- Institute for Advanced Sustainability Studies, Potsdam, Germany
Like other hard-to-abate sectors, the cement and concrete industry is facing growing pressure to reduce CO2 emissions. In this context, the carbonation of minerals or industrial wastes with CO2 (CO2 mineralization) is attracting growing interest in research and industry as well as among policy makers. Despite their technical feasibility, few of these innovative carbon capture and utilization (CCU) technologies have so far reached the commercialization stage. Due to their low maturity and potentially higher market prices, these technologies presently require policy support in order to realize their full sustainability potentials. This paper elucidates which policies are considered appropriate, in the literature, for fostering the further development and implementation of CCU technologies and thus achieving the sustainability potential of CO2 mineralization applications. First, we performed a meta-analysis of recent literature in order to identify policies and measures that potentially represent barriers or incentives to the development and deployment of CO2 mineralization technologies, and categorized them as technology-push or market-pull policies. As a second step, we conducted an online survey of policy-making priorities among experts in the field. This identified numerous relevant policies, of which the majority are market-oriented. While most existing market-pull policies do currently not support CCU technologies and would require adaptation to do so, technology-push policies already provide support for their development. However, while the need for technology-push support in the early development phases is still continued, the broad spectrum of market-pull policies that are considered relevant shows that a shifting focus of policy support is required to better address the current state of development of CO2 mineralization technologies and their upcoming market entry.
Introduction
Cement is among the most used substances on Earth (IEA, 2019). Since 2014, global cement production has remained consistently high at around 4 Gt per year (IEA, 2020), accounting for approximately 7 percent of annual global anthropogenic CO2 emissions (IEA, 2020; Le Quéré et al., 2018). Thus, the cement industry is facing the urgent necessity to reduce CO2 emissions in order to meet national and international climate goals, and, as a consequence, to remain competitive in the future. Therefore, ambitious agendas and strategies are being developed to secure the future viability of the sector, such as the Carbon Neutrality Roadmap published by the European Cement Association (Cembureau, 2020a) and HeidelbergCement’s “Beyond 2020” strategy (Beumelburg, 2020). In this context, the carbonation of minerals or industrial wastes with CO2 is attracting growing interest in research and industry as well as among policy makers (Schlögl et al., 2018; Sanna et al., 2014). In such “carbonation” or “mineralization” processes, CO2 is reacted with virgin minerals or certain types of industrial waste to form a solid carbonate, resulting in an effectively permanent means of sequestering CO2 (SCOT project, 2016; Sandalow et al., 2017). In addition to their possible contribution to reducing the carbon footprint of the construction sector, CCU technologies can create value-added products in the form of cement-like construction materials, such as cementitious materials, aggregates, and concrete (Hendriks et al., 2013; IEA, 2019). Thus, they may provide additional or more economical options for the industry’s emission reduction strategies.
CO2 mineralization pathways are considered a promising carbon capture and utilization (CCU) technology. These technologies aim to utilize carbon dioxide captured from sources—such as industrial point sources, direct air capture, or byproducts from chemical processes—in the production of valuable goods such as plastics, fuels, or construction materials. The aim of most CCU technologies is to replace carbon from fossil resources and thus reduce emissions and the use of fossil resources (Aresta and Dibenedetto, 2010; Styring et al., 2011; Zero Emissions Platform 2013; IASS, 2016; Group of Chief Scientific Advisors, 2018). The expected benefits of CCU pathways differ widely between applications and depend on many factors. Therefore, they need to be monitored and assessed individually (von der Assen et al., 2013). The development and deployment of CO2 carbonation technologies is expected to not only contribute to achieving Europe’s aims for decreasing overall carbon emissions, but to also foster transitioning towards a circular economy under certain conditions (European Circular Economy Stakeholder Platform, 2018; Cembureau, 2020b) In addition to representing a potential sink for CO2, mineral carbonation of certain sorts of waste has the ability to support ambitions to reduce landfilling (Wilson et al., 2016).
CO2 Mineralization Pathways
CO2 mineralization technologies can be grouped according to their input materials as well as their implementation along the value chain. In currently discussed pathways CO2 is either reacted with selected minerals or certain types of industrial wastes (Figure 1, left) or is used during the curing (i.e., hardening) of conventional concrete (CO2 curing of concrete) (Figure 1, right) to form a solid carbonate that permanently stores CO2 (SCOT project 2016). These processes can lead to marketable CO2-derived products for the construction industry, such as cement additives (supplementary cementitious materials, SCM), synthetic aggregates and CO2-cured concrete (Hendriks et al., 2013), resulting in low-emission building materials (i.e., cement, aggregates and concrete).
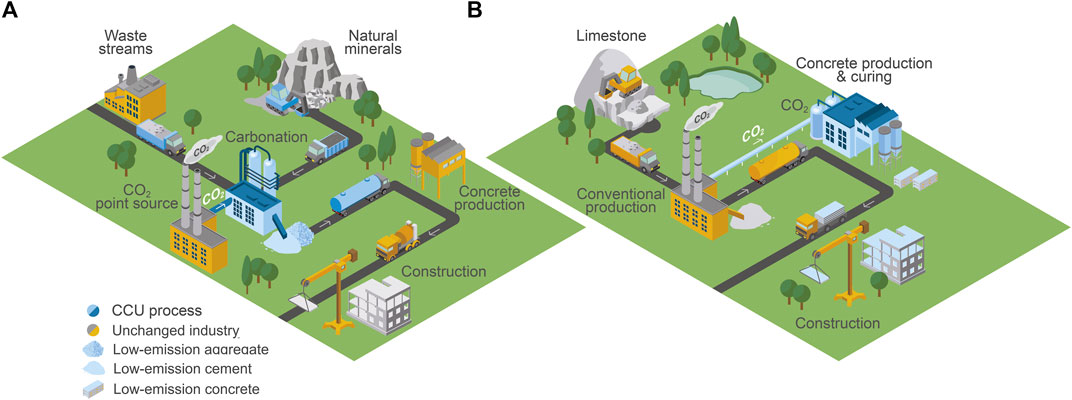
FIGURE 1. CO2 mineralization concepts in the cement and construction industry. (A): Carbonation of minerals or wastes (B): CO2 curing of concrete.
Processes using virgin minerals or industrial wastes mainly consider calcium or magnesium-rich silicates as well as steel slag, cement-kiln dust and fly ash as feedstocks (Sanna et al., 2014). During the reaction, silica is produced as a byproduct, which makes the carbonated material usable as a cement additive, thus reducing the amount of cement that needs to be produced (Ostovari et al., 2020; Kremer et al., 2019). However, although carbonation products are foreseen as a cement replacement, it must be recognized that cement is a highly standardized product with restrictions on the content of certain trace metals. Hence another usage with less stringent compositional specifications is aggregates, which has been demonstrated at scale (Carbon8, 2020). Both cement and aggregate (together with water) are used in the production of concrete. Concrete can be used in pre-cast products or else produced in ready-mixed form, which is transported in trucks and set on site (IEA, 2019). Concrete gains its strength as its constituents bind together during the curing stage. In this step CO2 can be introduced and reacts with alkaline Earth metal oxides (e.g., calcium oxide) present in cement to form stabile carbonates (Shao, 2014). CO2 can be either introduced when mixing the concrete on site or, in the case of pre-cast production, in curing chambers. Some methods of CO2 mineralization require less cement in the final concrete mixture, thereby reducing CO2 emissions from cement production. When using industrial wastes as feedstocks for carbonation, an additional outcome is the diversion of otherwise landfilled material. Therefore, converting waste materials into products for the construction sector creates economic benefits not only in terms of the value of the new products but also by avoiding landfill costs (Sandalow et al., 2017). Additionally, due to increasing restrictions on mining activities and the depletion of quality aggregate sources close to urban areas, pressures on natural aggregate resources have increased globally (Krausmann et al., 2018). Thus, producing CO2-based aggregates from waste-stream feedstocks may help alleviate this pressure by reducing the extraction of virgin materials (Turnau et al., 2019).
