- 1Pratt & Whitney, West Hartford, CT, United States
- 2GE Aviation, Evendale, OH, United States
- 3Department of Mechanical and Aerospace Engineering, University of Dayton, Dayton, OH, United States
- 4Boeing, Chicago, IL, United States
- 5Volpe National Transportation Systems Center, Cambridge, MA, United States
The aviation sector seeks to reduce greenhouse gas (GHG) emissions, with manufacturers and airlines announcing “zero-emission” goals and plans. Reduced carbon aviation fuels are central to meeting these goals. However, current and near-term aircraft, which will remain flying for decades, are designed around the combustion of petroleum-based aviation kerosene (e.g., Jet A/A-1). Therefore, the industry has focused on the qualification and approval of synthesized (e.g., non-petroleum-based) aviation fuel components with maximum blend limit percentages to avoid the blended fuel having properties outside the accepted ranges for Jet A/A-1. The synthesized components approved for blending are not necessarily interchangeable with Jet A/A-1. They may lack certain required chemical components, such as aromatics, or may have other characteristics outside the allowable ranges. To ensure safety, these synthesized aviation fuel components are only qualified to be used in commercial aviation when blended up to approved limits. The sector seeks to move toward the capability of using 100% synthesized aviation fuels that also meet sustainability criteria, known as sustainable aviation fuels, or SAF. However, these fuels must be developed, assessed, and deployed appropriately. This paper explores key questions relating to the introduction of 100% SAF, concluding that:
• Near-term unblended synthesized aviation fuels must be “drop-in,” meaning they are compatible with existing aircraft and infrastructure.
• Stand-alone complete fuels could be qualified within 1–2 years, with blends of blending components to reach 100% synthesized fuels to follow.
• Sustainability criteria, while critical to sector acceptance, will continue to be assessed separately from technical performance.
Introduction
The aviation industry seeks cost-competitive synthesized aviation fuels with a carbon benefit and sustainability performance to counter the effects of price spikes, competition for finite oil supplies, and aviation’s high profile as a greenhouse gas (GHG) and particulate emitter. This decarbonization is additionally needed to meet long-term net-zero emissions goals (ATAG, 2021). Current and near-term (10–20 years) aircraft will remain in operation for decades and are designed around aviation kerosene (e.g., Jet A/A-1). Technologies to increase the efficiency of new aircraft by a fleet average of 1–2% each year are offset by a 4–5% compound average annual travel growth rate (Fleming and de Lepinay, 2019) leading to projected emissions increases (IATA, 2019). Proposed “zero emissions” options, such as batteries (Hepperle, 2012; Schäfer, et al., 2019) or cryogenic fuels, are of low technology readiness, have restricted range, and require new energy supply networks (McKinsey and Co., 2020). However, reducing the carbon footprint of jet fuel reduces aviation’s impact on the environment now and in the long-term.
Since 2006, the Commercial Aviation Alternative Fuels Initiative (CAAFI®), a public-private partnership including United States government, aviation sector stakeholders, and aviation fuel supply chain participants, has worked to enhance energy security and environmental sustainability for aviation with alternative jet fuels by facilitating their deployment in the marketplace (CAAFI, 2021). Initially, due to safety and compatibility concerns, CAAFI and the industry focused on qualification of synthesized fuel blending components that come from sources other than petroleum to be added to conventional aviation fuel sourced from petroleum, which are qualified by ASTM D4054 for use in ASTM D7566 (ASTM International, 2021) (see Figure 1). These blending components are limited to a maximum blend percentage to ensure that all blended fuels properties are within accepted ranges for Jet A/A-1 (particularly aromatic content) (Zschocke et al., 2012). Thus, the resultant blended fuels are interchangeable with unblended Jet A/A-1 regarding handling, operability, and safety and referred to as “drop-in” aviation fuels (Colket., et al., 2016). The blended fuel is re-identified as Jet A/A-1 for transport, storage, purchase, and use under the ASTM D1655 petroleum-based jet fuel specification (ASTM International, 2020).
