- 1College of Nuclear Science and Technology, Xi’an Jiaotong University, Xi’an, China
- 2Wuhan Second Ship Design and Research Institute, Wuhan, China
The polymer-matrix nuclear radiation shielding material is an important component of nuclear power plants. However, its mechanical properties and shielding performance gradually deteriorate due to the long-term synergy of nuclear radiation and thermal effects, which brings hidden dangers to the safe operation of the device. Based on this problem, this article makes a comprehensive review. First, the degradation of mechanical properties and shielding performance of polymer-matrix nuclear radiation materials in service is briefly described. Then, the research methods adopted by scholars to study the change law of properties and performance are introduced, and the main existing difficulties encountered by the study are summarized. Finally, the physical mechanism of the change of material properties is explained in detail, and a reference approach to solving the problem is proposed.
Basic Principles of Material Mechanics and Shielding Properties
The polymer-matrix nuclear radiation shielding material is an important component of nuclear power plants, and its mechanical properties and shielding performance determine whether the device can operate safely. For polymer-matrix nuclear radiation shielding materials, polyethylene, resins, rubber, etc. are often used as the matrix. By adding boron carbide, lead, tungsten, and other reinforcing phase particles, they have good neutron and gamma-ray shielding effects (Singh and Badiger, 2014; Wang et al., 2015; Kim et al., 2014). The matrix material is a polymer structure with covalent bonds as the framework (Nambiar and Yeow, 2012), and the reinforcing phase particles are mostly elements with a higher neutron activation cross-section. When the material is in service in a nuclear power plant, its internal nuclei and extranuclear electrons will interact with the rays. Figure 1 shows the schematic diagram of the interaction of polymer-matrix nuclear radiation shielding materials with rays and the resulting effects. The interaction between the nuclei and the rays will produce a nuclide change, accompanied by the generation of secondary radiation (Alhajali et al., 2009; Trkov et al., 2009; Cai et al., 2018). The neutron activation reaction is a typical case. On one hand, the activation reaction will cause changes in the nuclides inside the material, and on the other hand, it will generate instantaneous and delayed gamma rays, making the shielding material a secondary radiation source, which will lead to the change of the material shielding effect. The direct or indirect interaction between extranuclear electrons and rays may cause the breakage of the molecular bonds of the matrix material (Zhang et al., 2000; Planes et al., 2009). The broken molecular bonds form new molecular chain structures through irradiation degradation and irradiation cross-linking. Low-dose irradiation can improve the mechanical properties of cross-linked polymer materials (Planes et al., 2009), while high-dose irradiation may cause the degradation of the mechanical properties of the material. In addition, the breakage of the molecular bonds of the matrix material can result in the generation of small-molecular-weight volatile gases, which take away the substances in the material and cause the degradation of the shielding effect of the material. The accumulation of delayed gamma rays generated by the reinforcing phase particles exacerbates the covalent bond breakage of the matrix material.