Aim and Scope of This Paper
Some carbonation technologies are already technologically feasible, and many could be scaled up (IEA, 2019). Nevertheless, very few carbonation products are currently commercially available, such as for instance the aggregate manufactured by the United Kingdom company Carbon8 (Carbon8, 2020). Others have not reached the commercialization stage, or have not yet entered the market despite being considered among the most mature CCU technologies (Zimmermann and Kant, 2016; Sandalow et al., 2017). Public support may be a decisive factor for implementing CCU technologies (or at least accelerating their advancement), otherwise their market entry is likely to be inhibited by anticipated higher costs relative to conventional benchmark products (Wilson et al., 2016; Sandalow et al., 2017). Thus, the subsequent pathways of these technologies might be significantly altered when accompanied by strategic political action (ICEF, CO2 Sciences and The Global CO2 Initiative, 2016). Therefore, this paper focuses on the relevant political framework conditions which could have an impact on CCU technologies, specifically in the field of mineralization.
To better understand why—despite proven technical feasibility—few CO2 mineralization technologies have so far reached the commercialization stage, this paper aims to clarify which policies are considered relevant to furthering their development and implementation and, thus, which policies might prove effective in unfolding the sustainable potential of such technologies.
Assessment
To elaborate on policy requirements for the market entry of CO2-mineralization technologies, this article presents the results of a two-step analysis. First, we performed a meta-analysis of recent policy reports on CCU, to identify and structure the policy measures that are considered to be potential barriers or incentives for the development and deployment of CO2 carbonation technologies. We also conducted an expert survey, to complement the findings of the literature review and allow for well-founded recommendations and conclusions on the policy framework necessary to foster the development and sustainable implementation of mineralization technologies.
Methods
Literature Review
As suitable material to gain insights on the perceived relevance of policy measures for CO2 mineralization technologies, we selected science-based reports about CCU intended for a non-scientific audience, usually targeting policy- and decision makers (“policy reports”). To match the particular focus of this paper, we chose not to include scientific papers covering technical aspects of CO2 mineralization processes, since they usually lack comment on relevant policies but instead focus on specific aspects of individual technical pathways. As an exception, the search included scientific papers that explicitly cover policy aspects. In order to reflect the most current policy developments, only reports that were published between 2010 and 2020 were selected. With regard to the spatial references of the relevant literature, such as the European Union or the United States, which are relevant for policy making, we did not limit the eligibility, reflecting our aim of including as many potential policy options as possible1.
Using these criteria, we conducted a Google search of the most common designations for CCU technologies [CCU; carbon capture and utili [s/z]ation; CCUS; carbon capture, utili [s/z]ation and storage; CDR; carbon dioxide recycling; CDU; carbon dioxide reuse; CO2 utilization] in combination with the more specific keywords [mineralization; carbonation; policy; legislation] and screened the results for relevant matches. The same search protocol was used in Google Scholar to identify relevant scientific papers. Overall, this first search identified 20 policy papers and one scientific paper as relevant. To verify the search results and add additional value to the sample, a second researcher conducted an additional independent search using the same parameters. This provided another five policy reports2. The final overall sample of 26 publications does not claim completeness; nevertheless, the two independent scanning processes and the resulting sample provide a sufficient degree of comprehensiveness for the purposes of this paper (for a separate list, please see additional materials).
Online Expert Survey
Additionally, we conducted an online survey of experts in the field of CO2 mineralization, concerning their opinions on relevant policy measures. The survey formed part of a larger, multi-criteria decision analysis that is currently under review3.The survey addressed key stakeholders from the established industry (e.g., cement producers and other established industry, start-ups, academia and non-governmental organizations (NGO), who are active in the field in various ways. The surveyed experts were first identified from the IASS contact database, which has been created over several years in context of dialogue events on CO2 utilization technologies and therefore includes a large range of experts and involved stakeholders in the field. In a second step, the contacts compiled in this way were supplemented with further possible participants by the members of the CO2MIN4 project consortium. A pretest was conducted with three experts from the consortium in order to ensure the comprehensibility and functionality of the survey Consequently, 105 experts were invited to take part in the survey. A final sample of 19 experts from different stakeholder group affiliations5 completed the survey between November 2019 and February 2020.
In the survey, the participants were asked to prioritize given policy measures in terms of fostering CO2 carbonation technologies in the construction sector. The research methodology employed the analytical hierarchy process (AHP) (Saaty, 1980; Saaty, 2008; Munda, 2016; De Montis et al., 2005; Diakoulaki et al., 2005). AHP is a well-established method that asks participants to compare different options, in this case different policy measures, in a pair-wise manner. As a result, priority scales for decision-making processes, in this case with regard to the relevance of policies, are derived. The importance of each policy is displayed as a percentage, with the overall sum of all factors being 100% (Saaty, 1980).
Classification of Relevant Policies
With regard to CO2 mineralization, just as for other technology innovations, supporting policies can be allocated in three functional areas, namely support for early development; incentives; and guidance for deployment and market regulation (Castillo-Castillo and Angelis-Dimakis, 2017). Within these functional areas, governments and funding agencies can address technology innovation through different approaches that, according to their objectives, can be differentiated as “technology-push” or “market-pull” (Horbach et al., 2012). Technology-push policies are measures that stimulate technological development by fostering research, demonstration projects, and deployment via funding and/or other incentives for early development (Hendriks et al., 2013). In contrast, market-pull policies aim to create or increase market demand for a product or service. Market-pull policies include market-based instruments (MBIs) and command-and-control (CAC) measures. MBIs encourage innovation through market signals by providing economic incentives (Ecorys, 2011; European Commission, 2009; Hendriks et al., 2013). Such policy options are manifold and comprise instruments based on price and quantity as well as information-based measures. Examples of price-based instruments include tax credits or subsidies, while quota- or carbon-trading schemes, such as the European Emissions Trading System (ETS), are quantity-driven measures. Informational policy tools refer to the disclosure of certain information and can include product labelling or voluntary certification systems (Ecorys 2011). CAC policies, or regulatory instruments, are orders backed by the force of law, whereby non-compliance is penalized. Public authorities may mandate a certain performance to be achieved or technologies to be used. Standard settings or banning products from the market are examples of such policies (Stavins, 2000; Hendriks et al., 2013).
In the following section, policies that are considered relevant for CO2 mineralization technologies in the selected materials are identified and grouped according to the scheme derived above (see Figure 2) in order to foster a better understanding of at which end policies are considered helpful for implementing CO2 mineralization technologies and accelerating their market entry.
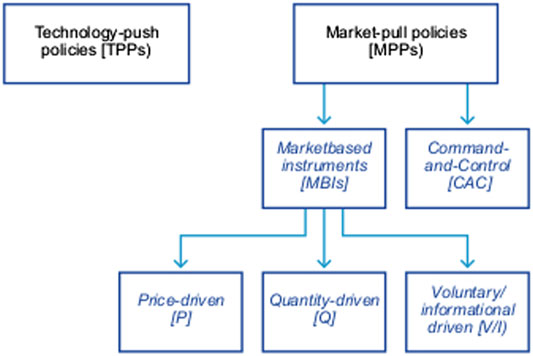
FIGURE 2. Classification of policies (e.g., Ecorys, 2011; Groba and Breitschopf, 2013; Hendriks et al., 2013).
Results
Before describing the overall outcomes of the analysis, it must be mentioned that the literature analysis revealed that most policies address CCU technologies as a whole and cannot be separated from relevant policies for CO2 mineralization. Only a few of the literature sources include specific measures to support CO2 mineralization technologies. Therefore, the first part of this section reports the identified policy measures that potentially represent barriers or incentives for the development and deployment of CCU technologies in general, referring to aspects of CO2 mineralization whenever applicable. This is followed by discussion of specific policy measures with relevance to CO2 mineralization only. Subsequently, the results of the online survey are discussed.
