- 1School of Physics, Southeast University, Nanjing, China
- 2National Laboratory of Solid State Microstructures, College of Engineering and Applied Sciences, Collaborative Innovation Center of Advanced Microstructures, Nanjing University, Nanjing, China
Solar interfacial evaporation, featured by high energy transfer efficiency, low cost, and environmental compatibility, has been widely regarded as a promising technology for solar desalination. However, the interplay between energy transfer and water transport in the same channels suggests that the tradeoff between high efficiency and long-term stability inherently exists in conventional photothermal nanomaterials. We summarize state-of-the-art research on various anti-salt clogging photothermal microstructures as long-term stable interfacial solar evaporators for solar desalination. The review starts with an overview of the current status and the fundamental limit of photothermal materials for solar desalination. Four representative strategies are analyzed in detail with the most recent experimental demonstrations, including fluid convection enhancement, surface wettability engineering, energy-mass-path decoupling, and surface chemistry engineering. Finally, this article focuses on the challenges in anti-salt clogging solar interfacial evaporators and potential point-of-use applications in the future.
Introduction
The shortage of freshwater resources has become one of the most severe global challenges nowadays (Shannon et al., 2008; Elimelech and Phillip, 2011; Cetrulo et al., 2019). To address the current situation of global water scarcity, widespread concerns have been garnered about freshwater extraction from seawater (Dobson et al., 2002; Gude, 2011). Solar desalination is an environment-friendly water treatment technology without the additional consumption of fossil energy (Kalogirou, 1997; Abdel-Rehim and Lasheen, 2005; Qiblawey and Banat, 2008). In recent years, a promising route, interfacial solar vapor generation, has been proposed and developed to promote the photothermal conversion efficiency of liquid-gas phase transition of water, dramatically improving vapor production (Tao et al., 2018; Zhou et al., 2019; Vaartstra et al., 2020). The performance of solar vapor generation can be significantly enhanced by floating the absorber or evaporator at the water-air interface to evaporate the water at a relatively localized photothermal area (the liquid/air interface), which is therefore named interfacial solar vapor generation (Ghasemi et al., 2014; Wang et al., 2014). The rapid development of solar water treatment technologies has thus been essentially improved.
However, most of the proposed interfacial solar evaporators have been more or less suffering from the rapid decay of energy transfer efficiency and poor stability during the long-term operation of solar desalination (Li et al., 2020; Zhang Q. et al., 2020). Therefore, it is vital to inhibit salt deposition to maintain long-term solar vaporization. Traditional methods are rinsing and washing in the post-treatment process (Finnerty et al., 2017; Jin et al., 2018; Zhang et al., 2018; Xiao et al., 2019; Zhu et al., 2019). However, they introduced extra treatment costs and merely worked for washable and flexible membrane absorbers. In addition, by logically segregating the light-absorbing surface from the surface of salt precipitation, rationally designed absorbers can precisely monitor the position of salt precipitation to allow long-lasting, high-performance, and ZLD solar desalination (Shi et al., 2018; Zeng et al., 2019; Zhang et al., 2020b; Wu et al., 2020; Xia et al., 2020; Zhuang et al., 2020). However, the imperfections of the above methods are that the threat of salt accumulation on the absorber surface to light absorption and permeability can interrupt the generation of stable and high-efficiency steam in long-term desalination and highly concentrated brine.
Aiming to solve or alleviate the salt clogging issue, the researchers have developed a couple of effective strategies to develop stable solar desalination devices with anti-salt clogging properties. We evaluate the state-of-the-art solar interfacial desalination as shown in Figure 1. It illustrates that the reported anti-clogging mechanisms for solar interfacial systems can be categorized into four major groups: 1) fluid convection can dilute the formed high concentration brine in the absorbers during desalination; 2) Hydrophobic designs can prohibit the contact of salt ions with the solar absorber; 3) Thermal engineering by re-radiating infrared photons can entirely avoid fouling via the physical separation from the brine; 4) Donnan that can prevent ions upward movement by creating the double electrode layer.
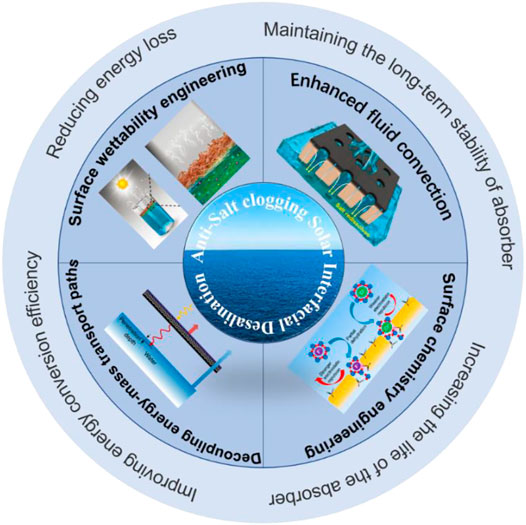
FIGURE 1. An overview of the anti-clogging interfacial solar desalination. The anti-clogging principle can be divided into four main categories: fluid convection, hydrophobic design, contactless solar evaporation, and the Donnan effect. Adapted from (Kuang et al., 2019). Adapted from (Xu et al., 2018). Adapted from (Cooper et al., 2018). Adapted from (Epsztein et al., 2018).