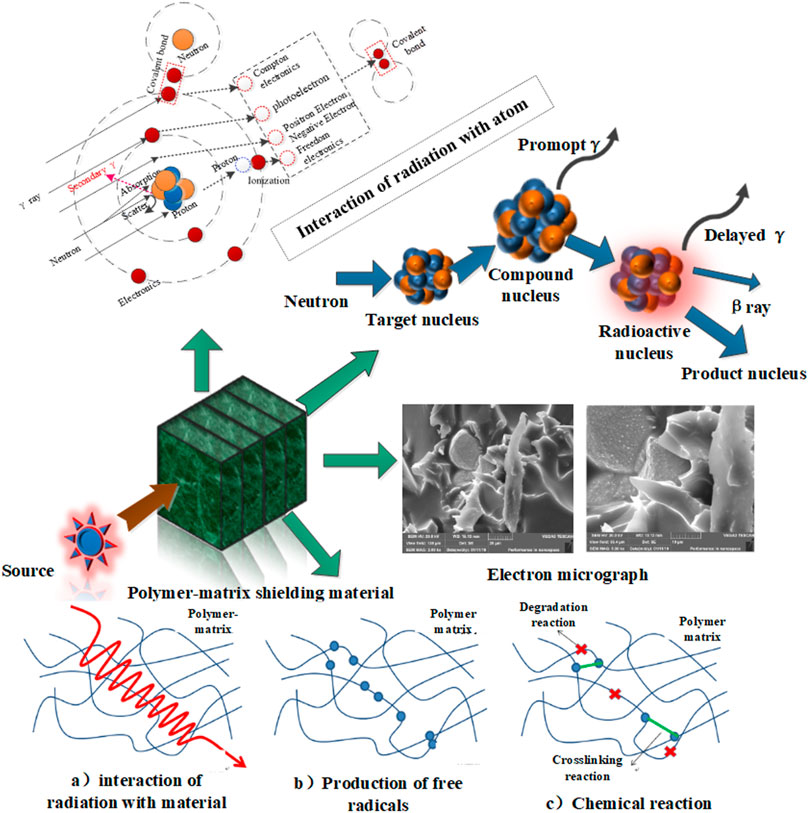
FIGURE 1. Schematic diagram of the interaction of polymer-matrix nuclear radiation shielding materials with rays and the resulting effects.
At the same time, the temperature of the external environment and the heat generated by the interaction between the rays and the material will act on the material. On one hand, it will change the cross-sections of the interaction between the thermal neutrons and the nuclei (Trumbull, 2006; Zu et al., 2018), and it indirectly affects the generation of secondary gamma rays. The relationship between thermal neutron cross-section and temperature is shown in Formula (1-1)
On the other hand, it will affect the reactions of irradiation degradation and irradiation cross-linking which occur after the molecular bonds are broken. Under the synergistic effect of nuclear radiation and thermal effects, polymer-matrix nuclear radiation shielding materials first experience the damage caused by the interaction of atomic-scale rays with the material nuclei and extranuclear electrons and then the evolution of the molecular scale and mesoscopic scale, and finally, it shows the change of macroscopic mechanical properties and shielding performance of the material. As the materials serve in nuclear power plants over time, the mechanical properties and shielding effect of the material will gradually degrade or even fail, which will result in hidden dangers to the safe operation of the nuclear power plant.
The Historical Development and Difficulties of the Research on This Problem
The Historical Development of the Research on This Problem
In the early days, researchers adopted techniques combining experiments with theoretical analysis to study the change law of the mechanical properties of polymers under an irradiation environment. For example, in 1953, the American Oak Ridge National Laboratory released the change of the mechanical properties of plastics and rubber with the dose of irradiation and discussed the stability of the material in the irradiation environment, which provided a reference for the safety estimation of the material in service (Bopp and Sissman, 1953). In 1969, based on the study of the corresponding relationship between multiple macroscopic property variables of materials and irradiation dose, Hanks and Hamman discussed the advantages and disadvantages of various electrical insulating materials in terms of radiation resistance and expanded the macroscopic property variables of the irradiation stability evaluation of polymer materials (Hanks and Hamman, 1969). Since 1979, the European Organization for Nuclear Research has released a series of research reports on the changes of mechanical properties of polymer materials under different radiation environments. These reports include commonly used thermosetting and thermoplastic polymers (Schönbacher and Stolarz-Izycka, 1979a; Schönbacher and Stolarz-Izycka, 1979b; Beynel and Schönbacher, 1982; Schönbacher and Tavlet, 1989; Tavlet et al., 1998).