Literature Analysis
Technology-Push Policies
The analyzed literature reflects the usefulness and necessity of technology-push measures. Sixteen of the 26 reports on CCU technologies (see Table 1 for an overview of all policy measures in the literature) recommend increased funding of research and development, thereby making this the highest-ranking technology-push policy (e.g., Group of Chief Scientific Advisors, 2018; Sandalow et al., 2017). Eleven reports emphasize the need for more funding for upscaling and during market implementation of CCU technologies (e.g., CarbonNext, 2018; IOGP, 2019). While the literature frequently calls for additional funding, states and nations already support CCU technologies during different development phases. In the European Union, the main push instruments are the EU framework programs, such as the previous Framework Programme seven FP7 (European Commission, n d-a), the current Horizon 2020 program (European Commission, n d-b), and the upcoming Horizon Europe program (European Commission, n d-c), as well as funding made available within the Green Deal (European Commission, 2019). A total of 61 projects on CCU technologies were funded during the period 2008–2018 under FP7 and Horizon 2020 for a total of €243 million (Group of Chief Scientific Advisors, 2018). The new ETS Innovation Fund explicitly covers CCU technologies, making further funding available until 2030 in the European Union. In addition to European Commission funding, some European Union member states also have their own national funding programs, such as Germany’s funding measures “CO2MIN” (Jülich, 2017) and “CO2WIN” (BMBF, 2018). As further technology push options, ten publications emphasize the need for improvements to enabling infrastructures, including CO2 and hydrogen (e.g., (Zero Emissions Platform, 2017; CCUS Taskforce, 2018). Technology prizes are mentioned three times as a policy measure to push CCU technologies (e.g.,IEA, 2019) Such prizes already exist, such as the European Horizon Prize for CO2 Reuse (European Commission, n d-d). which awards the winner 1.5 million euros, and the United States American X-Prize Carbon, awarding 20 million United States dollars (X-Prize, n d).
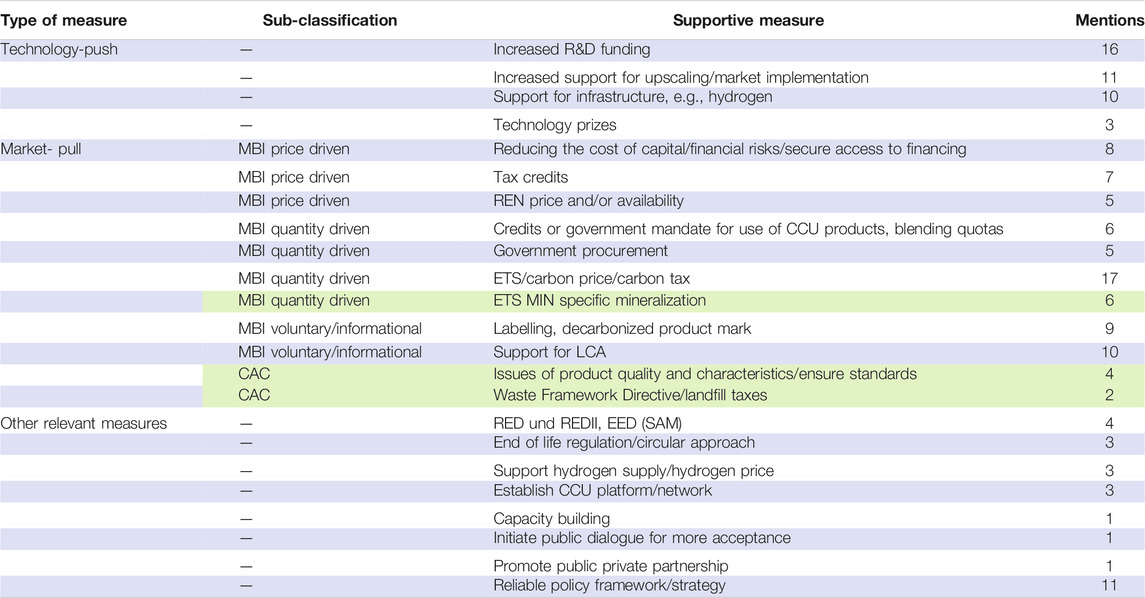
TABLE 1. Overview of policy measures in the analyzed reports. Green background: Policy measures that apply only to CO2-mineralization.
Thus, the main eligible technology-push policies for CCU technologies, i.e., funding programs, are already in place at present and will also be available in the foreseeable future. Therefore, they can be considered as an important incentive for the further development of CCU technologies including CO2 mineralization. Nevertheless, for successful rollout of the available technologies, the analyzed literature considers the amount of available funding in all development phases as still inadequate or not sufficiently focused (Hendriks et al., 2013). Furthermore, barriers to funding applications may be considerable for small companies or start-ups, and thus limit their access to funding (Zimmermann and Kant, 2016). This is particularly relevant for CO2 mineralization projects, since the development of mineralized construction materials utilizing CO2 presently seems to be of interest to start-ups (Zimmermann and Kant, 2016) additional to the interest from major companies such as HeidelbergCement or Lafarge. Taking this into account, all mentioned technology-push policy options are generally valid for CO2 mineralization technologies as much as for all other CCU applications. The analyzed literature does not consider any specific relevant technology-push policies with regard to mineralization.
Market-Pull Policies
Market-pull policies aim to create or increase market demand for a product or service, either through market-based instruments (MBIs) or command-and-control (CAC) measures.
Price-Driven MBIs
Among the market-based instruments, the analyzed literature considers price-driven policies as most relevant. Eight reports propose reducing the cost of capital or the financial risks, or else securing access to financing (e.g., Bobeck et al., 2019; International Chamber of Commerce (ICC), 2016). Seven reports support tax credits as a means of enhancing the market implementation of products made with CO2 (e.g., Styring et al., 2011; Coddington 2016). Five reports recommend ensuring the availability of renewable energy at competitive rates (e.g., Wilson et al., 2016; Whiriskey and Wolthuys, 2016), At present, no specific price-driven MBIs can be identified for CO2 mineralization technologies in the analyzed literature. For all CCU products, price-driven policy measures might prove particularly relevant, since the lack of economic competitiveness in CCU products is considered an obstacle to their rollout (Olfe-Kräutlein et al., 2016).
Quantity-Driven MBIs
Quantity-driven policy instruments are also considered influential for the market implementation of CCU technologies. Government mandates or credits for the use of CO2 based products (e.g., IEA, 2019; ICEF, CO2 Sciences and The Global CO2 Initiative, 2016) including blending quotas (Turnau et al., 2019) as well as government procurement (e.g., CarbonNext, 2018; Sandalow et al., 2017) are recommended six times and five times respectively in the analysed reports. Product-blending quotas (i.e., mandatory use of a certain amount of CCU products or low-emission products in a certain application) could be useful in helping CCU products penetrate conventional markets, creating investment certainty by providing assured demand for those products, and thus facilitate technological development and scaling-up and making CCU products more competitive (Turnau et al., 2019). Since public procurement accounts for about 16% of GDP across the European Union (European Commission, 2009), government procurement can play a decisive role for CCU products, including CO2 mineralization technologies, by initiating and building new product markets and by providing early market demand for emerging technologies (ICEF, CO2 Sciences and The Global CO2 Initiative, 2016; Sandalow et al., 2017). CO2-cured concrete and CO2-based aggregates are examples of products which may be included in government procurement guidelines for construction projects. While quantity-driven MBIs have already been implemented within the European Union’s instrument on Green Public Procurement (GPP) with the aim of facilitating eco-innovation processes (Day, 2005; European Commission, 2009), products made with CO2 are not currently eligible for any such scheme. This may be due to their early development in some cases, but also due to a lack of reliable knowledge about their ecological implications, and what types of ecological and societal benefits they might involve (if any). In contrast, an early example of an upcoming quantity-driven MBI with relevance for CO2 mineralization is the Buy Clean California Act (BCCA) in the United States (Department of General Services, 2020), which aims to create a market for construction materials (including concrete) with a low CO2 footprint, which might become applicable to CCU products.
