorptance to 0.917 and an increase in the thermal emittance to 0.065. The slight degradation was attributed to the accumulation of oxidation production, such as ZrO2 and TaON. The results demonstrated that the inward diffusion of O atoms and the random disorder of atoms can suppress the oxidation of the SSACs.
Much work associated with high-entropy ceramic-based spectrally selective coatings has been done. He et al. prepared novel TiVCrAlZrN-based SSACs via magnetron sputtering method (He et al., 2021a) as shown in Figure 2E. The refractive index and extinction coefficient of the TiVCrAlZrN are optimized by the coating design (CODE) software to fit the reflectance and transmittance spectra. The TiVCrAlZrN-based SSACs showed solar absorptance of 92.4% and low thermal emittance of 5.3%. Cr2O3 and Fe3O4 appeared when the annealing temperature increased to 900°C due to the oxidation and diffusion of the SS substrate. The stability of coatings upon annealing at 800°C for 2 h in vacuum indicated that it was suitable for solar hot temperature applications.
Further optimization of the high-entropy ceramic-based SSACs was carried out by Yu et al. (2021) as shown in Figure 2F. A double-layer coating, consisting of NbMoTaWN and NbMoTaWON films, was prepared by radiofrequency (RF) magnetron sputtering. The addition of the NbMoTaWON layer increased the solar absorptance from 0.625 to 0.834. The further addition of the SiO2 layer as an antireflection layer increased the solar absorptance to 0.944. In addition, the coating has good optical properties after being soaked in a 3.5-wt% NaCl solution for 30 days. The optical properties and the stability performance of the multiple SS/NbMoTaWN/NbMoTaWON/SiO2 indicated its potential for high-temperature applications.
Despite the fact that high-entropy ceramic (HEC)-based SSACs possess high thermal durability due to the entropy-driven structural stabilization, metal-based infrared reflector should be removed to avoid oxidation or diffusion at high temperature (He et al., 2020; He et al., 2021b; He et al., 2021c). Song et al. prepared (NiCuCrFeSi)N-based high-entropy ceramic SSACs by magnetron sputtering on an Si substrate (Song et al., 2020). The structure exhibited a high solar absorptance of ∼0.99 and low thermal emittance of 0.06 due to a combined effect of intrinsic redshift and pyramid structure metasurface. It can be seen that the performance of high-entropy ceramic SSACs without metal substrate is acceptable. Thus, it is recommended that the high-entropy ceramic SSACs should be deposited on a nonmetal substrate to ensure thermal stability at high temperature.
It is worth noting that HEC-based SSACs could also be prepared by simple pulsed laser irradiation scanning on salt solutions (PLMS method) (Yang et al., 2021). These methods are cost effective and are also capable of fabricating both flat or complicated 3D SSACs.
Conclusions and Outlook
In this paper, we reviewed the structure designs of all-ceramic SSACs in the latest literature. The optical properties and thermal stability of the all-ceramic SSACs are also compared. To the goal of high conversion efficiency and thermal stability at high temperature over 400°C, currently some novel all-ceramic SSACs can be used, such as the transition metal boride, carbide, oxide, nitride, oxynitride-based materials (TM-B/C/O/N/ON, TM = Ti, Hf, Zr, Cr, etc.), the all-ceramic plasmonic metamaterials, and the high-entropy ceramic-based materials.
We also proposed some suggestions to further improve the solar thermal conversion efficiency and the long-term thermal stability as follows.
1) Transition metals, such as Ti, Hf, Zr, Cr, etc., have ideal forbidden bandwidth, and after combining with oxygen, nitrogen, oxynitrides, etc., they can be promising absorbing materials for the all-ceramic SSACs. However, a multiple-layer structure design is essential to utilize the optical trap and the interference effect since all-ceramic SSACs with single layer are less effective in “spectral selectivity.” Furthermore, TiN and refractory metal boride or nitride, such as TiB2, ZrN, and ZrB2, can be used as the reflective base layer to reduce thermal emittance. The thickness of the base layer should be >100 nm to reflect all the IR light.
2) Like ordinary SSACs, for all-ceramic SSACs, the antireflection layer is essential to improve the spectral capacity factor, as well as to protect the intermediate layers from oxidation and abrasion. Similarly, multiple layers with gradient in refractive index and extinction coefficient should be optimized to further decrease the spectrum reflectance. It is worth noting that the coefficient of thermal expansion of the multiple layers should be matched to reduce the interfacial stresses at high temperature.