Enhanced Fluid Convection: Multiscale Hierarchical Structures
In the past years, various research groups have suggested that the primary issue that accounts for the salt accumulation is the emergence of the saturated salt solution on the solar absorber surface during the highly efficient interfacial solar desalination process. Therefore, it is of tremendous importance but rather challenging for rationally designing structures capable of high-flex ion diffusion. A variety of concepts have been proposed and demonstrated experimentally, including multi-scale precise manipulation of the structures of solar evaporators across the microscale to the macroscopic scale. One of the most representative strategies, reported by Chen group (Ni et al., 2018), is the regulation of micro/macro structures because of the rapid diffusion of ions through the micro/macro channels (Fang et al., 2019; He et al., 2019; Liu et al., 2019; Wang C. et al., 2020; Zhu et al., 2017). As shown in Figure 2A, in this salt-rejecting evaporator proposed by Chen et al., the hydrophilic white fabric is composed of microstructured pores and channels and artificially confined in macroscale narrow gaps among thermal insulation foams, which can finally wick the underlying water into the absorber layer. At the same time, the highly concentrated salt solution can be effectively diffused back into the remaining water. When the intriguing design integrated with a cost-effective polymer film condensation cover, the overall system can produce clean water at a rate of 2.5 L m2 per day at a total system cost of about $3 m2, which is sufficient to meet individual drinking requirements without energy framework while cheaper than conventional solar stills by order of magnitude, ideal for water-stressed and disaster-ridden populations with affordable drinking water. Similarly, inspired by banyan, a hierarchical evaporator consisting of an activated carbon-cotton fabric, a polyester pillar, and an expandable polyethylene foam was suggested by Zhang et al. (Zhang et al., 2020c). A banyan tree in nature grows with several aerial prop roots to meet the growth of plants, growing eventually into the soil for additional water availability. By modifying the amount of PPs during desalination, the suggested hierarchical evaporator was shown to exhibit anti-salt clogging ability.
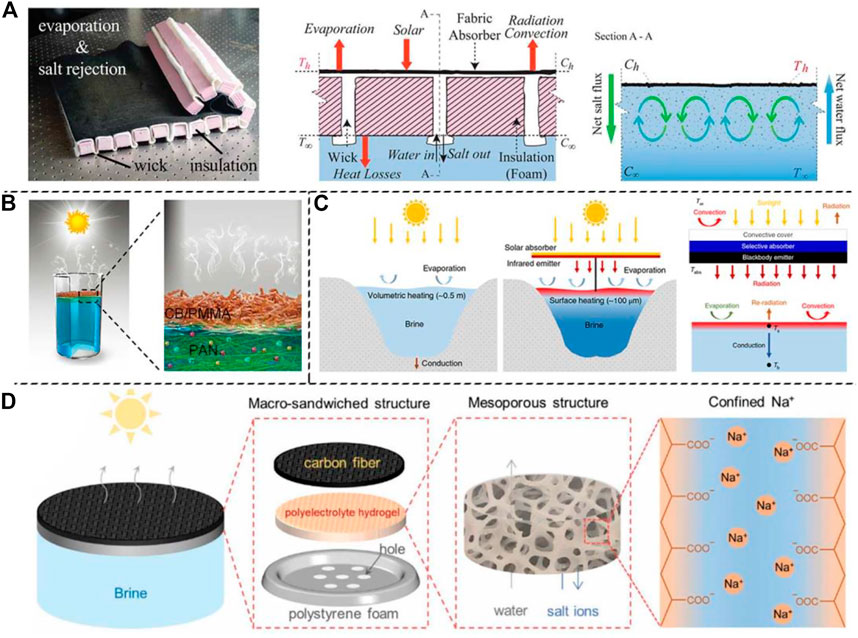
FIGURE 2. Salt diffusion is used to realize the salt-resistant solar desalination. (A) A floating solar salt-rejecting still for long-lasting and steady desalination. Schematic design of evaporation structure and advection of salt discharge. Adapted from (Ni et al., 2018). (B) Janus-structure based absorber for interfacial solar desalination processes. The schematic of a highly efficient solar steam generation system and a salt resistance strategy. Adapted from (Xu et al., 2018). (C) Surface heating using mid-infrared radiation (non-contacting heat localization). Schematic of a conventional evaporation pond and the proposed non-contact heat localization. Energy balance and heat transfer modes for the umbrella and water. Adapted from (Menon et al., 2020). (D) Salt-resistant solar desalination based on Donnan Effect. Adapted from (Zhao et al., 2021).
