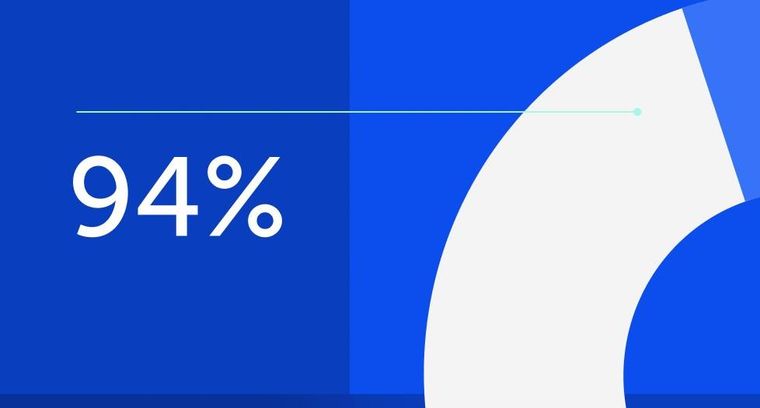
94% of researchers rate our articles as excellent or good
Learn more about the work of our research integrity team to safeguard the quality of each article we publish.
Find out more
ORIGINAL RESEARCH article
Front. Energy Res., 28 September 2021
Sec. Nuclear Energy
Volume 9 - 2021 | https://doi.org/10.3389/fenrg.2021.735199
This article is part of the Research TopicReactor Fuels, Materials and Systems under Extreme EnvironmentsView all 29 articles
A correction has been applied to this article in:
Corrigendum: Experimental Investigation on the Cleaning Effect and Influence Rule of Hydrogen Peroxide–Acetic Acid on Lead–Bismuth Eutectic Alloy
During the refueling process of lead–bismuth eutectic (LBE)–cooled fast reactors, lead–bismuth alloy can easily adhere to the surface of the fuel rod clads when spent fuel assemblies are unloaded from the reactor core. For some designs, the lead–bismuth alloy attached on the spent fuel rods will be cleaned via physical or/and chemical methods prior to their transportation, storage, and reprocessing. In this article, the cleaning effect of a washing lotion composed of hydrogen peroxide (H2O2) aqueous solution and concentrated acetic acid (CH3COOH) as the main components on LBE was experimentally investigated. By adjusting the composition ratio of the washing lotion and the reaction temperature, the law of their influence on the cleaning effect of LBE was determined. The optimal washing lotion composed of 30 Vol% hydrogen peroxide aqueous solution as oxidant, 40 Vol% concentrated acetic acid as acid, and 30 Vol% ultrapure water as the solvent was proposed based on experimental investigations. The optimal working temperature range was also obtained. The reaction intermediate product was characterized with the XRD analysis in order to understand the reaction mechanism. The composition of the released gas (even in a slight amount) was also analyzed with gas chromatography. The hydrogen concentration in the released gas was found to be lower than the detection limit of gas chromatography (10 ppm). Since the explosion limits of hydrogen at atmospheric pressure are between 3.95 and 75.73 Vol%, the risk of possible hydrogen explosion is concluded to be extremely low. Therefore, no special treatment of the released gas is required even in a large-scale industrial platform. Furthermore, corrosion effect of the washing lotion on austenitic stainless steel components was tested as well and found to be negligibly small. The relative mass loss of the thin-walled stainless steel samples immersed in the washing lotion was found to be less than 0.5% after an experimental duration of 144 h, which facilitates sufficient cleaning time in future industrial applications.
•Cleaning effect and influence rule of hydrogen peroxide–acetic acid lotion on lead–bismuth eutectic alloy were experimentally determined.
•Dissolving reaction mechanism was determined based on the XRD analysis of reaction intermediate products.
•Hydrogen concentration of the released gas during dissolution was found to be less than 10 ppm.
•Risk of possible hydrogen explosion during the dissolution process was concluded to be extremely small.
•Corrosion effect of the washing lotion on austenitic stainless steel components was experimentally determined and was found to be insignificant with a mass loss of less than 0.5% after 144 h.
As a clean, efficient, and sustainable energy source, nuclear power plays an important role in the long-term process of reducing carbon emissions and gradually achieving carbon neutrality. Liquid lead–bismuth eutectic alloy (LBE)–cooled fast reactors (LFRs) possess various technological advantages over the light water-cooled reactors (LWRs), such as inherent safety, high economy, and enhanced sustainability. Therefore, LFRs have attracted much attention in recent years (GIF, 2014; Smith and Cinotti, 2016; Lorusso et al., 2018). The large margin between the melting point (125°C) and the boiling point (1,670°C) of LBE (OECD/NEA, 2015) enables the reactor core cooled by liquid LBE to operate at relative low-pressure levels without any pressurization requirement. Due to the high boiling point of the coolant, the core outlet temperature is typically over 500°C and can be further increased to as high as 800°C, which makes LFRs applicable for hydrogen production (Smith and Cinotti, 2016; Wu et al., 2016). In addition, the excellent heat transfer performance of liquid LBE enables a more compact design of the reactor core, which makes the construction of small modular reactors (SMRs) possible and can largely broaden the application prospects of LFRs. Furthermore, the fast neutron spectrum in LFRs can breed new fissionable material to facilitate a closed fuel cycle, which can significantly increase the fuel utilization ratio and reduce the amount of high-level nuclear waste (HLW) simultaneously. Last but not least, coupling of an LFR with a proton accelerator builds up the so-called accelerator-driven subcritical system (ADS), which is considered as one of the most promising technological approaches to solve the problem of HLW (Abderrahim, 2012; Zhan and Xu, 2012).