As the shielding materials in nuclear power plants are required to be multifunctional and safe, they have also evolved from monocomponent to composite materials which use polymers as their matrix. Accordingly, scholars made research on the irradiation stability of polymer-matrix shielding materials, took into account the temperature factor on the basis of ray irradiation, and discussed the change of the mechanical properties of shielding materials under radiation with different intensities at different temperatures. For example, in 1989, Egusa S et al. explored the relationship between the mechanical properties of six typical materials and the absorbed dose at 77 K and room temperature (Egusa, 1988). In 2001, L. Vignoud et al. did research on influence of electron irradiation on the mobility and on the mechanical properties of DGEBA/TETA epoxy resins (Vignoud et al., 2001a; Vignoud et al., 2001b). In 2006 and 2007, the degradation of epoxy resins under high-energy electron beam irradiation was researched by N. Longieras et al. (Longiéras et al., 2006; Longiéras et al., 2007). In 2008, V.P. Laricheva researched the effect of ionizing radiation on epoxy oligomers of different structures and the manufacture of new promising materials on their base (Laricheva, 2008). In 2009, researchers from the Shanghai Institute of Applied Physics summarized the research progress of polymer high-temperature irradiation effects (Tang et al., 2009). In 2015, researchers at Nanjing University of Aeronautics and Astronautics studied the performance of resisting irradiation damage and interface stability of copper-nanographene composites at temperatures of 300, 500, and 700 K (Huang et al., 2015). Besides, research on the irradiation effect and irradiation stability of functional composites based on nano/graphene modification is being carried out (Jovanović et al., 2015; Kolanthai et al., 2015) so that a large number of related research techniques have been accumulated.
The changes in the mechanical properties of shielding materials in service determine whether they can be used safely, and the changes in shielding performance decide whether the materials are still effective. During service, changes of nuclides inside the material and secondary rays generated by activation reaction will cause changes in the shielding performances of the material. Some research on the calculation of material nuclide changes has been carried out, such as the burnup calculation of PWR components based on NECP-CACTI and UNICORN and the burnup analysis calculation of the molten salt fast reactor based on MCNP and Origen (Wan et al., 2017). In the research of shielding material activation, researchers from Xi’an Jiaotong University used MCNP6 to calculate and analyze the neutron activation products and radioactivity of Fe, Al, and other reinforcing phase particles under an irradiation environment. According to the demand of D-T neutron source shielding materials, we designed a series of low-activation, light, and compact epoxy resin matrix nuclear radiation shielding materials (Cai et al., 2018).
In recent years, the prediction of material performance in an irradiation environment by adopting multi-scale evolution techniques has become the focus of many scholars. For example, multi-scale modeling and simulation of the aging of polymer-matrix materials in a radiation environment were added for the first time in the international conference on multi-scale modeling and simulation of materials held by the University of California, Los Angeles, in 2004. Through analyzing the microscopic damage of the materials and with the help of theoretical calculations at different scales, the macroscopic properties of the materials were predicted (Odette and Wirth, 2005). In 2011, the United States announced the launch of the Material Genome Project, establishing an accurate material performance prediction model, and correcting the model based on theoretical and empirical data is one of its important contents (Jain et al., 2013). In 2012, NASA made it clear that the radiation damage of polymer composite materials in the cosmic radiation environment was a technical requirement in medium and long-term planning and carried out some exploratory research using the multi-scale coupling technology (Piascik et al., 2012). In the same year, Sandia National Laboratory in the United States also regarded the irradiation damage of neutrons and gamma rays to organic materials as an important research project (White and Bernstein, 2012). In China, researchers have also devoted themselves to analyzing multi-scale modeling and simulation of materials. Liu CS et al. used first principles to calculate the generation and diffusion of hydrogen in tungsten materials under a radiation environment (Kong et al., 2015). Liu H et al. used a multi-scale modeling and simulation method to simulate the curing reaction and the network structure of a typical epoxy resin system (Liu et al., 2011). Li M et al. adopted a multi-scale modeling and simulation method to study the interface formation process of carbon fiber/epoxy composites (Li et al., 2013).
The fractal method is an effective technique used in multi-scale modeling, which is divided into regular fractal and random fractal. Regular fractal methods have strict similarity, while random modeling methods only have statistical similarity (Kozlov et al., 2014). Radom fractal uses the fractal dimension to evaluate the macroscopic performance of the material. The fractal dimension can be obtained by fitting the relationship between the length and the number of microscopic damage small units through the box-counting dimension (Kozlov and Yanovskii, 2014). Based on fractal methods, some scholars have studied the macroscopic properties of composite materials. For example, Gianluca adopted the fractal method to study the effective thermal conductivity of salt/expanded graphite composite materials (Gianluca and Massimiliano, 2015), and on the basis of fractal theory, Xu Qiang et al. established a multi-scale numerical simulation model of concrete material performance (Xu et al., 2016).
