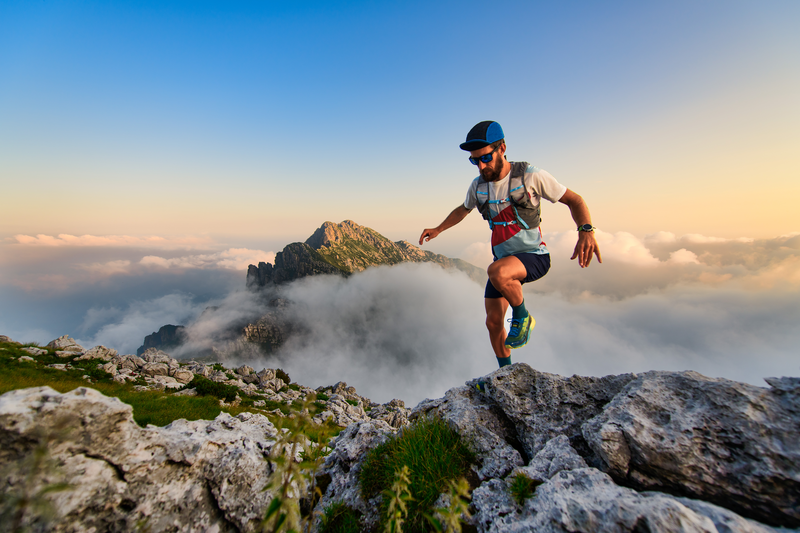
95% of researchers rate our articles as excellent or good
Learn more about the work of our research integrity team to safeguard the quality of each article we publish.
Find out more
MINI REVIEW article
Front. Energy Res. , 20 October 2021
Sec. Electrochemical Energy Storage
Volume 9 - 2021 | https://doi.org/10.3389/fenrg.2021.754532
This article is part of the Research Topic Oriented Nanostructures for Energy Conversion and Storage View all 9 articles
Thermoelectric material is a kind of functional material that uses the movement of carriers inside a solid to realize the direct mutual conversion of heat and electric energy. It provides a safe, reliable, pollution-free, noise-free, all-solid-state power generation and cooling method and has a wide range of application prospects. Among them, the characteristics of low valence band degeneracy, low effective quality of conduction band, strong phonon non-harmonicity, simple crystal structure and adjustable microstructure have made PbTe-based materials the focus of research in the thermoelectric field. In this review, two latest strategies to improve the thermoelectric properties of PbTe-based materials are discussed, and the challenges for the further development of PbTe-based thermoelectric materials and the prospects for the future are also outlined.
PbTe has a highly symmetric face-centered cubic crystal structure, as shown in Figure 1A (Xiao and Zhao, 2018), so it has excellent electrical conductivity. Its electronic energy band structure shows that the maximum value of the valence band is 7.00389 ev, the minimum value of the conduction band is 7.80069 ev and the Fermi level is located at 7.49630 ev (Akinlami et al., 2018), as shown in Figure 1B. Because the maximum value of the valence band and the minimum value of the conduction band are located in k-space at the same position (Akinlami et al., 2018), this means that the material is a direct bandgap semiconductor, and it is also a narrow bandgap semiconductor (Zhai et al., 2018), because its ideal forbidden band width is 0.32 ev (Tan et al., 2015). This band structure is conducive to electron transmission and gives PbTe a highly complex Seebeck coefficient (Zhai et al., 2018). In addition, due to the strong non-harmonics caused by the local eccentricity of Pb, PbTe has a lower lattice thermal conductivity (2.2 W/mk) (Zhu et al., 2020). Therefore, PbTe is a promising medium temperature power generation material and it is one of the most advanced traditional TE materials that have been used in the medium temperature range for the past 50 years. In recent decades, the p-type PbTe has excellent performance, and the maximum value of zT can reach about 2.5 and the average of zT is about 1.4. However, the maximum value of zT for n-type PbTe is only about 1.8 and the average value of zT is about 1 (Luo et al., 2019), as shown in Figures 1C,D. The key to the promotion and application of this promising power generation technology is to improve the low conversion efficiency of current TE materials, which is technically evaluated by the dimensionless thermoelectric
FIGURE 1. (A) Crystal structure of PbTe. (B) The electronic band structure of PbTe. Reproduced with permission (Xiao and Zhao, 2018) Copyright 2018, Springer Nature Limited. (C) The peak of zT. (D) The average value of zT. Reproduced with permission (Zhong et al., 2020) Copyright 2020, American Chemical Society. (E) Schematic image of band convergence. (F) The deep impurity level is located in the band gap and captures electrons at 300 K. (G) the deep impurity level releases electrons into the conduction band at high temperature. Reproduced with permission (Xiao and Zhao, 2018) Copyright 2018, Springer Nature Limited. (H) Defect levels introduced via In doping to optimize carrier concentration. (I) Enhance point defect scattering through In doping and S alloying to maintain high power factor. Reproduced with permission (Wang et al., 2019) Copyright 2019, John Wiley and Sons, Inc. (J) Dislocations. (K) Grain boundaries. (L) Nanoprecipitates. Reproduced with permission (Xiao and Zhao, 2018) Copyright 2018, Springer Nature Limited.
