- 1Department of Microbiology, University of North Bengal, Siliguri, India
- 2Department of Biotechnology, University of North Bengal, Siliguri, India
Thermoactive xylanases have important applications in the industrial deconstruction of lignocellulosic plant biomass, due to their sustained activity even at high temperature conditions of industrial bioreactors. We herein report the development of a thermoactive xylanolytic microbial consortium from the semi-digested contents of goat rumen and characterization of the xylanolytic enzyme cocktail produced by it. The consortium exhibited maximum endoxylanase activity at pH6 and at 60°C. Zymogram analysis revealed the production of multiple xylanases. The xylanase cocktail was stable over a pH range of 5–9 after pre-incubation for 3 h. It retained 74% activity after pre-incubation (60°C) for 50 min. It’s activity was enhanced in presence of β-mercaptoethanol, NH4+, Mg2⁺ and Ca2⁺, whereas Hg2⁺ had an inhibitory effect. The xylanolytic cocktail was further utilized for the saccharification of alkali pre-treated rice straw and mushroom spent rice straw. Saccharification was studied quantitatively using the dinitrosalicylic acid method and qualitatively using scanning electron microscopy. Results indicated the potential of the xylanolytic cocktail for the saccharification of rice straw and highlighted the significance of chemical and/or biological pre-treatment in improving the accessibility of the substrate towards the xylanase cocktail.
Introduction
With a rampant increase in agro-industrial activities, large quantities of agro residues are being generated worldwide. Such biomass being inexpensive and rich in polysaccharides (cellulose and hemicelluloses) can be subjected to microbial deconstruction (Singh et al., 2019) and the fermentable monosaccharides released can act as building blocks for value added products, such as chemicals, biofuels, etc (Aggarwal et al., 2017).
Hemicelluloses are a group of heteropolysaccharides consisting of xylan along with xyloglucans and mannans. Its depolymerization involves a xylanolytic enzyme system which is dissimilar to conventional cellulose degrading enzyme systems (Smith et al., 2017). Complete hydrolysis of xylan involves the combined action of enzymes with different substrate specificities, such as endo-β-1,4-xylanases (EC 3.2.1.8), β-D-xylosidases (EC 3.2.1.37), α-L-arabinofuranosidases (EC 3.2.1.55), α-glucuronidases (EC 3.2.1.139), acetyl xylan esterases (EC 3.1.1.72) (Thapa et al., 2020). Most fungi and bacteria are inherently efficient producers of xylanases (Thomas et al., 2013). Bacterial consortia with thermostable enzyme systems would be desirable for large scale industrial saccharification of prolonged duration due to lower possibilities of microbial contamination, reduced medium viscosity, increased solubility and surface area of substrate (Cao et al., 2014; Synowiecki., 2010; Gomes et al., 2016). Therefore, development of a thermophilic consortium can be of significance in the microbial production of thermostable xylanases for improved industrial hydrolysis of complex agroresidues.
Rice straw (RS), being one of the most abundant, renewable lignocellulosic waste materials in the world, is an attractive feedstock for bioethanol production (Takano and Hoshino, 2018). Due to lack of utilization, the bulk of it is disposed of by burning, releasing massive quantities of methane and particulate carbon. This not only negatively affects the tropospheric ozone layer with increase in global warming and pollution, but also leads to a significant deterioration of annual harvest and public health standards (Wood et al., 2016). RS is comprised of cellulose (∼35%), lignin (∼15%) and hemicellulose (∼18%) (Gou et al., 2018) and has potential to be used as feedstock for lignocellulolytic exoenzyme production as well as for saccharification to produce simple sugars (Djohan et al., 2016; Kogo et al., 2017). The spontaneous degradation of lignocellulosic compounds is extremely slow but there are microorganisms capable of degrading them (Chen et al., 2019). Microorganisms thriving in environments where natural lignocellulose degradation occurs, produce enzymes such as laccases, cellulases and xylanases to hydrolyze the different components of agroresidues (Ravindran et al., 2018). Hence, an environmental sample-based consortium would produce a plethora of microbial hydrolases as compared to single bacterial isolates and would be useful in agroresidue degradation.
RS is used as a substrate in small scale industries for the cultivation of oyster mushroom (Pleurotus sp). This fungal specimen is reported to produce peroxidase and laccase for lignin degradation and therefore spent rice straw (SRS) obtained after such biological pre-treatment has been considered suitable for downstream applications (Diaz-Godinez et al., 2017). Hence, the combined use of RS, initially for mushroom cultivation and then for microorganism-catalyzed depolymerization to fermentable sugars, could be an interesting research area and could be helpful in exploiting the full potential of RS.
With the aim to develop an industrially applicable enzyme cocktail, this study focuses on the development of a thermotolerant xylanolytic consortium from goat rumen contents, characterization of it’s xylanolytic enzymes and it’s utilization in the saccharification of alkali pre-treated RS and SRS at high temperatures.
Materials and Method
Materials
RS was collected from the Centre for Floriculture and Agri-business Management (COFAM), University of North Bengal, Siliguri, cut and ground into small pieces (approx.1 mm thick and 7 mm long) before storing in glass bottles at 4°C. SRS left after the cultivation of Pleurotus sp (Oyster mushroom) was obtained from the Department of Microbiology, University of North Bengal. SRS was processed by removing lumps of mycelium and then autoclaving at 15 psi for 15 min. The processed RS and SRS samples were stored in polyethylene bags for further use (Ryden et al., 2017).
The substrates for various enzymatic assays were purchased from Sigma-Aldrich, India. All other chemicals and media components were from Hi Media Laboratories and Sigma-Aldrich, India.
Development of Lignocellulose Degrading Thermophilic Consortium From Goat Rumen Contents
A microbial consortium was developed on M9 minimal medium which contained (gl−1): Na2HPO4, 6; KH2PO4, 3; NaCl, 5; NH4Cl, 1; MgSO4, 0.5 and CaCl2, 0.015 (Lepcha and Ghosh, 2018) and 3% rice straw as the sole carbon source. 50 ml of medium was inoculated with 4% (v/v) of semi-digested goat rumen contents and incubated under shaking (100 rpm), initially at 45°C. Consortium was sub-cultured (4% v/v) weekly into fresh M9 rice straw medium (45°C) for 2 weeks and then at 55°C for 2 weeks. Hereafter, the sub-cultures were incubated at 60°C and the cell-free supernatant (CFS) from the ninth pass onwards was used for further study. The thermophilic consortium (bred on RS) thus produced, henceforth has been referred to as Rice Straw-based Consortium (RSC). The original culture was continuously sub-cultured and maintained at 60°C.
Production of Xylanases by RSC
Extraction of Enzymes
The RSC culture was centrifuged at 12,000 xg for 15 min and the CFS obtained after passing through 0.2 µm filter was assayed for the presence of xylanases.
Agarose Well Diffusion Assay
A primary assessment of the consortium in terms of xylan hydrolysis capacity was performed via agarose well diffusion assay for seven consecutive days of growth. A mixture of 1% agarose and 0.5% birch wood xylan was layered on glass surface and wells of 0.7 cm diameter were bored in it. After introducing 50 µl of CFS into each well, the plate was incubated for 30 min at 60°C. Following this, the plate was stained with Congo Red solution (0.1% v/v) for 15 min and destained with 1M NaCl. Plates were visualized for zones of clearance and hydrolysis capacity was calculated (Gavande et al., 2021).