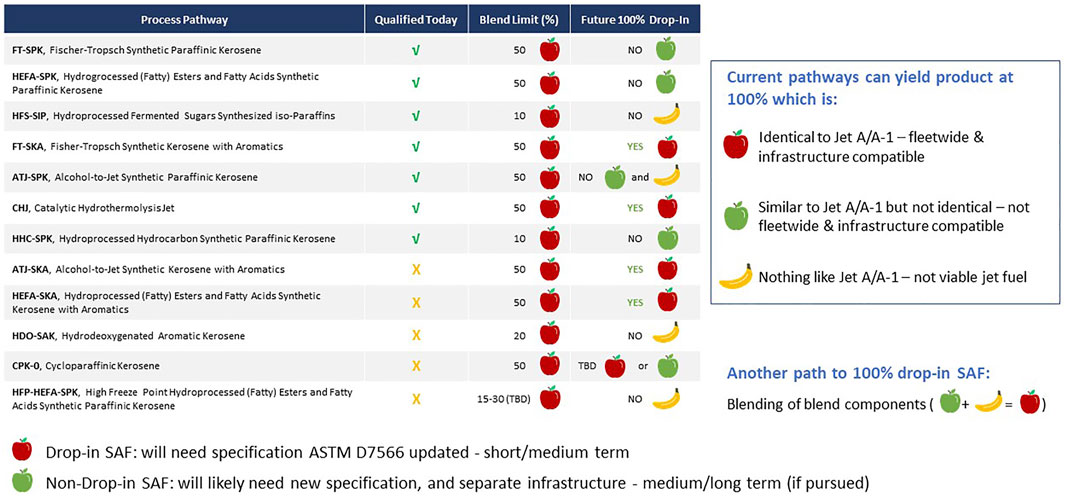
FIGURE 1. Types of synthesized fuel blendstocks and their ability to be used as drop-in fuels as blendstocks or as stand-alone fuel. The red apples indicate fuels that can be drop-in, green apples are similar to drop-ins and may be usable in some existing aircraft but would require modification of the existing specifications and possibly infrastructure, and bananas indicate fuels that are completely different from petroleum-based kerosene and could not be used as stand-alone jet fuel in current infrastructure or equipment.
The existing synthesized blending components are not necessarily sustainable, as environmental, social, and economic performance requirements are not part of ASTM qualification. To address sustainability goals, aviation stakeholders and International Civil Aviation Organization (ICAO) member States put a process in place to evaluate production, feedstock, land-use, social impact, and life-cycle carbon footprint of various possible paths, and consider relevant environmental, social, and economic risks, formalized via the ICAO Carbon Off-setting and Reduction Scheme for International Aviation (CORSIA) (ICAO, 2021a). Fuels certified as sustainable under this, and similar approaches, are called sustainable aviation fuels (SAF). The synthesized blending components complying with ASTM D7566 can be made according to sustainability criteria to become SAF.
The current production of SAF globally is much less than 1% (Csonka, 2020). However, the United States has set a target of producing 3 billion gallons of SAF per year by 2030, totaling about 10% of anticipated annual jet fuel consumption, and targets complete replacement of petroleum-based jet fuel by 2050 (U.S. White House, 2021), and SAF mandates are in place or are under consideration globally (Malicier, 2021). While overall SAF availability is currently low, 100% SAF may be available at particular locations very soon. Airfields could provide limited amounts of 100% SAF to those willing to pay for that distinction, such as private jet owners. Or a particular airport or nation could set goals to fuel a certain number of flights with 100% SAF, possibly within the decade. Aircraft manufacturers have made commitments to compatibility with 100% SAF by 2030 even in the absence of an agreed upon definition for 100% SAF (Boeing, 2021). Furthermore, the qualification of 100% SAF would eliminate the need for controlled blending of that SAF into the fuel pool, reducing supply chain complexity. Thus, the 100% synthesized fuel definition and qualification process should happen now to prepare for these future needs.
The ability to use neat SAF (without blending with conventional fuel), or “100% SAF” could further reduce aviation’s global GHG generation and human health impacts. Additionally, 100% SAFs containing reduced or no aromatics reduce non-volatile particulate matter (nvPM) emissions, which are linked to contrail formation (Voigt, et al., 2021), and contrails are suggested to contribute more to aviation radiative forcing than CO2 emissions (Lee, et al., 2021). Finally, 100% SAF has very low levels of sulfur, which leads to low levels of sulfur oxide (SOx) emissions (Moore, et al., 2015). Thus 100% SAF would reduce aviation’s GHG production, contrails, and SOx emissions, all significant environmental benefits.
However, the synthesized blending components approved to date are not by themselves necessarily drop-in or interchangeable with Jet A/A-1, and so cannot be used as 100% synthesized fuels alone (Figure 1). They may lack aromatics required in legacy aircraft and aircraft engines for seal compatibility (Anuar et al., 2021). Properties such as freeze point or mass density may be near the limits for the accepted Jet A/A-1 range (Edwards, 2017; Colket and Heyne, 2021). They may contain a restricted number of chemical species or have limited carbon number range and not meet the Jet A/A-1 distillation curve requirements (Bell et al., 2018; Won et al., 2019). These differences may impact the performance, operability, and/or safety of some aircraft models and engines currently flying (Bell et al., 2018; Won et al., 2019).
The challenge is to achieve 100% SAF that meets all the safety and operability requirements of the ASTM qualification process, as well as the affordability and sustainability goals of the industry. In this paper, we explain the importance of the “drop-in” requirement, highlight potential approaches to achieve fully synthesized aviation fuels and provide perspectives on the viability of those approaches, and discuss how sustainability criteria can be layered onto fully synthesized jet fuels to create complete sustainable aviation fuels.
Importance of “Drop-In” as a Requirement
Jet A/A-1s are unique mixtures of hydrocarbons that cannot be simply defined by a certain chemical composition. The characteristics of Jet A/A-1 are derived from petroleum going through modern refinery processes, e.g., distillation, hydrotreatment, catalytic reforming, etc. Specifications and tests have been developed to measure performance properties such as net heat of combustion, thermal stability, viscosity, distillation curve, freezing point, flash point, smoke point, density, lubricity, aromatic content, sulfur content, etc. (Hemighaus, et al., 2007). Over time, the property specifications have been reviewed and updated, testing improved, and new specifications added. The intent has been to make Jet A/A-1 the safest and most suitable possible fuel for aviation.
Aviation gas turbines are designed to operate on and utilize the properties of Jet A/A-1 (Heyne et al., 2021). Jet A/A-1 properties are tightly linked to the reliability and safety of aviation. For example, the flash point of Jet A/A-1 is such that a match will not ignite the fuel at room temperature (ASTM International, 2020). Yet gas turbines can ignite the fuel at conditions as cold as Fairbanks, Alaska, or as hot as Saudi Arabia, and can be re-lit in mid-flight at 30,000 feet.
The ASTM qualification process (ASTM D4054) has been adapted to enable synthesized fuel approvals (Rumizen, 2021). Thus far, all synthesized blended fuel has been required to be drop-in and meet every specification for petroleum-based Jet A/A-1 (ICAO, 2018), because it was not known what specific properties of the fuel were critical for operability and safety and which properties could be relaxed. An 8% minimum aromatics content was set to ensure compatibility for nitrile seals. Combustor performance includes factors such as cold weather ignition, altitude relight, lean blow-out characteristics, interactions with combustor acoustics and dynamics, flame stability, flame luminosity, heat release patterns, and so on. Safety and reliability in external components must consider such factors as cold fuel viscosity system performance, vapor pressure characteristics and impact on pump performance, cavitation potential, low lubricity, seal compatibility, thermal stability and tendency to varnish, icing characteristics, entrained water, biocide compatibility, flammability, and other criteria (Colket et al., 2016; Colket and Heyne, 2021).
Although jet fuel combustion has been studied for decades, unknowns remain. For example, critical factors for altitude relight are not well characterized: atomization is a complex interplay of fuel surface tension, viscosity, density, and air temperature and pressure; fuel vapor pressure, and molecular composition are also important (Peiffer et al., 2019; Boehm et al., 2021). Research, such as the National Jet Fuels Combustion Program (Colket et al., 2016; CAAFI R&D Team, 2019), has added to the understanding of the interaction of fuel chemical composition and physical properties with combustion. However, that understanding is not complete, and any uncertainty may impact safety.
Jet fuel is not only used for combustion in the aircraft. Fuel is used to exchange heat with the oil, to power fueldraulic actuators, and to lubricate (or at least not excessively wear) pumps (Heyne et al., 2021). Additionally, in legacy aircraft, the nitrile seals are sensitive to fuel composition and their performance might be impacted (Graham, et al., 2013). To be drop-in, the fuel must satisfy these functionalities as well.
The industry position is that safety for all past, present and future aircraft must be addressed in the specification of any fuel. For 100% synthesized fuels, new requirements may need to be added to the specification. For example, the NJFCP suggested several characteristics as potentially critical to the safety and operability of jet fuels, such as derived cetane number (Colket et al., 2016; Stachler et al., 2020; Boehm et al., 2021). These new requirements need to be considered, researched and verified. Similarly, to better enable synthesized fuel cost-effectiveness, it may be desired to redefine or add other specifications and properties, which would require additional research and verification to insure 100% drop-in compatibility.
Without knowing the impact of a fuel not meeting all Jet A/A-1 characteristics, a proposed non-drop-in fuel must be limited to validated applications. A non-drop-in fuel requires separate handling, storage, and logistics, and must be compatible with that separate infrastructure (e.g., fueling trucks, hydrant system, tanks, etc.). It requires safety measures to eliminate any possible mistakes in fuel identity. It may require separate fittings, separate fuel tanks, unique identification procedures, and testing similar to procedures used for gasoline and diesel fuels at gas stations, with the potential for much more severe safety consequences if mistakes are made.
Thus, 100% synthesized fuels should be drop-in for all aviation applications, at least in the short- to mid-term. However, the specifications for Jet A/A-1 may be refined and expanded as additional learning is acquired.
Approaches to Achieve 100% Synthesized Jet Fuel
Here are approaches to achieve 100% synthesized jet fuel that should be considered.
1. Replicate All Jet A/A-1 Properties in a Single Fuel. In 1999, Sasol developed Fischer-Tropsch (FT) fuels from coal, first as no more than a 50% blending component, then, with a process change to include aromatics to replicate all Jet A/A-1 properties, as a 100% fully synthesized jet fuel. Extensive testing, including long duration engine tests, ensured that all the required Jet A/A-1 performance characteristics were met. Similarly, there are current biomass-based pathways, some of which are FT processes, that replicate all Jet A/A-1 performance properties (MODUK, 2020).
2. Replicate All Jet A/A-1 Properties in a Blended Fuel. Current synthesized blending components must be combined with conventional Jet A/A-1 to meet all necessary performance properties including aromatic content. In the future, a synthesized blendstock with no aromatics could be combined with a synthesized blendstock with aromatic content to achieve a 100% synthesized that meets all required Jet A/A-1 properties. Conceivably, as many blending components as needed could be used to replicate the properties of Jet A/A-1.
3. Substitute for Aromatics or Reduce Aromatics Requirement. The requirement for aromatics is linked to the performance of nitrile seals in older engines: without the aromatics, the seals shrink and fuel leaks occur. Other molecules, such as cycloparaffins, can act like aromatics from seal performance perspective (Graham, et al., 2013). This potential is currently under evaluation. Additionally, the 8% lower limit for aromatics is known to be safe with margin (Heminghaus, et al., 2006). If 100% SAF with low or no aromatics is sought to reduce nvPM, the specification for aromatics could be reduced or removed, while retaining all other Jet A/A-1 performance properties. Research could identify substitute molecules and the true lower limit of aromatic content.
4. Remove the Requirement for Seal-Swelling Components (non-drop-in). Modern engines have replaced nitrile seals with better performing fluorocarbons and fluorosilicone seals. Engine and flight tests have been performed on “neat” SAFs (Applied Research Associates, 2016; Airbus, 2021; Palmer, 2021; Rolls-Royce, 2021). Thus, a fuel without aromatics, e.g. 100% paraffinic, that matches all other specifications for Jet A/A-1 could be considered for use in compatible aircraft. However, this fuel would not be “drop-in” for legacy aircraft and would face the reliability and safety concerns outlined previously.
5. Redefine Jet Fuel Requirements. It is possible that not all the current specifications for Jet A/A-1 are necessary for engine and aircraft performance. Changing, removing, or adding alternative requirements may make it easier to produce improved synthesized fuels from biological sources. Bacteria and yeast tend to produce very specific chemicals rather than a broad range of chemical components like petroleum-based Jet A/A-1. For example, the “Hydroprocessed Fermented Sugars to Synthetic Isoparaffins” process (ASTM D7566 A3, HFS-SIP) produces solely farnesene, a 15-carbon molecule (ASTM International, 2021), Extensive research and testing are needed to define specification modifications and assure the reliability and safety of fuels produced to the redefined specifications.
Currently, the first two options (100% synthesized fuels from a single or blended fuels) could be near term paths, while Options 3 and 5 (redefining jet fuel requirements based on further research) have future potential with sufficient research and learning. Option 4 (non-drop-in fuels) is not desirable since it would require significant, expensive changes to aircraft equipment and infrastructure.
Thus far, the ASTM D4054 specification process has been viewed from the perspective of comparison to a conventional fuel (Rumizen, 2021). A key question is whether and how this process could be changed to better enable 100% synthesized fuels. Does the ASTM specification process need to become more stringent to capture unknowns that have been ignored because they have been unknown for petroleum-based fuels? Or can it be simplified due to more physics and chemistry-based understanding? Recently an optional prescreening approach has been formalized that uses only a small quantity of fuel to perform analyses that help identify the suitability and potential gaps for a particular proposed fuel (CAAFI R&D Team, 2019). It may be possible to make other changes to make the process more effective and efficient while continuing to ensure the safety of aviation fuels.
The ASTM qualification process should be continuously reviewed and improved as additional learning with respect to 100% synthesized fuels and their properties is acquired, which in the longer term would enable modifications to jet fuel specifications needed for Options 3 and 5 above.
Sustainability of 100% Synthesized Jet Fuel
To further refine the definition of a 100% synthesized aviation fuel to 100% Sustainable Aviation Fuel, any SAF needs to be produced in a way that demonstrably meets sustainability criteria to ensure environmental, social, and economic performance. Sustainability requirements are applied to synthesized aviation fuels separately from the technical, safety, and performance characteristics that qualify a fuel to be used in aviation under the ASTM specifications; therefore, a 100% synthesized fuel is not necessarily a 100% sustainable aviation fuel, even if it comes from a renewable feedstock. Nevertheless, the sustainability performance is critical to the value proposition of these fuels and must be ensured.
There are existing approaches to evaluate the environmental performance of SAF, including regulatory scheme compliance, such as qualification for the United States Renewable Fuel Standard (US Environmental Protection Agency, 2010) or California’s Low Carbon Fuel Standard (State of California, 2020). The full sustainability (environmental, social, and economic) performance of SAF can be evaluated and assured through the use of voluntary sustainability certification schemes, such as those used for CORSIA qualification (ICAO, 2021b) or the European Union’s Renewable Energy Directive (European Union, 2021). The certification approach assures sustainable production of SAF to the extent possible.
Currently, there is no consistent definition for the use of the term SAF. The aviation sector must continue to decide how to evaluate the sustainability of SAF, which sustainability factors to address, and whether the existing approaches for regulatory compliance and voluntary certification are sufficient to qualify fuels as sustainable. At a minimum, it is reasonable to expect that the aviation sector will call fuels SAF that meet the sustainability criteria agreed upon by ICAO for CORSIA (ICAO, 2021c), as these are clearly defined and can be used to meet existing emissions obligations. Some nations and some airlines or aviation groups may commit to greater sustainability requirements or specific requirements in isolation (e.g., the United States Grand Challenge defines SAF as having a 50% reduction in carbon intensity for SAF, whereas CORSIA requires a 10% reduction). A minimum standard for labeling fuels as SAF will reduce confusion and ensure that SAF achieve the sustainability performance on which their value proposition depends.
It should be noted that sustainability certification as currently implemented does not fully address important societal choices and tradeoffs that are beyond the scope of aviation, such as interactions among economies, competition/balance with other renewable energy approaches, how wastes should be credited as feedstocks, and how these considerations should be valued both locally and internationally. Previous studies have concluded that biofuels in particular may have issues of overall scalability and environmental impact if deployed at a global level as a primary fossil energy replacement solution (de Castro et al., 2014; Gomiero, 2015). These factors are not addressed by certification at the fuel/feedstock producer level. The choice of which sectors of the economy use bio-based fuels and the scale of their use are societal decisions to which the aviation sector can contribute.
Discussion
While the ongoing ICAO Long Term Aspirational Goals (LTAG) exercise is considering technology horizons in aviation of 2050 and 2070 (ICAO, 2021d), action must be taken now, as the actions with the greatest impacts will take time to penetrate the global aviation market.
Considering the options presented herein, the definition and qualification process for Option 1—Replicate All Jet A/A-1 Properties in a Single Fuel—could be achievable within 2 years. This approach has already been pioneered by SASOL, and other fuels are following that pathway (DefStan 91-091). Option 2—Replicate All Jet A/A-1 Properties in a Blended Fuel—could follow closely, a year or two behind, since in essence, it is the pathway being followed for current blended SAFs. The ASTM Task Force AC598 (Standardization of Jet Fuel Fully Comprised of Synthesized Hydrocarbons) has begun work to consider the definition and qualification process for Options 1 and 2 (Polek, 2021); that process will need to take into account the challenges laid out in this paper.
Options 3 and 5 that would modify Jet A/A-1 properties require significant research and testing before the safety of either replacing or lowering the aromatics is assured, and any redefinition of, or addition to, current specifications or standards is made. Future research must include investigation of how fuel compositions interact with the operability, reliability and safety of aircraft and flight. Finally, since Option 4—Remove the Requirement for Seal-Swelling Components—leads to a non-drop-in fuel, it is not likely to be supported by industry.
Author Contributions
The individual authors listed here were all engaged in the drafting, revision, and referencing of this mini-review based on individual and organizational experience and knowledge.
Funding
KL and PH participation was funded by the United States Federal Aviation Administration Office of Environment and Energy through agreement number 693KA9-20-N-00013 under the supervision of Nathan Brown. JH work was funded by United States Federal Aviation Administration Office of Environment and Energy through ASCENT, the FAA Center of Excellence for Alternative Jet Fuels and the Environment, Project 34 through FAA Award Number 13-C-AJFE-UD-024 under the supervision of Anna Oldani. Any opinions, findings, conclusions or recommendations expressed in this material are those of the authors and do not necessarily reflect the views of the FAA. Open access publication fees are provided by the FAA through the Volpe National Transportation Systems Center.
Conflict of Interest
Author SK was employed by the company Pratt & Whitney. Author GA was employed by the Company General Electric. Author JE was employed by the company Boeing.
The remaining authors declare that the research was conducted in the absence of any commercial or financial relationships that could be construed as a potential conflict of interest.
Publisher’s Note
All claims expressed in this article are solely those of the authors and do not necessarily represent those of their affiliated organizations, or those of the publisher, the editors, and the reviewers. Any product that may be evaluated in this article, or claim that may be made by its manufacturer, is not guaranteed or endorsed by the publisher.
