- 1Key Laboratory of Unconventional Oil and Gas Development, China University of Petroleum (East China), Ministry of Education, Qingdao, China
- 2School of Petroleum Engineering, China University of Petroleum (East China), Qingdao, China
- 3Dongxin Oil Production Plant, Shengli Oilfield, Dongying, Shandong, China
Clay minerals are regarded as an important factor affecting the results of low salinity water (LSW) flooding. However, most researchers put great attention on their microscopic properties, only few of them investigated the influence of clay minerals by the sand pack flooding experiments. This paper quantifies the effects of clay minerals on LSW flooding with artificial sand pack samples. We used three kinds of sand pack samples (100% quartz sand; 90%/10% quartz sand/kaolinite or montmorillonite) and heavy oil in the LSW flooding experiments. For the samples made of montmorillonite, results show that there exists an optimal salinity range for the LSW flooding. The best salinity range of Na+ is between 1,000 mg/L and 5,000 mg/L, and Ca2+ is between 500 mg/L and 1,000 mg/L. As for kaolinite, a better result will be produced with lower salinity. In addition, using CaCl solution floods samples made of montmorillonite has a better result. A similar phenomenon was also observed when NaCl solution was used to flood kaolinite samples. Respect of flooding modes, the continuous injection of high-low salinity water is more effective, but the LSW flooding method is better considering the economy and time cost.
Introduction
With the exploitation of oil and gas resources, the development of oilfield becomes harder. Although chemical flooding method can help to improve the oil recovery, it needs a huge cost and causes great environmental concerns (Guo, 2020). Under this situation, researchers are eager to develop new enhanced oil recovery (EOR) technologies. Recently, they realized that the saturation of residual oil can be effectively reduced by injecting LSW. As a new oil recovery technology (Zhang et al., 2007), LSW flooding is widely used in the field due to its advantages of high efficiency, environment-friendly and low cost. Up to now, it has been used in Burgan oilfield, North Sea oilfield, Powder River basin in Wyoming and Endicott oilfield (Idowu et al., 2011; