- Key Laboratory for Ultrafine Materials of Ministry of Education, Shanghai Engineering Research Center of Hierarchical Nanomaterials, School of Materials Science and Engineering, East China University of Science and Technology, Shanghai, China
Cesium-based all-inorganic perovskite absorbers have attracted increasing attention due to their superior thermal stability, compared to their organic–inorganic counterparts. Up to now, it is a challenge to fabricate high-efficiency all-inorganic perovskite solar cells (PSCs) with low defect densities. Herein, we used bivalent metal chloride salts (SrCl2 and NiCl2) to optimize CsPbI2Br films. The experimental results indicate that this method could deliver high-quality films with improved electronic property. As a result, the champion device based on the 0.01 M SrCl2–doped CsPbI2Br film achieved a power conversion efficiency (PCE) of 16.07% with a high open voltage (VOC) of 1,322 mV, which is about 18% higher than that of the pristine device.
Introduction
Lead halide perovskites are promising photovoltaic materials because of their tunable bandgap, long carrier diffusion length, and easy fabrication (Chen et al., 2015; Shi et al., 2015; Lin et al., 2018; Yang et al., 2019a; Qiao et al., 2019; Fang et al., 2020; Qiao et al., 2020). Unfortunately, the long-term instability of perovskites, especially under thermal conditions, limits the commercial application of PSCs (Yang et al., 2019b; Zhong et al., 2020). All-inorganic cesium lead halide perovskites (CsPbX3, X = Cl, Br, I) have been developed quickly in recent years, whose PCEs have reached 19% with benign thermal endurance (Bai et al., 2018; Wang et al., 2019a; Wang et al., 2019b; Straus et al., 2019; Tan et al., 2019). The CsPbI3 perovskite has a low bandgap of ∼1.73 eV but suffers from the intrinsic structural transition from the α-phase to the δ-phase at room temperature (Li et al., 2018; Zeng et al., 2019).
Partial substitution of iodine for bromine anions can effectively stabilize the perovskite structure by tailoring the tolerant factor (Rehman et al., 2017; Fu et al., 2019; Dong et al., 2020). A paradigm is the CsPbI2Br perovskite with a bandgap of ∼1.9 eV and a stable cubic phase at room temperature (Sutton et al., 2016; Yan et al., 2018). However, the PCEs of CsPbI2Br solar cells were still much lower than those of CsPbI3 devices. Controlling the deposition process of perovskite thin films could optimize film coverage at a long-range scale, as well as reducing the atomic crystal defects (Chen et al., 2019; Duan et al., 2020; Liu et al., 2020). Processing parameters, such as coating speed and annealing temperature, have been initially studied in previous reports (Yu and Gao, 2017; Zhang et al., 2020a). Besides, additives can selectively adsorb on the perovskite surface and regulate perovskite crystallization (Zhang et al., 2019). A number of organic additives, such as dimethylammonium iodide, choline iodine, and dithiocarbamate, have been used to achieve high-quality perovskite films (Yang et al., 2019c; Fu et al., 2020; He et al., 2020). However, inorganic salts have been less investigated in this field, particularly for optimizing the film morphology and electronic property of all-inorganic perovskites (Wang et al., 2019c; Zhang et al., 2019). Bivalent metal salts are commonly used as inorganic additives to improve films’ quality, which is suitable for different organic–inorganic perovskite formulations (Aydin et al., 2019; Wang et al., 2019a). ZnI2 (Shai et al., 2018) and SnI2 (Eperon and Ginger, 2017) have been incorporated into organic–inorganic perovskites, which could enhance PCEs of the corresponding PSCs attributed to increased grain size and crystallinity. In addition, the Cl− ion could passivate grain boundaries and trap defects of perovskite polycrystalline films (Aydin et al., 2019).
Here, we studied the effect of bivalent metal chloride salts (SrCl2 and NiCl2) on the film quality of the CsPbI2Br perovskite. We revealed that certain amount of metal chloride salts is substantially beneficial for the formation of the perovskite with less trap sites, while excess metal chloride salts are detrimental to the perovskite films. By utilizing SrCl2 dopants, the best planar heterojunction CsPbI2Br perovskite solar cell achieved a high PCE of 16.07%, with ∼18% increment than the pristine one.
Experiment
Material Preparation
Titanium(IV) chloride (TiCl4, 99%), lead iodide (PbI2, 99%), cesium iodide (CsI, 99.9%), lead bromide (PbBr2, 98%), bis(trifluoromethane)sulfonimide lithium salt (Li-TFSI, 99.95%), 4-tert-butylpyridine (tBP, 96%), dimethyl sulfoxide (DMSO, 99.8%), and chlorobenzene (99.9%) were purchased from Sigma-Aldrich. Strontium chloride hexahydrate (SrCl2·6H2O, 99.9%) and nickel chloride hexahydrate (NiCl2·6H2O, 99.9%) were purchased from Sinopharm Chemical Reagent Co., Ltd. Poly(3-hexylthiophene-2,5-diyl) (P3HT) was purchased from Xi’an Polymer Light Technology Corp. All the chemicals and solvents were used as received without further purification.