Enzyme Assays and Protein Estimation
The endoxylanase activity of the CFS was determined at 24 h intervals at the standard mesophilic (37°C) and elevated temperatures (60°C) and at different pH (Bradford, 1976; Cao et al., 2014; Chakdar et al., 2016; Chen et al., 2019) for seven consecutive days of growth. The following buffers (100 mM) were used: citrate buffer (pH5 and pH6), phosphate buffer (pH7) and Tris Cl (pH8). The activities of accessory xylanolytic enzymes such as β-xylosidase, α-L-arabinofuranosidase and acetyl xylan esterase were determined on the day, temperature and pH which corresponded to the highest endoxylanase activity.
Endoxylanase assay was based on the reaction of enzymatically released reducing sugars with dinitrosalicylic acid (DNSA) reagent (Ghose, 1987). The assay mixture in a total volume of 1 ml contained 1% (w/v) birch wood xylan, 80 mM buffer and 100 μl CFS and the reaction was incubated at specified temperatures. After 30 min, the reaction was stopped by the addition of an equal volume of DNSA reagent followed by boiling in a water bath for 10 min. The released reducing sugars were determined by measuring the absorbance at 540 nm using xylose standard curve as reference. One unit of enzyme activity (EU) refers to the amount of enzyme that released 1 μmol of xylose min−1 under the standard assay conditions.
The activities of β-xylosidase, acetyl xylan esterase and α-L-arabinofuranosidase were assayed by using the respective substrates p-nitrophenyl-β-D-xylopyranoside, p-nitrophenyl-acetate and p-nitrophenyl-α-L-arabinofuranoside. The reaction mixture contained 2.5 mM of substrate, 80 mM buffer and 100 μl CFS. After incubation at specified temperatures for 30 min, the reaction was terminated by adding equal volume of 0.4M NaOH-glycine buffer (pH10.8) and the amount of released p-nitrophenol (pNP) was measured at 410 nm (Parry et al., 2001) using pNP as the calibration standard. One unit of enzyme activity (EU) refers to the amount of enzyme that released 1 μmol of product (pNP) min−1 under the standard assay conditions.
Protein concentration was determined by the dye binding method of Bradford using bovine serum albumin as standard (Bradford, 1976). The specific activities (EU/mg) of all four enzymes: endoxylanase, α-L-arabinofuranosidase, acetyl xylan esterase and β-xylosidase, were used to estimate their relative abundance in the CFS according to the equation:
Zymogram Analysis
The RSC culture was centrifuged after 3 days of growth and the protein content of CFS was precipitated by adding double volume of acetone, keeping overnight at 4°C and then centrifuging at 12000 xg for 15 min. The obtained pellet was suspended in a minimal volume of 100 mM citrate buffer, pH6 and the xylanase enzyme preparation (XEP) so obtained was used for zymogram analysis (Romano et al., 2013). For this, the protein sample (∼25 μg) was resolved in a polyacrylamide gel incorporated with 0.1% (w/v) birch wood xylan. The zymogram gel was incubated overnight in a renaturation reagent, 1% (v/v) Triton X 100 at 4°C, followed by thorough washing with deionised water. The gels were then incubated in 100 mM phosphate buffer (pH6) at 60°C for 30 min. The zymogram for xylanase was stained with 0.1% (w/v) Congo Red solution for 30 min followed by destaining with 1M NaCl.
Characterization of Endoxylanase
The RSC culture was centrifuged after 3 days of growth and XEP was prepared for characterization of endoxylanases.
pH Optima and Stability
The optimum pH of endoxylanase was determined by measuring enzymatic activity in a wide range of pH (Anasontzis et al., 2017; Bhardwaj et al., 2019; Bradford, 1976; Cao et al., 2014; Chakdar et al., 2016; Chen et al., 2019; Díaz-Godínez et al., 2017; Djohan et al., 2016) at 60°C. For determination of pH stability, pre-incubation (3 h) was performed in respective buffers at 4°C. The pre-incubation temperature of 4°C was chosen to prevent the temperature dependent loss of enzymatic activity. This was followed by determination of enzymatic activity at 60°C under standard assay conditions.
Temperature Optima and Stability
The optimum temperature of endoxylanase was determined by measuring enzymatic activity in the temperature range 4–100°C at pH6 under standard assay conditions. For determination of thermal stability, pre-incubation was performed in citrate buffer (pH6) for different time periods (0–50 min) (Wang et al., 2019) at 40, 60 and 80°C and then endoxylanase activity was determined at 60°C under standard assay conditions.
Effect of Additives on Xylanase Activity
The effect of additives was determined by monitoring activity in presence of metal ions, sodium dodecyl sulphate (SDS), β-mercaptoethanol (β-ME) and ethylene diamine tetraacetic acid (EDTA) at a concentration of 5 mM each (Shaikh et al., 2018). The reaction mixture with each of the additives was incubated at 60°C under standard assay conditions. Reaction mixtures without additives served as control.
Effect of Monosaccharides and Disaccharides on Xylanase Activity
Susceptibility to feedback inhibition was tested by carrying out endoxylanase assay in presence of xylose, glucose (Madarwati et al., 2014) or cellobiose at concentrations ranging from 50 to 200 mM at 60°C and pH6 under standard assay conditions as described above.
Pretreatment and Saccharification
Alkaline Pre-treatment of RS and SRS
RS and SRS were alkali pre-treated by soaking in 12% (w/v) sodium hydroxide (NaOH) solution for 1 h at 55°C in a solid to liquid ratio of 1:20 (w/v) and then they were segregated from the pre-treatment liquid or “black liquor” using filter paper (Harun and Goek, 2016). The alkali pre-treated RS (NaOH RS) and alkali pre-treated SRS (NaOH SRS) were thoroughly washed with distilled water till neutral pH and were pressed dry with filter papers instead of air-drying to preserve porosity according to the protocol of NREL (Resch et al., 2015) and stored in glass bottles.
High Temperature-small Scale Saccharification
XEP from RSC was implicated in HTSS of NaOH RS and NaOH SRS and those without alkali pre-treatment (RS and SRS). The saccharification mix in a final volume of 20 ml, contained 0.6 g (3% w/v) of substrate, 18 ml of 100 mM citrate buffer (pH6) and 2 ml of XEP. The reaction mix was incubated at 60°C and a separate reaction was set for each straw sample. Saccharification mixes were centrifuged at specified time intervals at 12000 xg for 15 min. The supernatant obtained, was used to estimate the total yield (TY) of reducing sugars (Ghose, 1987) and residual xylanase activity in the saccharification mix. The reaction mixture without XEP containing only the substrate in 20 ml buffer served as control in order to estimate the non-enzymatic yield (NEY) of reducing sugars from the substrates at 60°C.