References
Airbus (2021). An A350 Fuelled by 100% SAF Just Took off. Available at: https://www.airbus.com/newsroom/stories/A350-fuelled-by-100-percent-SAF-just-took-off.html (Accessed September 9, 2021).
Anuar, A., Undavalli, V. K., Khandelwal, B., and Blakey, S. (2021). Effect of Fuels, Aromatics and Preparation Methods on Seal Swell. Aeronaut. J. 125, 1542–1565. doi:10.1017/aer.2021.25
Applied Research Associates (2016). ARA's History-Making Sustainable Aviation Fuel (SAF) Technology. Available at: https://www.ara.com/products/readijet/(Accessed September 9, 2021).
ASTM International (2020). D1655-20d: Standard Specification for Aviation Turbine Fuels. West Conshohocken, PA, USA: ASTM International.
ASTM International (2021). D7566-20c: Standard Specification for Aviation Turbine Fuel Containing Synthesized Hydrocarbons. West Conshohocken, PA, USA. doi:10.1520/D7566-20C
ATAG (2021). Waypoint 2050. Air Transport Action Group. Available at: https://aviationbenefits.org/environmental-efficiency/climate-action/waypoint-2050/.
Bell, D. C., Heyne, J. S., Won, S. H., and Dryer, F. L. (2018). “The Impact of Preferential Vaporization on Lean Blowout in a Referee Combustor at Figure of Merit Conditions,” in Proceedings of the ASME 2018 Power Conference collocated with the ASME 2018 12th International Conference on Energy Sustainability and the ASME 2018 Nuclear Forum, Lake Buena Vista, FL, USA, June 24–28, 2018 (ASME). doi:10.1115/POWER2018-7432
Boehm, R. C., Colborn, J. G., and Heyne, J. S. (2021). Comparing Alternative Jet Fuel Dependencies between Combustors of Different Size and Mixing Approaches. Front. Energ. Res. 9, 701901. doi:10.3389/fenrg.2021.701901
Boeing (2021). Boeing Commits to Deliver Commercial Airplanes Ready to Fly on 100% Sustainable Fuels. Available at: https://boeing.mediaroom.com/2021-01-22-Boeing-Commits-to-Deliver-Commercial-Airplanes-Ready-to-Fly-on-100-Sustainable-Fuels (Accessed December 8, 2021).
CAAFI R&D Team (2019). Prescreening of Synthesized Hydrocarbons Intended for Candidates as Blending Components for Aviation Turbine Fuels (Aka Alternative Jet Fuels or AJF). Available at: https://www.caafi.org/tools/docs/CAAFI_RD_Prescreening_Guidance_Document_v1.0.pdf.
CAAFI (2021). About CAAFI. Available at: https://caafi.org/about/caafi.html.
Colket, M. B., Heyne, J., Rumizen, M., Edwards, J. T., Gupta, M., Roquemore, W. M., et al. (2016). An Overview of the National Jet Fuels Combustion Program. AIAA J., 1087–1104. doi:10.2514/1.J055361
Colket, M., and Heyne, J. (2021). Fuel Effects on Operability of Aircraft Gas Turbine Combustors. Prog. Astronautics Aeronautics, 1–534. doi:10.2514/4.106040
Csonka, S. (2020). Aviation’s Market Pull for SAF (Sustainable Aviation Fuel). Available at: https://www.caafi.org/focus_areas/docs/CAAFI_SAF_Market_Pull_from_Aviation.pdf (Accessed September 3, 2021).
de Castro, C., Carpintero, Ó., Frechoso, F., Mediavilla, M., and de Miguel, L. J. (2014). A Top-Down Approach to Assess Physical and Ecological Limits of Biofuels. Energy 64 (1), 506–512. doi:10.1016/j.energy.2013.10.049
Edwards, J. (2017). “Reference Jet Fuels for Combustion Testing,” in 55th AIAA Aerospace Sciences Meeting, Grapevine, TX, January 9-13, 2017 (American Institute of Aeronautics and Astronautics). Available at: https://www.caafi.org/news/pdf/Edwards_AIAA-2017-0146_Reference_Jet_Fuels.pdf.
European Union (2021). Voluntary Schemes. Available at: https://ec.europa.eu/energy/topics/renewable-energy/biofuels/voluntary-schemes_en.
Fleming, G., and de Lepinay, I. (2019). Environmental Trends in Aviation to 2050. International Civil Aviation Organization (ICAO). Available at: https://www.icao.int/environmental-protection/Documents/EnvironmentalReports/2019/ENVReport2019_pg17-23.pdf.