Device Fabrication
Fluorine-doped tin oxide (FTO) glasses (Nippon Sheet Glass, 8 Ω/square) were cleaned with soap, acetone, ethanol, and deionized water sequentially for 15 min, respectively. Then, the cleaned FTO glass substrates were treated by a plasma cleaner with O2 gas for 3 min at middle power radio frequency (10.2 W). Then, the substrates were immersed in a 25 mM TiCl4 aqueous solution for 60 min at 70°C and washed with distilled water and ethanol, followed by annealing at 500°C for 60 min in a muffle oven to form a compact TiO2 blocking layer. The preparation of CsPbI2Br films was based on a modified method according to the reported article (Liu et al., 2018). P3HT solution (15 mg/ml in chlorobenzene) as a hole transport layer (HTL) was spin-coated on perovskite films at 2,500 rpm for 30 s, followed by annealing at 120°C for 10 min. Finally, 100 nm-thick Ag was thermally evaporated onto the HTL as a metal electrode. All steps were carried out in a nitrogen glove box (H2O < 0.01 ppm, O2 < 0.01 ppm).
Characterization
Field emission scanning electron microscopy (FESEM, HITACHI S4800) was used to characterize the morphology of films. X-ray diffraction (XRD) patterns were acquired by powder X-ray diffraction (PXRD, Bruker Advance D8 X-ray diffractometer Cu Kα radiation, 40 kV). Ultraviolet and visible (UV-Vis) absorption spectrometry was collected using a Cary 500 UV-Vis-NIR spectrophotometer. Photoluminescence (PL) spectra were characterized at room temperature by exciting the sample deposited on the FTO substrate. The excitation wavelength of the Fluorolog-3-p spectrophotometer is 380 nm. The solar cells were illuminated by a solar light simulator (Solar IV-150A, Zolix), and light intensity was calibrated by a standard Newport calibrated KG5-filtered Si reference cell. Current density–voltage (J–V) curves of solar cells were measured by a Keithley 2400 digital source meter under simulated AM 1.5G illumination of 100 mW cm−2 with a reverse scan rate of 0.15 V s−1. Solar cells were masked with a metal aperture to define the active area of 0.0625 cm2. External quantum efficiency (EQE) spectra of solar cells were measured by an SCS10-X150-HBSD system. The electrochemical impedance spectra (EIS) were acquired under an applied voltage of 0–1 V with frequency ranging from 1 Hz to 1 MHz in dark condition. In transient photovoltage (TPV) decay tests, the cells were connected directly in series with an oscilloscope (DSOX3104T), and the input impedance of DSO was 106 Ω. An attenuated green laser pulse (DSP-532-A Laser) was used as a small perturbation to the background illumination on the device. The laser-pulse–induced photovoltage variation and the VOC are produced by the background illumination. The wavelength of the laser was 532 nm, the repeating frequency was about 10 Hz, and the pulse width was less than 10 ns.
Results and Discussion
The deposition of CsPbI2Br perovskites is based on the spin-coating of perovskite precursors dissolved in DMSO solution. We firstly included SrCl2 into perovskite precursor solution in the concentrations of 0.005, 0.01, and 0.05 M, respectively. The morphological characteristics of CsPbI2Br films were firstly visualized by SEM characterizations. As shown in Figure 1A, the pristine film presents a non-flat surface with a high coverage. By the addition of 0.005–0.01 M SrCl2, the CsPbI2Br films retained the fully covered morphology (Figures 1B,C). For the film with 0.05 M SrCl2 (Figure 1D), a rough surface with many pinholes was observed, implying the adverse effect of excess SrCl2 on film formation.
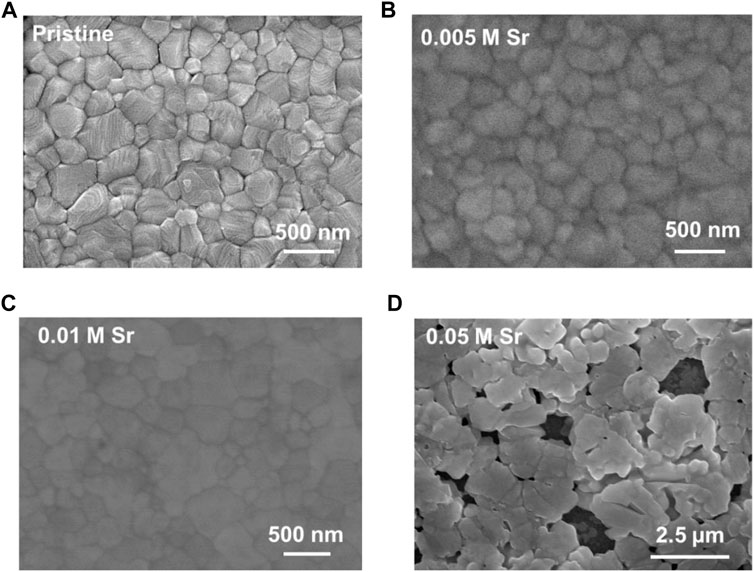
FIGURE 1. Top-view SEM images of (A) pristine, (B) 0.005 M SrCl2–doped, (C) 0.01 M SrCl2–doped, and (D) 0.05 M SrCl2–doped CsPbI2Br films.