In addition, macro-pores of solar absorbers can also be utilized as efficient water channels to prevent salt deposition through enhanced fluid convection. Porous wood-based solar evaporators were reported by the Hu group (Zhu et al., 2017; He et al., 2019) for effective and safe high-salinity water desalination. The outstanding antifouling characteristics are due to its distinctive porous bimodal and 3D interconnected microstructure. During desalination, the rapid diffusion and evaporation of water and the capillary pumping of micro-channels in the system lead to a rapid re-supply of surface vaporized brine to maintain consistent and rapid production of water vapor. Further research has shown that a self-regenerating solar evaporator with outstanding anti-properties is recognized via a rationally designed virtual channel array in a natural wood substrate (Liu et al., 2018). Artificial polymer porous foams (Zhang et al., 2019a; Wang X. et al., 2020; Dong et al., 2020; He et al., 2020) with super-hydrophilic wettability have also been introduced. The sufficient water transport in these solar evaporation systems have been widely regarded to be beneficial to resolve the crystalline salt through enhancing fluid convection.
Surface Wettability Engineering: Heterogeneous Hydrophilicity
By enhancing fluid convection, the anti-salt evaporators cannot maintain efficient evaporation out of high-salinity brine (over 10 wt%) because of the excessive salt solubility on the solar absorbers during desalination. Hence, the effective way to prevent salt deposition on the absorber is to blocks water molecules while allowing steam. It is also noteworthy that the hydrophobic surface of a solar absorber can effectively prevent water while blocking salt ions from penetrating into the absorber and thus water evaporation happens at the bottom of the absorber (Gao S. et al., 2019; Chen et al., 2020; Peng et al., 2021). The hydrophobic surface of solar absorbers has been gradually recognized as salt-rejective solar evaporators by a couple of groups (Kashyap et al., 2017).
As illustrated in Figure 2B, Xu et al. (Xu et al., 2018) indicate that safe and effective solar desalination can be facilitated by a sequential electrospinning flexible Janus absorber. The membrane can be divided into two primary parts-the hydrophobic upper levels for salt-resistance and the porous and hydrophilic lower level for water supply. Benefiting from Janus’ unique structure, solar absorption and water pumping are split into various layers with polymethylmethacrylate (PMMA) coating of the hydrophobic upper carbon black nanoparticles (CB) for light absorption and the lower polyacrylonitrile (PAN) hydrophilic layer for water pumping. As a result, salt can only be accumulated in the PAN hydrophilic layer and easily dissolved due to consistent water pumping. High efficiency and steady water generation (1.3 kg m-2 h-1, over 16 days) under one Sun is shown by the Janus absorber, which has not been obtained in many previous absorbers. Similar Janus cotton fabrics with salt-rejection properties may also be reported by Lai et al. (Gao T. et al., 2019). However, the absorbers mentioned above are in direct contact with the volume of water, resulting in heat dissipation through the water. The vertically oriented Janus MXene aerogel with a hydrophobic upper layer and a hydrophilic lower layer was designed by Quan et al. (Zhang et al., 2019b). MXene, which has a theoretical light-to-heat conversion capability of 100 percent, can easily convert light to heat in combination with the Janus structure and protect the photothermal layer from direct bulk water interaction with the hydrophobic upper layer, thereby reducing heat loss and thus effectively inhibiting the crystallization of salt owing to its fast dissolution with continuous pumping water.
Among most Janus absorbers, the hydrophobic upper layer of the absorber was generally generated by manipulating with a polymer solution, which may block the steam escape pathways. An innovative double-layer hydrophilic/hydrophobic nonporous structure was designed by Que et al. (Yang et al., 2018). A porous hydrophobic layer can withstand salt deposition and supply pathways for vapor evaporation. Water vapor is generated and then lost through the stomata on the upper epidermis in the natural water lily. The hydrophobic surface is the root cause of the self-cleaning property. Xu et al. modeled a water lily-inspired hierarchical structure composed of a bottom bracket and a top solar absorber (Xu et al., 2019). Unlike most conventional structures of salt rejection, there was a thin water film at the interface between the bottom supporter and the top hydrophobic absorber. This sandwich structure plays a vital role in achieving safe and efficient high-concentration brine evaporation in this design. The formed salt particles or high salt solution gradually diffused to the bulk brine along the one-dimensional channel rather than accumulating in the absorber during desalination.
Decoupling Energy-Mass Transport Paths: Non-Contacting Optical Heating by Thermal Emission Engineering
As in most of interfacial solar evaporation systems, mass transport and thermal energy transfer are basically existing in the same channel, in which case the high evaporation rate is accompanied with more salt reservation on the absorbers. The deposition of salt on the top surface of solar absorbers typically results in lower stability and efficiency degradation, which is difficult to prevent due to the direct interaction of the solar absorber with saline. Hence, the physical separation of the absorbers from the brine is an effective way to prevent salt deposition caused by evaporation. Non-contact solar interfacial evaporation is a new type of evaporation structure that fouling is entirely avoided. The brine absorbs solar radiation and transfers the heat from solar to the brine to generate steam by infrared radiation (Bian et al., 2020). Thomas A. Cooper et al. recently described a structure that can absorb solar radiation and re-radiate infrared photons absorbed directly by water within a penetration depth of sub-100 μm (Cooper et al., 2018). Due to thermal isolation, the structure is no longer modified at the boiling point and is used to overheat the steam generated. Steam was produced at temperatures up to 133°C, showing superheated steam in a single sunlight system in a non-pressurized system. Akanksha K. Menon et al. (Menon et al., 2020) recently used a photothermal system that converts sunlight into mid-infrared radiation where water is significantly absorbed to increase evaporation by more than 100 percent by a passive and non-contact procedure (Figure 2C). Apart from traditional evaporation ponds, heat is located by radiative coupling at the water’s surface, leading to better use of solar energy with 43% conversion efficiency. The non-contact design of the system makes it ideally suited for treating a wide variety of uncontaminated wastewater. The usage of industrial materials facilitates relatively cheap and highly efficient technology for effective wastewater management, with the additional advantage of the salt recovery.