The Difficulties of the Research on This Problem
In summary, in response to different service environments and backgrounds, researchers have carried out a lot of research work on the performance changes of materials, providing technical means and an experimental basis for the performance evaluation of materials. However, for polymer-matrix nuclear radiation shielding materials used in nuclear power plants, due to the dual effects of nuclear radiation and high temperature, the damage mechanism is relatively complicated, and there is a large-scale span from the starting point of material damage to the macroscopic property change process, which involves many physical and chemical processes. What is more, the gas generation during the evolution of mechanical properties affects the shielding performance of the material, and the problem of the accumulation of delayed gamma rays during the evolution of the shielding performance affects the mechanical properties of the material. Therefore, there is relatively little research on the modeling of the changing law of mechanical properties and shielding performance of polymer-matrix nuclear radiation shielding materials under the synergistic effect of nuclear radiation and thermal effects, which is rather challenging.
Physical Mechanism for the Change of Properties and Performance of Shielding Materials and its Solution
In response to this complex problem, this section will analyze the microscopic damage mechanism of the properties and performance changes of the materials caused by the synergy of nuclear radiation and thermal effects as shown in Figure 2. The matrix material is a polymer structure with covalent bonds as the framework. When the incident neutrons, gamma rays, and the generated secondary rays interact with the extranuclear electrons that form the covalent bond, the covalent bond breaks and forms free radicals. The free radicals are active; thus, irradiation cross-linking and irradiation degradation reactions will occur. In this process, a new molecular structure is formed and radioactive gases may be generated. The variation in molecular structure can lead to changes in the mechanical properties of the matrix material. During the interaction between the rays and the materials, heat will be released. The released heat and the external temperature will jointly affect not only the cross-sections of interactions between the rays and the extranuclear electrons of the matrix materials but also the irradiation cross-linking and irradiation degradation reactions of free radicals.
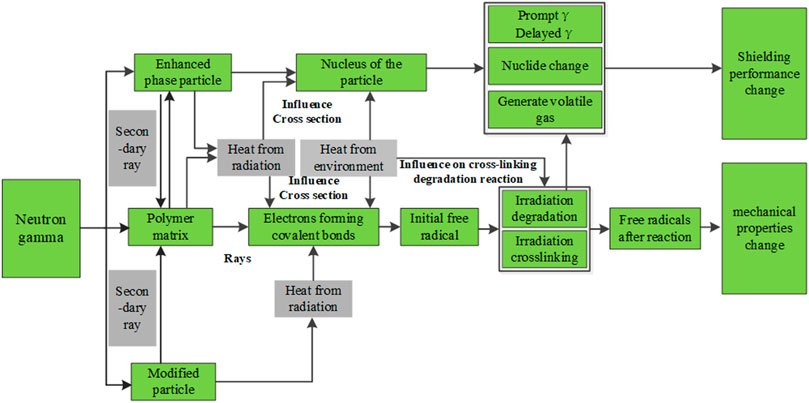
FIGURE 2. Damage mechanism analysis of the materials under the synergy of nuclear radiation and temperature.
The reinforcing phase particles are mostly elements with high neutron activation cross-sections. After the neutrons interact with the reinforcing phase particle nuclei, the nuclides of the reinforcing phase particles will change, accompanied by the generation of instantaneous and delayed gamma rays. These physical processes, together with the voids and volatilization of substances caused by gas generation in the matrix material, will result in changes of the shielding performance of the materials. Similarly, the cross-sections of the interaction between the neutrons and the reinforcing phase particles nuclei are also affected by the heat generated by the interaction between the radiation and the materials as well as the external environmental temperature. In the research, only the processes that have a greater impact on the properties and performance of the materials are initially considered, such as the interactions between the rays and the electrons that form covalent bonds in the matrix materials and those between the rays and the reinforcing phase particles’ nuclei. Processes such as the interactions between the rays and the matrix material nuclei, the photonuclear reaction of neutrons generated by the interaction between gamma rays and materials, and the changes in the mechanical properties of the reinforcing particles after they interact with rays are considered as influencing factors after modeling. The changes in the mechanical properties of the materials are mainly caused by the interactions between the matrix materials and the rays, and the changes in the shielding performance of the materials mostly result from the interactions between the reinforcing phase particles and the rays. Therefore, in the modeling process, the matrix materials and the reinforcing phase particles are initially considered in a separate way. After establishing a certain relationship, these two factors are combined through the equivalent physical property model of the composite materials and other models.
Finally, a solution is proposed and specified as follows: with regard to the changes of mechanical properties of materials, first, the type of instantaneous secondary rays and energy intensity generated by the interaction between incident neutrons, gamma rays, and materials are simulated. The relationship between the temperature and the interaction cross-sections of the rays and the covalently bonded electrons in the matrix materials is established. The energy equivalent method is used to calculate the heat generated by the interaction between the rays and the materials, and then, combined with the external environment temperature, the relationship is established between the temperatures and probabilities of interactions between the incident neutrons, gamma rays, instantaneous secondary rays, and the covalently bonded electrons in the matrix material. After that, by further considering the calculation results of the delayed gamma rays generated by the reinforcing phase particle activation reaction, the initial concentration, types, and molecular structure information of free radicals can be calculated. Multi-scale simulation software such as the molecular dynamics method, Monte Carlo method, or fractal method can be adopted separately in order to establish the relationship between free radical information and macroscopic mechanical properties of the shielding materials at different temperatures. In terms of shielding performance of the materials, the energy equivalent method can be utilized to calculate the heat generated by the interactions between the rays and the materials, and then, combined with the external environment temperature, the relationship is established between temperatures and cross-sections of interactions between the nuclei of reinforcing phase particles, the incident neutrons, and gamma rays at different temperatures. The reinforcing phase material nuclides change information, and generated secondary gamma rays are calculated, including instantaneous secondary gamma rays and delayed secondary gamma rays. By combining with the volatile gas information generated by the matrix material, the relationship between shielding performance of the materials and the nuclide changes, the amount of generated secondary gamma rays, and the volatile gas generated by the matrix materials at different temperatures is calculated. This research, on one hand, can establish the mapping relations between the properties and performance of the materials and their service environment, composition, and molecular configuration. Therefore, it can provide technical measures for the methodological study on material properties and performance improvement, such as the nanoparticle modification technology, the graphene modification technology, and the design and development of new materials as well. On the other hand, this research can provide a technical basis for the safety assessment on the long-term service of polymer-matrix nuclear radiation shielding materials in nuclear power plants.
Conclusion
The mechanical properties and shielding performance of the polymer-matrix nuclear radiation shielding material are gradually deteriorated due to the long-term synergy of nuclear radiation and thermal effects, which brings hidden dangers to the safe operation of the device. In this article, the development of the degradation of mechanical properties and shielding performance of polymer-matrix nuclear radiation materials in service is briefly described. Then, the difficulties of this problem are analyzed. On this basis, this problem is analyzed level by level. Taking the changes of mechanical properties and shielding properties of shielding materials as the mainline, the implied rays without material interactions and the interaction between heat and the material are analyzed. Finally, a reference approach to solve the problem is proposed.
Author Contributions
All authors listed have made a substantial, direct, and intellectual contribution to the work and approved it for publication.
Funding
This research is supported by the National Natural Science Foundation of China (11975182), General Projects of Shaanxi Provincial Nature Fund (2020JM-030), the NSAF Joint Fund set up by the National Natural Science Foundation of China and the Chinese Academy of Engineering Physics under Grant U1830128, and the Postdoctoral Science Foundation of China (2018M633521)
Conflict of Interest
The authors declare that the research was conducted in the absence of any commercial or financial relationships that could be construed as a potential conflict of interest.