3) Special structure designs are encouraged, such as out-of-plane nanostructures or nanoparticles, which are in favor of in-plane plasmon resonance and out-of-plane Fabry–Pérot resonance. Electromagnetic coupling could occur inside the micro/nanostructures to improve the spectrum absorption. Ultra-broadband light absorption can be achieved by combining the multisize and multilayer effect. The latest literature also verified that through reasonable structure design, the resonance of plasmonic metamaterial-based SSACs only depends on size, shape, and materials of meta-atoms instead of the in-plane periodicity, which is beneficial for the mass production of the plasmonic metamaterial SSACs.
4) Constituent of intermediate layers, such as TiNxOy, TiAlN, ZrNxOy, etc. should be optimized to tune the absorption bands for a better absorptance. As for high-entropy ceramic-based materials, distorted lattices and appropriate amorphous structures could be introduced to improve the optical properties and thermal robustness of the SSACs.
5) Though sputtering is widely used in preparing the SSACs, the relatively expensive cost and the restriction on the shape of the coatings will limit its application in commercial production. In the above cases, we find that both plasmonic metamaterial all-ceramic SSACs and HEC-based SSACs could be prepared in a cost-effective way. Except for the cost-effective trait, the solution-based methods also enable the large-scale fabrication of all-ceramic SSACs, and the PLMS method enables both flat or complicated 3D SSACs.
Author Contributions
HW investigated the literature and wrote the manuscript.
Funding
This work was supported by the Natural Science Foundation of Fujian Province (Grant No. 2021J05214), Fujian Innovation Center of Additive Manufacturing (Grant No. ZCZZ202-05), and Education Scientific Research Project of Young Teachers in Fujian Province (No. JAT190409).
Conflict of Interest
The authors declare that the research was conducted in the absence of any commercial or financial relationships that could be construed as a potential conflict of interest.
Publisher’s Note
All claims expressed in this article are solely those of the authors and do not necessarily represent those of their affiliated organizations, or those of the publisher, the editors and the reviewers. Any product that may be evaluated in this article, or claim that may be made by its manufacturer, is not guaranteed or endorsed by the publisher.
References
Bhatt, R., and Gupta, M. (2020). Design and Validation of a High-Efficiency Planar Solar Thermophotovoltaic System Using a Spectrally Selective Emitter. Opt. Express 28 (15), 21869–21890. doi:10.1364/oe.394321
Bilokur, M., Gentle, A., Arnold, M. D., Cortie, M. B., and Smith, G. B. (2020). Spectrally Selective Solar Absorbers Based on Ta:SiO 2 Cermets for Next‐Generation Concentrated Solar-Thermal Applications. Energy Technol. 8 (7), 2000125. doi:10.1002/ente.202000125
Cao, F., Kraemer, D., Sun, T., Lan, Y., Chen, G., and Ren, Z. (2015). Enhanced Thermal Stability of W-Ni-Al2O3Cermet-Based Spectrally Selective Solar Absorbers with Tungsten Infrared Reflectors. Adv. Energ. Mater. 5 (2), 1401042. doi:10.1002/aenm.201401042
Cao, F., Mcenaney, K., Chen, G., and Ren, Z. (2014). A Review of Cermet-Based Spectrally Selective Solar Absorbers. Energy Environ. Sci. 7 (5), 1615–1627. doi:10.1039/c3ee43825b
Chou, J. B., Yeng, Y. X., Lee, Y. E., Lenert, A., Rinnerbauer, V., Celanovic, I., et al. (2014). Enabling Ideal Selective Solar Absorption with 2D Metallic Dielectric Photonic Crystals. Adv. Mater. 26 (47), 8041–8045. doi:10.1002/adma.201403302
Dan, A., Chattopadhyay, K., Barshilia, H. C., and Basu, B. (2018). Shifting of the Absorption Edge in TiB2/TiB (N)/Si3N4 Solar Selective Coating for Enhanced Photothermal Conversion [J]. Langford Lane, Kidlington, Oxford, England: Pergamon-Elsevier Science Ltd , The Boulevard, 173 192–200. doi:10.1016/j.solener.2018.07.002
El-Mahallawy, N., Atia, M. R. A., Khaled, A., and Shoeib, M. (2018). Design and Simulation of Different Multilayer Solar Selective Coatings for Solar thermal Applications. Mater. Res. Express 5 (4), 046402. doi:10.1088/2053-1591/aab871
Gao, X.-H., Guo, Z.-M., Geng, Q.-F., Ma, P.-J., and Liu, G. (2016). Structure, Optical Properties and thermal Stability of TiC-Based Tandem Spectrally Selective Solar Absorber Coating. Solar Energ. Mater. Solar Cell 157, 543–549. doi:10.1016/j.solmat.2016.06.050
Gao, X.-H., Guo, Z.-M., Geng, Q.-F., Ma, P.-J., Wang, A.-Q., and Liu, G. (2017). Enhanced Optical Properties of TiN-Based Spectrally Selective Solar Absorbers Deposited at a High Substrate Temperature. Solar Energ. Mater. Solar Cell 163, 91–97. doi:10.1016/j.solmat.2017.01.023
Gao, X.-H., Guo, Z.-M., Geng, Q.-F., Ma, P.-J., Wang, A.-Q., and Liu, G. (2017). Microstructure, Chromaticity and thermal Stability of SS/TiC-WC/Al2O3 Spectrally Selective Solar Absorbers. Solar Energ. Mater. Solar Cell 164, 63–69. doi:10.1016/j.solmat.2017.02.009
He, C-Y., Gao, X-H., and Dong, M. (2020). Further Investigation of a Novel High Entropy alloy MoNbHfZrTi Based Solar Absorber Coating with Double Antireflective Layers [J]. Solar Energ. Mater. Solar Cell, 217 110709.