Nevertheless, commercial deployment of LFRs is hindered by a series of engineering challenges. Up to now, researches on the key technologies of LFRs were firstly focused on material compatibility aspects, most notably on the degradation effects of the candidate’s structure/clad materials due to liquid metal corrosion (LMC) and liquid metal embrittlement (LME) (OECD/NEA, 2015; Gong et al., 2016; Huang et al., 2020a). Studies on thermal-hydraulic aspects have also been widely reported in the literature, for instance, on the heat transfer behavior in a wire-wrapped fuel assembly (Cheng and Tak, 2006; Pacio et al., 2016; Pacio et al., 2017; Chai et al., 2019) and on the heat transfer deterioration due to flow blockage accident (Di Piazza et al., 2014; Chai et al., 2019). Furthermore, intensive investigations on liquid metal–water interaction during the steam generator tube rupture (SGTR) accident have also been conducted (Wang et al., 2008; Huang et al., 2020b; Yu et al., 2021). However, technology aspects regarding refueling and spent fuel management have been rarely reported in open literature.
During the refueling process, lead–bismuth alloy can adhere to the surface of the fuel rod clads easily when spent fuel assemblies are unloaded from the reactor core. For the purpose of minimizing the waste volume and mass, the lead–bismuth alloy attached on the spent fuel rods needs to be cleaned via physical or/and chemical methods prior to their transportation, storage, and reprocessing. The most commonly applied cleaning lotion of lead–bismuth alloy consists of three components, including an oxidant (hydrogen peroxide, H2O2, 30% aqueous solution), an acid (concentrated acetic acid, CH3COOH, > 96% aqueous solution), and a solvent (ultrapure water or ethanol), typically with a volume ratio of 1:1:1, and the cleaning process should be performed normally at room temperature. This cleaning method defined by Schroer (2015) is adopted as a standard procedure by many research groups in their routine laboratory applications (Fazio et al., 2003; Ejenstam et al., 2013; Shi et al., 2019; Gong et al., 2021).
However, no further information or reason was given in the literature regarding the definition of this specific volume ratio or the reaction temperature at room conditions. The cleaning lotion composition for laboratory usage could be defined in a rather arbitrary way, while a large industrial scale spent fuel management program requires a more precise definition of the washing lotion composition, in order to obtain an optimal cleaning effect without any material squander and more importantly, to minimize the volume of the final liquid waste simultaneously, which is considered at least as low-level nuclear waste (LLW) that needs to be carefully treated before disposal at any rate.
In a research framework co-initiated by Shenzhen University (SZU) and the Institute of Nuclear Power Operation Safety Technology (INPOST), a comprehensive research program regarding LFR spent fuel management was defined. The current study described the very first research activity regarding the cleaning effect and influence of a washing lotion mixture on the lead bismuth eutectic alloy. The washing lotion consists of hydrogen peroxide (30% aqueous solution) and concentrated acetic acid (99.5%) as the main component. In order to determine the optimal composition of the washing lotion, series of washing lotions were set up with varying volume concentration of the oxidant and acid, respectively. Influence of the washing lotion temperature on the cleaning effect of LBE was also investigated experimentally.
The current study focused on the chemical cleaning effect of the washing lotion. Three reagents were used to define the washing lotion in the experimental investigation, that is, hydrogen peroxide aqueous solution (30% analytical purity, manufactured by Sinopharm Chemical Reagent Co., Ltd.) as oxidant, concentrated acetic acid (99.5% analytical purity, manufactured by Shanghai McLean Biochemical Technology Co., Ltd.) as acid, and ultrapure water (self-made in laboratory with a ultrapure water meter Millipore Milli-Q Direct 8, manufactured by Millipore Corporation) or anhydrous ethanol (99.5%, anhydrous grade, moisture ≤0.005%, manufactured by Shanghai Aladdin Biochemical Technology Co., Ltd.) as solvent.
The lead–bismuth eutectic alloy applied in this study was provided by Zhengzhou Shengboda Special Alloy Co., Ltd. Its nominal composition is determined according to the binary eutectic phase diagram of Pb and Bi, that is, 44.5 Wt.% Pb and 55.5 Wt.% Bi (OECD/NEA, 2015). Content and composition of impurities in the lead–bismuth alloy as-received are given in Table 1.
TABLE 1. Content and composition of the impurities in the lead bismuth alloy as-received. All elemental concentrations are given in ppm Wt.%; the balance is LBE.
In the experimental investigations, lead–bismuth eutectic alloy blocks fabricated in a flat, cylindrical shape (outer diameter of Φ10 mm, height of 3.2 mm), and thin-walled austenitic 316L stainless steel blocks were used as test samples. A simple “weighing method” was adopted in the current study to quantify the cleaning effect of the washing lotion. Therefore, test samples (LBE and stainless steel blocks) were first dried in a vacuum chamber for 24 h and then weighed with the mass recorded as the initial sample mass minitial. After being cooled to room temperature, test samples were then put into the prepared washing lotion contained in a laboratory glass beaker for soaking and dissolving. The respective reaction phenomena of the dissolution process were observed. The mass of the remaining samples after certain dissolving duration was again weighed so that the dissolution rate (in [g/min]) can be calculated by the following:
where minitial and mfinal stand for the initial sample mass in [g] and the residual sample mass in [g] after a dissolution duration of ∆τ in [min], respectively. The average dissolution rate of the LBE blocks (in [g/min]) was then obtained by weighing the remaining sample mass after 24 h, if the initial blocks were not completely dissolved. In case of a complete dissolution, the duration of the dissolution process was also recorded, in order to calculate the average dissolution rate in line with Eq. 1. The error of the recorded dissolution duration is estimated to be less than 2 min, while weighing of the test samples, both before and after the dissolution (only in case of an incomplete dissolution), was conducted by a laboratory electronic balance with a linear error of 0.0002 g. Consequently, relative error of the dissolution rates obtained in the current study was estimated to be less than 5%.