Scanning Electron Microscopy (SEM) and Energy Dispersive X-Ray Spectroscopy (EDS)
After saccharification experiment, all the rice straw samples were dried in a dessicator and mounted on stubs using a carbon adhesive. Then they were sputter coated with gold (Au) nanoparticles in an ion-sputter coater before viewing under SEM (JOEL JSM-IT 100) at 10 mm and 5 kV acceleration voltage and ×900 magnification. SEM study was accompanied by the analysis of silica content by EDS (Oxford INCA350) (Phitsuwan et al., 2017).
Results
Production of Xylanases by RSC
Agarose Well Diffusion Assay
The hydrolysis of polysaccharides in an agarose-based medium is usually indicated by the formation of clearance zones due to the lower binding affinity of Congo Red dye with depolymerised polysaccharides. The xylanolytic potential of RSC was revealed by the formation of clearance zones on agarose gel supplemented with 1% birch wood xylan, when stained with 0.1% Congo Red solution. The hydrolytic index increased during the incubation period with the highest value (2.50) on Day3 which gradually decreased thereafter (Figure 1A).
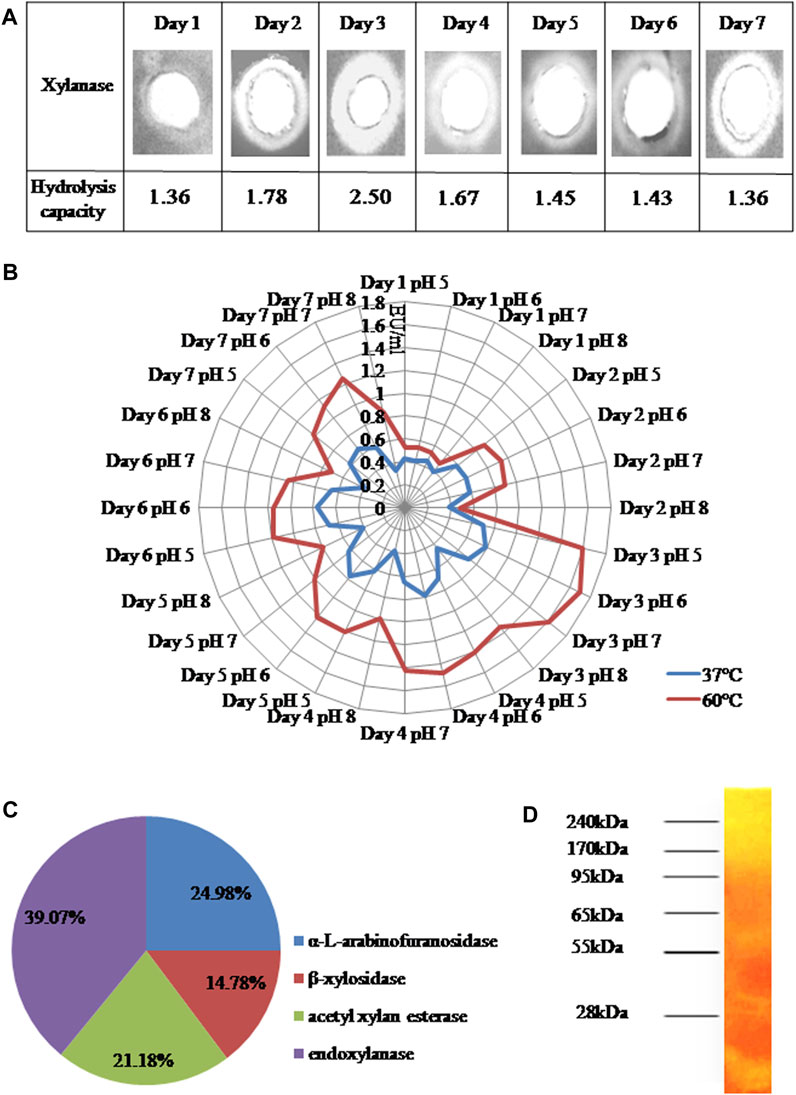
FIGURE 1. (A) Detection of xylan degradation as determined on various days of growth of RSC by agarose well diffusion assay (B) Endoxylanase activities (EU/ml) at different temperatures (37 and 60°C) and pH (C) Relative abundance of xylanolytic enzymes (endoxylanases and accessory enzymes) in CFS of RSC after 3 days of growth at 60°C (D) Zymogram of extracellular xylanases present in XEP after 3 days of growth of RSC.
Quantitative Estimation of Xylanase Production
The endoxylanase activity of RSC was estimated quantitatively at different pH (Bradford, 1976; Cao et al., 2014; Chakdar et al., 2016; Chen et al., 2019) and temperatures (37°C, 60°C) from Day 1 to Day 7 of incubation. Endoxylanase activity was detected at all the tested pH and was maximum at 60°C and pH6 on Day 3 (1.7 EU/ml). After Day 3, there was a gradual decline in activity up to Day 7 (1.13 EU/ml) (Figure 1B). The production of cellulase was insignificant as compared to that of xylanase and hence was not considered for further analysis.
Production of Accessory Xylanolytic Enzymes by RSC
Since maximum endoxylanase activity was observed on Day 3, it’s relative abundance in the CFS on the same day was compared with that of accessory xylanolytic enzymes, in terms of the specific activity. Endoxylanase (4.52 EU/mg) was the most abundant xylanolytic enzyme and constituted 39.07% of the CFS, followed by 24.98% of α-L-arabinofuranosidase (2.89 EU/mg), 21.18% of acetyl xylan esterase (2.45 EU/mg) and 14.78% of β-xylosidase (1.71 EU/mg) (Figure 1C).
Zymogram Analysis
The production of multiple xylanases by RSC was ascertained by zymogram analysis. The concentrated CFS formed four smears along the gel length due to xylan hydrolysis. One broad smear was obtained in the molecular weight range of (95–240) kDa and other smears were obtained in the range of 55, 28 and less than 28 kDa (Figure 1D).
Characterisation of Endoxylanase
Temperature and pH Optima and Stability
The cocktail was active over a wide range of pH (Bradford, 1976; Cao et al., 2014; Chakdar et al., 2016; Djohan et al., 2016; Anasontzis et al., 2017; Díaz-Godínez et al., 2017; Bhardwaj et al., 2019; Chen et al., 2019) with the optima being at pH6. It gave 53.41% of it’s activity at pH4 and 41.32 and 38.01% of it’s activity at pH9 and 10 respectively (Figure 2A). The results of pH stability showed that the mixture was most stable at pH7. There was no significant loss of activity in the pH range (Bradford, 1976; Cao et al., 2014; Chakdar et al., 2016; Díaz-Godínez et al., 2017; Chen et al., 2019). 86.4, 91.6 and 85% activities were retained at pH5, 6 and 9 respectively whereas only 56.9 and 46.4% activities were retained upon pre-incubation at pH3 and 10 respectively (Figure 2B).
