- 1State Key Laboratory of Pulp and Paper Engineering, School of Light Industry and Engineering, South China University of Technology, Guangzhou, China
- 2Guangxi Key Laboratory of Clean Pulp and Papermaking and Pollution Control, College of Light Industry and Food Engineering, Guangxi University, Nanning, China
Tricin as a monomer of grass lignin with unique biological properties is beneficial to human health with the potential for various applications. The abundant grass lignin could be an alternative source for tricin if an effective separation method is available. In this study, we used different lignin preparations, including alkali lignin (AL), mild acidolysis lignin (MAL), cellulase enzymatic lignin (CEL), γ-valerolactone lignin (GVL), and organosolv lignin (OL), to investigate the effect of different fractionation methods on the tricin content of the wheat straw lignin. The tricin signal of different lignins can be clearly identified by 2D heteronuclear singular quantum correlation (HSQC) spectra. GVL showed the highest tricin level among these lignin samples as the tricin content of GVL was accounted to be 8.6% by integrals. The tricin content was carefully determined using thioacidolysis combined with high-performance liquid chromatography-mass spectrometric (HPLC-MS), and the quantitative results of tricin by HPLC-MS were basically consistent with that of 2D HSQC integrals. Both methods have proved that the tricin contents of lignins isolated under acid conditions were significantly higher than that of AL. In addition, the determination of the sun protection factors (SPF) of lignin-based sunscreen and antioxidant activity of lignin preparations indicated that reserving more tricin was beneficial to the UV resistance of lignin samples. Therefore, this study not only provides new insights for the extraction methods of lignin with high tricin content but also is beneficial to the future study on the application of tricin and tricin-lignin.
Introduction
Lignin, a natural and renewable aromatic polymer with complex structures, is primarily distributed in the secondary plant cell walls. The content of lignin in timber plants is 20–40%, whereas it is generally lower in Gramineae, about 15–25%. Conventional lignin is composed of guaiacyl (G), syringyl (S), and p-hydroxyphenyl (H) units, which are, respectively, derived from coniferyl alcohol, sinapyl alcohol, and p-coumaryl alcohol and differed by the methoxylation degree on the aromatic ring (Boerjan et al., 2003; Ralph, 2009). Recent studies revealed that, in addition to these three monolignols, lignin also contained many other unconventional monomers, such as tricin, piceatannol, feruloyltyramine, and caffeyl alcohol (Chen et al., 2021; del Río et al., 2020; Vanholme et al., 2019).
Among these newly discovered monomers, tricin is one of the most interesting ones because it is the first phenolic from outside the monolignol biosynthetic pathway which is found to be integrated into the lignin polymer (Lan et al., 2015). Tricin has long been known as a secondary metabolite in monocots, which is extractable by organic solvents. In recent years, it was first disclosed to be present in the lignin preparation from wheat straw (del Rio et al., 2012) and was later authenticated as a monomer in grass lignin (Lan et al., 2015). Structural characterization of biomimetic radical coupling reaction proved that tricin linked with other monolignols exclusively through β–O–4 interlinkage and therefore only occurred at the starting point, i.e., serving as a nucleation site, of lignin chain. Chemical structures of tricin and linkages between tricin and other lignin units are provided by Figure 1 (Lan et al., 2016). Compared with other compounds with similar structure, the potential application of tricin is distinctly higher in the aspect of agricultural, nutraceutical, and medicinal activities (Zhou and Ibrahim, 2009), considering its biological activities including antioxidant, antiviral, anticancer, antiaging, and hepatoprotective activities (Ogo et al., 2013; Oyama et al., 2009; Solyanik et al., 2021). Structure-activity relationship study demonstrates that the phenolic hydroxyl, ketone, and carboxyl group display a strong absorption of ultraviolet (Cao et al., 1997; Heim et al., 2002). As for tricin, the long resonance structure formed by two aromatic rings and the C=C and carbonyl in between, as well as the intramolecular hydrogen bonds, further enhances the UV absorption ability, antioxidation, and antibacterial properties, making it a natural UV protector and antioxidant with great potential for use in daily protective products (del Río et al., 2020; Lan et al., 2016; Oyama et al., 2009). On the other hand, natural tricin is featured by premium pricing because of the low content in the extractives from plants and difficulty in purification (Li et al., 2016). Therefore, the abundant grass lignin could be an alternative source for tricin if an effective separation method is available.
At present, certain straws of crops are underutilized as many agricultural residues are treated as wastes. Some of them were even burned and therefore cause serious pollution in the rural area (Kim and Dale, 2004; Sarkar et al., 2012). Therefore, more efforts should be made to take full advantage of the agricultural residues considering environmental and economic aspects. Wheat is one of the most important cereal crops. The annual production of wheat is about 134 million tons and resulting in close to 175 million tons of wheat straw per year calculated by the Food and Agriculture Organization (FAO) standards in 2020 in China according to data provided by the Ministry of Agriculture of the PRC. The previous study showed that wheat straw contains the highest amount of tricin compared with other plants which could be a promising source for natural tricin (Lan et al., 2016).
Although tricin has been confirmed in the lignin from Gramineae plants and the amount has been measured by thioacidolysis followed by HPLC-MS (Lan et al., 2016), its stability under different conditions is still unclear. For example, tricin would be depleted with increasing acid concentration or pretreatment temperature after reaching a critical condition, whereas most could be preserved under mild diluted acid treatment conditions (Jensen et al., 2017; Lin W. et al., 2021). At a low-temperature alkali pretreatment (70°C, 18 h), the content of tricin in lignin isolated from wheat straw was much lower than that in the untreated samples (Zikeli et al., 2016). On the contrary, tricin can be retained well under some mild conditions such as mild acid γ-valerolactone lignin (GVL) pretreatment (Luterbacher et al., 2014). Furthermore, tricin in the waste liquor of conventional pulping mostly disappeared (Jiang et al., 2018; Lauwaert et al., 2019). To take the best advantage of tricin for the subsequent application, it is important to develop a lignin fractionation method that can mostly reserve tricin in isolated lignin.
In this study, we explore the effect of different fractionation methods on the tricin content of the lignin components, including alkali lignin (AL) (from both ball-milled and nonball-milled samples), mild acidolysis lignin (MAL), cellulase enzymatic lignin (CEL), GVL, and organosolv lignin (OL). 2D heteronuclear singular quantum correlation (HSQC) spectra were used to characterize the lignin structure and HPLC-MS was applied to quantify tricin in the lignin preparations. The sun protection factors (SPF) of lignin-based sunscreen and antioxidation property of lignin preparations were also determined to evaluate the correlation between tricin content and the abilities of anti-UV radiation and antioxidation.
Experimental
Materials
The following reagents were used: l-cysteine (99%), NaOH (97%), 1,4-dioxane (98%), cellulase (hydrolyzing activity is >1000 U/g), viscozyme L (a cellulase with enzymatic activity value of 100 FBG/g), γ-valerolactone (98%), methanol (≥99.9%, HPLC level), and acetonitrile (≥99.9%, HPLC level). All chemicals used in this study were purchased from Macklin except the cellulase was from Sigma and viscozyme L from Novozymes.
The wheat straw collected in Jiaxiang county, Shandong province, was physically cut, crushed, and sieved to obtain the 40–60 mesh fraction. The crushed wheat straw was dewaxed in 80% ethanol (v/v) at 80°C for 8 h. This step was repeated twice and then followed by extraction in dichloromethane at 40°C for 8 h (Li et al., 2019). The final dewaxed sample was air-dried in the fume hood and then stored in a desiccator for later use.
Measurement of Lignin Content
The lignin content of wheat straw was determined by the l-cysteine assisted dissolution method as reported previously (Lu et al., 2021). A 0.1 g/mL l-cysteine stock solution was prepared by dissolving 1.0 g of l-cysteine in 10 ml 72% H2SO4. 0.3 g of the absolutely dried lignocellulosic sample was added to 10 ml of l-cysteine solution at 60°C in a sand bath with continuous stirring for 30 min to dissolve lignin. 105 ml of deionized water was subsequently added to the reaction to dilute the solution. The content of lignin was calculated using Bill’s formula (1):
where Abs’ is the UV absorption values at 283 nm; Vf (ml) is the volume after dilution, Vf = 115 ml; ɛ (L/gcm) is the UV absorptivity, ɛ = 15.0; ms (mg) is the mass of absolutely dried lignin; p (cm) is the optical path length, p = 1 cm; δ is the absolute dryness, δ = 1.
The lignin content of wheat straw was 16.46% ± 0.31 according to our determination.
Lignin Extraction
Alkali lignin (AL)
5 g of the dewaxed wheat straw was mixed uniformly with 1 M NaOH solution in a solid-to-liquid ratio of 1:10 (g/ml) at 30°C. After 4 h, the liquor was collected by filtration, and the pH was adjusted to 3.0 by 1.0 M HCl solution. The neutralized liquor was placed in a fridge at 4°C for more than 12 h to let the lignin precipitate. The AL was separated by centrifugation and then freeze-dried to give a light brown powder. A control experiment was performed with ball-milled at 10 h wheat straw sample under the same reaction condition at 30°C.
Mild acidolysis lignin (MAL)
20 g of dewaxed wheat straw was added in a 1,4-dioxane/H2O (9:1, v/v) solution with 0.2 M HCl at a solid-to-liquid ratio of 1:12 (g/ml). The reaction mixture was refluxed in a 120°C sand bath with magnetic stirring (400 rpm) for 30 min. After cooling to room temperature, the liquor was collected by filtration and the pH value was adjusted to about 3.0 by saturated NaHCO3 solution. The liquor was then concentrated to about 50 ml under reduced pressure at 45°C. The concentrated liquor was poured into 500 ml of deionized ice water to precipitate the lignin. The raw MAL was obtained by centrifugation and lyophilization.
Cellulase enzymatic lignin (CEL)
Enzymatic hydrolysis lignin of wheat straw was extracted according to previous literature (Chang et al., 1975). Briefly, the dewaxed wheat straw was ball-milled for 20 h in a plenary ball mill machine. 14.0 g of wheat straw powder, along with 525 mg of cellulase and 700 mg of viscozyme L, was added to a 175 ml of sodium acetate/acetic acid buffer (pH ≈ 5.0). The mixture was placed in a thermostatic incubator at 35°C for 72 h with 250 rpm vibration. After the reaction, the supernatant was removed by centrifugation and the solid residue was washed with buffer twice. After enzymatic hydrolysis for another excess 3 days, the solid sediment separated from being centrifuged and washed with deionized water three times to get the crude CEL.
γ-valerolactone lignin (GVL)
The preparation of GVL was based on the previous reference (Li et al., 2020). 3 g of wheat straw was placed in a 100 ml hydrothermal reactor with PTFE liner containing 36 ml GVL, 9 ml deionized water, and 24.6 μL 98% H2SO4 (the solid-to-liquid ratio was 3:40 and the acid concentration was 0.0113 M) and then soaked and stirred for 30 min. The reactor was heated at 170°C for 1 h. The reaction mixture was separated by filtration and the filtrate was collected, which was then added to 1,600 ml distilled ice water. The precipitated lignin was collected by centrifugation, then washed twice with deionized water, and freeze-dried.
Organosolv lignin (OL)
5 g of wheat straw was added to a 50 ml of acetic acid/formic acid/water (55:30:15, v/v/v) solution at a solid-to-liquid ratio of 1:10 (g/ml) reacted hermetically at 105°C for 3.5 h. Then, after being filtered and collected, the liquid was concentrated to about 3 ml by rotary evaporation and titrated back to 200 ml of deionized water. During the titration process, the sediment was continuously stirred, stood for 12 h for centrifugation, and freeze-dried to obtain a solid. Finally, the rough organic acid lignin was extracted and purified with a 1,4-dioxane/water (96/4, v/v) solution.
Lignin Purification
The above-extracted lignin samples were purified by the following method. 150 mg of the crude lignin was added to 15 ml 96% (v/v) 1,4-dioxane/water mixture and stirred at room temperature for 4 h, repeated three times. For the AL, the extraction liquid was evaporated to obtain pure lignin after centrifugation. As for the acid lignins, the 1,4-dioxane solutions were concentrated to ∼3 ml, transferred into a centrifuge tube filled with 40 ml cold deionized water by drops, then centrifuged to remove the supernatant, and lyophilized to get the purified lignins.
Analytical Methods
High-Performance Liquid Chromatography-Mass Spectrometric (HPLC-MS) Analysis
Tricin was quantified by thioacidolysis followed by HPLC-MS according to an established method (Lan et al., 2016). The specific operations of analytical thioacidolysis are as follows: 2.5 ml ethanethiol (EtSH), 0.625 ml boron trifluoride diethyl etherate, and enough freshly distilled dioxane were added to a 25 ml volumetric flask to prepare the thioacidolysis reagent. Then, 4 ml of the newly preparative thioacidolysis reagent and 20 mg of biomass material were put into a 10 ml reaction flask to react at 100°C for 4 h. After natural cooling to room temperature, the reaction mixture was transferred to a 60 ml separating funnel, adding 0.4 M NaHCO3 to pH ≈ 7.0 and 1 M HCl to pH ≈ 3.0. The degradation products were extracted with EtOAc, dehydrated with saturated NH4Cl and anhydrous MgSO4, and distilled under reduced pressure at 45°C, and the residue was dissolved in ethanol for HPLC-MS determination.
HPLC-MS analysis was performed based on the literature (Lan et al., 2019) using LC-electrospray ionization (ESI)/atmospheric pressure ionization- (API-) MS (LCMS-8050, Shimadzu). A Kinetex C18 (2.1 × 100 mm, 1.9 μm) column was used in the LC system and eluted with water (solvent A) and acetonitrile (solvent B), using a constant method (80% B from 0 to 12.5 min) with 1 μL of the injection volume and column temperature at 40°C and 0.5 ml min−1 of flow rate. And ionization was performed under dual ionization (ESI and API) using a dual ion source, with the nebulizing gas at 2.5 L min−1 and drying gas at 10 L min−1. The fragmented ions were measured by MS in positive mode and the quantitation of tricin was based on the peak area of the precursor ions in m/z 330.10.
A standard curve of tricin in HPLC-MS was established (Supplementary Figure S1). The content of wheat flavone in the original sample was calculated according to the following formula (2):
where TS (%) is the content of tricin in sample, CT (mg/ml) is the concentration of tricin, DM is diluted multiples, and SM is sample mass.
HSQC Characterization
50–100 mg of lignin sample was completely dissolved in 0.5 ml of deuterium dimethyl sulfoxide DMSO-d6 and then transferred to an NMR tube. A 500 MHz superconducting nuclear magnetic resonance spectroscopy (NMR, Avance Neo 500M, Bruker) was used to record the 2D HSQC spectrum. The spectra were processed using Bruker Topspin 4.1.3 (Windows version). The content of tricin was calculated by the integrals of the corresponding contours.
Determination of SPF
The sample to be tested was ground and sifted to pass 300 meshes for collection. It was accurately weighed at 20.0 mg mixing with 980.0 mg moisturizing cream and the mixture was stirred at 500 rpm for more than 24 h under dark conditions (Qian et al., 2015; Lin M. et al., 2021).
Then, 25 mg of the sunscreen sample prepared above, after being evenly spread on a glass sheet of 1 mm thickness with 3 M adhesive tape and dried in the dark for 20 min, was taken seven different points to test the transmittance of the sample at 290–400 nm to calculate the SPF value (Sohn et al., 2016). The calculation formula (3) was as follows (ISO 24443: 2012 and 24,444: 2010):
where Ser(λ) is the spectral weight of the action of erythema, Ss(λ) is the spectral irradiance of the ultraviolet source, and T(λ) is the transmittance of the sample.
Determination of Radical Scavenging Activity
DPPH (2,2′-diphenyl-1-picrylhydrazyl) radical scavenging activities of tricin and lignin samples were measured to evaluate the antioxidation activity according to the reported method (Jia et al., 2018). First, different concentration (0–0.025 mg/ml) of DPPH in methanol solution was prepared to obtain a standard curve between DPPH concentration and absorbance value by measuring their absorbance at λ = 517 nm. Then mixtures made by 0.1 ml methanol solution of different concentrations of each sample with 3.9 ml 0.025 mg/ml DPPH/methanol solution were evenly mixed and stored at room temperature for 60 min in dark to measure the absorbance of them at λ = 517 nm. The percentage of remaining DPPH radical was calculated as follows:
where the
Results and Discussion
Lignin Extraction Using Different Method
Wheat straw contains 15.9 mg tricin per g of dry matter biomass according to our determination, which is higher than many other plant species. Wheat, as one of the most common staple cereals, is widespread in Asia, especially in China. Accordingly, wheat straw lignin could be a potential source of natural tricin with high value for human health. In this study, we investigated the influence of fractionation methods on the tricin content of the lignin from wheat straw under different conditions, including those performed under acid, alkaline, and neutral conditions. For instance, we used alkaline extraction, mild acidolysis, cellulosic enzymatic hydrolysis, GVL pretreatment, and organosolv pretreatment to extract the wheat straw lignin and six different lignin samples were obtained (Table 1). As report previously, tricin was almost completely depleted under the alkaline condition at high temperature (Zikeli et al., 2016). Aiming to reserve the tricin component, we, therefore, performed the alkaline extraction under low temperature and obtained 13.1% crude AL on the base of wheat straw. The crude lignin was purified by dissolving in dioxane/H2O solution and gave 8.9% yield, accounting for 54.2% of the lignin in wheat straw. A controlled experiment using ball-milled wheat straw samples was also performed hoping to improve the lignin yield. The crude lignin yield increased to 21.2%. But the increment turned out to be from the carbohydrate fraction, which was eliminated during purification and leading to a similar purified lignin yield (55.1%). The mild acidolysis, organic acid, and cellulase enzymatic hydrolysis methods all produced about 50% lignin. But the GVL pretreatment yielded only 22.5% lignin, which might be because of the incomplete precipitation of lignin from the GVL solution.
HSQC Characterization of the Lignin Sample
The extracted lignin samples were characterized by HSQC NMR spectra to determine their structure (Figure 2 and Table 2). In general, all the methods applied in this study were able to extract the lignin from the wheat straw without serious alternation. The characteristic signals of guaiacyl, syringyl, and p-hydroxyphenyl units were clearly identified on the HSQC spectra, as well as the peaks of tricin and p-coumarate (Zheng et al., 2021). Unlike most of the AL in other studies (Lan et al., 2019; Zikeli et al., 2016), a certain amount of p-coumarate remained on the lignin after alkaline treatment in this study, which should be due to the mild condition (30°C) that we applied. The relative content of tricin was calculated by integrals of the corresponding peaks. The percentages of tricin in GVL-pretreated lignin, CEL, and MAL were 8.6, 6.7, and 6.3, respectively, higher than that in AL and organic acid lignin, which was about 1.1–1.7 (Table 3). A close inspection on the spectrum of the GVL lignin revealed that a certain amount of free tricin was presented in this sample, as indicated by the characteristic peak of C3/H3 in free tricin at δC/δH 103/7.0. The ratio of linked tricin to free tricin was about 6:4 according to the contour area of the corresponding signals. This result suggests that the dilute H2SO4 treatment can selectively cleave the β–O–4-tricin ether bonds in GVL at high temperature.