It should be pointed out that the main objective of the current study was to quantify the influence of the washing lotion composition and reaction temperature on the cleaning effect of LBE. Therefore, the absolute value of the dissolution rate is of less relevance. Within a test series, the dissolution rate was then normalized with the maximal dissolution rate of the current test series as reference:
where Dissolution Rate Normalized i is the dissolution rate of the i th case of a test series with the same washing lotion composition and reaction temperature, while max (Dissolution Rate) is the maximal dissolution rate of the current test series.
In the current study, series of washing lotions were set up with varying concentration of the oxidant and acid, respectively, for the purpose of determining an optimal composition of the washing lotion. The test sample of LBE blocks have the same dimension (outer diameter of Φ10 mm, height of 3.2 mm) and mass (roughly 2.5 g). The LBE test sample can be completely dissolved with 30 ml washing lotion of the optimal composition (see conclusion of this subsection) within 2 h, which is a feasible time for routine undertaking in our laboratory. Therefore, the total volume of the washing lotion was kept constant at 30 ml in the study of the influence of the washing lotion composition.
Metallic bismuth cannot directly react with nonoxidizing acids, and lead is also difficult to react with organic acids under anaerobic/anoxic conditions. As a consequence, both bismuth and lead need to be first oxidized and then react with acetic acid to dissolve, so the oxidant (hydrogen peroxide, H2O2) plays a significant role in the entire dissolution process of the LBE blocks. In this respect, increasing the oxidant concentration contributes to promoting the dissolution of lead–bismuth alloys. On the other hand, however, hydrogen peroxide is unstable and is prone to self-decomposition when promoted by heating. In order to obtain the optimal oxidant concentration, nine groups of washing lotion with different hydrogen peroxide concentrations (6–12 ml 30% H2O2 aqueous solution, 0.5–1 ml interval between two neighboring groups) were set up. On the contrary, volume of the concentrated acetic acid (8 ml 99.5% acetic acid) and the total volume of the washing lotion (30 ml, with ultrapure water as solvent) were kept unchanged in the test series. The corresponding volume concentration of the H2O2 aqueous solution in the washing lotion is hence varied between 20 and 40 Vol %.
As depicted in Figure 1, the normalized dissolution rate and, hence, the cleaning effect of the washing lotion strongly depends on the volume concentration of the H2O2 aqueous solution. The main results are summarized as follows:
a) Theoretically, increasing the oxidant concentration help to promote the dissolution of lead–bismuth alloys. In the range of 20 Vol% to 30 Vol%, as the concentration of H2O2 aqueous solution increases, the dissolution rate of the washing lotion increases significantly. While the flat, cylindrical-shaped LBE block could not be completely dissolved with the washing lotion of 20 Vol% of H2O2 aqueous solution after 24 h; at an oxidant volume concentration of 30 Vol%, the dissolution rate increases by an order of magnitude and the same LBE block was completely dissolved after soaking for about 2 h.
b) With the nine groups of washing lotions of the same total volume (30 ml) containing the same amount of concentrated acetic acid (8 ml), the best cleaning effect of LBE is obtained when the volume concentration of H2O2 aqueous solution is 30%, corresponding to a washing lotion consisting of 9 ml H2O2 aqueous solution, 8 ml concentrated acetic acid, and ultrapure water as solvent.
c) However, as the volume concentration of H2O2 aqueous solution further increases beyond 30 Vol%, the dissolution rate starts to drop dramatically. It can be observed that the three high concentration cases (33.3, 36.7, and 40 Vol%, respectively) have the worst performance in the dissolution of the LBE block. The main reason is that higher hydrogen peroxide concentration results in a violent dissolution reaction in the early stage and hence releases a large amount of reaction heat to the reaction system, which leads to a thermal runaway phenomenon similar to bumping. Consequently, the system temperature increases rapidly in the early stage. At high system temperature, hydrogen peroxide is prone to self-decomposition. The self-decomposition of hydrogen peroxide into water and oxygen is an exothermic reaction, which again releases even more reaction heat and accelerates the self-decomposition. Consequently, the self-decomposition causes a large loss of hydrogen peroxide, and finally gives rise to a sharp drop in the dissolution rate of the lead–bismuth alloy block. After soaking for 24 h, the lead–bismuth alloy block was still not completely dissolved and the remaining sample in the form of a white powdery solid was observed.
d) To sum up, the positive effect of increasing oxidant concentration to promote the dissolution LBE is more significant at a H2O2 aqueous solution concentration less than the critical concentration of 30 Vol%. Once the hydrogen peroxide concentration is higher than this critical concentration, intensive heat accumulation is likely to occur already during the early stage of the dissolution process; the higher temperature results in decomposition of the hydrogen peroxide, and the cleaning effect of the washing lotion will be weakened to a great extent.
The acetic acid not only acts as a washing liquid during the reaction but also has an effect on the oxidability of hydrogen peroxide. Consequently, changing the concentration of acetic acid has a certain impact on the dissolution rate and the cleaning performance of the washing lotion. Therefore, a sensitivity study of the acid concentration was conducted in a like manner.