Surface Chemistry Engineering: Donnan Effect for Pre-Intercalation of Salt Ions
As discussed in the previous part, the deposition of salt is mainly due to the increasing concentration of salt on the top surface of the solar absorber. The regulation of the local salt concentration in the absorbers is an effective and desirable way to avoid salt deposition. The salt ion has positive/negative charges on the top surface of the solar absorber. Hence, the key is to regulate the number of salt ions in the absorbers according to the boundary-layer differential equation for mass transfer (Pantoja et al., 2015). Donnan effect can prevent ions upward movement by creating a double electrode layer due to electric neutrality, which can be a good way for enhanced anti-fouling performance (Chang and Kaplan, 1977; Cumbal and SenGupta, 2005; Galama et al., 2013; Ma et al., 2020).
Depending on the pH of the polyamide NF membrane, the rejection behavior of sodium cation-containing ternary ion solutions (Na+) and two monovalent anions has been consistently examined by Razi Epsztein et al. (Epsztein et al., 2018). The Donnan (charge) exclusion mechanism in the NF is more likely to affect anion with a smaller ion radius and a relatively high charge density. Membranes based on graphene oxide (GO)-based suffer from either low water flow or low ion rejection when employed for desalination. Chengzhi Hu et al. (Hu et al., 2018) successfully created an electroconductive three-dimensional hybrid membrane by employing reduced GO and carbon nanotubes (GCN) and showed significant ability to overcome the tradeoff between selectivity and permeability when performed concurrently as a philter membrane and electrode. In addition to developing various water transport channels to facilitate permeability, the intercalation of carbon nanotubes (CNTs) in the reduced GO matrix has also improved the active adsorption sites for salt ions, contributing to an improved Donnan effect and extraordinary salt rejection. An exceptional NaCl rejection of 71 percent was accomplished by the optimized GCN (containing 15 percent CNTs), three times higher than without bias. As shown in Figure 2D, Zhu et al. recently described a hierarchically designed evaporator with salt-resistant ability based on the Donnan effect (Zhao et al., 2021). Due to the Donnan distribution equilibrium, this structure can minimize the number of salt ions in the absorber while supplying water. This hierarchically designed evaporator is shown by high efficiency (80%) and steady water generation under one Sun in high-salinity brine (15 wt% NaCl).
Conclusions and Outlook
Interfacial solar desalination has been intensely pursued as the most effective method for obtaining cleaner freshwater, attributing to an intrinsic low cost, high conversion efficiency, and eco-friendliness. However, the accumulated salt on the absorbers during desalination blocks continues the solar energy input and the vapor escape channels, leading to a significant decrease in the steam yield. In response to this problem, tremendous efforts have been dedicated to developing solar interfacial evaporators with an antifouling performance for long-term and stable desalination. This mini review summarized different solutions for inhibiting the salt deposition on absorbers’ surfaces, including enhancing fluid convection, surface wettability engineering, decoupling energy-mass transport paths, and surface chemistry engineering. These researches are of paramount importance in improving our insight into the development of stable and continuous desalination.
There is still a wide gap between the current strategy and the practical uses due to the complicated process of crystalline salts. Therefore, the deep study still needs more effort in future studies. 1) The yield of freshwater is critical for practical application in the solar interfacial desalination process. However, most of the previous devices still focus on the steam yields rather than the water yield to date. Therefore, the high yield of freshwater from seawater is still a fundamental challenge in solar interfacial desalination. 2) Based on the deep understanding of the principles of water transport, salt crystallization, evaporation, continuous steam production, and solid salts harvesting were proposed (Xia et al., 2019; Shao et al., 2020; Xia et al., 2020; Xu et al., 2021), which provides a new direction in further research. Hence, the collection of salt or chemicals of economic value should be consecrated deserves some attention in the process of solar interfacial desalination. 3) Regarding the current solar interfacial desalination devices, the main obstacle to solar interfacial desalination in practical uses is commercial-scale manufacturing.
Author Contributions
All authors listed have made substantial, direct, and intellectual contribution to the work and approved it for publication.
Funding
This work is jointly supported by the National Natural Science Foundation of China (Nos. 61735008, 12022403, 51925204) and National Key Research and Development Program of China (Nos. 2017YFA0403600, 2017YFA0205700) and the Fundamental Research Funds for the Central Universities (Nos. 021314380150, 021314380140).