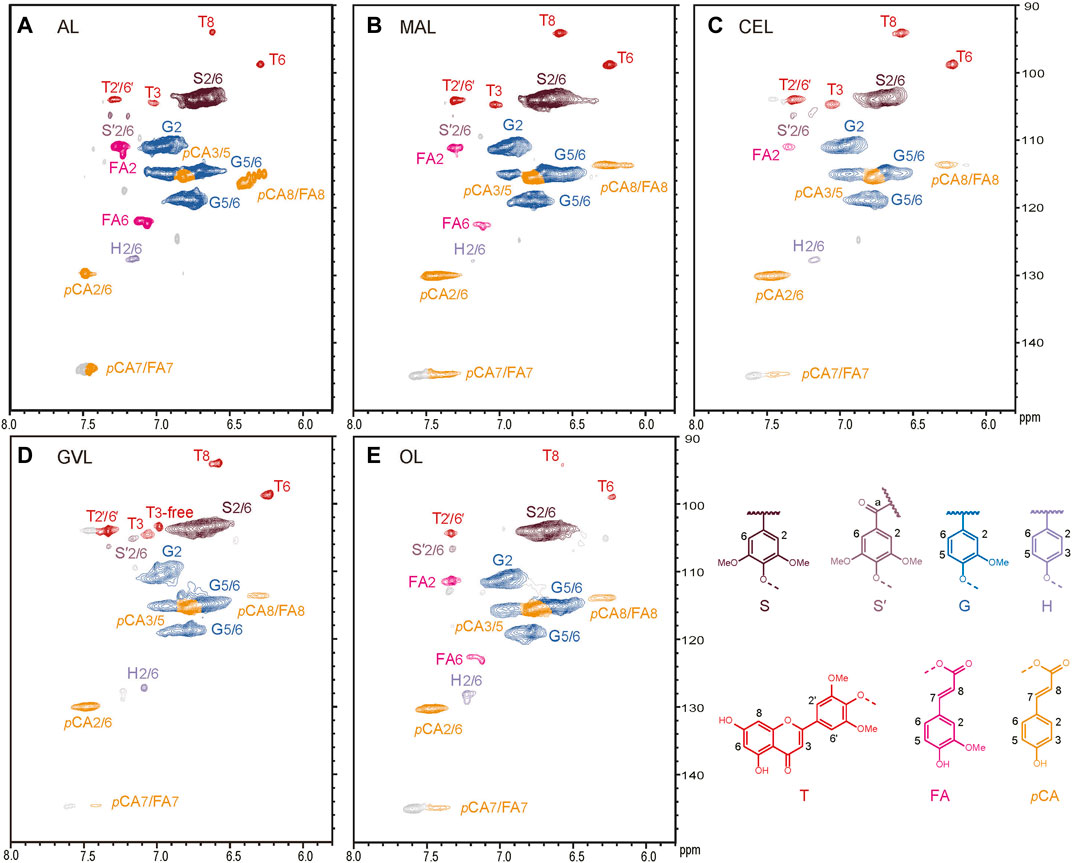
FIGURE 2. Aromatic region of the 2D HSQC spectra of (A) alkali lignin (AL), (B) mild acidolysis lignin (MAL), (C) cellulase enzymatic lignin (CEL), (D) γ-valerolactone lignin (GVL), and (E) organosolv lignin (OL).
Thioacidolysis for Tricin Quantitation
In order to determine the absolute content of tricin in the lignin samples, we applied the thioacidolysis degradation method followed by measurement using HPLC-MS under multiple reaction monitoring mode (MRM), as reported previously (Lan et al., 2016; Chen et al., 2021). The GVL lignin contained 89.3 mg/g of tricin. This was the highest tricin level among the six lignin samples, even higher than the tricin content in CEL (67.3 mg/g). The previous study also showed that the tricin level in the GVL lignin sample was higher than that in the CEL sample, according to the HSQC characterization. One of the possible reasons is that some of the tricin-(β–O–4)-lignin linkages were cleaved during GVL lignin extraction and the clipped-off tricin was isolated along with lignin, leading to a bit higher tricin level in the GVL lignin sample. The OL contained only 35.7 mg/g lignin, which might imply that tricin was degrade under such high concentration of acid solution at high temperature. The AL displayed the lowest tricin content with only 23.1 mg/g, although a mild condition was applied for the extraction. These results are generally in accordance with the semiquantitative result from HSQC. We further evaluated the stability of tricin under similar conditions using a pure tricin compound. Results showed that more than 98% of tricin remained after 9 h reaction at 30°C in 1.0 M NaOH solution, indicating that the tricin moiety was stable under this condition. The reason for the poor yield of tricin under alkaline conditions is still unclear. Further study using the dimeric tricin-monolignol model compound would be required to figure out the underlying mechanism.