CO2 Emission Trading Schemes as Quantity-Driven MBIs
The role that emission trading schemes, specifically the European ETS, may play for the market implementation of CCU technologies has not yet been conclusively clarified. For one thing, it is assumed that a high CO2 price is beneficial for the economic attractiveness of CCU technologies, since carbon pricing can incentivize the capture of CO2 and its use, or sell it for use in the manufacture of products, given this may be a cost-effective compliance strategy for emitters (IEA, 2019). Also relevant is the possibility of direct recognition as a CO2 emission mitigation tool under such schemes. Currently, in the EU-ETS Monitoring and Reporting Regulation (EC/601/2012, MRR), captured CO₂ that is used in products must be reported and thus excludes CCU technologies from being categorized as emission mitigation tools.
The assessment of policy reports shows the importance attached to such schemes. Of the 26 analyzed reports, 17 point to the relevance of the future inclusion of CCU technologies in the EU-ETS. Only one report generally opposes such inclusion (Whiriskey and Wolthuys, 2016) Several reports call directly for some sort of recognition (Hendriks et al., 2013; CO2 Value Europe, n d), while others at least recommend examining such options (e.g., (SCOT project, 2016; Turnau et al., 2019). However, it is evident that application-specific assessment of any resulting emissions is essential (e.g., CarbonNext 2018).
With regard to the exclusion of CO2 mineralization applications in the European ETS, some of the most recent reports reference the Schaefer Kalk ruling (Group of Chief Scientific Advisors, 2018; Turnau et al., 2019; CO2 Value Europe, n d). Following the European Court of Justice’s preliminary ruling in the Schaefer Kalk case in 2017, the ETS must acknowledge emission reduction through producing calcium carbonates via CO2 mineralization (IOGP, 2019). The legal precedent set by this ruling may pave the way for other CO2 mineralization technologies to be recognized in the EU-ETS or in other emission trading systems, accounting for their ability to permanently store CO2 (Whiriskey and Wolthuys, 2016). Overall, six reports consider the recognition of mineralization technologies in the ETS as specifically relevant (e.g., Whiriskey and Wolthuys, 2016; Wilson et al., 2016; Group of Chief Scientific Advisors, 2018).
Voluntary and Informational MBIs
Voluntary and informational MBIs imply, for example, the disclosure of certain product information, and are expressed through product labelling or voluntary certification systems (Ecorys, 2011). Labels indicating the environmental characteristics or other beneficial aspects of products can help to create or increase demand for such products. In the analyzed literature, nine out of 26 reports consider the introduction of a label for products made with CO2 as beneficial (e.g., IOGP, 2019; Bobeck et al., 2019), Strategies to implement a labelling system for CCU products could either intend to indicate the presence of captured CO2 in a product or to modify existing labelling schemes, with the aim of giving credit for the use of CO2 in a way that reduces a product’s life cycle emissions. Difficulties in labelling CCU products lay, for example, in the diversity of possible products or in differences between intermediate versus end-products (Sandalow et al., 2017; Olfe-Kräutlein et al., 2016; Turnau et al., 2019). As a second policy option to foster voluntary informational MBIs, ten reports recommend supporting the development of standardized and transparent assessment methods, such as life cycle assessments (LCA), with a specific focus and applicability to CCU technologies (e.g., Coddington, 2016; Wilson et al., 2016).
Command and Control Instruments
Command and control instruments (CAC) are regulatory orders backed by the force of law, such as emission or product standards. In contrast to the MBIs described above, CACs are prescriptive in nature. Many different products that may be produced using CCU technologies will be subject to different specific CACs. For CO2 mineralization products, CACs are of particular importance, since construction materials must typically meet composition-based and performance-based codes and standards for use (Deolalkar et al., 2015; European Standard, 2020; Müller, 2012) Such codes and standards may inhibit or delay adoption of novel cement or concrete products, as some will not fall under current composition-based specifications when new feedstocks are used (e.g., carbonated virgin minerals), even when they meet performance requirements (Deolalkar et al., 2015; Sandalow et al., 2017). A change to a system of harmonized performance-based standards might accelerate the adoption and acceptance of new, CCU-derived construction materials (Sandalow et al., 2017; McNutt, 2015).
Reflecting this, four of the analyzed reports support the use of testing and certification procedures in order to quickly ensure that new products made with CO2 meet the required standards (e.g., Sandalow et al., 2017; IEA, 2019). With particular reference to CO2 mineralization processes, four reports highlight the need to address issues of product quality and characteristics, and to ensure that new products meet the relevant standards (e.g., GCCSI, 2011; Sandalow et al., 2017).
Policies Specific to Waste-Based Building Materials
Most of the policy measures evaluated in the literature sample are relevant for CCU technologies in general, which includes CO2 mineralization; and some reports add or highlight policies that are of specific relevance to CO2 mineralization. Additionally, the reports consider two other policy measures as specifically relevant for waste-based building materials made with CO2, namely the EU Waste Framework Directive (WFD) and landfill taxes (Turnau et al., 2019; Castillo-Castillo and Angelis-Dimakis, 2017).
The Waste Framework Directive (2008/98/EC, WFD) is an EU CAC instrument. It is particularly important and relevant for waste-based CO2 mineralization routes, since (in the United Kingdom, for example) waste streams are already used as feedstock for manufacturing building materials (Carbon8, 2020) and thus, without modification, the WFD is currently obstructing the market adoption of such aggregates made with products from waste incineration (Turnau et al., 2019; Castillo-Castillo and Angelis-Dimakis, 2017).
The reduction of landfill is an important policy target in many regions (e.g., (European Environment Agency, 2000). Landfilling is therefore subject to taxation in several countries within and beyond the EU, for example Denmark (DIS, n d) Finland (Sustainable Development Goals Knowledge Platform, n d), and Australia (NSW Environment Protection Authority, 2020) The development of waste-based materials made with CO2 may benefit from such taxes, since they will incur comparatively lower costs once previously landfilled waste is instead diverted to mineralization processes. Their scale-up might even provide opportunities to entirely phase out the landfilling of certain types of solid wastes, such as steel-sector fines, bauxite, quarry fines, wood and paper ashes, or metal dust, and stabilizing their potentially hazardous elements (SCOT project, 2016).
Additional Recommendations
The analyzed reports also mention several other policy measures with indirect effects on the development of CCU technologies. For example, four reports refer to the importance of energy-related policies such as the EU RED (Renewable Energy Directive) and EED (Energy Efficiency Directive) (Group of Chief Scientific Advisors, 2018; CarbonNext, 2018; Turnau et al., 2019), since the environmental benefits of CCU technologies very often rely on the abundant supply of energy from renewable sources. Also, the further advancement of political measures to foster a circular economy are considered helpful, under the condition that the potential role of CCU technologies in circular approaches is clarified (Club CO2, n d; Wilson et al., 2016; Schlögl et al., 2018). Since hydrogen is used in some CCU applications such as the production of synthetic fuels, support for greater production of hydrogen from renewable sources and the expansion of the required infrastructure is also mentioned as supportive for CO2 utilization technologies (ICEF, CO2 Sciences and The Global CO2 Initiative, 2016; Wilson et al., 2016; CarbonNext, 2018). This has no relevance to mineralization processes with CO2.
Furthermore, several reports recommend establishing a CCU platform to enhance exchange among experts (Styring et al., 2011; Wilson et al., 2016; Schlögl et al., 2018), to support educational efforts in terms of capacity building (Wilson et al., 2016) and to foster and initiate a public dialogue to enhance the acceptance of CO2 utilization (International Chamber of Commerce (ICC), 2016).