Conflict of Interest
The authors declare that the research was conducted in the absence of any commercial or financial relationships that could be construed as a potential conflict of interest.
The reviewer XW declared a shared affiliation, with no collaboration, with the authors to the handling editor at the time of review.
Publisher’s Note
All claims expressed in this article are solely those of the authors and do not necessarily represent those of their affiliated organizations, or those of the publisher, the editors and the reviewers. Any product that may be evaluated in this article, or claim that may be made by its manufacturer, is not guaranteed or endorsed by the publisher.
References
Abdel-Rehim, Z. S., and Lasheen, A. (2005). Improving the Performance of Solar Desalination Systems. Renew. Energ. 30, 1955–1971. doi:10.1016/j.renene.2005.01.008
Bian, Y., Tian, Y., Tang, K., Li, W., Zhao, L., Yang, Y., et al. (2020). Sustainable Solar Evaporation from Solute Surface via Energy Downconversion. Glob. Challenges 5, 2000077. doi:10.1002/gch2.202000077
Cetrulo, T. B., Marques, R. C., and Malheiros, T. F. (2019). An Analytical Review of the Efficiency of Water and Sanitation Utilities in Developing Countries. Water Res. 161, 372–380. doi:10.1016/j.watres.2019.05.044
Chang, R., and Kaplan, L. J. (1977). The Donnan Equilibrium and Osmotic Pressure. J. Chem. Educ. 54, 218. doi:10.1021/ed054p218
Chen, J., Yin, J. L., Li, B., Ye, Z., Liu, D., Ding, D., et al. (2020). Janus Evaporators with Self-Recovering Hydrophobicity for Salt-Rejecting Interfacial Solar Desalination. ACS Nano 14, 17419–17427. doi:10.1021/acsnano.0c07677
Cooper, T. A., Zandavi, S. H., Ni, G. W., Tsurimaki, Y., Huang, Y., Boriskina, S. V., et al. (2018). Contactless Steam Generation and Superheating Under One Sun Illumination. Nat. Commun. 9, 5086. doi:10.1038/s41467-018-07494-2
Cumbal, L., and SenGupta, A. K. (2005). Arsenic Removal Using Polymer-Supported Hydrated Iron(III) Oxide Nanoparticles: Role of Donnan Membrane Effect†. Environ. Sci. Technol. 39, 6508–6515. doi:10.1021/es050175e
Dobson, D. P., Meredith, P. G., and Boon, S. A. (2002). Simulation of Subduction Zone Seismicity by Dehydration of Serpentine. Science 298, 1407–1410. doi:10.1126/science.1075390
Dong, X., Cao, L., Si, Y., Ding, B., and Deng, H. (2020). Cellular Structured CNTs@SiO 2 Nanofibrous Aerogels with Vertically Aligned Vessels for Salt‐Resistant Solar Desalination. Adv. Mater. 32, 1908269. doi:10.1002/adma.201908269
Elimelech, M., and Phillip, W. A. (2011). The Future of Seawater Desalination: Energy, Technology, and the Environment. Science 333, 712–717. doi:10.1126/science.1200488
Epsztein, R., Shaulsky, E., Dizge, N., Warsinger, D. M., and Elimelech, M. (2018). Role of Ionic Charge Density in Donnan Exclusion of Monovalent Anions by Nanofiltration. Environ. Sci. Technol. 52, 4108–4116. doi:10.1021/acs.est.7b06400
Fang, Q., Li, T., Lin, H., Jiang, R., and Liu, F. (2019). Highly Efficient Solar Steam Generation from Activated Carbon Fiber Cloth with Matching Water Supply and Durable Fouling Resistance. ACS Appl. Energ. Mater. 2, 4354–4361. doi:10.1021/acsaem.9b00562
Finnerty, C., Zhang, L., Sedlak, D. L., Nelson, K. L., and Mi, B. (2017). Synthetic Graphene Oxide Leaf for Solar Desalination with Zero Liquid Discharge. Environ. Sci. Technol. 51, 11701–11709. doi:10.1021/acs.est.7b03040
Galama, A. H., Post, J. W., Cohen Stuart, M. A., and Biesheuvel, P. M. (2013). Validity of the Boltzmann Equation to Describe Donnan Equilibrium at the Membrane-Solution Interface. J. Membr. Sci. 442, 131–139. doi:10.1016/j.memsci.2013.04.022
Gao, S., Dong, X., Huang, J., Dong, J., Maggio, F. D., Wang, S., et al. (2019a). Bioinspired Soot‐Deposited Janus Fabrics for Sustainable Solar Steam Generation with Salt‐Rejection. Glob. Challenges 3, 1800117. doi:10.1002/gch2.201800117