UV Protection and Radical Scavenging Ability of the Lignin
Tricin is beneficial to human beings because of its various biological activities. The flavonoid backbone structure containing phenyl, carbonyl, and C=C double bonds endows strong UV absorption and radical scavenging ability to tricin. To explore the potential application of tricin-lignin, we further evaluated the SPF value of lignin-based sunscreens and radical scavenging ability of the extracted lignin samples, Table 4, to figure out how does the tricin level affect these properties.
The SPF of tricin-based sunscreen was 5.65, which was significantly higher than that of other dimeric model compounds (β–O–4, β–β, β–1, and β–5) and their derivatives formed by conventional monolignol (Lin M. et al., 2021). The SPF of AL was 4.30, similar to that of CEL, even though its tricin content was much lower than CEL. On the other hand, GVL lignin-based sunscreen displayed the highest SPF value, even slightly higher than that of pure tricin. The free radical scavenging activity showed a similar trend as the SPF value. The EC50 of the free radical towards tricin was 0.26 mg/ml (0.00079 mmol/ml). The AL and CEL presented a similar EC50 value (1.63 and 1.80 mg/ml), while the GVL lignin was the most active lignin towards radical with EC50 of 1.02 mg/ml. In our previous study, we revealed that the β–O–4 and β–β interlinkages were not favorable to UV absorption and radical scavenging. Moreover, the CEL showed lower SPF and radical scavenging ability compared with OL and kraft extracted from Pinus kesiya and Eucalyptus. This might explain why even the CEL contains a much higher tricin level but showed similar SPF and EC50 values with AL. The SPF value of tricin itself was remarkable, and the lignins containing tricin extracted from wheat straw displayed better SPF than those from softwood and hardwood without tricin. This suggests that retaining tricin is beneficial to the anti-UV property of the lignin samples. However, tricin does not impact the radical scavenging ability that much, as the kraft lignin of eucalyptus exhibited very low EC50 as well (Lin M. et al., 2021).
Conclusion
In this study, we applied alkaline pretreatment, organic solvent extraction, cellulase enzymatic hydrolysis, GVL pretreatment, and organic acid pretreatment to extract lignin from wheat straw. The tricin content varied significantly from the lignin samples extracted by different methods. Within these lignin preparations, although the GVL lignin contained a higher tricin level than other samples, the yield of tricin was not high due to the low lignin yield, and the CEL gave the highest tricin yield. The AL produced the lowest tricin content and yield, but the reason was still unclear. SPF testing of the lignin samples showed that tricin significantly improved the anti-UV radiation ability of the lignin. DPPH results demonstrated that tricin is a strong radical scavenger, but the lignin containing a higher tricin level was not necessary resulting in a lower EC50 value of DPPH radicals. Overall, our study provides fundamental understanding of the effect of the lignin extraction method on tricin content and the correlation between tricin content and anti-UV radiation/antioxidation ability, which is beneficial to the future study on the application of tricin.
Data Availability Statement
The original contributions presented in the study are included in the article/Supplementary Material, and further inquiries can be directed to the corresponding authors.
Author Contributions
MX performed the experiment and analysis and wrote the paper. WL and FY designed the project, supervised the experiments and analysis, and obtained funding supporting. ZC, YX, ML, and JL performed lignin separation and aided in the analysis. LZ aided in the analysis and obtained funding supporting. All authors were involved in the writing and revisions.
Funding
The authors are grateful to the financial support for this work by the National Natural Science Foundation of China (21908072; 22108088), Guangxi Key Laboratory of Clean Pulp and Papermaking and Pollution Control (KF201805-5), and State Key Laboratory of Pulp and Paper Engineering (202105).
Conflict of Interest
The authors declare that the research was conducted in the absence of any commercial or financial relationships that could be construed as a potential conflict of interest.
Publisher’s Note
All claims expressed in this article are solely those of the authors and do not necessarily represent those of their affiliated organizations or those of the publisher, the editors, and the reviewers. Any product that may be evaluated in this article or claim that may be made by its manufacturer is not guaranteed or endorsed by the publisher.
Acknowledgments
The authors also acknowledge the Climbing Plan of South China University of Technology (j2tw2021020007) and the National Undergraduate Innovation and Entrepreneurship Training Program (202010561100).