The total volume of the washing lotion was kept constant at 30 ml. Based on the above sensitivity study of the hydrogen peroxide concentration, it was concluded that for an optimal cleaning effect 9 ml hydrogen peroxide aqueous solution should be contained in the 30 ml washing lotion. As mentioned in the introduction, the volume ratio of hydrogen peroxide aqueous solution to concentrated acetic acid was normally set at 1:1 in the literature (Schroer, 2015), yet without any detailed explanation. Nevertheless, this 1:1 ratio provides a good reference for the experimental setups. Therefore, aiming to find an optimal concentration of acetic acid, eight groups of washing solutions with different acid concentrations (7–14 ml concentrated acetic acid, 1 ml interval between two neighboring groups) were prepared. The volume concentration of hydrogen peroxide aqueous solution (30 Vol%, the optimal concentration) and the total volume of the washing lotion (30 ml, ultrapure water as solvent) were kept unchanged in the test series.
Figure 2 shows the relationship between the dissolution rates and the volume concentration of the concentrated acetic acid. The main results are summarized as follows:
a) As the concentration of acetic acid increases, the dissolution rate experiences a steady, but slow growth firstly. There exists apparently a threshold volume concentration between 33.3 and 36.7 Vol%. While the dissolution rate at the acid concentration of 33.3 Vol% (corresponding volume of the concentrated acetic acid in the washing lotion is 10 ml) is less than 10 % of the maximal dissolution rate of the test series, it increases rapidly to roughly 70% of the maximal dissolution rate of the test series at the acid concentration of 36.7 Vol% (corresponding volume of the concentrated acetic acid is 11 ml), once the acid volume concentration surpasses the threshold volume concentration. Finally, the peak value of the dissolution rate was found at the acid concentration of 40 Vol% (corresponding volume of the concentrated acetic acid is 12 ml).
b) However, the dissolution rate decreases again at a higher acid volume concentration of 46.7% (corresponding volume of the concentrated acetic acid is 14 ml). With volume concentration of acid increasing, the amount of ultrapure water (as solvent) in the washing lotion decreases, which affects the dissociation of acetic acid, and finally the oxidability of hydrogen peroxide decreases, resulting in the decrease in the dissolution rate of the lead–bismuth alloy block. According to this test series, the optimal volume concentration of the concentrated acetic acid with the best dissolution performance should be 40 Vol%.
FIGURE 2. The relationship between the dissolution rate and the volume concentration of acetic acid and ultrapure water as solvent.
Up to now, the solvent of the washing solution has always been ultrapure water. However, as defined in the standard cleaning method (Schroer, 2015), the choice of the solvent is normally ethanol. In order to study the influence of the solvent type on the performance of the washing lotion, the ultrapure water solvent was replaced by a mixed solvent consisting of 50% ultrapure water and 50% ethanol. Six groups of different acetic acid concentrations (7 ml, 8 ml, 9 ml, 10 ml, 12 ml, and 14 ml concentrated acetic acid) were prepared, while the volume concentration of hydrogen peroxide aqueous solution (30 Vol%, the optimal concentration) and the total volume of the washing lotion (30 ml) remained unchanged.
Figure 3 then compares the dissolution rate for the two different solvent type of ultrapure water and the mixed solvent consisting of 50% ultrapure water and 50% ethanol. For consistency, dissolution rates were normalized with the same maximal value of the dissolution rate obtained at the acid concentration of 40 Vol% with ultrapure water as solvent. As depicted in the figure, the dissolution rate drops drastically after replacing part of the solvent with ethanol. When the solvent changes from ultrapure water to water–ethanol mixture, the ionization constant of acetic acid increases (Hu et al., 1991). However, after ionization of acetic acid, the ionized protons (H+) will combine with ethanol and water, respectively, and exist in the form of solvation in the solution. The solvated protons C2H5OH2+ are more stable than H3O+ (Lei et al., 1995), which reduces the reactivity of the water–ethanol mixed solvent-based washing lotion, and the dissolution rate is significantly lower than that of the ultrapure water solvent-based washing lotion.
FIGURE 3. The relationship between the dissolution rate and the solvent type: (A) 100% ultrapure water; (B) 50% ultrapure water + 50% ethanol.
From the above three sets of experiments with respect to the influence of oxidant and acid concentration as well as solvent type, it can be concluded that for a 30 ml washing lotion, the optimal composition should consist of 9 ml H2O2 aqueous solution and 12 ml concentrated acetic acid, with the rest is ultrapure water as solvent. Volume concentration of H2O2 aqueous solution and concentrated acetic acid in the optimal washing lotion are 30 Vol% and 40 Vol%, respectively. Furthermore, it is recommended that ultrapure water should be used as solvent instead of ethanol.
Up to now, all the experiments have been conducted under room condition. The LBE blocks as test samples were put into the prepared washing lotion contained in a laboratory glass beaker for soaking and dissolving, while the glass beaker was standing free in air without any temperature control of the reaction system consisting of washing lotion and a LBE block. Although the reaction system was put free in air at room temperature, the actual reaction temperature also depends on the reaction heat released during the dissolution of the LBE block in the washing lotion. Consequently, this temperature might differ from the room temperature largely. Obviously, the washing lotion temperature has a certain significant impact on the reaction kinetics, and more importantly on the stability of the oxidant H2O2.
For the purpose of studying the effect of temperature on the cleaning effect, eight groups of different experimental temperatures settings were set up, that is, room condition at a measured room temperature of 23.2 °C, as well as seven different temperature values controlled with a water bath of 23.8 °C (measured room temperature), 25 °C, 30 °C, 32.5 °C, 35 °C, 37.5 °C, and 40 °C, respectively. It should be pointed out that room condition without water bath means that the reaction system was standing free in air at room temperature. But room temperature at 23.8 °C with water bath means that the temperature of the reaction system was controlled with a water bath, which was set at the measured room temperature of 23.8 °C. The total washing lotion volume of 30 ml as well as its composition were kept unchanged, which consist of 9 ml hydrogen peroxide aqueous solution, 12 ml concentrated acetic acid, and 9 ml ultrapure water.