Gao, T., Li, Y., Chen, C., Yang, Z., Kuang, Y., Jia, C., et al. (2019b). Architecting a Floatable, Durable, and Scalable Steam Generator: Hydrophobic/Hydrophilic Bifunctional Structure for Solar Evaporation Enhancement. Small Methods 3, 1800176. doi:10.1002/smtd.201800176
Ghasemi, H., Ni, G., Marconnet, A. M., Loomis, J., Yerci, S., Miljkovic, N., et al. (2014). Solar Steam Generation by Heat Localization. Nat. Commun. 5, 4449. doi:10.1038/ncomms5449
Gude, V. G. (2011). Energy Consumption and Recovery in Reverse Osmosis. Desalination Water Treat. 36, 239–260. doi:10.5004/dwt.2011.2534
He, J., Zhang, Z., Xiao, C., Liu, F., Sun, H., Zhu, Z., et al. (2020). High-Performance Salt-Rejecting and Cost-Effective Superhydrophilic Porous Monolithic Polymer Foam for Solar Steam Generation. ACS Appl. Mater. Inter. 12, 16308–16318. doi:10.1021/acsami.9b22832
He, S., Chen, C., Kuang, Y., Mi, R., Liu, Y., Pei, Y., et al. (2019). Nature-Inspired Salt Resistant Bimodal Porous Solar Evaporator for Efficient and Stable Water Desalination. Energy Environ. Sci. 12, 1558–1567. doi:10.1039/C9EE00945K
Hu, C., Liu, Z., Lu, X., Sun, J., Liu, H., and Qu, J. (2018). Enhancement of the Donnan Effect Through Capacitive Ion Increase Using an Electroconductive rGO-CNT Nanofiltration Membrane. J. Mater. Chem. A. 6, 4737–4745. doi:10.1039/C7TA11003K
Jin, Y., Chang, J., Shi, Y., Shi, L., Hong, S., and Wang, P. (2018). A Highly Flexible and Washable Nonwoven Photothermal Cloth for Efficient and Practical Solar Steam Generation. J. Mater. Chem. A. 6, 7942–7949. doi:10.1039/C8TA00187A
Kalogirou, S. (1997). Survey of Solar Desalination Systems and System Selection. Energy 22, 69–81. doi:10.1016/S0360-5442(96)00100-4
Kashyap, V., Al-Bayati, A., Sajadi, S. M., Irajizad, P., Wang, S. H., and Ghasemi, H. (2017). A Flexible Anti-Clogging Graphite Film for Scalable Solar Desalination by Heat Localization. J. Mater. Chem. A. 5, 15227–15234. doi:10.1039/C7TA03977H
Kuang, Y., Chen, C., He, S., Hitz, E. M., Wang, Y., Gan, W., et al. (2019). A High‐Performance Self‐Regenerating Solar Evaporator for Continuous Water Desalination. Adv. Mater. 31, 1900498. doi:10.1002/adma.201900498
Li, H., Yan, Z., Li, Y., and Hong, W. (2020). Latest Development in Salt Removal from Solar-Driven Interfacial saline Water Evaporators: Advanced Strategies and Challenges. Water Res. 177, 115770. doi:10.1016/j.watres.2020.115770
Liu, H., Chen, C., Wen, H., Guo, R., Williams, N. A., Wang, B., et al. (2018). Narrow Bandgap Semiconductor Decorated wood Membrane for High-Efficiency Solar-Assisted Water Purification. J. Mater. Chem. A. 6, 18839–18846. doi:10.1039/C8TA05924A
Liu, Y., Liu, Z., Huang, Q., Liang, X., Zhou, X., Fu, H., et al. (2019). A High-Absorption and Self-Driven Salt-Resistant Black Gold Nanoparticle-Deposited Sponge for Highly Efficient, Salt-free, and Long-Term Durable Solar Desalination. J. Mater. Chem. A. 7, 2581–2588. doi:10.1039/C8TA10227A
Ma, X., Fang, W., Ying, W., Chen, D., Li, Z., Deng, Z., et al. (2020). A Robust Asymmetric Porous SWCNT/Gelatin Thin Membrane with Salt-Resistant for Efficient Solar Vapor Generation. Appl. Mater. Today 18, 100459. doi:10.1016/j.apmt.2019.100459
Menon, A. K., Haechler, I., Kaur, S., Lubner, S., and Prasher, R. S. (2020). Enhanced Solar Evaporation Using a Photo-Thermal Umbrella for Wastewater Management. Nat. Sustain. 3, 144–151. doi:10.1038/s41893-019-0445-5
Ni, G., Zandavi, S. H., Javid, S. M., Boriskina, S. V., Cooper, T. A., and Chen, G. (2018). A Salt-Rejecting Floating Solar Still for Low-Cost Desalination. Energ. Environ. Sci. 11, 1510–1519. doi:10.1039/C8EE00220G
Pantoja, C. E., Nariyoshi, Y. N., and Seckler, M. M. (2015). Membrane Distillation Crystallization Applied to Brine Desalination: A Hierarchical Design Procedure. Ind. Eng. Chem. Res. 54, 2776–2793. doi:10.1021/ie504695p
Peng, F., Xu, J., Bai, X., Feng, G., Zeng, X., Ibn Raihan, M. R., et al. (2021). A Janus Solar Evaporator with 2D Water Path for Highly Efficient Salt-Resisting Solar Steam Generation. Solar Energ. Mater. Solar Cell 221, 110910. doi:10.1016/j.solmat.2020.110910