The fundamental challenge of designing high zT TE materials comes from the strong correlation between the carrier concentration and S, σ and κ, which can be improved by controlling the doping level to adjust the carrier concentration (Zhou et al., 2018). In addition, the introduction of nanostructures into the system to reduce the thermal conductivity of materials by enhancing phonon scattering is also the main method to optimize thermoelectric performance (Chen et al., 2018). Below we will briefly describe these two strategies.
The chemical bond in PbTe is a mixed ion-covalent bond, so it is generally classified as a polar semiconductor. N-type or p-type semiconductor materials can be formed by doping, where n-type semiconductor means that the concentration of free electrons is much higher than the concentration of holes and vice versa, it is a p-type semiconductor. When the dopant is not present, the atoms in the crystalline material are arranged regularly and orderly. When the dopant is present, as the temperature changes, the atoms are arranged disorderly and produce lattice defects. The different atomic sizes of various dopants cause atomic-level lattice distortion and significant strain around the impurity atoms (Cojocaru et al., 2017). It changes the material of carrier concentration, band structure and lattice compatibility, reduce the thermal conductivity (Chang et al., 2020). For PbTe-based TE materials, it is usually used to adjust donor and acceptor doping to obtain n-type or p-type TE materials, respectively. When introducing heterovalent point defects to electronically dope the material, these defects are first thought to provide carriers for the system, rather than causing charged vacancies. In order to obtain p-type PbTe, alkali metals can be used as acceptor dopants. In the experiment, most of the p-type PbTe materials with excellent performance are doped with Na and K. One of the solubility of doping atoms depends on the temperature. By adjusting the temperature, the solubility of the doping atoms can be improved. The number of holes introduced by each doping atom is as close as possible to 100%, which improves the doping efficiency (Jood et al., 2020). For example, at high temperatures, Na can enter the PbTe lattice beyond the solubility limit, converge the energy band, as shown in Figure 1E, enhance TE transmission and increase Seebeck coefficient, thereby improving thermoelectric performance (Jood et al., 2020). In recent years of research, PbTe is alloyed with elements such as Eu (Chen et al., 2017), Sr and Mn to converge the energy band and then the dislocation density is increased by doping with heterovalent atoms (Jood et al., 2020). As the concentration of heterovalent atoms further increased, nano-scale precipitation appeared and the dislocation density gradually decreased. This transition from point defects to lattice defects and finally into nano-precipitation directly leads to a decrease in thermal conductivity, which further improves the zT value. For example, when the temperature is 823k, Chen et al. making a new type of alloy material EuTe is used in the energy band engineering PbTe material. Compared with Pb, Eu has a slightly larger size, which expands the crystal lattice. Eu replacing Pb can increase the unit cell size of PbTe and alloying with EuTe can promote band convergence. The solubility of Na in PbTe increases with the increase of EuTe content. When the Na content increases to a certain value, the zT value can reach 2.4 (Chen et al., 2017).