As depicted in Figure 4, the temperatures setting has a vital impact on the dissolution rate and, hence, on the cleaning effect of LBE. The main results can be summarized as follows:
a) As the system temperature controlled by water bath increase from 23.8 °C to 25 °C to above 30 °C, the dissolution rate of LBE increases rapidly and reaches the highest value at the water bath temperature of 32.5 °C, under which the dissolution rate is almost an order of magnitude larger than under room temperature at 23.8 °C with water bath. The main reason can be attributed to the increase of reaction kinetics promoted by temperature.
b) However, as the water bath temperature further increase to 35 °C, 37.5 °C, and 40 °C, the dissolution rate drops dramatically. This may be caused by the superposition of the reaction heat and the heat provided by water bath, which intensifies the self-decomposition of hydrogen peroxide, reduces the hydrogen peroxide concentration and, hence, lowers the dissolution rate.
c) Furthermore, during the experiment under room condition without water bath, it was found by infrared thermometers (Fluke VT04A, Fluke Company) that surface temperature of the wash lotion was around 30 °C, even though the measured room temperature was 23.2 °C. This is mainly due to the reaction heat released during the dissolution process. Consequently, it is also observed in Figure 4 that the dissolution rate of LBE under room condition without water bath is significantly higher than under a water bath temperature of 23.8 °C and 25 °C, where the water bath has a heat dissipation effect on the released reaction heat and, hence, reduces the dissolution rate.
d) On the basis of the experiment, it can be concluded that a system temperature of 30 °C–35 °C controlled by water bath is the ideal range for the cleaning effect of the washing lotion. The optimal reaction temperature in the experimental test series was found at 32.5 °C controlled by water bath.
e) Nevertheless, it should be pointed out that cooling devices to control the system temperature should be installed in a large-scale industrial platform, since the washing lotion will be heated by reaction heat superimposed with decay heat released by spent fuel. The reaction temperature should be controlled/cooled under 35 °C in order to alleviate possible self-decomposition of hydrogen peroxide.
FIGURE 4. The relationship between system temperature and the dissolution rate of the lead–bismuth alloy block.
In order to understand the specific dissolution reaction path of the washing lotion to the lead–bismuth alloy block, X-ray diffractometer, XRD (Empyrean, PANalytical Corporation, Netherlands), was employed to characterize the reaction intermediate product, that is, the incompletely dissolved lead–bismuth alloy block, which is present in the form of a white powdery solid.
Two washing lotions of the same optimal composition, that is, 30 Vol% hydrogen peroxide aqueous solution, 40 Vol% concentrated acetic acid, and ultrapure water serves as solvent, but different total volume of 10 and 15 ml were prepared for dissolution of the LBE blocks under room condition. After 2 h of reaction, it was observed that the LBE blocks were not completely dissolved in both washing lotions. The residual LBE block was covered by the intermediate reaction product in the form of a white powdery solid. For characterization of its composition, the white powdery solid was then carefully scratched and collected for further analysis with XRD. As depicted in Figure 5, the white powdery solid collected from the 10 ml washing lotion was labeled as “Sample 1” in the figure, while that collected from the 15 ml washing lotion was labeled as “Sample 2”. For comparison, the standard XRD patterns of bismuth (III) acetic acid (C2H3BiO3) and bismuth (III) acetate (C6H9BiO6) were also included in the figure. As illustrated in Figure 5, it could be concluded that the main components of the intermediate reaction product are bismuth (III) acetic acid (C2H3BiO3) and bismuth (III) acetate (C6H9BiO6). Since bismuth (III) acetic acid is a hydrolysis product of bismuth (III) acetate, it can be concluded that bismuth acetate has undergone a hydrolysis reaction. The main components of the intermediate reaction product are the compounds of bismuth instead of the compounds of lead. The main reason is that the standard electrode potential of lead (−1.262 V) is lower than that of bismuth (≤ 0.32 V), therefore it is more susceptible to oxidation by hydrogen peroxide, followed by a dissolution with acetic acid. Accordingly, it can be concluded that the main reactions of dissolving the lead–bismuth alloy block with the washing lotion are as follows:
a) oxidation of the metallic elements with hydrogen peroxide:
b) dissolution of the metallic oxide in acetic acid:
c) hydrolysis of bismuth acetate:
During the dissolving process of the lead–bismuth alloy block, it was observed that there are a few bubbles in the solution, and more gas products were formed on the surface of the lead–bismuth alloy block. As acid and metallic elements were present in the reaction system, it was suspected that hydrogen gas might be released. Even though the amount of the gas products is not significant in laboratory, for large-scale industrial applications, the gas components produced during the cleaning process possess a significant impact on production safety, especially when the highly explosive hydrogen is accumulated locally. In this experiment, four groups of washing lotion with the same composition yet different total volumes (20 ml, 30 ml, 40 ml, and 50 ml) were set up. The lead-bismuth alloy block was cleaned separately and the released gas products were collected with laboratory gas-sampling bags. Their respective compositions were then analyzed by 5 A molecular sieve column gas chromatography.