Gomiero, T. (2015). Are Biofuels an Effective and Viable Energy Strategy for Industrialized Societies? A Reasoned Overview of Potentials and Limits. Sustainability 7 (7), 8491–8521. doi:10.3390/su7078491
Graham, J. L., Rahmes, T. F., Kay, M. C., Belières, J.-P., Kinder, J. D., Millett, S. A., et al. (2013). Impact of Alternative Jet Fuel and Fuel Blends on Non- Metallic Materials Used in Commercial Aircraft Fuel Systems. Available at: https://www.faa.gov/about/office_org/headquarters_offices/apl/research/aircraft_technology/cleen/reports/media/Boeing_Alt_Fuels_Final.pdf.
Hemighaus, G., Boval, T., Bacha, J., Barnes, F., Franklin, M., Gibbs, L., et al. (2007). Aviation Fuels Technical Review. San Ramon, CA: Chevron Products Company.
Heminghaus, G., Boval, T., Bosley, C., Organ, R., Lind, J., Brouette, R., et al. (2006). Alternative Jet Fuels, Addendum 1 to Aviation Fuels Technical Review. San Ramon, CA: Chevron Corporation.
Hepperle, M. (2012). “Electric Flight – Potential and Limitations,” in AVT-209 Workshop on Energy Efficient Technologies and Concepts Operation, Lisbon, Portugal. Available at: https://www.sto.nato.int/publications/STO%20Meeting%20Proceedings/Forms/Meeting%20Proceedings%20Document%20Set/docsethomepage.aspx?ID=30995&FolderCTID=0x0120D5200078F9E87043356C409A0D30823AFA16F602008CF184CAB7588E468F5E9FA364E05BA5&List=7e2cc123-6186-4c30-8082-1ba072228ca7&RootFolder=%2Fpublications%2FSTO%20Meeting%20Proceedings%2FSTO%2DMP%2DAVT%2D209 (Accessed August 10, 2021).
Heyne, J., Rauch, B., Le Clercq, P., and Colket, M. (2021). Sustainable Aviation Fuel Prescreening Tools and Procedures. Fuel 290, 120004. doi:10.1016/j.fuel.2020.120004
IATA (2019). Aircraft Technology Roadmap to 2050. International AIr Transport Association (IATA). Available at: https://www.iata.org/contentassets/8d19e716636a47c184e7221c77563c93/technology20roadmap20to20205020no20foreword.pdf.
ICAO (2021a). Carbon Offsetting and Reduction Scheme for International Aviation (CORSIA). Montreal, Quebec, Canada. Available at: https://www.icao.int/environmental-protection/CORSIA/Pages/default.aspx.
ICAO (2021b). CORSIA Approved Sustainability Certification Schemes. Available at: https://www.icao.int/environmental-protection/CORSIA/Documents/ICAO%20document%2004%20-%20Approved%20SCSs.pdf.
ICAO (2021c). CORSIA Eligible Fuels. Available at: https://www.icao.int/environmental-protection/CORSIA/Pages/CORSIA-Eligible-Fuels.aspx.
ICAO (2021d). Feasibility of a Long Term Global Aspirational Goal for International Aviation. Available at: https://www.icao.int/environmental-protection/Pages/LTAG.aspx.
ICAO (2018). Sustainable Aviation Fuels Guide Version 2. Available at: https://www.icao.int/environmental-protection/Documents/Sustainable%20Aviation%20Fuels%20Guide_100519.pdf.
Lee, D. S., Fahey, D. W., Skowron, A., Allen, M. R., Burkhardt, U., Chen, Q., et al. (2021). The Contribution of Global Aviation to Anthropogenic Climate Forcing for 2000 to 2018. Atmos. Environ. 244, 117834. doi:10.1016/j.atmosenv.2020.117834
Malicier, V. (2021). Fuel Suppliers Urge Governments to Impose SAF Blending Mandates. Editor J. Fox (London, UK: S&P Global Platts).
McKinsey and Co (2020). Hydrogen-powered Aviation: A Fact-Based Study of Hydrogen Technology, Economics, and Climate Impact by 2050. Brussels: European Commission. Available at: https://www.fch.europa.eu/sites/default/files/FCH%20Docs/20200507_Hydrogen%20Powered%20Aviation%20report_FINAL%20web%20%28ID%208706035%29.pdf.
MODUK (2020). DEF STAN 91-091, Revision I12. MODUK - British Defense Standards (MODUK). Available at: https://www.academia.edu/28340693/Ministry_of_Defence_Defence_Standard_91_91.
Moore, R. H., Shook, M., Beyersdorf, A., Corr, C., Herndon, S., Knighton, W. B., et al. (2015). Influence of Jet Fuel Composition on Aircraft Engine Emissions: A Synthesis of Aerosol Emissions Data from the NASA APEX, AAFEX, and ACCESS Missions. Energy Fuels 29 (4), 2591–2600. doi:10.1021/ef502618w
Palmer, W. (2021). United Flies World’s First Passenger Flight on 100% Sustainable Aviation Fuel Supplying One of its Engines. GE Future of Flight. Available at: https://www.ge.com/news/reports/united-flies-worlds-first-passenger-flight-on-100-sustainable-aviation-fuel-supplying-one.