The n-type PbTe TE material can be realized by doping Ga, In, La, Sb, Al, Bi and other atoms at the Pb site and Cl, Br, I and other atoms doping at the Te site. For example, by changing the stoichiometric ratio of PbTe and doping with I atoms, various thin film samples with electron concentrations ranging from n = 8 × 1016–2 × 1018 cm−3 can be obtained. Its Seebeck coefficient, lattice thermal conductivity, quality factor and other TE properties increase with the increase of electron concentration (Dzundza et al., 2020). Due to the intensity of the Pb atoms eccentric vibration, the band gap of PbTe increases significantly with the increase of temperature. As the temperature rises, the band gap evolves and the band shape begins to flatten, which means that the effective carrier mass also increases (Xiao et al., 2018). Because larger effective carrier mass requires higher carrier density to achieve the best power coefficient (Su et al., 2018), it is necessary to adjust the carrier density dynamically with the increase of temperature in PbTe based materials (Chen et al., 2019). In Pb0.98In0.005Sb0.015Te, the impurity level formed by adding In element will dynamically optimize the carrier concentration in the entire working temperature range with the increase of temperature (Zhang et al., 2018), as shown in Figures 1F–H. This is because when In is doped in PbTe, its deep defect state can trap electrons, so that the trapped electrons at low temperatures are thermally activated to return to the conduction band at high temperatures. As the temperature increases, the charge state of In changes from a mixed charge state to a complete +3 state, so the carrier concentration can be increased (Bali et al., 2016). Increase the carrier density from 2.18 × 1019 cm−3 at 300 K to 4.84 × 1019 cm−3 at 823 K and further adjust the conductivity, Seebeck coefficient and electrical transmission properties. Then, the phonon propagation is suppressed by alloying with sulfur to form a complete solid solution and at the same time, it is beneficial to the carrier mobility to maintain high power factor, as shown in Figure 1I. The lattice thermal conductivity can be further reduced from 0.76 Wm-1 K−1 to 0.42 Wm−1K−1. Combining the advantages of doping and S alloying, zTmax = 1.4 and zTave = 0.87 (300–873 K) can be obtained in Pb0.98In0.005Sb0.015Te0.94S0.06 (Wang et al., 2019).
One of the important factors to achieve high TE conversion efficiency is the need for low thermal conductivity. The lattice thermal conductivity
FIGURE 2. (A) Full-scale hierarchical structure of scattered phonons. (B) Phase diagram to guide full-scale hierarchical architecture: Eutectic phase diagram. (C) Spinodal decomposition diagram. Reproduced with permission (Xiao and Zhao, 2018) Copyright 2018, Springer Nature Limited. (D) A typical radioisotope thermoelectric generator, including thermal insulation, thermoelectric modules and so on. (Source from NASA/DOE/JPL) (E) Wearable TE device. (F) Principle. Reproduced with permission (Li et al., 2019) Copyright 2019, Elsevier B.V.
PbTe is a semiconductor TE material with excellent performance in the mid-temperature region. It is widely used in deep space exploration and waste heat recovery systems, such as radioisotope thermoelectric generators (as shown in Figure 2D) and waste heat recovery in automotive thermoelectric generators. In addition, researchers have also found it can be used to manufacture flexible TE conversion equipment for wearable applications and many other fields (Yang et al., 2017), as shown in Figures 2E,F. These devices have many advantages, such as excellent reliability, no noise and no pollution.
For how to improve the thermoelectric performance of PbTe-based TE materials, researchers have proposed a variety of strategies, such as nanostructures, adjustment of doping and alloy concentration, energy band engineering, design of all-scale layered architectures, synergistic effects, etc. The trend of future improvement should be to maximize the performance of TE by using collaborative strategies and integrating all effective factors to the greatest extent. At the same time, the improvement of the thermoelectric performance of n-type PbTe cannot be ignored. The use of reducing point defects to obtain higher carrier mobility (Wang et al., 2019) and the design of a comprehensive layered structure to disperse the whole field phonons (Liu et al., 2019) are important ways to increase the zT value. Secondly, there is huge room for improvement in TE conversion efficiency. The maximum conversion efficiency of PbTe based nanostructure module is about 8.8% at a temperature difference of 570 K (Tan et al., 2019). When Bi2Te3 is used for segmentation, the efficiency increases to about 11% at a temperature difference of 590 K, which is a record high value of PbTe based system (Tan et al., 2019). Exploring these possibilities and further improving thermoelectric performance can make TE materials and equipment more suitable for daily life.
Compatible PbTe thermoelectric semiconductor materials are the key components of TE devices. For PbTe thermoelectric materials, it is a strategy to dynamically dope PbTe with elements whose solubility is closely related to temperature. Another method is to introduce a deep level defect, which provides holes as acceptor impurities at low temperatures, and at high temperatures, the deep defect level is excited to provide electrons to optimize the carrier concentration. When the dislocation density is uniform, its scattering is about the same as the phonon-phonon scattering at room temperature (Shi et al., 2020), which can reduce the thermal conductivity by about 80%. Therefore, the study of dislocation scattering in the crystal may be the main direction that can reduce the thermal conductivity. The use of n-type and p-type dopants has also been widely used in various materials to prepare high-performance thermoelectric devices. For example, the use of n-type and p-type doping to produce a p-n junction with a power density of 1.18 mW cm−2 TE module (An et al., 2017).