Publisher’s Note
All claims expressed in this article are solely those of the authors and do not necessarily represent those of their affiliated organizations or those of the publisher, the editors, and the reviewers. Any product that may be evaluated in this article or claim that may be made by its manufacturer is not guaranteed or endorsed by the publisher.
References
Alhajali, S., Kharita, M. H., Naoom, B., Yousef, S., and AlNassar, M. (2009). Estimation of the Activation of Local Reactor Shielding Concretes. Prog. Nucl. Energ. 51 (1), 374–377. doi:10.1016/j.pnucene.2008.04.007
Beynel, P. P. M., and Schönbacher, H. (1982). Compilation of Radiation Damage Test Data, Part 3 Materials Used Around High-Energy accelerators[R]. Geneva: Health and Safety Division.
Cai, Y., Hu, H., Lu, S., and Jia, Q. (2018). Optimization of Radiation Shielding Material Aiming at Compactness, Lightweight, and Low Activation for a Vehicle-Mounted Accelerator-Driven D-T Neutron Source. Appl. Radiat. Isot. 135 (1), 147–154. doi:10.1016/j.apradiso.2018.01.021
Egusa, S. (1988). Mechanism of Radiation-Induced Degradation in Mechanical Properties of Polymer Matrix Composites. J. Mater. Sci. 23 (1), 2753–2760. doi:10.1007/bf00547447
Gianluca, A., and Massimiliano, Z. (2015). Theoretical Study on Effective thermal Conductivity of Salt/Expanded Graphite Composite Material by Using Fractal Method[J]. Commun. Nonlinear Sci. Numer. Simulation 22 (1-3), 889–902.
Hanks, C., and Hamman, D. (1969). The Effect of Radiation on Electrical Insulating Materials[R]. Columbus: DTIC Document.
Huang, H., Tang, X., Chen, F., Yang, Y., Liu, J., Li, H., et al. (2015). Radiation Damage Resistance and Interface Stability of Copper-Graphene Nanolayered Composite. J. Nucl. Mater. 460 (1), 16–22. doi:10.1016/j.jnucmat.2015.02.003
Jain, A., Ong, S. P., Hautier, G., Chen, W., Richards, W. D., Dacek, S., et al. (2013). Commentary: The Materials Project: A Materials Genome Approach to Accelerating Materials Innovation. APL Mater. 1 (1), 011002–101102211. doi:10.1063/1.4812323
Jovanović, V., Samaržija-Jovanović, S., Petković, B., Dekić, V., Marković, G., Marinović-Cincović, M., et al. (2015). Effect of γ-irradiation on the Hydrolytic and thermal Stability of Micro- and Nano-TiO2 Based Urea–Formaldehyde Composites[J]. RSC Adv. 5, 59715–59722. doi:10.1039/c5ra10627c
Kim, J., Lee, B.-C., Uhm, Y. R., and Miller, W. H. (2014). Enhancement of thermal Neutron Attenuation of Nano-B4c, -BN Dispersed Neutron Shielding Polymer Nanocomposites. J. Nucl. Mater. 453 (1-3), 48–53. doi:10.1016/j.jnucmat.2014.06.026
Kolanthai, E., Bose, S., Bhagyashree, K. S., Bhat, S. V., Asokan, K., Kanjilal, D., et al. (2015). Graphene Scavenges Free Radicals to Synergistically Enhance Structural Properties in a Gamma-Irradiated Polyethylene Composite through Enhanced Interfacial Interactions. Phys. Chem. Chem. Phys. 17, 22900–22910. doi:10.1039/c5cp02609a
Kong, X.-S., Wang, S., Wu, X., You, Y.-W., Liu, C. S., Fang, Q. F., et al. (2015). First-principles Calculations of Hydrogen Solution and Diffusion in Tungsten: Temperature and Defect-Trapping Effects. Acta Materialia 84 (1), 426–435. doi:10.1016/j.actamat.2014.10.039
Kozlov, G. V., Mikitaev, A. K., Zaikov, G. E., Scotti, S., Stewart, J., and Calomino, A. (2014). The Fractal Physics of Polymer Synthesis[M]. America Apple Academic Press.