Peiffer, E. E., Heyne, J. S., and Colket, M. (2019). Sustainable Aviation Fuels Approval Streamlining: Auxiliary Power Unit Lean Blowout Testing. AIAA J. 57 (11), 4854–4862. doi:10.2514/1.J058348
Polek, G. (2021). OEMs All in on SAF Development. AIN Online. Available at: https://www.ainonline.com/aviation-news/air-transport/2021-06-01/oems-all-saf-development.
Rolls-Royce (2021). Rolls-Royce Conducts First Tests of 100% Sustainable Aviation Fuel for Use in Business Jets. Available at: https://www.rolls-royce.com/https://www.rolls-royce.com/media/press-releases/2021/01-02-2021-business-aviation-rr-conducts-first-tests-of-100-precent-sustainable-aviation-fuel.aspx (Accessed September 9, 2021).
Rumizen, M. A. (2021). Qualification of Alternative Jet Fuels. Front. Energ. Res. 9, 760713. doi:10.3389/fenrg.2021.760713
Schäfer, A. W., Barrett, S. R. H., Doyme, K., Dray, L. M., Gnadt, A. R., Self, R., et al. (2019). Technological, Economic and Environmental Prospects of All-Electric Aircraft. Nat. Energ. 4, 160–166. doi:10.1038/s41560-018-0294-x
Stachler, R., Heyne, J., Stouffer, S., and Miller, J. (2020). Lean Blowoff in a Toroidal Jet-Stirred Reactor: Implications for Alternative Fuel Approval and Potential Mechanisms for Autoignition and Extinction. Energy Fuels 34 (5), 6306–6316. doi:10.1021/acs.energyfuels.9b01644
State of California (2020). Low Carbon Fuel Standard Regulation. Sacramento, CA, USA: State of California.
U.S. White House (2021). FACT SHEET: Biden Administration Advances the Future of Sustainable Fuels in American Aviation. Washington, DC, USA. Available at: https://www.whitehouse.gov/briefing-room/statements-releases/2021/09/09/fact-sheet-biden-administration-advances-the-future-of-sustainable-fuels-in-american-aviation/(Accessed December 07, 2021).
US Environmental Protection Agency (2010). 40 CFR Part 80, Subpart M. Renewable Fuel Stnadard. USA. Available at: https://www.epa.gov/renewable-fuel-standard-program/regulations-and-volume-standards-renewable-fuel-standards (Accessed August 10, 2021).
Voigt, C., Kleine, J., Sauer, D., Moore, R. H., Bräuer, T., Le Clercq, P., et al. (2021). Cleaner Burning Aviation Fuels Can Reduce Contrail Cloudiness. Commun. Earth Environ. 2 (114). doi:10.1038/s43247-021-00174-y
Won, S. H., Rock, N., Lim, S. J., Nates, S., Carpenter, D., Emerson, B., et al. (2019). Preferential Vaporization Impacts on Lean Blow-Out of Liquid Fueled Combustors. Combustion and Flame 205, 295–304. doi:10.1016/j.combustflame.2019.04.008
Zschocke, A., Scheuermann, S., and Ortner, J. (2012). High Biofuel Blends in Aviation (HBBA): ENER/C2/2012/420-1 Final Report. Available at: https://ec.europa.eu/energy/sites/ener/files/documents/final_report_for_publication.pdf.
Keywords: ASTM fuel qualification, drop-in, fungible, sustainable aviation fuel, synthesized aviation fuel
Citation: Kramer S, Andac G, Heyne J, Ellsworth J, Herzig P and Lewis KC (2022) Perspectives on Fully Synthesized Sustainable Aviation Fuels: Direction and Opportunities. Front. Energy Res. 9:782823. doi: 10.3389/fenrg.2021.782823
Received: 24 September 2021; Accepted: 31 December 2021;
Published: 24 January 2022.
Edited by:
Umakanta Jena, New Mexico State University, United StatesReviewed by:
Ibukun Oluwoye, Murdoch University, AustraliaSgouris Sgouridis, Masdar Institute of Science and Technology, United Arab Emirates
Copyright © 2022 Kramer, Andac, Heyne, Ellsworth, Herzig and Lewis. This is an open-access article distributed under the terms of the Creative Commons Attribution License (CC BY). The use, distribution or reproduction in other forums is permitted, provided the original author(s) and the copyright owner(s) are credited and that the original publication in this journal is cited, in accordance with accepted academic practice. No use, distribution or reproduction is permitted which does not comply with these terms.
*Correspondence: Kristin C. Lewis, a3Jpc3Rpbi5sZXdpc0Bkb3QuZ292