Since the 5 A molecular sieve column used in gas chromatography is unable to identify the residual components such as carbon dioxide, water steam, and acetic acid, these residual components were tested after backflushing and separation. For the sake of safety, however, we should pay more attention to the hydrogen which may be produced via direct reaction of lead with acetic acid. As depicted in Figure 6, hydrogen was not detected in all experimental groups, that is, concentration of hydrogen was found to be lower than the detection limit of gas chromatography (10 ppm), indicating that the direct reaction of lead–bismuth alloy block with acetic acid is insignificant. The negligibly small amount of hydrogen released during the dissolution process might stem from the direct reaction of acetic acid with impurities in the lead bismuth alloy, most notably iron and magnesium elements (see Table 1). It should be pointed out that the nitrogen and the majority of the oxygen as detected in the gas products are stemming from the environmental atmosphere, which was presented in the reaction beaker and was, hence, inevitably collected in the gas-sampling bags. Furthermore, oxygen might also stem from the self-decomposition of hydrogen peroxide. Nevertheless, since the explosion limits of hydrogen at atmospheric pressure are between 3.95 and 75.73 Vol% (Le et al., 2012; Le et al., 2013), the negligibly small amount of hydrogen component indicates that the risk of hydrogen explosion is minimal. Consequently, the released gas products require no special treatment for hydrogen. However, the washing lotion contains a higher concentration of acetic acid, it is recommended to remove the irritating acid from the released gas products in the actual industrial operation, for instance, with an acid-base neutralization.
FIGURE 6. Composition of the gas products with different washing lotion volume: (A) 20ml, (B) 30ml, (C) 40ml, and (D) 50 ml.
Objective of washing lotion is cleaning the lead–bismuth alloy attached on spent fuel clad of LFRs, typically made of austenitic stainless steel. Therefore, corrosive effect of the washing lotion on the stainless steel elements should be investigated as well, especially considering the fact that the thickness of a typical fuel rod clad is in the order of 1 mm. If the washing lotion has an evident corrosive effect on the fuel rod clad material, then the cleaning process and duration must be carefully controlled in order to avoid intensive corrosion that might endanger the spent fuel clad integrity. In this part of investigation, corrosion effect of the washing lotion on thin-walled stainless steel blocks (thickness less than 1 mm) was investigated in two different models, that is, the stainless steel block soaked alone in the washing lotion and soaked together with LBE block in the washing lotion. Washing lotions of the same composition, that is, 30 Vol% hydrogen peroxide aqueous solution, 40 Vol% concentrated acetic acid and 30 Vol% ultrapure water, were adopted. The relative mass loss of the stainless steel blocks was determined after different soaking duration of 48, 96, and 144 h, respectively.
As depicted in Figure 7, corrosion of stainless steel components is positively correlated with the immersion time in an obvious way. In addition, the relative mass loss of stainless steel components immersed together with the lead–bismuth alloy block was significantly higher than that when the stainless steel blocks were immersed in the washing lotion alone. The reason is that the lead–bismuth alloy block generates heat during the dissolution process, which increases the possibility of the stainless steel components being oxidized by hydrogen peroxide, thereby raises the mass loss of the co-immersed stainless steel blocks as a consequence.
FIGURE 7. Influence of soaking duration on the corrosion mass loss rate of austenitic stainless steel.
Nevertheless, after 144 h, the relative mass loss of the stainless steel blocks was found still less than 0.5 %, and a tendency of approaching saturation was also observed. Furthermore, stainless steel test samples were observed with a simple visual observation after the experiment and no localized pit corrosion was found. Therefore, in practical applications, the immersion time of stainless steel components in the washing lotion should be minimized, but no special attention is required within at least 144 h.
The most commonly applied cleaning lotion of lead–bismuth alloy consists of an oxidant (30% hydrogen peroxide aqueous solution), an acid (concentrated acetic acid), and a solvent (ultrapure water or ethanol), typically with a volume ratio of 1:1:1. This cleaning method is adopted by many research groups in their routine laboratory applications. However, no further reasons were given regarding this definition of the volume ratio and the reaction temperature under room conditions. Such information is still insufficient in the open literature.
Therefore, in this study, series of experiments were carried out on the cleaning effect of lead–bismuth eutectic alloy adhering to stainless steel clad of spent fuel, and the key issues such as influence of the washing lotion composition and reaction temperature, reaction mechanism and reaction gas component, and washing lotion corrosiveness on the stainless steel components were explored as well. The current study fulfills the missing gap in the literature and also provides valuable reference data for the subsequent construction of a large-scale industrial experiment platform that is in progress at Shenzhen University (SZU) now. The main conclusions derived are summarized as follows:
a) Composition of the washing lotion has a significant influence on the cleaning effect. Based on experimental investigations conducted in the current study, an optimal washing lotion composition was proposed. Volume concentration of H2O2 aqueous solution (as oxidant) and concentrated acetic acid (as acid) in the optimal washing lotion are 30 Vol% and 40 Vol%, respectively. Furthermore, it is recommended that ultrapure water should be used as solvent.
b) In addition, system temperature also plays an important role in the cleaning effect, mainly due to the stability of hydrogen peroxide in the washing lotion, which is prone to self-decomposition at elevated system temperature. The cleaning effect and stability of the lotion were found to be fairly good in a temperature range between 30 °C and 35 °C, and the highest dissolution rate was observed in a water bath of a temperature of 32.5 °C.
c) Based on the XRD analysis of the unresolved samples, the main reactions of dissolving the lead–bismuth alloy block with the washing lotion are oxidation of the metallic elements with hydrogen peroxide, followed by dissolution of the metallic oxide in acetic acid.
d) Moreover, no detectable hydrogen component was found in the released gas products. It shows that lead and bismuth do not directly react with acetic acid, rather they are first oxidized by hydrogen peroxide and then react with acetic acid. Consequently, the risk of hydrogen explosion is extremely low, and there is no need to deal with the hydrogen explosion risk that may exist. But in practice, the acetic acid component of the lotion may volatilize, so the tail gas should be deacidified before ejecting to the environment.
e) Last but not the least, corrosion effect of the washing lotion on austenitic stainless steel components was found to be negligibly small. The relative mass loss of the stainless steel samples was ≤0.5% within 144 h, which facilitate sufficient cleaning time in industrial applications.