Expert Survey Results
The second stage of this study surveyed the opinions of experts in the field of CO2 mineralization on the issue of market-based policy instruments derived from the results of the literature analysis. The online survey included 19 key European stakeholders from the established industry, start-ups, academia, and non-governmental organizations (NGOs)6.
To determine which policies measures are considered particularly relevant, survey participants were asked to prioritize the importance of: 1) emission trading systems7 (as the most important MBI in the literature; see Market-Pull Policies section. b), 2) product labelling and standardization issues as the visible outcomes of standardized assessments (as required in the literature; see Market-Pull Policies section. d), 3) the EU Waste Framework Directive as an important policy measure with regard to mineralization products that are already available in some countries (Carbon8, 2020) (see Market-Pull Policies section. f), and 4) and 5) government procurement and blending quotas as policy measures that most directly target market implementation (see Market-Pull Policies section. b).
The survey results show that CCU experts rank governmental procurement rules for sustainable building material as highly relevant for the market implementation of CO2 mineralization products, followed by a set product-blending quota for low-emission building materials as well as adaptation or price changes in the EU-ETS system. Although the survey responses were relatively uniform across all policy options, the Waste Framework Directive and product labelling and standardization options were rated as slightly less important (see Figure 3).
Clustering the results according to stakeholder group affiliation, the picture is more distinct (see Figure 4). Experts from academia and established industry regard the EU-ETS as the major driver for implementation of CO2 mineralization technologies. In contrast, those affiliated with NGOs and start-ups expect governmental procurement measures to be the most effective lever. Additionally, it becomes clear that NGOs foresee the most effective action as involving product-blending quotas and governmental procurement measures (combined priority score of 85%), while established industry favours almost an equal mixture of all presented measures to foster change.
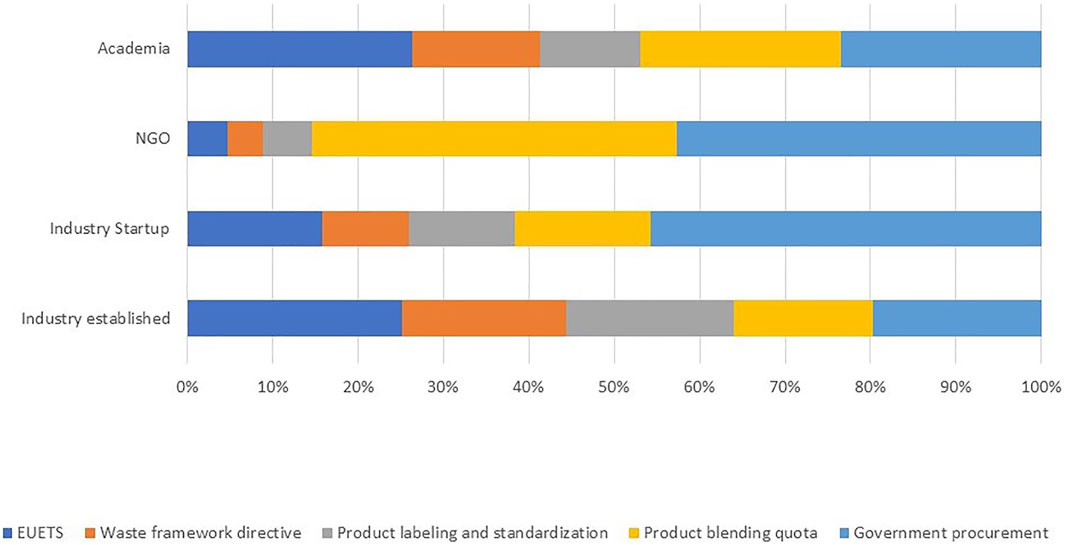
FIGURE 4. Prioritization of policy measures: group responses (n = 19, seven academia, two NGO, five industry start-ups, five industry established).
Survey participants assigned slightly different weightings to the given policy measures compared with the original authors of the reviewed policy reports. Whereas both cases assign similar relevance to emission trading schemes, measures such as government procurement and blending quotas are ranking higher among the interviewed experts. This finding hints to such measures being considered desirable, with immediate positive effects on market demand for products made with CO2, rather than counting on the indirect or long-term effects of other policy tools.
Actionable Recommendations
The policies identified from the literature review were categorized as technology-push or market-pull. In the literature, the relevance of technology-push policies is evident. However, the literature tends to focus on just a few instruments. Although several such instruments are already in place, the references point to continued demand for measures to support research and development. With regard to market-pull policies, the literature shows a broad variety of potentially influential measures, but with a clear focus on emission trading schemes and the acknowledged necessity for support in developing reliable tools for assessing emissions throughout the entire life cycle. The experts’ expressed priorities underline this, but additionally point to the expected relevance of government procurement and blending quotas.
Technology-pull policies already offer support for CCU technologies and can mostly be considered as incentives—bearing in mind an expressed need for an increase in volume and focus. Market-pull policies are considered to have major potential, for example to overcome the hurdle of higher costs involved with CCU, but they mostly need to be either installed or amended in order to provide incentives, rather than presenting barriers to the further development and deployment of CCU technologies. This finding points to the need for a change of perspective in policy making: While there is continued need for technology-push support in the early development phases, the broad spectrum of required market-pull policies shows that policy support measures must have a movable focus in order to better address the evolving technology readiness levels (TRL) of CCU technologies, including CO2 mineralization, and their upcoming market entry.
Thus, in order to support CO2 utilization technologies in general, including CO2 mineralization, in reaching markets and unfolding their full sustainable potential, it is recommended to design policies:
1) on the existing basis of applicable push and pull policies, which need to be amended to fully cover CCU technologies;
2) in continuation of the technology-push policies already in place, further extending them to cover CCU applications with higher TRLs,
3) providing reliable long-term policy frameworks that give industry actors confidence in deciding on CO2 utilization pathways,
4) with a shifting focus towards a market-oriented policy mix, helping tackle barriers to market entry and implementation.
Concerning CO2 mineralization, the relevant specific frameworks and regulations need to be addressed and adapted in the same ways as measures intended to support the transition to a decarbonized industry sector.
Discussion
This study aims to contribute to a better understanding of why—despite proven technological feasibility—few CO2 mineralization applications yet reached the commercialization stage. The methodology analyzed the policy conditions deemed favorable to supporting the development and implementation of CCU technologies: as described in 26 policy reports from the recent literature; and also as expressed in an online survey of expert stakeholders, examining the perceived influences of technology-push and market-pull policy measures.
The review of the policy literature indicates that numerous policy measures are regarded as currently or potentially influential on the further development and deployment of CCU technologies, including CO2 mineralization technologies. In some cases, policies are considered as more relevant or only applicable to (certain) CO2 mineralization applications. With regard to CO2 mineralization, overall, the analysis reveals that, for the time being, such processes and products face significant regulatory barriers and a lack of policy support, thereby hindering or limiting their scale-up and market entry. This may help explain why—despite their proven technical feasibility—most of these technologies are yet to be commercialized. Conversely, various existing policy options already have the potential to incentivize the deployment of CO2 mineralization technologies, on condition of some amendment or clarification in their scope and application. In that sense, this study provides an overview of suitable measures available to policy-making in the pursuit of fostering the sustainability potential of CO2 mineralization technologies.
The need to act in terms of policy making is not only shown by the many relevant policies that have potential to support the further development and implementation of CO2 mineralization technologies. While the increasing technical feasibility of many mineralization pathways during the last decade can be regarded as a result of existing support for early development in the field by technology-push policies, future measures now need to additionally address their upscaling and market implementation, and do so in a reliable and continuous manner while assessing their potential benefits for environment and society. The necessity of a reliable policy framework as a basis for the development of CCU innovations is expressed explicitly in eleven of the analyzed policy reports. Current political strategies and other initiatives (European Commission, 2020, 2019; Cembureau, 2020a; FAZ.NET, 2021) have already set the agenda accordingly providing the underlying conditions. As most recent development in the European Union, the “Fit for 55 - package”8, announced in July 2021, outlined new options, for example the recognition of CO2 mineralization processes in the ETS (CO2 Value Europe, 2021), which might offer new incentives for the implementation of CCU technologies after its final adoption and is thus mapping out a promising pathway for the future.