He, C-Y., Zhang, X., and Yu, D-M. (2021). Toward a Scalable and Cost-Conscious Structure in Spectrally Selective Absorbers: Using High-Entropy Nitride TiVCrAlZrN [J]. ACS Appl. Energ. Mater.
He, C.-Y., Gao, X.-H., Qiu, X.-L., Yu, D.-M., Guo, H.-X., and Liu, G. (2021). Scalable and Ultrathin High‐Temperature Solar Selective Absorbing Coatings Based on the High‐Entropy Nanoceramic AlCrWTaNbTiN with High Photothermal Conversion Efficiency. Sol. RRL 5 (4), 2000790. doi:10.1002/solr.202000790
He, C.-Y., Gao, X.-H., Yu, D.-M., Zhao, S.-S., Guo, H.-X., and Liu, G. (2021). Toward High-Temperature thermal Tolerance in Solar Selective Absorber Coatings: Choosing High Entropy Ceramic HfNbTaTiZrN. J. Mater. Chem. A. 9 (37), 21270–21280. doi:10.1039/d1ta06682j
Hijazi, H., Mokhiamar, O., and Elsamni, O. (2016). Mechanical Design of a Low Cost Parabolic Solar Dish Concentrator. Alexandria Eng. J. 55 (1), 1–11. doi:10.1016/j.aej.2016.01.028
Hu, E.-T., Liu, X.-X., Yao, Y., Zang, K.-Y., Tu, Z.-J., Jiang, A.-Q., et al. (2018). Multilayered Metal-Dielectric Film Structure for Highly Efficient Solar Selective Absorption. Mater. Res. Express 5 (6), 066428. doi:10.1088/2053-1591/aacdb3
Jiang, D., and Yang, W. (2017). Refractory Material Based Frequency Selective Emitters/absorbers for High Efficiency and thermal Stable Thermophotovoltaics. Solar Energ. Mater. Solar Cell 163, 98–104. doi:10.1016/j.solmat.2017.01.022
Khamlich, S., Ismail, F., and Schouw, M. Photo-Thermal Conversion Efficiency of Spectrally Selective Cr 2 O 3/Cr/Cr 2 O 3 Multilayered Solar Absorber. In proceedings of the AIUE Proceedings of the 18th Industrial and Commercial Use of Energy Conference, F, (2020).
Khamlich, S., Mccrindle, R., Nuru, Z. Y., Cingo, N., and Maaza, M. (2013). Annealing Effect on the Structural and Optical Properties of Cr/α-Cr2O3 Monodispersed Particles Based Solar Absorbers. Appl. Surf. Sci. 265, 745–749. doi:10.1016/j.apsusc.2012.11.099
Khodasevych, I. E., Wang, L., Mitchell, A., and Rosengarten, G. (2015). Micro- and Nanostructured Surfaces for Selective Solar Absorption. Adv. Opt. Mater. 3 (7), 852–881. doi:10.1002/adom.201500063
Kondaiah, P., Niranjan, K., and John, S. (2019). Tantalum Carbide Based Spectrally Selective Coatings for Solar thermal Absorber Applications [J]. Solar Energ. Mater. Solar Cell, 198 26–34.
Kraemer, D., Jie, Q., and Mcenaney, K. (2016). Concentrating Solar Thermoelectric Generators with a Peak Efficiency of 7.4% [J]. Nat. Energ. 1 (11), 1–8.