All authors listed have made a substantial, direct, and intellectual contribution to the work, and approved it for publication.
National Natural Science Foundation of China (51702091) and Natural Science Foundation of Hubei Province of China (No. 2020CFB245).
The authors declare that the research was conducted in the absence of any commercial or financial relationships that could be construed as a potential conflict of interest.
All claims expressed in this article are solely those of the authors and do not necessarily represent those of their affiliated organizations, or those of the publisher, the editors and the reviewers. Any product that may be evaluated in this article, orclaim that may be made by its manufacturer, is not guaranteed or endorsed by the publisher.
The authors thank the College Outstanding Young Scientific and Technological Innovation Team of Hubei province (T201922).
Akinlami, J. O., Omeike, M. O., Akindiilete, J. A., and Abdulfatai, L. O. (2018). Electronic Properties and the Phonon Band Structure of PbTe. Comput. Condens. Matter 15, 90–94. doi:10.1016/j.cocom.2017.10.005
An, C. J., Kang, Y. H., Song, H., Jeong, Y., and Cho, S. Y. (2017). High-performance Flexible Thermoelectric Generator by Control of Electronic Structure of Directly Spun Carbon Nanotube Webs with Various Molecular Dopants. J. Mater. Chem. A. 5 (30), 15631–15639. doi:10.1039/C7TA02509B
Bali, A., Chetty, R., Sharma, A., Rogl, G., Heinrich, P., Suwas, S., et al. (2016). Thermoelectric Properties of in and I Doped PbTe. J. Appl. Phys. 120 (17), 175101. doi:10.1063/1.4965865
Biswas, K., He, J., Blum, I. D., Wu, C.-I., Hogan, T. P., Seidman, D. N., et al. (2012). High-performance Bulk Thermoelectrics with All-Scale Hierarchical Architectures. Nature 489 (7416), 414–418. doi:10.1038/nature11439
Chang, C.-C., Liu, C.-H., Wu, C.-C., Bag, P., and Kuo, Y.-K. (2020). Thermoelectric Properties of (HgTe)0.55(PbTe)0.45 Eutectic Composite with in Doping. Mater. Res. Bull. 129, 110916. doi:10.1016/j.materresbull.2020.110916
Chen, X.-Q., Fan, S.-J., Han, C., Wu, T., Wang, L.-J., Jiang, W., et al. (2021). Multiscale Architectures Boosting Thermoelectric Performance of Copper Sulfide Compound. Rare Met. 40 (8), 2017–2025. doi:10.1007/s12598-020-01698-6
Chen, X., Li, Z., Yang, J., Sun, Q., and Dou, S. (2015). Aqueous Preparation of Surfactant-free Copper Selenide Nanowires. J. Colloid Interf. Sci. 442, 140–146. doi:10.1016/j.jcis.2014.11.052
Chen, X., Yang, J., Wu, T., Li, L., Luo, W., Jiang, W., et al. (2018). Nanostructured Binary Copper Chalcogenides: Synthesis Strategies and Common Applications. Nanoscale 10 (32), 15130–15163. doi:10.1039/c8nr05558k
Chen, X., Zhang, H., Zhao, Y., Liu, W.-D., Dai, W., Wu, T., et al. (2019). Carbon-encapsulated Copper Sulfide Leading to Enhanced Thermoelectric Properties. ACS Appl. Mater. Inter. 11 (25), 22457–22463. doi:10.1021/acsami.9b06212
Chen, Z., Jian, Z., Li, W., Chang, Y., Ge, B., Hanus, R., et al. (2017). Lattice Dislocations Enhancing Thermoelectric PbTe in Addition to Band Convergence. Adv. Mater. 29 (23), 1606768. doi:10.1002/adma.201606768
Cojocaru, B., Avram, D., Kessler, V., Parvulescu, V., Seisenbaeva, G., and Tiseanu, C. (2017). Nanoscale Insights into Doping Behavior, Particle Size and Surface Effects in Trivalent Metal Doped SnO2. Sci. Rep. 7 (1), 9598. doi:10.1038/s41598-017-09026-2
Dzundza, B., Nykyruy, L., Parashchuk, T., Ivakin, E., Yavorsky, Y., Chernyak, L., et al. (2020). Transport and Thermoelectric Performance of N-type PbTe Films. Physica B Condens. Matter 588, 412178. doi:10.1016/j.physb.2020.412178