Kozlov, G. V., and Yanovskii, Y. G. (2014). Fractal Mechanics of Polymers: Chemistry and Physics of Complex Polymeric Materials[M]. America Apple Academic Press.
Laricheva, V. P. (2008). Effect of Ionizing Radiation on Epoxy Oligomers of Different Structures and Manufacture of New Promising Materials on Their Base. Radiat. Phys. Chem. 77, 29–33. doi:10.1016/j.radphyschem.2007.02.033
Li, M., Gu, Y.-Z., Liu, H., Li, Y.-X., Wang, S.-K., Wu, Q., et al. (2013). Investigation the Interphase Formation Process of Carbon Fiber/epoxy Composites Using a Multiscale Simulation Method. Composites Sci. Tech. 86, 117–121. doi:10.1016/j.compscitech.2013.07.008
Liu, H., Li, M., Lu, Z.-Y., Zhang, Z.-G., Sun, C.-C., and Cui, T. (2011). Multiscale Simulation Study on the Curing Reaction and the Network Structure in a Typical Epoxy System. Macromolecules 44 (21), 8650–8660. doi:10.1021/ma201390k
Longiéras, N., Sebban, M., Palmas, P., Rivaton, A., and Gardette, J. L. (2007). Degradation of Epoxy Resins under High Energy Electron Beam Irradiation: Radio-Oxidation. Polym. Degrad. Stab. 92, 2190–2197. doi:10.1016/j.polymdegradstab.2007.01.035
Longiéras, N., Sebban, M., Palmas, P., Rivaton, A., and Gardette, J. L. (2006). Multiscale Approach to Investigate the Radiochemical Degradation of Epoxy Resins under High-Energy Electron-Beam Irradiation. J. Polym. Sci. A. Polym. Chem. 44, 865–887. doi:10.1002/pola.21176
Nambiar, S., and Yeow, J. T. W. (2012). Polymer-Composite Materials for Radiation Protection. ACS Appl. Mater. Inter. 4 (11), 5717–5726. doi:10.1021/am300783d
Odette, G. R., and Wirth, B. D. (2005). Radiation Effects in Fission and Fusion Reactors. Handbook Mater. Model., 999–1037. doi:10.1007/978-1-4020-3286-8_50
Piascik, B., Vickers, J., and Lowry, D. (2012). Materials, Structure, Mechanical Systems and Manufacturing Roadmap Technology area[R]. Washington: National Aeronautics and Space Administration.
Planes, E., Chazeau, L., Vigier, G., Chenal, J.-M., and Stuhldreier, T. (2009). Crystalline Microstructure and Mechanical Properties of Crosslinked EPDM Aged under Gamma Irradiation. J. Polym. Sci. B Polym. Phys. 48 (2), 97–105. doi:10.1002/polb.21848
Schönbacher, H., and Stolarz-Izycka, A. (1979). Compilation of Radiation Damage Test Data, Part 1 Cable Insulation materials[R]. Geneva: Health and Safety Division.
Schönbacher, H., and Stolarz-Izycka, A. (1979). Compilation of Radiation Damage Test Data, Part 2 Thermosetting and Thermoplastic resins[R]. Geneva: Health and Safety Division.
Schönbacher, H., and Tavlet, M. (1989). Compilation of Radiation Damage Test Data, Halogen-free cable-insulating materials[R]. Geneva: Health and Safety Division.
Singh, V. P., and Badiger, N. M. (2014). Gamma ray and Neutron Shielding Properties of Some alloy Materials. Ann. Nucl. Energ. 64 (1), 301–310. doi:10.1016/j.anucene.2013.10.003
Tang, Z., Wang, M., and Zhao, Y. (2009). Research Progress of High Temperature Irradiation Effect of Polymer. J. Mater. Rev. 23 (9), 9–12.