We also noted that the 0.05 M SrCl2–doped films degraded rapidly in ambient condition. XRD patterns in Figure 2A disclose the non-perovskite phase of the doped film with 0.05 M SrCl2. Although the ionic radius of Sr2+ (118 pm) is similar to that of Pb2+ (119 pm) (Shannon, 1976), we still infer that excess metal dopants would favor the formation of non-perovskite phases (Phung et al., 2020). An intriguing phenomenon is that the full width at half maximum (FWHM) of the (100) diffraction peak reduces from 0.161 of pristine to 0.139 of film with 0.01 M SrCl2. The narrowed XRD diffraction peak signifies the enhanced crystallinity with less crystal defects (Li et al., 2020). Therefore, we conclude that the SrCl2 additive is a double-edged sword for the perovskite: low concentration of SrCl2 can promote the formation, while high concentration can generate the non-perovskite structure. The identical trend was also observed in the UV-Vis spectra in Figure 2B.
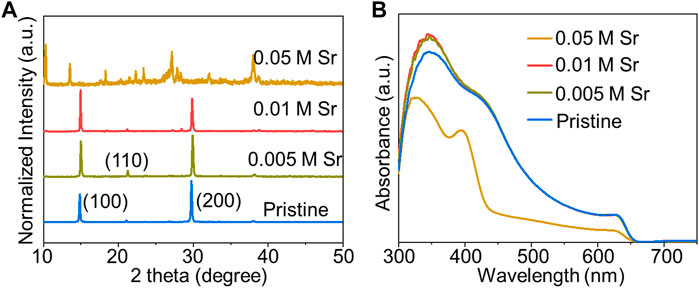
FIGURE 2. (A) XRD patterns and (B) UV-Vis spectra of CsPbI2Br films with different dopant concentrations of SrCl2 deposited on compact TiO2/FTO.
To further examine the effect of bivalent metal chlorides on CsPbI2Br perovskite films, we used NiCl2 as an additive into perovskite films. The concentration of NiCl2 is 0.01 M for all samples, which corresponds to 0.83% of the molar ratio with Pb ions. As expected, the NiCl2-doped sample exhibits smooth and uniform morphology (Figure 3). XRD patterns in Figure 4A further ascertain the cubic perovskite phase of all samples. Absorbance offsets of films were determined to be ∼650 nm by UV-Vis spectra in Figure 4B. Steady-state PL spectra were operated to assess the photoelectrical property of perovskite films deposited on glass substrates. As shown in Figure 4C, the PL intensities of SrCl2- and NiCl2-doped perovskite films are 4.46 and 2.72 times higher than that of the pristine film, respectively, indicating mitigated non-radiative recombination in bivalent metal chloride–doped films (Han et al., 2016; You et al., 2018).
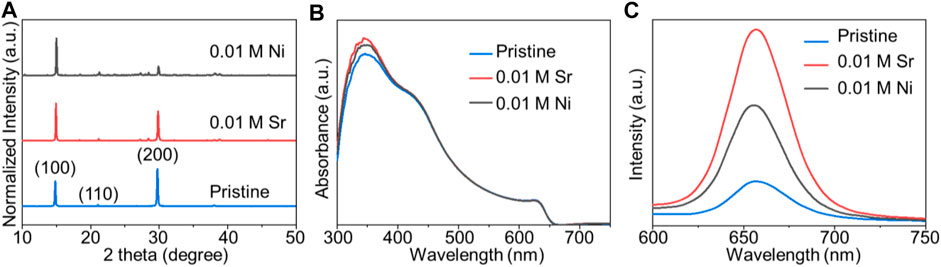
FIGURE 4. (A) XRD patterns and (B) UV-Vis spectra of the pristine, 0.01 M SrCl2–doped, and 0.01 M NiCl2–doped CsPbI2Br thin films on compact TiO2/FTO. (C) Photoluminescence (PL) spectra of the pristine, 0.01 M SrCl2–doped, and 0.01 M NiCl2–doped CsPbI2Br thin films deposited on glass.