Fan, S., Zhao, J., Guo, J., Yan, Q., Ma, J., and Hng, H. H. (2010). p-Type Bi0.4Sb1.6Te3 Nanocomposites with Enhanced Figure of merit. Appl. Phys. Lett. 96 (18), 182104. doi:10.1063/1.3427427
Fu, L., Yin, M., Wu, D., Li, W., Feng, D., Huang, L., et al. (2017). Large Enhancement of Thermoelectric Properties in N-type PbTe via Dual-Site point Defects. Energy Environ. Sci. 10 (9), 2030–2040. doi:10.1039/c7ee01871a
Jood, P., Male, J. P., Anand, S., Matsushita, Y., Takagiwa, Y., Kanatzidis, M. G., et al. (2020). Na Doping in PbTe: Solubility, Band Convergence, Phase Boundary Mapping, and Thermoelectric Properties. J. Am. Chem. Soc. 142 (36), 15464–15475. doi:10.1021/jacs.0c07067
Li, C., Jiang, F., Liu, C., Liu, P., and Xu, J. (2019). Present and Future Thermoelectric Materials toward Wearable Energy Harvesting. Appl. Mater. Today 15, 543–557. doi:10.1016/j.apmt.2019.04.007
Liu, H., Chen, Z., Yin, C., Zhou, B., Liu, B., and Ang, R. (2019). Carrier Tuning and Multiple Phonon Scattering Induced High Thermoelectric Performance in N-type Sb-Doped PbTe Alloys. Appl. Phys. A. 125 (4). doi:10.1007/s00339-019-2525-9
Liu, Z., Mao, J., Liu, T.-H., Chen, G., and Ren, Z. (2018). Nano-microstructural Control of Phonon Engineering for Thermoelectric Energy Harvesting. MRS Bull. 43 (3), 181–186. doi:10.1557/mrs.2018.7
Luo, Z.-Z., Cai, S., Hao, S., Bailey, T. P., Su, X., Spanopoulos, I., et al. (2019). High Figure of Merit in Gallium-Doped Nanostructured N-type PbTe-xGeTe with Midgap States. J. Am. Chem. Soc. 141 (40), 16169–16177. doi:10.1021/jacs.9b09249
Sharma, P., Minakshi Sundaram, M., Watcharatharapong, T., Laird, D., Euchner, H., Ahuja, R., et al. (2020). Zn Metal Atom Doping on the Surface Plane of One-Dimesional NiMoO4 Nanorods with Improved Redox Chemistry. ACS Appl. Mater. Inter. 12 (40), 44815–44829. doi:10.1021/acsami.0c13755
Shi, X.-L., Zou, J., and Chen, Z.-G. (2020). Advanced Thermoelectric Design: from Materials and Structures to Devices. Chem. Rev. 120 (15), 7399–7515. doi:10.1021/acs.chemrev.0c00026
Su, X., Hao, S., Bailey, T. P., Wang, S., Hadar, I., Tan, G., et al. (2018). Weak Electron Phonon Coupling and Deep Level Impurity for High Thermoelectric Performance Pb 1− X Ga X Te. Adv. Energ. Mater. 8 (21), 1800659. doi:10.1002/aenm.201800659
Tan, G., Ohta, M., and Kanatzidis, M. G. (2019). Thermoelectric Power Generation: from New Materials to Devices. Phil. Trans. R. Soc. A. 377 (2152), 20180450. doi:10.1098/rsta.2018.0450
Tan, G., Zhao, L.-D., and Kanatzidis, M. G. (2016). Rationally Designing High-Performance Bulk Thermoelectric Materials. Chem. Rev. 116 (19), 12123–12149. doi:10.1021/acs.chemrev.6b00255
Tan, X., Shao, H., Hu, T., Liu, G.-Q., and Ren, S.-F. (2015). Theoretical Understanding on Band Engineering of Mn-Doped lead Chalcogenides PbX (X = Te, Se, S). J. Phys. Condens. Matter 27 (9), 095501. doi:10.1088/0953-8984/27/9/095501
Wang, D., Qin, Y., Wang, S., Qiu, Y., Ren, D., Xiao, Y., et al. (2019). Synergistically Enhancing Thermoelectric Performance of n‐Type PbTe with Indium Doping and Sulfur Alloying. Annalen Der Physik 532 (11), 1900421. doi:10.1002/andp.201900421
Watcharatharapong, T., Minakshi Sundaram, M., Chakraborty, S., Li, D., Shafiullah, G., Aughterson, R. D., et al. (2017). Effect of Transition Metal Cations on Stability Enhancement for Molybdate-Based Hybrid Supercapacitor. ACS Appl. Mater. Inter. 9 (21), 17977–17991. doi:10.1021/acsami.7b03836