Kumar, R., and Dixit, A. (2020). Issue and Challenges with High-Temperature Solar Selective Material for Solar Thermal Application. In Renewable Energy and Climate Change. Springer, 99–108. doi:10.1007/978-981-32-9578-0_9
Li, P., Liu, B., Ni, Y., Liew, K. K., Sze, J., Chen, S., et al. (2015). Large-Scale Nanophotonic Solar Selective Absorbers for High-Efficiency Solar Thermal Energy Conversion. Adv. Mater. 27 (31), 4585–4591. doi:10.1002/adma.201501686
Li, W., Guler, U., Kinsey, N., Naik, G. V., Boltasseva, A., Guan, J., et al. (2014). Refractory Plasmonics with Titanium Nitride: Broadband Metamaterial Absorber. Adv. Mater. 26 (47), 7959–7965. doi:10.1002/adma.201401874
Li, Y., Lin, C., and Zhou, D. (2019). Scalable All-Ceramic Nanofilms as Highly Efficient and Thermally Stable Selective Solar Absorbers [J]. Nano Energy, 64 103947.
Li, Y., Li, D., Zhou, D., Chi, C., Yang, S., and Huang, B. (2018). Efficient, Scalable, and High-Temperature Selective Solar Absorbers Based on Hybrid-Strategy Plasmonic Metamaterials. Sol. RRL 2 (8), 1800057. doi:10.1002/solr.201800057
Li, Y., Lin, C., Wu, Z., Chen, Z., Chi, C., Cao, F., et al. (2021). Solution‐Processed All‐Ceramic Plasmonic Metamaterials for Efficient Solar-Thermal Conversion over 100-727 ° C. Adv. Mater. 33 (1), 2005074. doi:10.1002/adma.202005074
Loni, R., Asli-Ardeh, E. A., and Ghobadian, B. (2018). Numerical Comparison of a Solar Dish Concentrator with Different Cavity Receivers and Working Fluids [J]. J. Clean. Prod., 198 1013–1030.
Mandal, J., Wang, D., Overvig, A. C., Shi, N. N., Paley, D., Zangiabadi, A., et al. (2017). Scalable, "Dip‐and‐Dry" Fabrication of a Wide‐Angle Plasmonic Selective Absorber for High‐Efficiency Solar-Thermal Energy Conversion. Adv. Mater. 29 (41), 1702156. doi:10.1002/adma.201702156
Mao, Q., Shuai, Y., and Yuan, Y. (2014). Study on Radiation Flux of the Receiver with a Parabolic Solar Concentrator System. Energ. Convers. Manag. 84, 1–6. doi:10.1016/j.enconman.2014.03.083
Qiu, X-L., Gao, X-H., and He, C-Y. (2020). Optical Design, thermal Shock Resistance and Failure Mechanism of a Novel Multilayer Spectrally Selective Absorber Coating Based on HfB2 and ZrB2 [J]. Solar Energ. Mater. Solar Cell, 211 110533.
Qiu, X.-L., Gao, X.-H., He, C.-Y., Chen, B.-H., and Liu, G. (2019). Structure, Optical Simulation and thermal Stability of the HfB2-Based High-Temperature Solar Selective Absorbing Coatings. RSC Adv. 9 (51), 29726–29733. doi:10.1039/c9ra05014k
Rinnerbauer, V., Lausecker, E., Schäffler, F., Reininger, P., Strasser, G., Geil, R. D., et al. (2015). Nanoimprinted Superlattice Metallic Photonic crystal as Ultraselective Solar Absorber. Optica 2 (8), 743–746. doi:10.1364/optica.2.000743
Rinnerbauer, V., Lenert, A., Bierman, D. M., Yeng, Y. X., Chan, W. R., Geil, R. D., et al. (2014). Metallic Photonic Crystal Absorber-Emitter for Efficient Spectral Control in High-Temperature Solar Thermophotovoltaics. Adv. Energ. Mater. 4 (12), 1400334. doi:10.1002/aenm.201400334
Sharma, A. K., Sharma, C., Mullick, S. C., and Kandpal, T. C. (2017). Solar Industrial Process Heating: A Review. Renew. Sust. Energ. Rev. 78, 124–137. doi:10.1016/j.rser.2017.04.079
Shimizu, M., Kohiyama, A., and Yugami, H. (2018). Evaluation of thermal Stability in Spectrally Selective Few-Layer Metallo-Dielectric Structures for Solar Thermophotovoltaics. J. Quantitative Spectrosc. Radiative Transfer 212, 45–49. doi:10.1016/j.jqsrt.2018.02.037
Song, P., Wang, C., Ren, J., Sun, Y., Zhang, Y., Bousquet, A., et al. (2020). Modulation of the Cutoff Wavelength in the Spectra for Solar Selective Absorbing Coating Based on High-Entropy Films. Int. J. Miner Metall. Mater. 27 (10), 1371–1378. doi:10.1007/s12613-020-1982-7
Tian, Y., Liu, X., Ghanekar, A., Chen, F., Caratenuto, A., and Zheng, Y. (2020). Blackbody-cavity Ideal Absorbers for Solar Energy Harvesting. Sci. Rep. 10 (1), 20304–20307. doi:10.1038/s41598-020-77372-9
Wang, H., and Wang, L. (2013). Perfect Selective Metamaterial Solar Absorbers. Opt. Express 21 Suppl 6 (106), A1078–A1093. doi:10.1364/OE.21.0A1078
Wang, X., Hsieh, M-L., and Bur, J. (2020). Capillary-driven Solar-thermal Water Desalination Using a Porous Selective Absorber [J]. Mater. Today Energ., 17 100453.