We then measured the J–V characteristics of as-prepared solar cells. Solar cells were fabricated with a planar n-i-p configuration of FTO/compact-TiO2 (c-TiO2)/CsPbI2Br/P3HT/Au (Figure 5A). All J–V characteristics were measured under simulated AM 1.5G illumination with a reverse scan rate of 0.15 V s−1. As shown in Figure 5B and Table 1, the pristine device shows a short-circuit current density (JSC) of 15.28 mA cm−2, an open-circuit voltage (VOC) of 1,197 mV, a fill factor (FF) of 0.71%, and a PCE of 12.89%. The addition of SrCl2 mainly enhances the VOC values of devices: the VOC are 1,266, 1,278, 1,278, and 1,163 mV, by using 0.001, 0.005, 0.01, and 0.05 M SrCl2 additives. The champion doped device was obtained by using 0.01 M SrCl2 with a JSC of 15.75 mA cm−2, a VOC of 1,322 mV, a FF of 0.77, and a PCE of 16.07% under reverse scan condition (Figure 5C and Table 2). Notably, negligible hysteresis was observed for this champion device. In contrast, the best pristine sample exhibited a JSC of 15.69 mA cm−2, a VOC of 1,217 mV, a FF of 0.73, and a PCE of 13.66% under reverse scan condition (Supplementary Figure S1 and Supplementary Table S1). We then held these devices under maximum power point (MPP) to measure the stabilized power output, as shown in Figure 5D and Supplementary Figure S2. The current density at the MPP was 14.39 mA cm−2 at a bias voltage of 1,100 mV, corresponding to an efficiency of 15.83%. The current density at the MPP was 13.47 mA cm−2 at a bias voltage of 960 mV, corresponding to an efficiency of 12.93%. Supplementary Figure S3 exhibits the external quantum efficiency (EQE) spectra of PSCs. The integration of the EQE spectrum yields the photocurrent of 15.37 mA cm−2 for the 0.01 M SrCl2–doped device and 15.24 mA cm−2 for the pristine device, which are consistent with the corresponding J–V measurements shown in Figure 5C and Supplementary Figure S1.
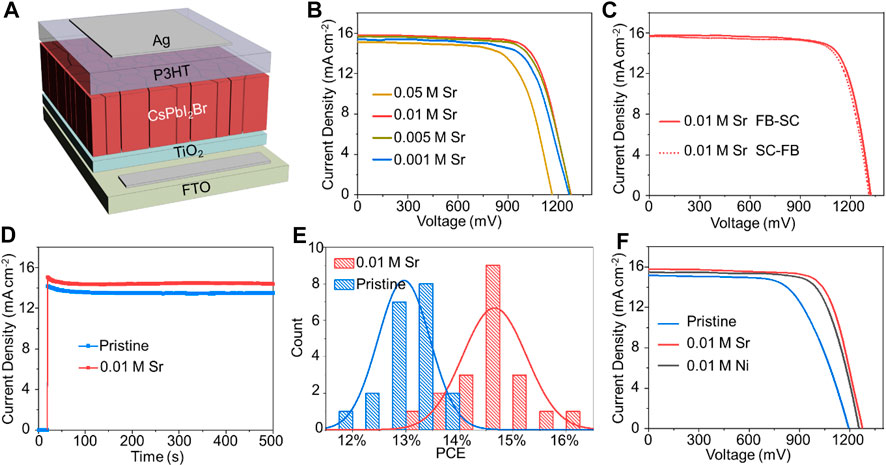
FIGURE 5. (A) Device architecture of a typical PSC device. (B)J–V curves of PSCs based on SrCl2-doped CsPbI2Br films with different dopant concentrations. (C)J–V curves of the champion device for the SrCl2-doped PSC scanned from forward bias (FB) to short circuit (SC) and SC to FB with a scan rate of 0.15 V s−1. (D) Photocurrent density as a function of time for a device held at the maximum power point. (E) Statistical distribution of PCEs of 20 individual PSCs based on pristine and 0.01 M SrCl2–doped CsPbI2Br films. (F)J–V curves of typical devices under simulated AM 1.5G illumination of 100 mW cm−2 for pristine, 0.01 M SrCl2–doped, and 0.01 M NiCl2–doped CsPbI2Br cells.
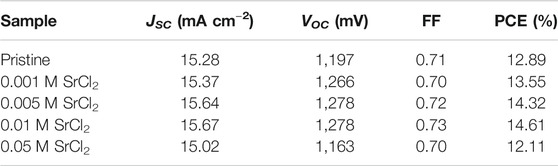
TABLE 1. Photovoltaic parameters of PSCs with different SrCl2 dopant concentrations under simulated AM 1.5G of 100 mW/cm2 solar irradiation.

TABLE 2. Summary of photovoltaic performance for champion devices (FB–SC and SC–FB) based on the 0.01 M SrCl2–doped perovskite film.
A statistical analysis of 20 individual devices reveals the good productivity of SrCl2-doped devices such that the mean PCEs of pristine and SrCl2-doped devices are 14.66 and 12.96%, respectively (Figure 5E). Moreover, inclusion of NiCl2 also improved the device performance significantly (Figure 5F and Supplementary Table S2). The VOC of the NiCl2-doped device increased to 1,245 mV, yielding a PCE of 13.83%. The results presented herein manifest our strategy as versatile and universal with a large variety of possible metal salts for perovskite devices.