Overview of the Analyzed Policy Reports
1. Acatech. 2018. CCU und CCS—Bausteine für den Klimaschutz in der Industrie Berlin.
2. Bobeck, Jeffrey; Peace, Janet; Ahmad, Fatima Maria. 2019. Carbon Utilization: A Vital and Effective Pathway for Decarbonization. edited by Center for Climate and Energy Solutions, Arlington.
3. CarbonNext. 2018. Methodology to assess the business case and economic potential of CCU.
4. Castillo-Castillo, Arturo; Angelis-Dimakis, Athanasios. 2017. “Policy analysis and recommendations for EU CO2 utilisation policies.” 15th International Conference on Environmental Science and Technology, Rhodes, Greece.
5. CCUS Taskforce. 2018. Delivering Clean Growth: CCUS Cost Challenge Taskforce Report. edited by Energy and Industrial Strategy United Kingdom Department for Business, London.
6. Club CO2. n. d. “Club CO2: Carbon Capture, Use and Storage (CCUS)." accessed January 15, 2020. http://www.captage-stockage-valorisation-co2.fr/en/home.
7. CO2 Value Europe. n. d. “CCU and the ETS.” https://www.co2value.eu/wp-content/uploads/2020/06/CVE-paper-on-CCU-in-ETS-Recommendations-for-the-revision-of-the-Monitoring-and-Reporting-Regulation-MRR.pdf.
8. Coddington, Kipp. 2016. CO2 Building Blocks. Assessing the CO2 Utilization Options. edited by National Coal Council, Washington.
9. Delft, CE. 2017. CCU market options in the Rotterdam Harbour Industrial Complex. Delft CE Delft.
10. GCCSI. 2011. Accelerating the Uptake of CCS: Industrial Use of Captured Carbon Dioxide. edited by GLobal CCS Institute.
11. Group of Chief Scientific Advisors. 2018. Novel Carbon Capture and Utilisation Technologies (Scientific Opinion). Brussels: European Commission, Directorate-General for Research and Innovation.
12. Hendriks, Chris; Paul Noothout; Paul Zakkour; Greg Cook. 2013. Implications of the Reuse of Captured CO2 for European Climate Action Policies. Utrecht: ECOFYS Netherlands B.V.
13. ICEF, CO2 Sciences and The Global CO2 Initiative. 2016. Global Roadmap for Implementing CO2 Utilization, Ann Arbor.
14. IEA. 2019. Putting CO2 to Use, IEA, Paris.
15. International Chamber of Commerce (ICC). 2016. Role of carbon and long-term mitigation strategies beyond 2050 through carbon capture and use (CCU) and closing the carbon cycles (C³), ICC, Paris.
16. IOGP. 2019. The potential for CCS and CCU in Europe. Report to the 32nd meeting of the European Gas Regulatory Forum, IOGP, Brussels.
17. Olfe-Kräutlein, Barbara, Henriette Naims, Thomas Bruhn, and Ana Maria Lorente Lafuente. 2016. C O 2 as an Asset - Potentials and Challenges for Society. Potsdam IASS.
18. Sandalow, David;, Roger Aines; , Julio Friedmann; , Colin McCormick; , and Sean McCoy . 2017. Carbon Dioxide Utilization (CO2U). Edited by ICEF.
19. Schlögl, Robert, Carlos Abanades, Michele Aresta, Adisa Azapagic, Edd Anders Blekkan, Thibault Cantat, Gabriele Centi, Neven Duic, Aïcha El Khamlichi, and Graham Hutchings. 2018. “Novel carbon capture and utilisation technologies: Research and climate aspects." SAPEA, Brussels.
20. SCOT project. 2016. EU_ETS to incentivise CO2 utilisation? SCOT Project Briefing Paper, SCOT project, Brussels.
21. Styring, Peter, Daan Jansen, Heleen de Coninck, Hans Reith, and Katy Armstrong. 2011. Carbon Capture and Utilization in the green economy.
22. Turnau, Sebastian; Diederik Jaspers; Annika Marxen; Henriette Naims; Sander de Bruyn; Stefan Bringezu; Simon Kaiser; Ada Gimnes Jarøy; Samy Porteron; Ingvild Ombudstvedt; Maria Ellingsen Gran; Xavier Le Den; Ferdinand Zotz; Barbara Olfe-Kräutlein; Emiel de Bruijne. Emiel. 2019. Identification and analysis of promising carbon capture and utilisation technologies, including their regulatory aspects. European Commission.
23. Whiriskey, K. W. J. W. Wolthuys 2016. CCU in the EU ETS: Risk of CO2 laundering preventing a permanent CO2 solution. Edited by Bellona Europa.
24. Wilson, Grant, Youssef Travaly, Talia Brun, Hans Knippels, Katy Armstrong, Peter Styring, Dennis Krämer, Géraldine Saussez, and Hans Bolscher. 2016. “A VISION for Smart CO2 Transformation in Europe: Using CO2 as a resource."
25. Zero Emissions Platform. 2013. CO2 Capture and Use (CCU) - The potential to reduce CO2 emissions and accelerate CCS deployment in Europe, Zero Emissions Platform, Brussels.
26. Zimmermann, Arno, and Marvin Kant. 2016. “The Business Side of Innovative CO2 Utilisation.” EIT Climate KIC/TU Berlin. http://enco2re.climate-kic.org/wp-content/uploads/2016/01/The-business-side-of-innovative-CO2-utilisation.pdf.
Author Contributions
BO-K is responsible for research and data analysis. TS covered technical parts and contributed to the overall article. AC conducted research and data analysis while at the IASS.
Funding
The research for this study was funded by the German Federal Ministry of Research and Education (BMBF) in the funding scheme CO2MIN.
Conflict of Interest
The authors declare that the research was conducted in the absence of any commercial or financial relationships that could be construed as a potential conflict of interest.
Publisher’s Note
All claims expressed in this article are solely those of the authors and do not necessarily represent those of their affiliated organizations, or those of the publisher, the editors and the reviewers. Any product that may be evaluated in this article, or claim that may be made by its manufacturer, is not guaranteed or endorsed by the publisher.
Footnotes
1The methodology acknowledges that this paper does not aim to fully assess the current policy situation in a specific region, which would require locational constraints and associated filtering of the relevant literature
2The first literature research was conducted between November 2019 and January 2020, the additional search was undertaken in april 2020
3Till Strunge, Henriette Naims, Hesam Ostovari, Barbara Olfe-Kräutlein n.d. “Priorities for supporting emission reduction technologies in the cement sector—A multi-criteria decision analysis of CO2 mineralisation” submitted to Journal of Cleaner Production on June 7th, 2021
4The project “CO2MIN” (033RC014) investigated the sequestration of CO2 with simultaneous production of marketable products within the cement industry. The project ran from June 2017 until December 2020 and was funded by the German Federal Ministry of Education and Research (BMBF).
5Distribution of stakeholder groups in the sample: academia 7, industry established 7, industry start-up 7, NGO 2
6The online survey on policies for CO2 mineralization was undertaken as part of a larger survey of CO2 mineralization technologies, which is currently under review: Till Strunge, Henriette Naims, Hesam Ostovari, Barbara Olfe-Kräutlein n.d. “Priorities for supporting emission reduction technologies in the cement sector—A multi-criteria decision analysis of CO2 mineralisation” submitted to Journal of Cleaner Production on June 7th, 2021
7Since only experts from the European Union were invited to participate in the study, the “emission trading” category was specified as the EU-ETS.