At last, limitations of the current study should be discussed, which also define the future prospects of this study. They are proposed as follows:
a) In the current study, LBE test samples fabricated in flat, cylindrical blocks were adopted in the experiments. However, in practical industrial applications, LBE adhering on the surface of the fuel clad, normally in thin films, needs to be cleaned and removed. Consequently, in future investigations, mock-up fuel assemblies with LBE adherent on the clad surface should be used as test samples, in order to quantify the cleaning effect of the washing lotion defined in the current study by a greater degree. Furthermore, physical cleaning effect, for instance, with an oil bath, should be explored as well, which might be defined as a complementary cleaning method to the chemical cleaning method as investigated in the current study. In practical industrial applications, both physical and chemical cleaning methods will play an important role.
b) In the current study, all the cleaning process was performed in laboratory scale in the so-called one-batch model, in which one test sample was cleaned in one-batch washing lotion. However, in large-scale industrial applications, multi-batch model is preferred, in which multiple spent fuel assemblies should be cleaned in one-batch washing lotion one after another, for the purpose of material saving and simplifying the working procedure. Therefore, online detection and control of the concentration of hydrogen peroxide in the washing lotion are of essential importance, in order to keep the hydrogen peroxide concentration within a reasonable range during a continuous cleaning model. Therefore, methods for concentration detection of hydrogen peroxide in the reaction system, for instance, a hydrogen peroxide concentration detector or a hydrogen peroxide test paper, should be tested first in a laboratory scale.
The raw data supporting the conclusions of this article will be made available by the authors, without undue reservation.
XH: conceptualization, methodology, writing—original draft, funding acquisition, and investigation; BP: conceptualization, methodology, writing—review and editing, funding acquisition, investigation, and project administration; XZ: investigation, data curation, formal analysis, and visualization; YY: conceptualization, methodology, and supervision.
The present study was supported partially by the Guangdong Provincial Natural Science Foundation (Grant No. 2021A1515010391) and by the Science and Technology Program of Shenzhen, Guangdong Province, China (Grant No. JCYJ20180305124244969).
The authors declare that the research was conducted in the absence of any commercial or financial relationships that could be construed as a potential conflict of interest.
All claims expressed in this article are solely those of the authors and do not necessarily represent those of their affiliated organizations, or those of the publisher, the editors, and the reviewers. Any product that may be evaluated in this article, or claim that may be made by its manufacturer, is not guaranteed or endorsed by the publisher.
Dissolution Rate, dissolution rate of the LBE block in [g/min]; Dissolution Rate Normalized, Normalized dissolution rate in [-]; max (Dissolution Rate), maximal dissolution rate of the LBE block of a test series in [g/min];
Abderrahim, H. A. (2012). Multi-purpose hYbrid Research Reactor for High-tech Applications a multipurpose fast spectrum research reactor. Int. J. Energ. Res. 36, 1331–1337. doi:10.1002/er.1891
Chai, X., Liu, X., Xiong, J., and Cheng, X. (2019). CFD analysis of flow blockage phenomena in a LBE-cooled 19-pin wire-wrapped rod bundle. Nucl. Eng. Des. 344, 107–121. doi:10.1016/j.nucengdes.2019.01.019
Cheng, X., and Tak, N.-I. (2006). CFD Analysis of Thermal-Hydraulic Behavior of heavy Liquid Metals in Sub-channels. Nucl. Eng. Des. 236, 385–393. doi:10.1016/j.nucengdes.2006.02.001
Di Piazza, I., Magugliani, F., Tarantino, M., and Alemberti, A. (2014). A CFD analysis of flow blockage phenomena in ALFRED LFR demo fuel assembly. Nucl. Eng. Des. 276, 202–215. doi:10.1016/j.nucengdes.2014.05.033
Ejenstam, J., Halvarsson, M., Weidow, J., Jönsson, B., and Szakalos, P. (2013). Oxidation studies of Fe10CrAl-RE alloys exposed to Pb at 550°C for 10,000h. J. Nucl. Mater. 443, 161–170. doi:10.1016/j.jnucmat.2013.07.023
Fazio, C., Ricapito, I., Scaddozzo, G., and Benamati, G. (2003). Corrosion behaviour of steels and refractory metals and tensile features of steels exposed to flowing PbBi in the LECOR loop. J. Nucl. Mater. 318, 325–332. doi:10.1016/s0022-3115(03)00009-6
Generation IV International Forum (GIF) (2014). Technology roadmap update for generation IV nuclear energy systems.