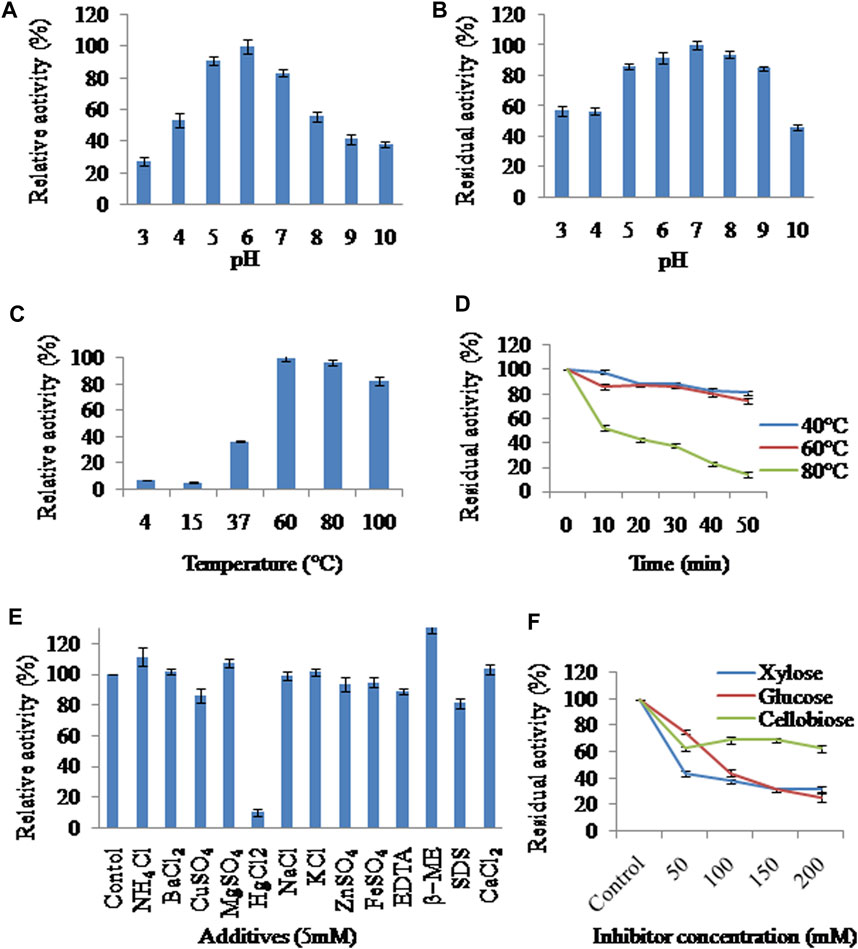
FIGURE 2. Effect of (A) pH on activity (B) pH on stability (C) temperature on activity (D) temperature on stability (E) additives on activity (F) mono- and disaccharides on activity of xylanases present in XEP after 3 days of growth of RSC.
Optimum activity was obtained at 60°C, while 96 and 82% activity were exhibited at 80°C and 100°C respectively (Figure 2C), demonstrating saccharification capacity at higher temperatures and under harsh pre-treatment conditions. The cocktail was moderately thermostable as it retained 80.86 and 74.20% of activity after pre-incubation for 50 min at 40°C and 60°C respectively, whereas 13.9% activity was retained after pre-incubation for 50 min at 80°C (Figure 2D).
Effect of Metals, Additives and Sugars on Activity
Metal ions interact with the -SH or -COOH functional groups present in enzymes and may either stimulate or inhibit its performance (Kumar et al., 2017). The xylanase activity was increased upto 130.2% by β-ME, upto 111.08% by NH4⁺, upto 107.3% by Mg2⁺ and upto 103.35% by Ca2⁺ whereas it was diminished to 10% by Hg2+, to 93.63% by Zn2⁺, to 94.81% by Fe2⁺ and to 86% by Cu2⁺. The presence of EDTA and SDS in the reaction mixture reduced xylanase activity to 88.67 and 81.2% respectively (Figure 2E). The influence of various concentrations of xylose, glucose and cellobiose on xylanase activity was also investigated. Xylose at 200 mM diminished the enzymatic activity to 31.25% suggesting feedback inhibition. Both glucose and cellobiose had an inhibitory effect. Glucose (200 mM) diminished the enzymatic activity to 25%, whereas cellobiose had a relatively lower inhibitory effect with 68.75 and 62.50% activities remaining at 150 and 200 mM concentrations, respectively (Figure 2F).
Implication of XEP in Saccharification
Estimation of Release of Reducing Sugars
A progressive increase in TY was noted from Day 1 to Day 5 in case of all samples, with maximum TY of reducing sugars on Day 5 for NaOH RS (196 mg/g dry matter (DM)) (Figure 3A). This was 1.36 fold greater than the TY of NaOH SRS on Day 5 (144 mg/g DM). Both NaOH RS and NaOH SRS had higher TY values than the samples without NaOH treatment (RS and SRS). On Day5, the TY of NaOH RS was 4.08 fold higher than the TY of RS (48 mg/g DM), whereas the TY of NaOH SRS was 1.47 times higher than the TY of SRS (98 mg/g DM).
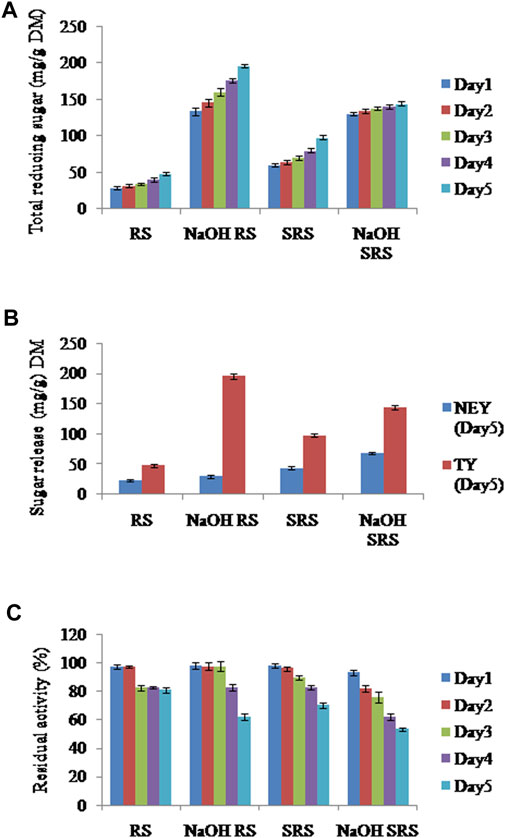
FIGURE 3. (A) Release of reducing sugars from different straw samples during 5 days of HTSS (B) Total yield (TY) and non-enzymatic yield (NEY) of sugars from untreated and alkali pre-treated straw samples after 5 days of HTSS (C) Residual activity of xylanases in the different saccharification mixes during 5 days of HTSS.
The NEY in NaOH SRS on Day 5 (68 mg/g DM) was 1.55 fold higher as compared to the NEY of SRS (44 mg/g DM) and the NEY in NaOH RS on Day 5 (30 mg/g DM) was 1.25 fold higher as compared to the NEY of RS (24 mg/g DM) (Figure 3B) indicating the role of pre-treatment strategies in affecting the structural integrity of rice straw and hence facilitating higher non-enzymatic release of sugars during saccharification.
Estimation of Residual Xylanase Activity in Saccharification Mix
Residual activity was estimated to ascertain enzymatic thermostability in the saccharification mix over 5 days (Figure 3C). XEP of RSC was thermoactive as it retained activity till the end of the saccharification experiment. The XEP applied to RS and NaOH-RS retained 80.7 and 62% of initial activity, whereas 70 and 53.33% of initial activity were retained in case of SRS and NaOH SRS, respectively.