We then studied the optical and electrical properties of the doped perovskite devices to probe the origin for performance enhancement. EIS spectra were operated to characterize the carrier behaviors of as-fabricated CsPbI2Br devices, and the equivalent circuit model (Cui et al., 2020; Zhang et al., 2020b) is displayed in Figure 6A. The charge recombination resistance (Rrec) of the SrCl2-doped device is much higher than that of the pristine device at a bias of 0.8 V (Figure 6A). The Rrec values of the SrCl2-doped device are about one time larger than Rrec values of the pristine one under the bias range of 0–1 V, which confirms the longer carrier lifetime of the SrCl2-doped device (Figure 6B) (Quilettes et al., 2015). We further investigated charge-transport properties of PSCs with and without SrCl2 by using TPV measurements. The TPV curve revealed that incorporation of SrCl2 increased the charge-carrier lifetime (Figure 6C), indicating a decrease in the undesired charge-carrier recombination (Seitkhan et al., 2020). As shown in Supplementary Figure S4, the ideality factor (m) was measured to evaluate the recombination process in PSCs. The value of m for SrCl2-doped devices (1.31 kT/e) is smaller than 1.74 kT/e of the pristine device, illustrating the reduced trap-assisted charge recombination, where K is the Boltzmann constant, T is an absolute temperature of 300 K, and e is the elementary charge (Aydin et al., 2019; Dong et al., 2021).
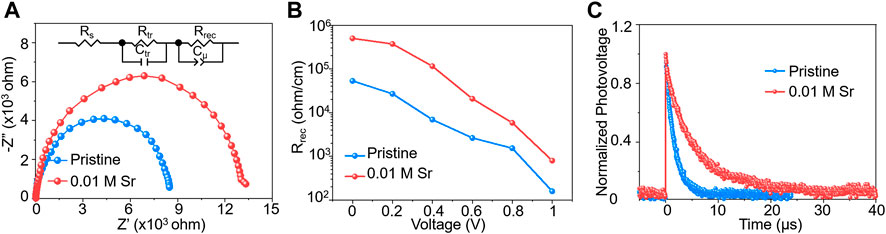
FIGURE 6. (A) Nyquist plots of the PSCs based on pristine and 0.01 M SrCl2–doped CsPbI2Br films recorded at 0.8 V in dark condition. (B) Rrec of PSCs based on pristine and 0.01 M SrCl2–doped CsPbI2Br films measured at different applied bias voltages. (C) TPV measurements of CsPbI2Br cells with and without SrCl2 dopants.
Conclusion
In summary, we report a simple strategy to modulate the CsPbI2Br perovskite by using bivalent metal chloride additives, which facilitates the formation of high-quality perovskite films. A high PCE of 16.07% was achieved on solar cells by SrCl2 doping, which could eliminate defective trap states and extend carrier lifetime. Our strategy would provide new insights into controllably fabricating high-efficiency all-inorganic perovskite solar cells.
Data Availability Statement
The original contributions presented in the study are included in the article/Supplementary Material, and further inquiries can be directed to the corresponding authors.
Author Contributions
HY and YH conceived the project and contributed to the design of the experiments and analysis of the data. HQ performed the material preparation, device fabrication, and characterizations and wrote the manuscript with the help of HY, YH, MC, ZZ, and QC coordinated this research. All authors contributed to the scientific discussion and manuscript revisions.
Funding
This work was financially supported by the National Natural Science Foundation of China (51972111), National Ten Thousand Talents Program for Young Top-Notch Talent, National Natural Science Fund for Distinguished Young Scholars (51725201), International (Regional) Cooperation and Exchange Projects of the National Natural Science Foundation of China (51920105003), Innovation Program of Shanghai Municipal Education Commission (E00014), Fundamental Research Funds for the Central Universities (JKD012016025), and Shanghai Engineering Research Center of Hierarchical Nanomaterials (18DZ2252400).
Conflict of Interest
The authors declare that the research was conducted in the absence of any commercial or financial relationships that could be construed as a potential conflict of interest.
Acknowledgments
The authors thank the Frontiers Science Center for Materiobiology and Dynamic Chemistry for providing some facilities to carry out this work.