Wang, X., Gao, J., Hu, H., Zhang, H., Liang, L., Javaid, K., et al. (2017). High-temperature Tolerance in WTi-Al 2 O 3 Cermet-Based Solar Selective Absorbing Coatings with Low thermal Emissivity. Nano Energy 37, 232–241. doi:10.1016/j.nanoen.2017.05.036
Wang, X., Hsieh, M.-L., Bur, J. A., Lin, S.-Y., and Narayanan, S. (2020). The Role of Nanostructure Morphology of Nickel-Infused Alumina on Solar-thermal Energy Conversion. J. Opt. 23 (1), 015101. doi:10.1088/2040-8986/abcc53
Wang, X., Yang, H., Hsieh, M.-L., Bur, J. A., Lin, S.-Y., and Narayanan, S. (2020). Nickel-Infused Nanoporous Alumina as Tunable Solar Absorber. MRS Adv. 5 (50), 2575–2583. doi:10.1557/adv.2020.300
Wang, Y., Liu, H., and Zhu, J. (2019). Solar Thermophotovoltaics: Progress, Challenges, and Opportunities. APL Mater. 7 (8), 080906. doi:10.1063/1.5114829
Weinstein, L. A., Loomis, J., Bhatia, B., Bierman, D. M., Wang, E. N., and Chen, G. (2015). Concentrating Solar Power. Chem. Rev. 115 (23), 12797–12838. doi:10.1021/acs.chemrev.5b00397
Xiang, H., Dai, F., and Zhou, Y. (2021). Theoretical Insight into the Solar thermal Absorption Property of Ultra-high Temperature Ceramics TMB2 (TM= Ti, Zr, and Hf) [J]. Solar Energ. Mater. Solar Cell, 225 111032.
Xu, K., Du, M., Hao, L., Mi, J., Yu, Q., and Li, S. (2020). A Review of High-Temperature Selective Absorbing Coatings for Solar thermal Applications. J. Materiomics 6 (1), 167–182. doi:10.1016/j.jmat.2019.12.012
Yang, J. X., Dai, B.-H., Chiang, C.-Y., Chiu, I.-C., Pao, C.-W., Lu, S.-Y., et al. (2021). Rapid Fabrication of High-Entropy Ceramic Nanomaterials for Catalytic Reactions. ACS nano 15 (7), 12324–12333. doi:10.1021/acsnano.1c04259
Yu, D-M., He, C-Y., and Zhao, S-S. (2021). A Novel Multilayer High Temperature Solar Absorber Coating Based on High-Entropy alloy NbMoTaW: Optical Properties, thermal Stability and Corrosion Properties [J]. J. Materiomics.
Keywords: solar selective absorbing coatings, all ceramic, structure design, thermal stability, optical properties
Citation: Wang H (2021) Structure, Optical Properties and Thermal Stability of All-Ceramic Solar Selective Absorbing Coatings: A Mini Review. Front. Energy Res. 9:789917. doi: 10.3389/fenrg.2021.789917
Received: 05 October 2021; Accepted: 01 November 2021;
Published: 26 November 2021.
Edited by:
Yang Li, Hong Kong University of Science and Technology, Hong Kong SAR, ChinaReviewed by:
Xuanjie Wang, Rensselaer Polytechnic Institute, United StatesXiang-Hu Gao, Lanzhou Institute of Chemical Physics (CAS), China
Copyright © 2021 Wang. This is an open-access article distributed under the terms of the Creative Commons Attribution License (CC BY). The use, distribution or reproduction in other forums is permitted, provided the original author(s) and the copyright owner(s) are credited and that the original publication in this journal is cited, in accordance with accepted academic practice. No use, distribution or reproduction is permitted which does not comply with these terms.
*Correspondence: Hui Wang, MTk4OTIwOTdAZmp1dC5lZHUuY24=