Supplementary Material
The Supplementary Material for this article can be found online at: https://www.frontiersin.org/articles/10.3389/fenrg.2021.756285/full#supplementary-material
References
Boerjan, W., Ralph, J., and Baucher, M. (2003). Ligninbiosynthesis. Annu. Rev. Plant Biol. 54, 519–546. doi:10.1146/annurev.arplant.54.031902.134938
Cao, G., Sofic, E., and Prior, R. L. (1997). Antioxidant and Prooxidant Behavior of Flavonoids: Structure-Activity Relationships. Free Radic. Biol. Med. 22, 749–760. doi:10.1016/s0891-5849(96)00351-6
Chang, H., Cowling, E. B., Brown, W., Adler, E., and Miksche, G. (1975). Comparative Studies on Cellulolytic Enzyme Lignin and Milled Wood Lignin of Sweetgum and spruce. Holzforschung 29, 153–159. doi:10.1515/hfsg.1975.29.5.153
Chen, F., Zhuo, C., Xiao, X., Pendergast, T. H., and Devos, K. M. (2021). A Rapid Thioacidolysis Method for Biomass Lignin Composition and Tricin Analysis. Biotechnol. Biofuels 14, 18. doi:10.1186/s13068-020-01865-y
del Río, J. C., Rencoret, J., Gutiérrez, A., Elder, T., Kim, H., and Ralph, J. (2020). Lignin Monomers from beyond the Canonical Monolignol Biosynthetic Pathway: Another brick in the wall. ACS Sustain. Chem. Eng. 8, 4997–5012. doi:10.1021/acssuschemeng.0c01109
del Río, J. C., Rencoret, J., Prinsen, P., Martínez, Á. T., Ralph, J., and Gutiérrez, A. (2012). Structural Characterization of Wheat Straw Lignin as Revealed by Analytical Pyrolysis, 2D-NMR, and Reductive Cleavage Methods. J. Agric. Food Chem. 60, 5922–5935. doi:10.1021/jf301002n
Heim, K. E., Tagliaferro, A. R., and Bobilya, D. J. (2002). Flavonoid Antioxidants: Chemistry, Metabolism and Structure-Activity Relationships. J. Nutr. Biochem. 13, 572–584. doi:10.1016/s0955-2863(02)00208-5
Jensen, A., Cabrera, Y., Hsieh, C.-W., Nielsen, J., Ralph, J., and Felby, C. (2017). 2D NMR Characterization of Wheat Straw Residual Lignin after Dilute Acid Pretreatment with Different Severities. Holzforschung 71, 461–469. doi:10.1515/hf-2016-0112
Jia, Y., He, Y., and Lu, F. (2018). The Structure-Antioxidant Activity Relationship of Dehydrodiferulates. Food Chem. 269, 480–485. doi:10.1016/j.foodchem.2018.07.038
Jiang, B., Zhang, Y., Gu, L., Wu, W., Zhao, H., and Jin, Y. (2018). Structural Elucidation and Antioxidant Activity of Lignin Isolated from Rice Straw and Alkali-oxygen Black Liquor. Int. J. Biol. Macromol. 116, 513–519. doi:10.1016/j.ijbiomac.2018.05.063
Kim, S., and Dale, B. E. (2004). Global Potential Bioethanol Production from Wasted Crops and Crop Residues. Biomass Bioenergy 26, 361–375. doi:10.1016/j.biombioe.2003.08.002
Lan, W., Lu, F., Regner, M., Zhu, Y., Rencoret, J., Ralph, S. A., et al. (2015). Tricin, a Flavonoid Monomer in Monocot Lignification. Plant Physiol. 167, 1284–1295. doi:10.1104/pp.114.253757
Lan, W., Rencoret, J., Lu, F., Karlen, S. D., Smith, B. G., Harris, P. J., et al. (2016). Tricin‐lignins: Occurrence and Quantitation of Tricin in Relation to Phylogeny. Plant J. 88, 1046–1057. doi:10.1111/tpj.13315
Lan, W., Rencoret, J., Del Rio, J. C., and Ralph, J. (2019). “Tricin in Grass Lignin: Biosynthesis, Characterization, and Quantitation,” in Chapter in Lignin, Biosynthesis, Functions and Economic Significance. New York: Nova science publishers.
Lauwaert, J., Stals, I., Lancefield, C. S., Deschaumes, W., Depuydt, D., Vanlerberghe, B., et al. (2019). Pilot Scale Recovery of Lignin from Black Liquor and Advanced Characterization of the Final Product. Separat. Purif. Techn. 221, 226–235. doi:10.1016/j.seppur.2019.03.081
Li, M., Pu, Y., Yoo, C. G., and Ragauskas, A. J. (2016). The Occurrence of Tricin and its Derivatives in Plants. Green. Chem. 18, 1439–1454. doi:10.1039/c5gc03062e
Li, J., Feng, P., Xiu, H., Li, J., Yang, X., Ma, F., et al. (2019). Morphological Changes of Lignin during Separation of Wheat Straw Components by the Hydrothermal-Ethanol Method. Bioresour. Techn. 294, 122157. doi:10.1016/j.biortech.2019.122157
Li, S., Zhao, C., Yue, F., and Lu, F. (2020). Revealing Structural Modifications of Lignin in Acidic γ-Valerolactone-H2O Pretreatment. Polymers 12, 116. doi:10.3390/polym12010116
Lin, M., Yang, L., Zhang, H., Xia, Y., He, Y., Lan, W., et al. (2021a). Revealing the Structure-Activity Relationship between Lignin and Anti-UV Radiation. Ind. Crops Prod.