8EU economy and society to meet climate ambitions (europa.eu), accessed on 20 August 2021
References
Aresta, M., and Dibenedetto, A. (2010). “Industrial Utilization of Carbon Dioxide (CO2),” in Developments and Innovation in Carbon Dioxide (CO2) Capture and Storage Technology: Volume 2: Carbon Dixide (CO2) Storage and Utilisation. Editor M. Mercedes Maroto-Valer (Great Abington: Woodhead Publishing), 377–410. doi:10.1533/9781845699581.4.377
Beumelburg, C. (2020). HeidelbergCement Presents New Strategy "Beyond 2020" and Pushes Climate Goals. Available at: https://www.heidelbergcement.com/en/pr-16-09-2020 (accessed January).
BMBF (2018). CO2 als nachhaltige Kohlenstoffquelle – Wege zur industriellen Nutzung (CO2-WIN). Available at: https://www.fona.de/de/massnahmen/foerdermassnahmen/co2-als-nachhaltige-kohlenstoffquelle.php (accessed July, , 2021).
Bobeck, J., Peace, J., and Ahmad, F. M. (2019). Carbon Utilization: A Vital and Effective Pathway for Decarbonization. Arlington: Center for Climate and Energy Solutions.
Carbon8 (2020). Thinking Differently. Available at: https://c8s.co.uk (accessed January).
CarbonNext (2018). Methodology to Assess the Business Case and Economic Potential of CCU. Brussels: CarbonNext.
Castillo-Castillo, A., and Angelis-Dimakis, A. (2017). “Policy Analysis and Recommendations for EU CO2 Utilisation Policies,” in 15th International Conference on Environmental Science and Technology, Rhodes, Greece, 31st August - 2nd September 2017 .
CCUS Taskforce (2018). Delivering Clean Growth: CCUS Cost Challenge Taskforce Report. London: Energy and Industrial Strategy UK Department for Business.
Cembureau (2020b). Circular Economy. Available at: https://cembureau.eu/policy-focus/environment/circular-economy/(accessed January).
Club CO2 (). Club CO2: Carbon Capture, Use and Storage (CCUS). Available at: http://www.captage-stockage-valorisation-co2.fr/en/home (accessed August 16, 2019).
CO2 Value Europe (). CCU and the ETS. Available at: https://www.co2value.eu/wp-content/uploads/2020/06/CVE-paper-on-CCU-in-ETS-Recommendations-for-the-revision-of-the-Monitoring-and-Reporting-Regulation-MRR.pdf (Accessed June 2021).
CO2 Value Europe (2021). Fit-for-55 Package: Where and How Carbon Capture and Utilisation Will Contribute to Reducing CO2 Emissions in Europe. (co2value.eu). Available at: https://www.co2value.eu/wp-content/uploads/2020/06/CVE-paper-on-CCU-in-ETS-Recommendations-for-the-revision-of-the-Monitoring-and-Reporting-Regulation-MRR.pdf (Accessed August 20, 2021).
Coddington, K. (2016). “CO2 Building Blocks,” in Assessing the CO2 Utilization Options (Washington: National Coal Council).
Day, C. (2005). Buying green: the Crucial Role of Public Authorities. Local Environ. 10 (2), 201–209. doi:10.1080/1354983042000388214
De Montis, A., De Toro, P., Droste-Franke, B., Omann, I., and Stagl, S. (2005). “Assessing the Quality of Different MCDA Methods,” in Alternatives for Environmental Valuation. Editors G Michael, S. L Clive, and S Sigrid (Milton Park: Routledge), 99–133.
Deolalkar, S. P., Shah, A., and Davergave, N. (2015). Designing green Cement Plants. Oxford: Butterworth-Heinemann.
Department of General Services (2020). Buy Clean California Act. Available at: https://www.dgs.ca.gov/PD/Resources/Page-Content/Procurement-Division-Resources-List-Folder/Buy-Clean-California-Act (accessed January, 2021).
Diakoulaki, D., Antunes, C. H., and Gomes, M. A. (2005). “MCDA and Energy Planning,” in Multiple Criteria Decision Analysis: State of the Art Surveys. Editors J Figueira, S Greco, and M Ehrogott (New York, NY: Springer), 859–890.
DIS (). Denmark's Landfill Tax. EU-27 Green Tax Wiki. Available at: https://canvas.disabroad.org/courses/4391/pages/denmarks-landfill-tax#:∼:text=Results%20of%20the%20landfill%20tax,measures%20to%20incentivize%20recycling%20waste (accessed January, 2021).
Ecorys (2011). “The Role of Market-Based Instruments in Achieving a Resource Efficient Economy,” in Report for the European Commission, DG Environment (Rotterdam: Ecorys, Cambridge Econometrics and COWI).
European Circular Economy Stakeholder Platform (2018). Circular Cement: Processing Waste to Create Cement in a Circular Economy. Available at: https://circulareconomy.europa.eu/platform/en/good-practices/circular-cement-processing-waste-create-cement-circular-economy (accessed January).
European Commission (). 7th Framework Programme. Available at: https://ec.europa.eu/research/fp7/index_en.cfm (accessed February).
European Commission (2020). Circular Economy Action Plan. Available at: https://ec.europa.eu/environment/strategy/circular-economy-action-plan_de (accessed July, 2021).
European Commission (). Horizon 2020. Available at: https://ec.europa.eu/programmes/horizon2020/en (accessed February).
European Commission (). Horizon Europe. Research and Innovation Funding Programme until 2027. How to Get Funding, Programme Structure, Missions, European Partnerships, News and Events. Available at: https://ec.europa.eu/info/research-and-innovation/funding/funding-opportunities/funding-programmes-and-open-calls/horizon-europe_en (accessed July, 2021).
European Commission (). Horizon Prize for CO2 Reuse. Available at: https://www.h2020.md/en/horizon-prize-co2-reuse (accessed February, 2021).
European Commission (2019). The European Green Deal. Available at: https://ec.europa.eu/info/strategy/priorities-2019-2024/european-green-deal_en (accessed July, 2021).
European Commission (2009). The Potential of Market Pull Instruments for Promoting Innovation in Environmental Characteristics Brussels. Brussels: Directorate General Environment.
European Environment Agency (2000). Environmental Assessment Report No 6. Available at: https://www.eea.europa.eu/publications/92-9167-205-X/page012.html (accessed January, 2021).
European Standard (2020). EN 197-1:2000 Cement. Composition, Specification and Conformity Driteria for Common Cements. London: British Standards Institute.
FAZ.NET (2021). CO2-Bindung: Für diese Technologie will Elon Musk einen Millionenpreis vergeben. Available at: https://www.faz.net/aktuell/wirtschaft/co2-bindung-elon-musik-vergibt-preis-fuer-diese-technologie-17159260.html (accessed January, 2021).
GCCSI (2011). Accelerating the Uptake of CCS: Industrial Use of Captured Carbon Dioxide. Melbourne: GLobal CCS Institute.
Groba, F., and Breitschopf, B. (2013). Impact of Renewable Energy Policy and Use on Innovation: A Literature Review. Berlin: DIW Berlin. Available at: SSRN: https://ssrn.com/abstract=2327428
Group of Chief Scientific Advisors (2018). Novel Carbon Capture and Utilisation Technologies (Scientific Opinion). Brussels: European Commission, Directorate-General for Research and Innovation.
Hendriks, C., Paul, N., Paul, Z., and Cook, G. (2013). Implications of the Reuse of Captured CO2 for European Climate Action Policies. Utrecht: ECOFYS Netherlands B.V.
Horbach, J., Rammer, C., and Rennings, K. (2012). Determinants of Eco-Innovations by Type of Environmental Impact - The Role of Regulatory Push/pull, Technology Push and Market Pull. Ecol. Econ. 78, 112–122. doi:10.1016/j.ecolecon.2012.04.005
IASS (2016). CO₂ als Wertstoff (CCU) - Potenziale & Herausforderungen für die Gesellschaft. IASS Potsdam. Available at: http://www.iass-potsdam.de/de/forschung/technologischer-wandel/ccu (accessed Feb 28).