SEM and EDS Analysis
SEM analysis revealed prominent cracks on the surface of SRS as compared to the relatively intact surface of RS. The structure of RS and SRS after alkali pre-treatment and saccharification showed distinct disorganisation of structure, which was higher for NaOH SRS as compared to other substrates (Figures 4A–D). Furthermore, EDS analysis revealed higher silica levels in RS (19.06 weight%) and SRS (17.61 weight%) which was considerably reduced in NaOH RS (6.91 weight%) and NaOH SRS (2.14 weight%), respectively (Figures 4E–H).
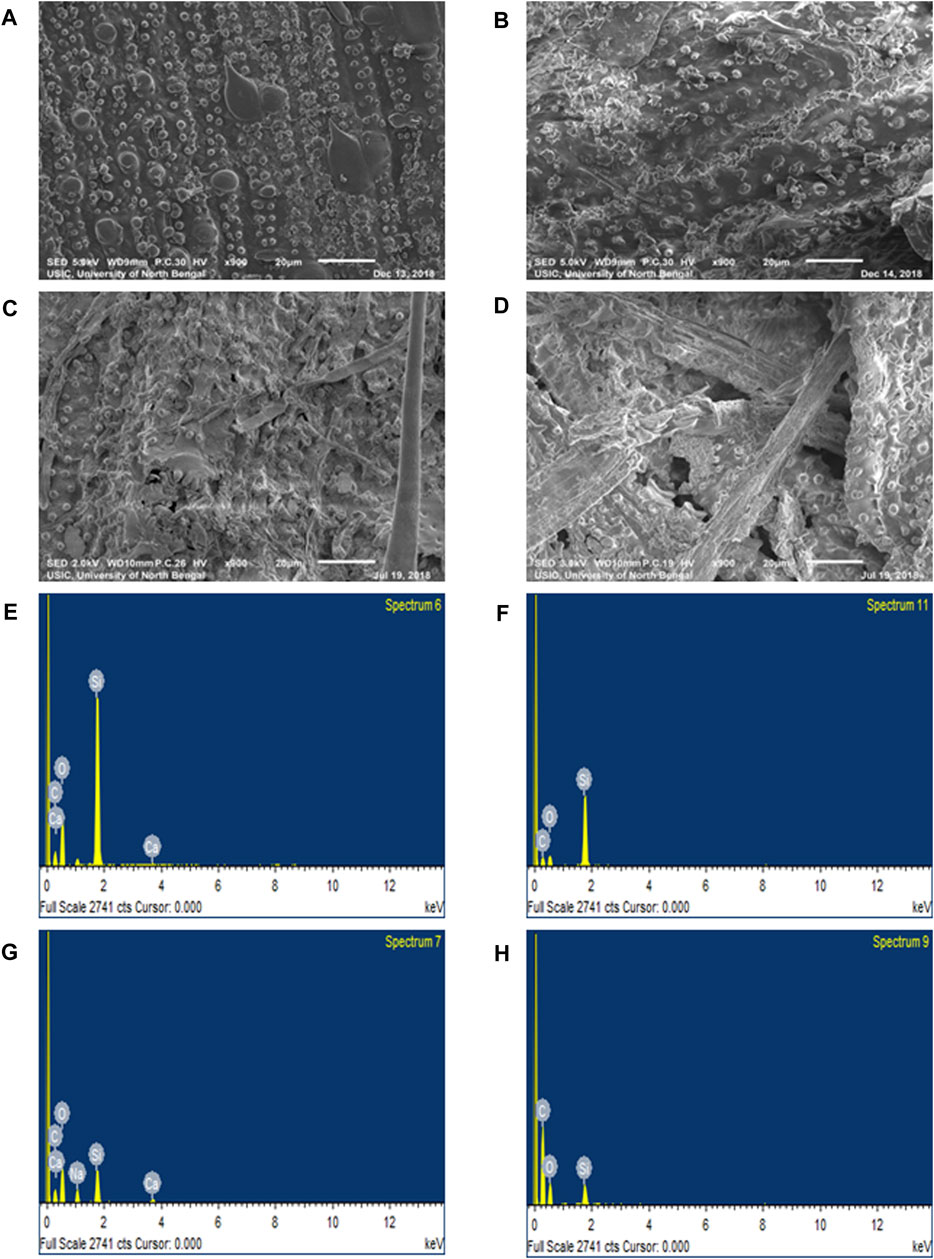
FIGURE 4. SEM analyses (A) RS (B) SRS (C) NaOH RS (D) NaOH SRS and EDS analysis of silica percentage (E) RS (F) SRS (G) NaOH RS (H) NaOH SRS of different samples after 5 days of HTSS.
Discussion
Lignocellulolytic environments happen to be a potential source of cellulolytic and xylanolytic enzymes for the efficient depolymerization of agro-residues to reducing sugars and can be used for industrial applications. Ruminant diet contains crude fibers such as cellulose, xylan and starch as basal feed. These materials are fermented in rumen by microbial communities including bacteria, fungi and protozoa. Among these, bacterial population is the most abundant in the rumen ecosystem comprising 1010 to 1011 cells/ml (Kamra, 2005). Biomass degradation by thermotolerant microorganisms is beneficial due to their production of thermostable enzymes with higher specific activity and stability, thus allowing flexibility for process configuration. The ruminal (core) temperature in live goat is around 39.8°C (Szabuniewicz et al., 1972). Even though mesophiles are expected to predominate under normal conditions, there are reports on the metagenomic analysis of rumen microbial communities revealing the presence of numerous thermotolerant species belonging to bacterial phyla such as Bacteroidetes, Firmicutes, Proteobacteria, Euryarchaeota, Actinobacteria, Spirochaetes, Fusobacteria, Chloroflexi and Chlorobi (Suryawanshi et al., 2019). The microbiota of goat rumen can therefore serve as a unique source of thermostable cellulolytic and hemicellulolytic enzymes.
In the present study, a thermotolerant bacterial consortium, RSC, was developed from goat rumen contents using RS as the sole carbon source. The culturable bacterial isolates obtained from the consortium were mostly Gram positive. The molecular characterisation of the isolates by 16SrRNA analysis, revealed that most of them belonged to genus Bacillus, which is not surprising because Bacillus sp has long been associated with thermotolerance and xylanolytic property (Chakdar et al., 2016). Some other bacterial genera such as Staphylococcus and Lysinibacillus, were also present (Supplementary Material Figure S1).
The CFS derived from the heat-adapted RSC exhibited the ability to degrade xylan in the agarose well diffusion assay. RSC produced thermoactive xylanolytic enzymes among which, endoxylanase exhibited the maximum activity at different pH (Bradford, 1976; Cao et al., 2014; Chakdar et al., 2016; Chen et al., 2019). It was also found to be produced in proportionately higher quantity as compared with the accessory xylanolytic enzymes and therefore studied in detail. The presence of endoxylanase activity in a broad range of pH along with the multiple band formation during zymogram analysis, was owed to the production of multiple xylanolytic enzymes by the RSC, with diverse physicochemical properties (Chakdar et al., 2016), and to the presence of isoenzymes, whose production has previously been reported in notable thermophilic genera such as Cellulomonas and Nesterenkonia (Walia et al., 2017).