Lin, W., Yang, J., Zheng, Y., Huang, C., and Yong, Q. (2021b). Understanding the Effects of Different Residual Lignin Fractions in Acid-Pretreated Bamboo Residues on its Enzymatic Digestibility. Biotechnol. Biofuels 14, 143. doi:10.1186/s13068-021-01994-y
Lu, F., Wang, C., Chen, M., Yue, F., and Ralph, J. (2021). A Facile Spectroscopic Method for Measuring Lignin Content in Lignocellulosic Biomass. Green. Chem. 23, 5106–5112. doi:10.1039/d1gc01507a
Luterbacher, J. S., Rand, J. M., Alonso, D. M., Han, J., Youngquist, J. T., Maravelias, C. T., et al. (2014). Nonenzymatic Sugar Production from Biomass Using Biomass-Derived -Valerolactone. Science 343, 277–280. doi:10.1126/science.1246748
Ogo, Y., Ozawa, K., Ishimaru, T., Murayama, T., and Takaiwa, F. (2013). Transgenic rice Seed Synthesizing Diverse Flavonoids at High Levels: a New Platform for Flavonoid Production with Associated Health Benefits. Plant Biotechnol. J. 11, 734–746. doi:10.1111/pbi.12064
Oyama, T., Yasui, Y., Sugie, S., Koketsu, M., Watanabe, K., and Tanaka, T. (2009). Dietary Tricin Suppresses Inflammation-Related colon Carcinogenesis in Male Crj: CD-1 Mice. Cancer Prev. Res. 2, 1031–1038. doi:10.1158/1940-6207.CAPR-09-0061
Qian, Y., Qiu, X., and Zhu, S. (2015). Lignin: a Nature-Inspired Sun Blocker for Broad-Spectrum Sunscreens. Green. Chem. 17, 320–324. doi:10.1039/c4gc01333f
Ralph, J. (2009). Hydroxycinnamates in Lignification. Phytochem. Rev. 9, 65–83. doi:10.1007/s11101-009-9141-9
Sarkar, N., Ghosh, S. K., Bannerjee, S., and Aikat, K. (2012). Bioethanol Production from Agricultural Wastes: An Overview. Renew. Energ. 37, 19–27. doi:10.1016/j.renene.2011.06.045
Sohn, M., Herzog, B., Osterwalder, U., and Imanidis, G. (2016). Calculation of the Sun protection Factor of Sunscreens with Different Vehicles Using Measured Film Thickness Distribution - Comparison with the SPF In Vitro. J. Photochem. Photobiol. B: Biol. 159, 74–81. doi:10.1016/j.jphotobiol.2016.02.038
Solyanik, G. I., Zulphigarov, O. S., Prokhorova, I. V., Pyaskovskaya, O. N., Kolesnik, D. L., and Atamanyuk, V. P. (2021). A Comparative Study on Pharmacokinetics of Tricin, a Flavone from Gramineous Plants with Antiviral Activity. Jbm 09, 76–91. doi:10.4236/jbm.2021.92008
Vanholme, R., De Meester, B., Ralph, J., and Boerjan, W. (2019). Lignin Biosynthesis and its Integration into Metabolism. Curr. Opin. Biotechnol. 56, 230–239. doi:10.1016/j.copbio.2019.02.018
Zheng, L., Yu, P., Zhang, Y., Wang, P., Yan, W., Guo, B., et al. (2021). Evaluating the Bio-Application of Biomacromolecule of Lignin-Carbohydrate Complexes (LCC) from Wheat Straw in Bone Metabolism via ROS Scavenging. Int. J. Biol. Macromol. 176, 13–25. doi:10.1016/j.ijbiomac.2021.01.103
Zhou, J.-M., and Ibrahim, R. K. (2009). Tricin-a Potential Multifunctional Nutraceutical. Phytochem. Rev. 9, 413–424. doi:10.1007/s11101-009-9161-5
Keywords: tricin, lignin, antioxidation, SPF, HSQC, HPLC-MS
Citation: Xie M, Chen Z, Xia Y, Lin M, Li J, Lan W, Zhang L and Yue F (2021) Influence of the Lignin Extraction Methods on the Content of Tricin in Grass Lignins. Front. Energy Res. 9:756285. doi: 10.3389/fenrg.2021.756285
Received: 10 August 2021; Accepted: 06 September 2021;
Published: 15 October 2021.
Edited by:
Xiaojun Shen, Dalian Institute of Chemical Physics (CAS), ChinaReviewed by:
Caoxing Huang, Nanjing Forestry University, ChinaChenhuan Lai, Nanjing Forestry University, China
Copyright © 2021 Xie, Chen, Xia, Lin, Li, Lan, Zhang and Yue. This is an open-access article distributed under the terms of the Creative Commons Attribution License (CC BY). The use, distribution or reproduction in other forums is permitted, provided the original author(s) and the copyright owner(s) are credited and that the original publication in this journal is cited, in accordance with accepted academic practice. No use, distribution or reproduction is permitted which does not comply with these terms.
*Correspondence: Wu Lan, bGFud3VAc2N1dC5lZHUuY24=; Fengxia Yue, eXVlZnhAc2N1dC5lZHUuY24=