ICEF, CO2 Sciences and The Global CO2 Initiative (2016). Global Roadmap for Implementing CO2 Utilization United States: ICEF.
International Chamber of Commerce (ICC) (2016). Role of Carbon and Long-Term Mitigation Strategies beyond 2050 through Carbon Capture and Use (CCU) and Closing the Carbon Cycles (C³).
IOGP (2019). “The Potential for CCS and CCU in Europe,” in Report to the Thirty Second Meeting of the European Gas Regulatory Forum.
Jülich, P. (2017). Umweltfreundliche Baumaterialien aus CO2. Available at: https://www.ptj.de/ueber-uns/aktuelles/archiv?news=news/co2min (accessed July, 2021).
Krausmann, F., Lauk, C., Haas, W., and Wiedenhofer, D. (2018). From Resource Extraction to Outflows of Wastes and Emissions: The Socioeconomic Metabolism of the Global Economy, 1900-2015. Glob. Environ. Change 52, 131–140. doi:10.1016/j.gloenvcha.2018.07.003
Kremer, D., Etzold, S., Boldt, J., Blaum, P., Hahn, K. M., Wotruba, H., et al. (2019). Geological Mapping and Characterization of Possible Primary Input Materials for the Mineral Sequestration of Carbon Dioxide in Europe. Minerals 9 (8), 485. doi:10.3390/min9080485
Le Quéré, C., Andrew, R. M., Friedlingstein, P., Sitch, S., Hauck, J., Pongratz, J., et al. (2018). Global Carbon Budget 2018. Earth Syst. Sci. Data 10 (4), 2141–2194. doi:10.5194/essd-10-2141-2018
McNutt, M. (2015). Climate Intervention: Carbon Dioxide Removal and Reliable Sequestration. Washington D.C: National Academy of Sciences.
Müller, C. (2012). Use of Cement in concrete According to European Standard EN 206-1. HBRC J. 8 (1), 1–7. doi:10.1016/j.hbrcj.2012.08.001
Munda, G. (2016). “Multiple Criteria Decision Analysis and Sustainable Development,” in Multiple Criteria Decision Analysis (New York, NY: Springer), 1235–1267. doi:10.1007/978-1-4939-3094-4_27
NSW Environment Protection Authority (2020). Waste Levy. Available at: https://www.epa.nsw.gov.au/your-environment/waste/waste-levy (accessed January, 2021).
Olfe-Kräutlein, B., Naims, H., Bruhn, T., and Lafuente, A. M. L. (2016). CO2 as an Asset - Potentials and Challenges for Society. Potsdam: IASS.
Ostovari, H., Sternberg, A., and Bardow, A. (2020). Rock 'n' Use of CO2: Carbon Footprint of Carbon Capture and Utilization by Mineralization. Sustain. Energ. Fuels 4 (9), 4482–4496. doi:10.1039/d0se00190b
Saaty, T. L. (1980). The Analytic Hierarchy Process Mcgraw Hill, 70. New York: Agricultural Economics Review.
Saaty, T. L. (2008). Decision Making with the Analytic Hierarchy Process. Int. J. Serv. Sci. 1 (1), 83–98. doi:10.1504/ijssci.2008.017590
Sandalow, D., Aines, R., Friedmann, J., McCormick, C., and McCoy, S. (2017). Carbon Dioxide Utilization (CO2U). United States: ICEF.
Sanna, A., Uibu, M., Caramanna, G., Kuusik, R., and Maroto-Valer, M. M. (2014). A Review of mineral Carbonation Technologies to Sequester CO2. Chem. Soc. Rev. 43 (23), 8049–8080. doi:10.1039/c4cs00035h
Schlögl, R., Abanades, C., Aresta, M., Azapagic, A., Blekkan, E. A., Cantat, T., et al. (2018). Novel Carbon Capture and Utilisation Technologies: Research and Climate Aspects.
Shao, Y. (2014). Beneficial Use of Carbon Dioxide in Precast Concrete Production. Montreal, QC(Canada): McGill Univ.
Stavins, R. N. (2000). “Market-based Environmental Policies, ” in Public Policies for Environmental Protection. 2nd Edn. Editors P. R. Portney, and R. N. Stavins (Washington, DC: Resources for the Future).
Styring, P., Jansen, D., de Coninck, H., Reith, H., and Armstrong, K. (2011). Carbon Capture and Utilization in the green Economy. (Accessed 10 25, 2016)
Sustainable Development Goals Knowledge Platform (). Tax on Waste (Landfill Tax). Available at: https://sustainabledevelopment.un.org/index.php?page=view&type=99&nr=133&menu=1449 (accessed January, 2021).
Turnau, S., Jaspers, D., Marxen, A., Naims, H., de Bruyn, S., Bringezu, S., et al. (2019). Identification and Analysis of Promising Carbon Capture and Utilisation Technologies, Including Their Regulatory Aspects. Brussels: European Commission.
von der Assen, N., Jung, J., and Bardow, A. (2013). Life-cycle Assessment of Carbon Dioxide Capture and Utilization: Avoiding the Pitfalls. Energy Environ. Sci. 6 (9), 2721–2734. doi:10.1039/c3ee41151f
Whiriskey, K. W., and Wolthuys, J. W. (2016). CCU in the EU ETS: Risk of CO2 Laundering Preventing a Permanent CO2 Solution. Oslo: Bellona Europa.
Wilson, G., Travaly, Y., Brun, T., Knippels, H., Armstrong, K., Peter, S., et al. (2016). A VISION for Smart CO2 Transformation in Europe: Using CO2 as a Resource Brussels: SCOT project.
X-Prize (). Turning CO2 into Products. Available at: https://www.xprize.org/prizes/carbon (accessed July, 2021).
Zero Emissions Plattform (2017). Climate Solutions for EU Industry: Interaction between Electrification, CO2 Use and CO2 Storage Brussels: Zero Emissions Platform.
Zero Emissions Platform (2013). CO2 Capture and Use (CCU) - the Potential to Reduce CO2 Emissions and Accelerate CCS Deployment in Europe.
Zimmermann, A., and Kant, M. (2016). The Business Side of Innovative CO2 Utilisation. EIT Climate KIC/TU Berlin. Available at: http://enco2re.climate-kic.org/wp-content/uploads/2016/01/The-business-side-of-innovative-CO2-utilisation.pdf (accessed August 11, 2016).
Keywords: CCU carbon capture and utilisation, CCS, carbon capture and sequestration, CCUS carbon capture, utilization and storage, mineralisation, carbonatisation, policy
Citation: Olfe-Kräutlein B, Strunge T and Chanin A (2021) Push or Pull? Policy Barriers and Incentives to the Development and Deployment of CO2 Utilization, in Particular CO2 Mineralization. Front. Energy Res. 9:742709. doi: 10.3389/fenrg.2021.742709
Received: 16 July 2021; Accepted: 25 August 2021;
Published: 14 December 2021.
Edited by:
Greeshma Gadikota, Cornell University, United StatesReviewed by:
Hannu-Petteri Mattila, Independent researcher, Parainen, FinlandRaffaella Pomi, Sapienza University of Rome, Italy
Copyright © 2021 Olfe-Kräutlein, Strunge and Chanin. This is an open-access article distributed under the terms of the Creative Commons Attribution License (CC BY). The use, distribution or reproduction in other forums is permitted, provided the original author(s) and the copyright owner(s) are credited and that the original publication in this journal is cited, in accordance with accepted academic practice. No use, distribution or reproduction is permitted which does not comply with these terms.
*Correspondence: Barbara Olfe-Kräutlein, YmFyYmFyYS5vbGZlLWtyYWV1dGxlaW5AaWFzcy1wb3RzZGFtLmRl