Gong, X., Chen, J., Xiang, C., Yu, Z., Gong, H., and Yin, Y. (2021). A comparative study on liquid metal embrittlement susceptibility of three FeCrAl ferritic alloys in contact with liquid lead-bismuth eutectic at 350°C. Corrosion Sci. 183, 109346. doi:10.1016/j.corsci.2021.109346
Gong, X., Li, R., Sun, M., Ren, Q., Liu, T., and Short, M. P. (2016). Opportunities for the LWR ATF materials development program to contribute to the LBE-cooled ADS materials qualification program. J. Nucl. Mater. 482, 218–228. doi:10.1016/j.jnucmat.2016.10.012
Hu, R., Yen, W., Lin, R., and Yu, Q. (1991). Heats of ionization of acetic acid and halogen-acetic acids in water-ethanol and water-dimethyl formamide mixtures. Thermochim. Acta 183, 65–71. doi:10.1016/0040-6031(91)80446-p
Huang, X., Chen, P., Yin, Y., Pang, B., Li, Y., Gong, X., et al. (2020). Numerical investigation on LBE-water interaction for heavy liquid metal cooled fast reactors. Nucl. Eng. Des. 361, 110567. doi:10.1016/j.nucengdes.2020.110567
Huang, X., Gong, X., Song, M., Chen, J., Hu, F., Yin, Y., et al. (2020). Liquid metal embrittlement susceptibility of a high-entropy alloy exposed to oxygen-depleted liquid lead-bismuth eutectic at 250 and 350 °C. J. Nucl. Mater. 528, 151859. doi:10.1016/j.jnucmat.2019.151859
Le, H., Liu, Y., and Mannan, M. S. (2013). Lower Flammability Limits of Hydrogen and Light Hydrocarbons at Subatmospheric Pressures. Ind. Eng. Chem. Res. 52, 1372–1378. doi:10.1021/ie302504h
Le, H., Nayak, S., and Mannan, M. S. (2012). Upper Flammability Limits of Hydrogen and Light Hydrocarbons in Air at Subatmospheric Pressures. Ind. Eng. Chem. Res. 51, 9396–9402. doi:10.1021/ie300268x
Lei, Q., Yu, Q., Zong, H., and Lin, R. (1995). Study on ionization thermodynamics of six organic acids in water-ethanol mixtures at 298.15 K. J. Zhejiang Univ. 29, 172–178.
Lorusso, P., Bassini, S., Del Nevo, A., Di Piazza, I., Giannetti, F., Tarantino, M., et al. (2018). GEN-IV LFR development: Status & perspectives. Prog. Nucl. Energ. 105, 318–331. doi:10.1016/j.pnucene.2018.02.005
OECD/NEA (2015). Handbook on lead-bismuth eutectic alloy and lead properties, materials compatibility, thermal hydraulics and technologies.
Pacio, J., Daubner, M., Fellmoser, F., Litfin, K., and Wetzel, T. (2016). Experimental study of heavy-liquid metal (LBE) flow and heat transfer along a hexagonal 19-rod bundle with wire spacers. Nucl. Eng. Des. 301, 111–127. doi:10.1016/j.nucengdes.2016.03.003
Pacio, J., Wetzel, T., Doolaard, H., Roelofs, F., and Van Tichelen, K. (2017). Thermal-hydraulic study of the LBE-cooled fuel assembly in the MYRRHA reactor: Experiments and simulations. Nucl. Eng. Des. 312, 327–337. doi:10.1016/j.nucengdes.2016.08.023
Schroer, C., Guidelines for corrosion testing in liquid metals (Pb, LBE), MATTER project, deliverable report D 3.4., 2015.
Shi, H., Jianu, A., Weisenburger, A., Tang, C., Heinzel, A., Fetzer, R., et al. (2019). Corrosion resistance and microstructural stability of austenitic Fe-Cr-Al-Ni model alloys exposed to oxygen-containing molten lead. J. Nucl. Mater. 524, 177–190. doi:10.1016/j.jnucmat.2019.06.043
Smith, C. F., and Cinotti, L. (2016). “Lead-cooled fast reactor,” in Handbook of Generation IV Nuclear Reactors (Cambridge: Woodhead Publishing), 119–155. doi:10.1016/b978-0-08-100149-3.00006-9
Wang, S., Flad, M., Maschek, W., Agostini, P., Pellini, D., Bandini, G., et al. (2008). Evaluation of a steam generator tube rupture accident in an accelerator driven system with lead cooling. Prog. Nucl. Energ. 50, 363–369. doi:10.1016/j.pnucene.2007.11.018
Wu, Y., Bai, Y., Song, Y., Huang, Q., Zhao, Z., and Hu, L. (2016). Development strategy and conceptual design of China lead-based research reactor. Ann. Nucl. Energ. 87, 511–516. doi:10.1016/j.anucene.2015.08.015
Yu, Q., Zhang, Y., Wang, C., Guo, C., Deng, J., Zhang, D., et al. (2021). Numerical simulation of bubble transport during steam generator tube rupture accident of Lead-cooled Fast Reactor. Ann. Nucl. Energ. 153, 108066. doi:10.1016/j.anucene.2020.108066
Keywords: Lead–bismuth eutectic (LBE) alloy, hydrogen peroxide–acetic acid washing lotion, cleaning effect, Lead-cooled fast reactor (LFR), spent fuel
Citation: Huang X, Pang B, Zhou X and Yin Y (2021) Experimental Investigation on the Cleaning Effect and Influence Rule of Hydrogen Peroxide–Acetic Acid on Lead–Bismuth Eutectic Alloy. Front. Energy Res. 9:735199. doi: 10.3389/fenrg.2021.735199
Received: 02 July 2021; Accepted: 20 August 2021;
Published: 28 September 2021.
Edited by:
Shripad. T Revankar, Purdue University, United StatesReviewed by:
Kamachi Mudali Uthandi Mudali, VIT Bhopal University, IndiaCopyright © 2021 Huang, Pang, Zhou and Yin. This is an open-access article distributed under the terms of the Creative Commons Attribution License (CC BY). The use, distribution or reproduction in other forums is permitted, provided the original author(s) and the copyright owner(s) are credited and that the original publication in this journal is cited, in accordance with accepted academic practice. No use, distribution or reproduction is permitted which does not comply with these terms.
*Correspondence: Bo Pang, Ym8ucGFuZ0BzenUuZWR1LmNu
Disclaimer: All claims expressed in this article are solely those of the authors and do not necessarily represent those of their affiliated organizations, or those of the publisher, the editors and the reviewers. Any product that may be evaluated in this article or claim that may be made by its manufacturer is not guaranteed or endorsed by the publisher.
Research integrity at Frontiers
Learn more about the work of our research integrity team to safeguard the quality of each article we publish.