Supplementary Material
The Supplementary Material for this article can be found online at: https://www.frontiersin.org/articles/10.3389/fenrg.2021.692059/full#supplementary-material
References
Aydin, E., Bastiani, M., and Wolf, S. (2019). Defect and Contact Passivation for Perovskite Solar Cells. Adv. Mater. 31, e1900428. doi:10.1002/adma.201900428
Bai, D., Zhang, J., Jin, Z., Bian, H., Wang, K., Wang, H., et al. (2018). Interstitial Mn2+-Driven High-Aspect-Ratio Grain Growth for Low-Trap-Density Microcrystalline Films for Record Efficiency CsPbI2Br Solar Cells. ACS Energy Lett. 3, 970–978. doi:10.1021/acsenergylett.8b00270
Chen, W., Chen, H., Xu, G., Xue, R., Wang, S., Li, Y., et al. (2019). Precise Control of Crystal Growth for Highly Efficient CsPbI2Br Perovskite Solar Cells. Joule 3, 191–204. doi:10.1016/j.joule.2018.10.011
Chen, W., Wu, Y., Yue, Y., Liu, J., Zhang, W., Yang, X., et al. (2015). Efficient and Stable Large-Area Perovskite Solar Cells with Inorganic Charge Extraction Layers. Science 350, 944–948. doi:10.1126/science.aad1015
Cui, X., Chen, Y., Zhang, M., Harn, Y. W., Qi, J., Gao, L., et al. (2020). Tailoring Carrier Dynamics in Perovskite Solar Cells via Precise Dimension and Architecture Control and Interfacial Positioning of Plasmonic Nanoparticles. Energy Environ. Sci. 13, 1743–1752. doi:10.1039/C9EE03937F
deQuilettes, D. W., Vorpahl, S. M., Stranks, S. D., Nagaoka, H., Eperon, G. E., Ziffer, M. E., et al. (2015). Solar Cells Impact of Microstructure on Local Carrier Lifetime in Perovskite Solar Cells. Science 348, 683–686. doi:10.1126/science.aaa5333
Dong, H., Zhang, C., Liu, X., Yao, J., and Zhao, Y. S. (2020). Materials Chemistry and Engineering in Metal Halide Perovskite Lasers. Chem. Soc. Rev. 49, 951–982. doi:10.1039/C9CS00598F
Dong, W., Xiong, S., Yang, J., Qiao, W., Zeng, Q., Wang, X., et al. (2021). Black Phosphorus Doped Poly(triarylamine) as Hole Transport Layer for Highly Efficient Perovskite Solar Cells. Org. Electron. 89, 106052. doi:10.1016/j.orgel.2020.106052
Duan, C., Cui, J., Zhang, M., Han, Y., Yang, S., Zhao, H., et al. (2020). Precursor Engineering for Ambient‐Compatible Antisolvent‐Free Fabrication of High‐Efficiency CsPbI2Br Perovskite Solar Cells. Adv. Energy Mater. 10, 2000691. doi:10.1002/aenm.202000691
Eperon, G. E., and Ginger, D. S. (2017). B-site Metal Cation Exchange in Halide Perovskites. ACS Energy Lett. 2, 1190–1196. doi:10.1021/acsenergylett.7b00290
Fang, T., Wang, T., Li, X., Dong, Y., Bai, S., and Song, J. (2020). Perovskite QLED with an External Quantum Efficiency of over 21% by Modulating Electronic Transport. Sci. Bull. 66, 36–43. doi:10.1016/j.scib.2020.08.025
Fu, S., Zhang, W., Li, X., Wan, L., Wu, Y., Chen, L., et al. (2020). Dual-Protection Strategy for High-Efficiency and Stable CsPbI2Br Inorganic Perovskite Solar Cells. ACS Energy Lett. 5, 676–684. doi:10.1021/acsenergylett.9b02716
Fu, Y., Zhu, H., Chen, J., Hautzinger, M. P., Zhu, X.-Y., and Jin, S. (2019). Metal Halide Perovskite Nanostructures for Optoelectronic Applications and the Study of Physical Properties. Nat. Rev. Mater. 4, 169–188. doi:10.1038/s41578-019-0080-9
Han, Q., Bae, S.-H., Sun, P., Hsieh, Y.-T., Yang, Y. M., Rim, Y. S., et al. (2016). Single Crystal Formamidinium Lead Iodide (FAPbI3): Insight into the Structural, Optical, and Electrical Properties. Adv. Mater. 28, 2253–2258. doi:10.1002/adma.201505002
He, J., Liu, J., Hou, Y., Wang, Y., Yang, S., and Yang, H. G. (2020). Surface Chelation of Cesium Halide Perovskite by Dithiocarbamate for Efficient and Stable Solar Cells. Nat. Commun. 11, 4237. doi:10.1038/s41467-020-18015-5
Li, B., Zhang, Y., Fu, L., Yu, T., Zhou, S., Zhang, L., et al. (2018). Surface Passivation Engineering Strategy to Fully-Inorganic Cubic FaPbI3 Perovskites for High-Performance Solar Cells. Nat. Commun. 9, 1076. doi:10.1038/s41467-018-03169-0