Qiblawey, H. M., and Banat, F. (2008). Solar thermal Desalination Technologies. Desalination 220, 633–644. doi:10.1016/j.desal.2007.01.059
Shannon, M. A., Bohn, P. W., Elimelech, M., Georgiadis, J. G., Mariñas, B. J., and Mayes, A. M. (2008). Science and Technology for Water Purification in the Coming Decades. Nature 452, 301–310. doi:10.1038/nature06599
Shao, Y., Tang, J., Li, N., Sun, T., Yang, L., Chen, D., et al. (2020). Designing a Bioinspired Synthetic Tree by Unidirectional Freezing for Simultaneous Solar Steam Generation and Salt Collection. EcoMat 2, e12018. doi:10.1002/eom2.12018
Shi, Y., Zhang, C., Li, R., Zhuo, S., Jin, Y., Shi, L., et al. (2018). Solar Evaporator with Controlled Salt Precipitation for Zero Liquid Discharge Desalination. Environ. Sci. Technol. 52, 11822–11830. doi:10.1021/acs.est.8b03300
Tao, P., Ni, G., Song, C., Shang, W., Wu, J., Zhu, J., et al. (2018). Solar-Driven Interfacial Evaporation. Nat. Energ. 3, 1031–1041. doi:10.1038/s41560-018-0260-7
Vaartstra, G., Zhang, L., Lu, Z., Díaz-Marín, C. D., Grossman, J. C., and Wang, E. N. (2020). Capillary-Fed, Thin Film Evaporation Devices. J. Appl. Phys. 128, 130901. doi:10.1063/5.0021674
Wang, C., Wang, J., Li, Z., Xu, K., Lei, T., and Wang, W. (2020a). Superhydrophilic Porous Carbon Foam as a Self-Desalting Monolithic Solar Steam Generation Device with High Energy Efficiency. J. Mater. Chem. A. 8, 9528–9535. doi:10.1039/D0TA01439G
Wang, X., Gan, Q., Chen, R., Peng, H., Zhang, T., and Ye, M. (2020b). Water Delivery Channel Design in Solar Evaporator for Efficient and Durable Water Evaporation with Salt Rejection. ACS Sustain. Chem. Eng. 8, 7753–7761. doi:10.1021/acssuschemeng.9b06844
Wang, Z., Liu, Y., Tao, P., Shen, Q., Yi, N., Zhang, F., et al. (2014). Bio-Inspired Evaporation Through Plasmonic Film of Nanoparticles at the Air-Water Interface. Small 10, 3234–3239. doi:10.1002/smll.201401071
Wu, L., Dong, Z., Cai, Z., Ganapathy, T., Fang, N. X., Li, C., et al. (2020). Highly Efficient Three-Dimensional Solar Evaporator for High Salinity Desalination by Localized Crystallization. Nat. Commun. 11, 521. doi:10.1038/s41467-020-14366-1
Xia, Y., Hou, Q., Jubaer, H., Li, Y., Kang, Y., Yuan, S., et al. (2019). Spatially Isolating Salt Crystallisation from Water Evaporation for Continuous Solar Steam Generation and Salt Harvesting. Energ. Environ. Sci. 12, 1840–1847. doi:10.1039/C9EE00692C
Xia, Y., Li, Y., Yuan, S., Kang, Y., Jian, M., Hou, Q., et al. (2020). A Self-Rotating Solar Evaporator for Continuous and Efficient Desalination of Hypersaline Brine. J. Mater. Chem. A. 8, 16212–16217. doi:10.1039/D0TA04677A
Xiao, P., Gu, J., Zhang, C., Ni, F., Liang, Y., He, J., et al. (2019). A Scalable, Low-Cost and Robust Photo-Thermal Fabric with Tunable and Programmable 2D/3D Structures Towards Environmentally Adaptable Liquid/Solid-Medium Water Extraction. Nano Energy 65, 104002. doi:10.1016/j.nanoen.2019.104002
Xu, N., Li, J., Wang, Y., Fang, C., Li, X., Wang, Y., et al. (2019). A Water Lily-Inspired Hierarchical Design for Stable and Efficient Solar Evaporation of High-Salinity Brine. Sci. Adv. 5, eaaw7013. doi:10.1126/sciadv.aaw7013
Xu, N., Zhang, H., Lin, Z., Li, J., Liu, G., Li, X., et al. (2021). A Scalable Fish-School Inspired Self-Assembled Particle System for Solar-Powered Water-Solute Separation. Natl. Sci. Rev., nwab065. doi:10.1093/nsr/nwab065
Xu, W., Hu, X., Zhuang, S., Wang, Y., Li, X., Zhou, L., et al. (2018). Flexible and Salt Resistant Janus Absorbers by Electrospinning for Stable and Efficient Solar Desalination. Adv. Energ. Mater. 8, 1702884. doi:10.1002/aenm.201702884
Yang, Y., Zhao, H., Yin, Z., Zhao, J., Yin, X., Li, N., et al. (2018). A General Salt-Resistant Hydrophilic/Hydrophobic Nanoporous Double Layer Design for Efficient and Stable Solar Water Evaporation Distillation. Mater. Horiz. 5, 1143–1150. doi:10.1039/C8MH00386F