Wu, D., Zhao, L.-D., Tong, X., Li, W., Wu, L., Tan, Q., et al. (2015). Superior Thermoelectric Performance in PbTe-PbS Pseudo-binary: Extremely Low thermal Conductivity and Modulated Carrier Concentration. Energ. Environ. Sci. 8 (7), 2056–2068. doi:10.1039/c5ee01147g
Xiao, Y., Wu, H., Cui, J., Wang, D., Fu, L., Zhang, Y., et al. (2018). Realizing High Performance N-type PbTe by Synergistically Optimizing Effective Mass and Carrier Mobility and Suppressing Bipolar thermal Conductivity. Energ. Environ. Sci. 11 (9), 2486–2495. doi:10.1039/c8ee01151f
Xiao, Y., and Zhao, L.-D. (2018). Charge and Phonon Transport in PbTe-Based Thermoelectric Materials. Npj Quant Mater. 3 (1), 55. doi:10.1038/s41535-018-0127-y
Yang, L., Chen, Z. G., Dargusch, M. S., and Zou, J. (2017). High Performance Thermoelectric Materials: Progress and Their Applications. Adv. Energ. Mater. 8 (6), 1701797. doi:10.1002/aenm.201701797
Zhai, J., Wang, T., Wang, H., Su, W., Wang, X., Chen, T., et al. (2018). Strategies for Optimizing the Thermoelectricity of PbTe Alloys. Chin. Phys. B 27 (4), 047306. doi:10.1088/1674-1056/27/4/047306
Zhang, J., Wu, D., He, D., Feng, D., Yin, M., Qin, X., et al. (2017). Extraordinary Thermoelectric Performance Realized in N-type PbTe through Multiphase Nanostructure Engineering. Adv. Mater. 29 (39), 1703148. doi:10.1002/adma.201703148
Zhang, Q., Song, Q., Wang, X., Sun, J., Zhu, Q., Dahal, K., et al. (2018). Deep Defect Level Engineering: a Strategy of Optimizing the Carrier Concentration for High Thermoelectric Performance. Energ. Environ. Sci. 11 (4), 933–940. doi:10.1039/c8ee00112j
Zhong, Y., Tang, J., Liu, H., Chen, Z., Lin, L., Ren, D., et al. (2020). Optimized Strategies for Advancing N-type PbTe Thermoelectrics: a Review. ACS Appl. Mater. Inter. 12 (44), 49323–49334. doi:10.1021/acsami.0c15730
Zhou, X., Yan, Y., Lu, X., Zhu, H., Han, X., Chen, G., et al. (2018). Routes for High-Performance Thermoelectric Materials. Mater. Today 21 (9), 974–988. doi:10.1016/j.mattod.2018.03.039
Keywords: thermoelectric properties, nanostructure, thermal conductivity, carrier concentration, doping modification
Citation: Hao X, Chen X, Zhou X, Zhang L, Tao J, Wang C, Wu T and Dai W (2021) Performance Optimization for PbTe-Based Thermoelectric Materials. Front. Energy Res. 9:754532. doi: 10.3389/fenrg.2021.754532
Received: 06 August 2021; Accepted: 04 October 2021;
Published: 20 October 2021.
Edited by:
Kai S. Exner, University of Duisburg-Essen, GermanyReviewed by:
Manickam Minakshi, Murdoch University, AustraliaCopyright © 2021 Hao, Chen, Zhou, Zhang, Tao, Wang, Wu and Dai. This is an open-access article distributed under the terms of the Creative Commons Attribution License (CC BY). The use, distribution or reproduction in other forums is permitted, provided the original author(s) and the copyright owner(s) are credited and that the original publication in this journal is cited, in accordance with accepted academic practice. No use, distribution or reproduction is permitted which does not comply with these terms.
*Correspondence: Xinqi Chen, Y2hlbnhpbnFpQGh1ZS5lZHUuY24=
Disclaimer: All claims expressed in this article are solely those of the authors and do not necessarily represent those of their affiliated organizations, or those of the publisher, the editors and the reviewers. Any product that may be evaluated in this article or claim that may be made by its manufacturer is not guaranteed or endorsed by the publisher.
Research integrity at Frontiers
Learn more about the work of our research integrity team to safeguard the quality of each article we publish.