Tavlet, M., Fontaine, A., and Schönbacher, H. (1998). Compilation of Radiation Damage Test Data, Part 2 Thermosetting and Thermopastic Resins, Composite materials[R]. Geneva: Health and Safety Division.
Trkov, A., Žerovnik, G., Snoj, L., and Ravnik, M. (2009). On the Self-Shielding Factors in Neutron Activation Analysis. Nucl. Instr. Methods Phys. Res. Section A: Acc. Spectrometers, Detectors Associated Equipment 610 (2), 553–565. doi:10.1016/j.nima.2009.08.079
Trumbull, T. H. (2006). Treatment of Nuclear Data for Transport Problems Containing Detailed Temperature Distributions. Nucl. Tech. 156 (1), 75–86. doi:10.13182/nt156-75
Vignoud, L., David, L., Sixou, B., and Vigier, G. (2001). Influence of Electron Irradiation on the Mobility and on the Mechanical Properties of DGEBA/TETA Epoxy Resins. Polymer 42, 4657–4665. doi:10.1016/s0032-3861(00)00791-6
Vignoud, L., David, L., Sixou, B., Vigier, G., and Stevenson, I. (2001). Effect of Electron Irradiation on the Mechanical Properties of DGEBA/DDM Epoxy Resins. Nucl. Instr. Methods Phys. Res. Section B: Beam Interactions Mater. Atoms 185, 336–340. doi:10.1016/s0168-583x(01)00758-3
Wan, C., Cao, L., Wu, H., and Shen, W. (2017). Nuclear-data Uncertainty Propagations in Burnup Calculation for the PWR Assembly. Ann. Nucl. Energ. 100 (2), 20–31. doi:10.1016/j.anucene.2016.09.037
Wang, P., Tang, X., Chai, H., Chen, D., and Qiu, Y. (2015). Design, Fabrication, and Properties of a Continuous Carbon-Fiber Reinforced Sm 2 O 3/polyimide Gamma ray/neutron Shielding Material. Fusion Eng. Des. 101 (1), 218–225. doi:10.1016/j.fusengdes.2015.09.007
White, G. V. I. I., and Bernstein, R. (2012). Neutron and Gamma Irradiation Damage to Organic materials[R]. Albuquerque: SANDIA.
Xu, Q., Chen, J. Y., Li, J., and Wang, M. (2016). Multi-scale Numerical Model for Simulating concrete Material Based on Fractal Theory[J]. Acta Mechanica Solida Sinica 26 (4), 344–352. doi:10.1016/s0894-9166(13)60031-2
Zhang, Z., Ge, X., and Zhang, M. (2000). Radiation Chemistry of Polymers [M]. Beijing: China University of science and Technology Press.
Keywords: polymer-matrix shielding materials, coupling of heat and radiation, mechanical properties, shielding performance, changing law
Citation: Hu G, Sun W, Yan Y, Wu R and Xu H (2021) Review on the Change Law of the Properties and Performance of Polymer-Matrix Nuclear Radiation Shielding Materials Under the Coupling of Nuclear Radiation and Thermal Effects. Front. Energy Res. 9:777956. doi: 10.3389/fenrg.2021.777956
Received: 16 September 2021; Accepted: 25 October 2021;
Published: 23 November 2021.
Edited by:
Wenzhong Zhou, Sun Yat-sen University, ChinaReviewed by:
Sreedha Sambhudevan, Amrita Vishwa Vidyapeetham University, IndiaYizhuo Gu, Beihang University, China
Sudhir Amritphale, Louisiana Tech University, United States
Copyright © 2021 Hu, Sun, Yan, Wu and Xu. This is an open-access article distributed under the terms of the Creative Commons Attribution License (CC BY). The use, distribution or reproduction in other forums is permitted, provided the original author(s) and the copyright owner(s) are credited and that the original publication in this journal is cited, in accordance with accepted academic practice. No use, distribution or reproduction is permitted which does not comply with these terms.
*Correspondence: Guang Hu, guanghu@mail.xjtu.edu.cn; Hu Xu, xuhu_xu@126.com