Li, C., Pan, Y., Hu, J., Qiu, S., Zhang, C., Yang, Y., et al. (2020). Vertically Aligned 2D/3D Pb-Sn Perovskites with Enhanced Charge Extraction and Suppressed Phase Segregation for Efficient Printable Solar Cells. ACS Energy Lett. 5, 1386–1395. doi:10.1021/acsenergylett.0c00634
Lin, K., Xing, J., Quan, L. N., de Arquer, F. P. G., Gong, X., Lu, J., et al. (2018). Perovskite Light-Emitting Diodes with External Quantum Efficiency Exceeding 20 Per Cent. Nature 562, 245–248. doi:10.1038/s41586-018-0575-3
Liu, C., Li, W., Zhang, C., Ma, Y., Fan, J., and Mai, Y. (2018). All-Inorganic CsPbI2Br Perovskite Solar Cells with High Efficiency Exceeding 13. J. Am. Chem. Soc. 140, 3825–3828. doi:10.1021/jacs.7b13229
Liu, C., Yang, Y., Syzgantseva, O. A., Ding, Y., Syzgantseva, M. A., Zhang, X., et al. (2020). α‐CsPbI3 Bilayers via One‐Step Deposition for Efficient and Stable All‐Inorganic Perovskite Solar Cells. Adv. Mater. 32, 2002632. doi:10.1002/adma.202002632
Phung, N., Félix, R., Meggiolaro, D., Al-Ashouri, A., Sousa e Silva, G., Hartmann, C., et al. (2020). The Doping Mechanism of Halide Perovskite Unveiled by Alkaline Earth Metals. J. Am. Chem. Soc. 142, 2364–2374. doi:10.1021/jacs.9b11637
Qiao, H. W., Yang, S., Wang, Y., Chen, X., Wen, T. Y., Tang, L. J., et al. (2019). A Gradient Heterostructure Based on Tolerance Factor in High-Performance Perovskite Solar Cells with 0.84 Fill Factor. Adv. Mater. 31, 1804217. doi:10.1002/adma.201804217
Qiao, W.-C., Wu, J., Zhang, R., Ou-Yang, W., Chen, X., Yang, G., et al. (2020). In Situ NMR Investigation of the Photoresponse of Perovskite crystal. Matter 3, 2042–2054. doi:10.1016/j.matt.2020.09.004
Rehman, W., McMeekin, D. P., Patel, J. B., Milot, R. L., Johnston, M. B., Snaith, H. J., et al. (2017). Photovoltaic Mixed-Cation lead Mixed-Halide Perovskites: Links between Crystallinity, Photo-Stability and Electronic Properties. Energy Environ. Sci. 10, 361–369. doi:10.1039/C6EE03014A
Seitkhan, A., Neophytou, M., Hallani, R. K., Troughton, J., Gasparini, N., Faber, H., et al. (2020). A Multilayered Electron Extracting System for Efficient Perovskite Solar Cells. Adv. Funct. Mater. 30, 2004273. doi:10.1002/adfm.202004273
Shai, X., Wang, J., Sun, P., Huang, W., Liao, P., Cheng, F., et al. (2018). Achieving Ordered and Stable Binary Metal Perovskite via Strain Engineering. Nano Energy 48, 117–127. doi:10.1016/j.nanoen.2018.03.047
Shannon, R. D. (1976). Revised Effective Ionic Radii and Systematic Studies of Interatomic Distances in Halides and Chalcogenides. Acta Cryst. Sect A. 32, 751–767. doi:10.1107/S0567739476001551
Shi, D., Adinolfi, V., Comin, R., Yuan, M., Alarousu, E., Buin, A., et al. (2015). Low Trap-State Density and Long Carrier Diffusion in Organolead Trihalide Perovskite Single Crystals. Science 347, 519–522. doi:10.1126/science.aaa2725
Straus, D. B., Guo, S., and Cava, R. J. (2019). Kinetically Stable Single Crystals of Perovskite-phase CsPbI3. J. Am. Chem. Soc. 141, 11435–11439. doi:10.1021/jacs.9b06055
Sutton, R. J., Eperon, G. E., Miranda, L., Parrott, E. S., Kamino, B. A., Patel, J. B., et al. (2016). Bandgap-Tunable Cesium Lead Halide Perovskites with High Thermal Stability for Efficient Solar Cells. Adv. Energy Mater. 6, 1502458. doi:10.1002/aenm.201502458
Tan, Q., Ye, G., Zhang, Y., Du, X., Liu, H., Xie, L., et al. (2019). Vacuum-filtration Enabled Large-Area CsPbBr3 Films on Porous Substrates for Flexible Photodetectors. J. Mater. Chem. C 7, 13402–13409. doi:10.1039/C9TC04032C
Wang, K., Subhani, W. S., Wang, Y., Zuo, X., Wang, H., Duan, L., et al. (2019a). Metal Cations in Efficient Perovskite Solar Cells: Progress and Perspective. Adv. Mater. 31, 1902037. doi:10.1002/adma.201902037