The degradation of the heterogeneous structure of xylan requires the action of a complex enzyme system, composed of β-1,4-endoxylanase, β-xylosidase, α-L-arabinofuranosidase and acetyl xylan esterase (Bhardwaj et al., 2019). The cooperative effect of the xylan-degrading enzymes enhances the susceptibility of the heteropolymer to degradation. The removal of acetyl group from acetyl xylan by acetyl xylan esterase increases the accessibility of the xylan backbone for endoxylanase attack. Conversely, endoxylanase creates shorter acetylated polymers, which are the preferred substrates for esterase activity. β-xylosidase hydrolyzes the released xylooligosaccharides hence preventing end-product inhibition of endoxylanase, while α-L-arabinofuranosidase enhances the saccharification of arabinoxylans (Malgas et al., 2019). Among the various xylanolytic enzymes produced by RSC, endoxylanase exhibited maximum activity. Previous studies on the production of cellulolytic enzymes by bacterial consortia developed from compost (Zhu et al., 2016) and vermicompost (Gavande et al., 2021) also showed relatively higher proportion of endoxylanase activity.
Endoxylanase activity in the consortium was moderately thermostable and it showed optimum activity at 60°C with marked activity even at 80 and 100°C. The RSC endoxylanase activity was weakly inhibited by EDTA indicating non-obligate role of metal ions in catalysis and by SDS indicating the importance of hydrophobic interactions in maintaining enzyme structure (Seemakram et al., 2020). Strong inhibition by Hg2+ suggested the presence of free sulphydryl (-SH) groups in the active sites. Greater inhibitory effect of monosaccharides (as compared to cellobiose) on xylanase activity was in accordance with the findings of (Madarwati et al., 2014) who stated that the presence of glucose and xylose in hydrolysis reaction sets can decrease the yield of simple sugars. The research work of Ajijolakewu et al. (2016) also highlighted a greater inhibitory effect of monosaccharides as compared to disaccharides (cellobiose) on xylanase activity which implied that XEP was prone to feedback inhibition and continuous removal of end products might be required for more efficient saccharification.
The implication of the RSC enzyme cocktail in industrial saccharification was analysed by performing HTSS on untreated, alkali pre-treated (NaOH-RS and NaOH-SRS), and combined biologically and alkali pre-treated straw samples (NaOH RS and NaOH SRS). A significantly higher yield of RRS from NaOH RS and NaOH SRS in comparison to the untreated samples showed the effectiveness of pre-treatments in removal of lignin and increased accessibility of xylanases to hemicelluloses (Ikramullah et al., 2018). Phitsuwan et al. (2016) reported an 84% removal of lignin from napier grass by using NaOH, thereby making substrates more available to the enzymes. The XEP retained considerable activity during the 5 days of saccharification at 60°C, which was due to the production of multiple thermostable xylanases by the RSC as was seen during zymogram analysis. This remarkable retention of activity was accredited to the fact that XEP contains a cocktail of several xylanases and although some forms might have been inactivated, majority of the isoforms retained activity.
SEM analysis revealed cracks and a distinct structural disintegration, with a higher level of deconstruction in NaOH-SRS after saccharification. This corresponds to hyphal penetration by Pleurotus sp (Anasontzis et al., 2017) leading to higher NEY of sugars from SRS. EDS analysis showed higher levels of silica in RS and SRS, which were reduced upon alkali pre-treatment. The surface of RS contains silica globules, which remain complexed with lignin polymers (Do et al., 2020). Lignin is a significant barrier in the deconstruction of lignocellulose as it reduces the enzymatic accessibility towards the cellulosic and hemicellulosic components. The reduction of silica percentage can be linked to delignification. Therefore the results of SEM analysis along with EDS showed the highest level of deconstruction in case of NaOH SRS followed by NaOH RS, SRS and RS, whereas sugar yield results indicated the highest total yield in case of NaOH RS followed by NaOH SRS, SRS and RS. A relatively lower yield of sugar in case of NaOH-SRS could be due to the partial hemicellulosic loss due to the combined pre-treatment. The results thus indicated that the combination of Pleurotus and NaOH pre-treatments may not be the best pre-treatment strategy for saccharification although they are efficient pre-treatment methods when used independently. To the best of our knowledge, this is the first report on the effect of combination of Pleurotus sp and NaOH pre-treatment on the HTSS of RS by a thermoactive xylanolytic cocktail. Our future research work is directed towards the isolation of xylanase-producing bacteria, molecular characterization of the xylanolytic enzymes produced by them and the cloning and expression of novel xylanase genes in suitable hosts for industrial applications.
Data Availability Statement
The original contributions presented in the study are included in the article/Supplementary Material, further inquiries can be directed to the corresponding author.
Author Contributions
SG and KL conceived and designed the study, SG, KL, and AB prepared the manuscript, KL, SK, PS, and PB collected the sample, developed the consortium and performed the experiments.
Funding
1. SERB, Department of Science and Technology (Project No. SB/SO/BB-020/2015), Government of India, 2. Departmental Research Project grant from University of North Bengal.
Conflict of Interest
The authors declare that the research was conducted in the absence of any commercial or financial relationships that could be construed as a potential conflict of interest.
Publisher’s Note
All claims expressed in this article are solely those of the authors and do not necessarily represent those of their affiliated organizations, or those of the publisher, the editors and the reviewers. Any product that may be evaluated in this article, or claim that may be made by its manufacturer, is not guaranteed or endorsed by the publisher.
Acknowledgments
SG acknowledges the SERB, Department of Science and Technology (Project No. SB/SO/BB-020/2015), Government of India, for financial support. KL acknowledges the Departmental Research Project grant from University of North Bengal for financial support. AB acknowledges the departmental fellowship (GATE) from University of North Bengal and PB acknowledges CSIR Junior Research Fellowship (NET). University Science Instrumentation Centre (USIC), NBU is acknowledged for performing SEM analysis.
Supplementary Material
The Supplementary Material for this article can be found online at: https://www.frontiersin.org/articles/10.3389/fenrg.2021.755779/full#supplementary-material
Abbreviations
β-ME, β-mercaptoethanol; CFS, Cell Free Supernatant; COFAM, Centre for Floriculture and Agri-Business Management; DM, Dry Matter; DNSA, Di Nitro Salicylic Acid; EDS, Energy Dispersive X-Ray Spectroscopy; EDTA, Ethylene Diamine Tetra Acetic acid; EU, Enzyme Unit; HTSS, High Temperature Small-scale Saccharification; NaOH RS, Alkali pre-treated rice straw; NaOH SRS, Alkali pre-treated spent rice straw; NEY, Non-Enzymatic Yield; pNP, para nitrophenol; RS, Rice straw; RSC, Rice Straw-based Consortium; SDS, Sodium Dodecyl Sulphate; SEM, Scanning Electron Microscopy; SRS, Spent Rice Straw; TY, Total Yield; XEP, Xylanase Enzyme Preparation.