Zeng, J., Wang, Q., Shi, Y., Liu, P., and Chen, R. (2019). Osmotic Pumping and Salt Rejection by Polyelectrolyte Hydrogel for Continuous Solar Desalination. Adv. Energ. Mater. 9, 1900552. doi:10.1002/aenm.201900552
Zhang, Q., Hu, R., Chen, Y., Xiao, X., Zhao, G., Yang, H., et al. (2020a). Banyan-Inspired Hierarchical Evaporators for Efficient Solar Photothermal Conversion. Appl. Energ. 276, 115545. doi:10.1016/j.apenergy.2020.115545
Zhang, Q., Xiao, X., Wang, G., Ming, X., Liu, X., Wang, H., et al. (2018). Silk-Based Systems for Highly Efficient Photothermal Conversion Under One Sun: Portability, Flexibility, and Durability. J. Mater. Chem. A. 6, 17212–17219. doi:10.1039/C8TA05193C
Zhang, Q., Yang, H., Xiao, X., Wang, H., Yan, L., Shi, Z., et al. (2019a). A New Self-Desalting Solar Evaporation System Based on a Vertically Oriented Porous Polyacrylonitrile Foam. J. Mater. Chem. A. 7, 14620–14628. doi:10.1039/C9TA03045J
Zhang, Q., Yi, G., Fu, Z., Yu, H., Chen, S., and Quan, X. (2019b). Vertically Aligned Janus MXene-Based Aerogels for Solar Desalination with High Efficiency and Salt Resistance. ACS Nano 13, 13196–13207. doi:10.1021/acsnano.9b06180
Zhang, Y., Xiong, T., Nandakumar, D. K., and Tan, S. C. (2020b). Structure Architecting for Salt‐Rejecting Solar Interfacial Desalination to Achieve High‐Performance Evaporation with In Situ Energy Generation. Adv. Sci. 7, 1903478. doi:10.1002/advs.201903478
Zhang, Y., Zhang, H., Xiong, T., Qu, H., Koh, J. J., Nandakumar, D. K., et al. (2020c). Manipulating Unidirectional Fluid Transportation to Drive Sustainable Solar Water Extraction and Brine-Drenching Induced Energy Generation. Energ. Environ. Sci. 13, 4891–4902. doi:10.1039/D0EE01572E
Zhao, W., Gong, H., Song, Y., Li, B., Xu, N., Min, X., et al. (2021). Hierarchically Designed Salt‐Resistant Solar Evaporator Based on Donnan Effect for Stable and High‐Performance Brine Treatment. Adv. Funct. Mater. 31, 2100025. doi:10.1002/adfm.202100025
Zhou, L., Li, X., Ni, G. W., Zhu, S., and Zhu, J. (2019). The Revival of Thermal Utilization from the Sun: Interfacial Solar Vapor Generation. Natl. Sci. Rev. 6, 562–578. doi:10.1093/nsr/nwz030
Zhu, B., Kou, H., Liu, Z., Wang, Z., Macharia, D. K., Zhu, M., et al. (2019). Flexible and Washable CNT-Embedded PAN Nonwoven Fabrics for Solar-Enabled Evaporation and Desalination of Seawater. ACS Appl. Mater. Inter. 11, 35005–35014. doi:10.1021/acsami.9b12806
Zhu, M., Li, Y., Chen, G., Jiang, F., Yang, Z., Luo, X., et al. (2017). Tree-Inspired Design for High-Efficiency Water Extraction. Adv. Mater. 29, 1704107. doi:10.1002/adma.201704107
Keywords: photothermal, desalination, solar interfacial evaporation, anti-salt clogging, structure design
Citation: Yu X, Zhang Q, Liu X, Xu N and Zhou L (2021) Salt-Resistive Photothermal Materials and Microstructures for Interfacial Solar Desalination. Front. Energy Res. 9:721407. doi: 10.3389/fenrg.2021.721407
Received: 07 June 2021; Accepted: 26 July 2021;
Published: 17 August 2021.
Edited by:
Xiuqiang Li, Duke University, United StatesReviewed by:
Xueyang Wang, Nanjing University, ChinaShendong Zhuang, Shenzhen University, China
Yang Li, Hong Kong University of Science and Technology, Hong Kong, SAR China
Copyright © 2021 Yu, Zhang, Liu, Xu and Zhou. This is an open-access article distributed under the terms of the Creative Commons Attribution License (CC BY). The use, distribution or reproduction in other forums is permitted, provided the original author(s) and the copyright owner(s) are credited and that the original publication in this journal is cited, in accordance with accepted academic practice. No use, distribution or reproduction is permitted which does not comply with these terms.
*Correspondence: Xiaoqiang Yu, eHF5dUBzZXUuZWR1LmNu; Lin Zhou, bGluemhvdUBuanUuZWR1LmNu
†These authors have contributed equally to this work