Wang, Y., Dar, M. I., Ono, L. K., Zhang, T., Kan, M., Li, Y., et al. (2019b). Thermodynamically Stabilized β-CsPbI3-based Perovskite Solar Cells with Efficiencies >18. Science 365, 591–595. doi:10.1126/science.aav8680
Wang, Y., Liu, X., Zhang, T., Wang, X., Kan, M., Shi, J., et al. (2019c). The Role of Dimethylammonium Iodide in CsPbI3 Perovskite Fabrication: Additive or Dopant? Angew. Chem. Int. Ed. 58, 16691–16696. doi:10.1002/anie.201910800
Yan, L., Xue, Q., Liu, M., Zhu, Z., Tian, J., Li, Z., et al. (2018). Interface Engineering for All-Inorganic CsPbI2Br Perovskite Solar Cells with Effciency over 14%. Adv. Mater. 30, 1802509. doi:10.1002/adma.201802509
Yang, S., Chen, S., Mosconi, E., Fang, Y., Xiao, X., Wang, C., et al. (2019a). Stabilizing Halide Perovskite Surfaces for Solar Cell Operation with Wide-Bandgap lead Oxysalts. Science 365, 473–478. doi:10.1126/science.aax3294
Yang, S., Dai, J., Yu, Z., Shao, Y., Zhou, Y., Xiao, X., et al. (2019b). Tailoring Passivation Molecular Structures for Extremely Small Open-Circuit Voltage Loss in Perovskite Solar Cells. J. Am. Chem. Soc. 141, 5781–5787. doi:10.1021/jacs.0c0642110.1021/jacs.8b13091
Yang, Z., Yu, Z., Wei, H., Xiao, X., Ni, Z., Chen, B., et al. (2019c). Enhancing Electron Diffusion Length in Narrow-Bandgap Perovskites for Efficient Monolithic Perovskite Tandem Solar Cells. Nat. Commun. 10, 4498. doi:10.1038/s41467-019-12513-x
You, S., Wang, H., Bi, S., Zhou, J., Qin, L., Qiu, X., et al. (2018). A Biopolymer Heparin Sodium Interlayer Anchoring TiO2 and MAPbI3 Enhances Trap Passivation and Device Stability in Perovskite Solar Cells. Adv. Mater. 30, 1706924. doi:10.1002/adma.201706924
Yu, Y., and Gao, P. (2017). Development of Electron and Hole Selective Contact Materials for Perovskite Solar Cells. Chin. Chem. Lett. 28, 1144–1152. doi:10.1016/j.cclet.2017.04.020
Zeng, Q., Zhang, X., Liu, C., Feng, T., Chen, Z., Zhang, W., et al. (2019). Inorganic CsPbI2Br Perovskite Solar Cells: The Progress and Perspective. Sol. RRL 3, 1800239. doi:10.1002/solr.201800239
Zhang, C., Wang, K., Wang, Y., Subhani, W. S., Jiang, X., Wang, S., et al. (2020a). Low‐Temperature Crystallization of CsPbIBr2 Perovskite for High Performance Solar Cells. Sol. RRL 4, 2000254. doi:10.1002/solr.202000254
Zhang, J., Hodes, G., Jin, Z., and Liu, S. (2019). All‐Inorganic CsPbX 3 Perovskite Solar Cells: Progress and Prospects. Angew. Chem. Int. Ed. 58, 15596–15618. doi:10.1002/anie.201901081
Zhang, S., Si, H., Fan, W., Shi, M., Li, M., Xu, C., et al. (2020b). Graphdiyne: Bridging SnO2 and Perovskite in Planar Solar Cells. Angew. Chem. Int. Ed. 59, 11573–11582. doi:10.1002/ange.20200350210.1002/anie.202003502
Keywords: all-inorganic PSC, CsPbI2Br, bivalent metal chloride salts, doping, PCE
Citation: Qiao HW, Chen M, Zhou Z, Cheng Q, Hou Y and Yang HG (2021) Improved Photovoltaic Performance of CsPbI2Br Perovskite Films via Bivalent Metal Chloride Doping. Front. Energy Res. 9:692059. doi: 10.3389/fenrg.2021.692059
Received: 07 April 2021; Accepted: 06 May 2021;
Published: 25 May 2021.
Edited by:
Xingxing Gu, Chongqing Technology and Business University, ChinaReviewed by:
Xue Lu Wang, East China Normal University, ChinaHua Yu, Southwest Petroleum University, China
Yu Zhou, Central South University, China
Copyright © 2021 Qiao, Chen, Zhou, Cheng, Hou and Yang. This is an open-access article distributed under the terms of the Creative Commons Attribution License (CC BY). The use, distribution or reproduction in other forums is permitted, provided the original author(s) and the copyright owner(s) are credited and that the original publication in this journal is cited, in accordance with accepted academic practice. No use, distribution or reproduction is permitted which does not comply with these terms.
*Correspondence: Yu Hou, yhou@ecust.edu.cn; Hua Gui Yang, hgyang@ecust.edu.cn