References
Aggarwal, N. K., Goyal, V., Saini, A., Yadav, A., and Gupta, R. (2017). Enzymatic saccharification of pretreated rice straw by cellulases from Aspergillus niger BK01. 3 Biotech. 7, 158. doi:10.1007/s13205-017-0755-0
Ajijolakewu, K. A., Leh, C. P., Wan AbduLLah, W. N., and Lee, C. K. (2016). Assessment of the Effect of Easily-metabolised Carbon Supplements on Xylanase Production by Newly Isolated Trichoderma Asperellum USM SD4 Cultivated on Oil Palm Empty Fruit Bunches. BioResources 11, 9611–9627. doi:10.15376/biores.11.4.9611-9627
Anasontzis, G. E., Thuy, N. T., Hang, D. T. M., Huong, H. T., Thanh, D. T., Hien, D. D., et al. (2017). Rice straw hydrolysis using secretomes from novel fungal isolates from Vietnam. Biomass and Bioenergy 99, 11–20. doi:10.1016/j.biombioe.2017.02.008
Bhardwaj, N., Kumar, B., and Verma, P. (2019). A detailed overview of xylanases: an emerging biomolecule for current and future prospective. Bioresour. Bioproc. 6, 40. doi:10.1186/s40643-019-0276-2
Bradford, M. M. (1976). A rapid and sensitive method for the quantitation of microgram quantities of protein utilizing the principle of protein-dye binding. Anal. Biochem. 72, 248–254. doi:10.1016/0003-2697(76)90527-3
Cao, G.-L., Zhao, L., Wang, A.-J., Wang, Z.-Y., and Ren, N.-Q. (2014). Single-step bioconversion of lignocellulose to hydrogen using novel moderately thermophilic bacteria. Biotechnol. Biofuels 7, 82. doi:10.1186/1754-6834-7-82https://www.biotechnologyforbiofuels.com/content/7/1/82
Chakdar, H., Kumar, M., Pandiyan, K., Singh, A., Nanjappan, K., Kashyap, P. L., et al. (2016). Bacterial xylanases: biology to biotechnology. 3 Biotech. 6, 150. doi:10.1007/s13205-016-0457-z
Chen, K.-J., Tang, J.-C., Xu, B.-H., Lan, S.-L., and Cao, Y. (2019). Degradation enhancement of rice straw by co-culture of Phanerochaete chrysosporium and Trichoderma viride. Sci. Rep. 9, 19708. doi:10.1038/s41598-019-56123-5
Díaz-Godínez, G., Téllez-Téllez, M., Sánchez, C., and Díaz, R. (2017). “Characterization of the Solid-State and Liquid Fermentation for the Production of Laccases of Pleurotus ostreatus,” in Fermentation Processes. Editor A. F. Jozala (Mexico: IntechOpen), 57–74. doi:10.5772/64239
Djohan, A. C., Perwitasari, U., and Yopi, Y. (2016). Saccharification Waste Biomass Rice Straw IR-64 by Using Xylanase from Indigenous Marine Bacteria Bacillus safensis LBF-002. Int. J. Adv. Sci. Eng. Inf. Technol. 6, 40–44. doi:10.18517/ijaseit.6.1.558
Do, N. H., Pham, H. H., Le, T. M., Lauwaert, J., Diels, L., Verberckmoes, A., et al. (2020). The novel method to reduce the silica content in lignin recovered from black liquor originating from rice straw. Sci. Rep. 10, 21263. doi:10.1038/s41598-02077867-510.1038/s41598-020-77867-5
Gavande, P. V., Basak, A., Sen, S., Lepcha, K., Murmu, N., Rai, V., et al. (2021). Functional characterization of thermotolerant microbial consortium for lignocellulolytic enzymes with central role of Firmicutes in rice straw depolymerization. Sci. Rep. 11, 3032. doi:10.1038/s41598-021-82163-x
Ghose, T. K. (1987). Measurement of cellulase activities. Pure Appl. Chem. 59, 257–268. doi:10.1351/pac198759020257
Gomes, E., de Souza, A. R., Orjuela, G. L., Da Silva, R., de Oliveira, T. B., and Rodrigues, A. (2016). “Applications and Benefits of Thermophilic Microorganisms and Their Enzymes for Industrial Biotechnology,” in Gene Expression Systems in Fungi: Advancements and Applications. Editors M. Schmoll, and C. Dattenbock (Cham): Fungal Biology. Springer), 459–492. doi:10.1007/978-3-319-27951-010.1007/978-3-319-27951-0_21
Gou, G., Wei, W., Jiang, M., Zhang, S., Lu, T., Xie, X., et al. (2018). “Environmentally Friendly Method for the Separation of Cellulose from Steam-Exploded Rice Straw and Its High-Value Applications,” in Pulp and Paper Processing. (China: IntechOpen), 133–154. doi:10.5772/intechopen.79014
Harun, S., and Geok, S. K. (2016). Effect of Sodium Hydroxide Pretreatment on Rice Straw Composition. Indian J. Sci. Technol. 9, 1–9. doi:10.17485/ijst/2016/v9i21/95245
Ikramullah, Rizal, S., Thalib, S., and Huzni, S. (2018). Hemicellulose and Lignin Removal on Typha Fiber by Alkali Treatment. IOP Conf. Ser. Mater. Sci. Eng. 352, 012019. doi:10.1088/1757-899X/352/1/012019
Kamra, D. N. (2005). Rumen Microbial Ecosystem. Curr. Sci. 89, 124–135. https://www.jstor.org/stable/24110438.
Kogo, T., Yoshida, Y., Koganei, K., Matsumoto, H., Watanabe, T., Ogihara, J., et al. (2017). Production of rice straw hydrolysis enzymes by the fungi Trichoderma reesei and Humicola insolens using rice straw as a carbon source. Bioresour. Tech. 233, 67–73. doi:10.1016/j.biotech.2017.01.075
Kumar, S., Haq, I., Prakash, J., Singh, S. K., Mishra, S., and Raj, A. (2017). Purification, characterization and thermostability improvement of xylanase from Bacillus amyloliquefaciens and its application in pre-bleaching of kraft pulp. 3 Biotech. 7, 20. doi:10.1007/s13205-017-0615-y
Lepcha, K., and Ghosh, S. (2018). Glycoside hydrolases from a thermophilic microbial consortium and their implication in the saccharification of agroresidues. Biocatal. Agric. Biotechnol. 15, 160–166. doi:10.1016/j.bcab.2018.05.021
Malgas, S., Mafa, M. S., Mkabayi, L., and Pletschke, B. I. (2019). A mini review of xylanolytic enzymes with regards to their synergistic interactions during hetero-xylan degradation. World J. Microbiol. Biotechnol. 35, 187. doi:10.1007/s11274-019-2765-z
Mardawati, E., Werner, A., Bley, T., Mtap, K., and Setiadi, T. (2014). The enzymatic hydrolysis of oil palm empty fruit bunches to xylose. J. Jpn. Inst. Energ. 93, 973–978. doi:10.3775/jie.93.973
Parry, N. J., Beever, D. E., Owen, E., Vandenberghe, I., Beeumen, J. V., and Bhat, M. K. (2001). Biochemical characterization and mechanism of action of a thermostable β-glucosidase purified from Thermoascus aurantiacus. Biochem. J. 353, 117–127. doi:10.1042/bj3530117https://www.researchgate.net/publication/12213616
Phitsuwan, P., Permsriburasuk, C., Baramee, S., Teeravivattanakit, T., and Ratanakhanokchai, K. (2017). Structural Analysis of Alkaline Pretreated Rice Straw for Ethanol Production. Int. J. Polym. Sci. 2017, 1–9. doi:10.1155/2017/4876969
Phitsuwan, P., Sakka, K., and Ratanakhanokchai, K. (2016). Structural changes and enzymatic response of Napier grass (Pennisetum purpureum) stem induced by alkaline pretreatment. Bioresour. Tech. 218, 247–256. doi:10.1016/j.biotech.2016.06.089
Ravindran, R., Hassan, S., Williams, G., and Jaiswal, A. (2018). A Review on Bioconversion of Agro-Industrial Wastes to Industrially Important Enzymes. Bioengineering 5, 93. doi:10.3390/bioengineering5040093
Resch, M. G., Baker, J. O., and Decker, S. R. (2015). Low Solids Enzymatic Saccharification of Lignocellulosic Biomass. United States: National Renewable Energy Laboratory: Laboratory Analytical Procedure (LAP).
Romano, N., Gioffré, A., Sede, S. M., Campos, E., Cataldi, A., and Talia, P. (2013). Characterization of cellulolytic activities of environmental bacterial consortia from an Argentinian native forest. Curr. Microbiol. 67, 138–147. doi:10.1007/s00284-013-0345-2
Ryden, P., Efthymiou, M.-N., Tindyebwa, T. A. M., Elliston, A., Wilson, D. R., Waldron, K. W., et al. (2017). Bioethanol production from spent mushroom compost derived from chaff of millet and sorghum. Biotechnol. Biofuels 10, 195. doi:10.1186/s13068-017-0880-3
Seemakram, W., Boonrung, S., Aimi, T., Ekprasert, J., Lumyong, S., and Boonlue, S. (2020). Purification, characterization and partial amino acid sequences of thermo-alkali-stable and mercury ion-tolerant xylanase from Thermomyces dupontii KKU-CLD-E2-3. Sci. Rep. 10, 21663. doi:10.1038/s41598-020-78670-y
Shaikh, I. K., Dixit, P. P., and Shaikh, T. M. (2018). Purification and characterization of alkaline soda-bleach stable protease from Bacillus sp. APP-07 isolated from Laundromat soil. J. Genet. Eng. Biotechnol. 16, 273–279. doi:10.1016/j.jgeb.2018.07.003
Singh, D. P., Prabha, R., Renu, S., Sahu, P. K., and Singh, V. (2019). Agrowaste bioconversion and microbial fortification have prospects for soil health, crop productivity, and eco-enterprising. Int. J. Recycl Org. Waste Agricult 8, 457–472. doi:10.1007/s40093-019-0243-0
Smith, P. J., Wang, H.-T., York, W. S., Peña, M. J., and Urbanowicz, B. R. (2017). Designer biomass for next-generation biorefineries: leveraging recent insights into xylan structure and biosynthesis. Biotechnol. Biofuels 10, 286. doi:10.1186/s13068-017-0973-z
Suryawanshi, P. R., Badapanda, C., Singh, K. M., and Rathore, A. (2019). Exploration of the rumen microbial diversity and carbohydrate active enzyme profile of black Bengal goat using metagenomic approach. Anim. Biotechnol. 12, 1–14. doi:10.1080/10495398.2019.1609489
Synowiecki, J. (2010). Some applications of thermophiles and their enzymes for protein processing. Afr. J. Biotechnol. 9, 7020–7025. doi:10.5897/AJB10.966
Szabuniewicz, M., Hupp, E. W., and Brown, S. O. (1972). Clinical Relevancy of Deep Body Temperature, Heart Rate and Respiratory Rate in the Goat. Zentralbl Veterinarmed A 19, 555–562. doi:10.1111/j.1439-0441.1972.tb00509.x10.1111/j.1439-0442.1972.tb00509.x
Takano, M., and Hoshino, K. (2018). Bioethanol production from rice straw by simultaneous saccharification and fermentation with statistical optimized cellulase cocktail and fermenting fungus. Bioresour. Bioproc. 5, 16. doi:10.1186/s40643-018-0203-y
Thapa, S., Mishra, J., Arora, N., Mishra, P., Li, H., O′Hair, J., et al. (2020). Microbial cellulolytic enzymes: diversity and biotechnology with reference to lignocellulosic biomass degradation. Rev. Environ. Sci. Biotechnol. 19, 621–648. doi:10.1007/s11157-020-09536-y
Thomas, L., Joseph, A., Arumugam, M., and Pandey, A. (2013). Production, purification, characterization and over-expression of xylanases from actinomycetes. Indian J. Exp. Biol. 51, 875–884.
Walia, A., Guleria, S., Mehta, P., Chauhan, A., and Parkash, J. (2017). Microbial xylanases and their industrial application in pulp and paper biobleaching: a review. 3 Biotech. 7, 11. doi:10.1007/s13205-016-0584-6
Wang, K., Cao, R., Wang, M., Lin, Q., Zhan, R., Xu, H., et al. (2019). A novel thermostable GH10 xylanase with activities on a wide variety of cellulosic substrates from a xylanolytic Bacillus strain exhibiting significant synergy with commercial Celluclast 1.5 L in pretreated corn stover hydrolysis. Biotechnol. Biofuels 12, 48. doi:10.1186/s13068-019-1389-8
Wood, I. P., Cao, H.-G., Tran, L., Cook, N., Ryden, P., Wilson, D. R., et al. (2016). Comparison of saccharification and fermentation of steam exploded rice straw and rice husk. Biotechnol. Biofuels 9, 193. doi:10.1186/s13068-016-0599-6
Keywords: thermoactive xylanases, thermoactive bacterial consortium, pre-treatment, saccharification, spent rice straw
Citation: Lepcha K, Basak A, Kanoo S, Sharma P, BK P and Ghosh S (2021) Thermoxylanolytic and Thermosaccharolytic Potential of a Heat Adapted Bacterial Consortium Developed From Goat Rumen Contents. Front. Energy Res. 9:755779. doi: 10.3389/fenrg.2021.755779
Received: 09 August 2021; Accepted: 14 September 2021;
Published: 01 October 2021.
Edited by:
Abhishek Guldhe, Amity University, IndiaReviewed by:
Santhosh Pillai, Durban University of Technology, South AfricaRicha Arora, Punjab Agricultural University, India
Copyright © 2021 Lepcha, Basak, Kanoo, Sharma, BK and Ghosh. This is an open-access article distributed under the terms of the Creative Commons Attribution License (CC BY). The use, distribution or reproduction in other forums is permitted, provided the original author(s) and the copyright owner(s) are credited and that the original publication in this journal is cited, in accordance with accepted academic practice. No use, distribution or reproduction is permitted which does not comply with these terms.
*Correspondence: Khusboo Lepcha, a2h1c2Jvb2xlcGNoYTg4QG5idS5hYy5pbg==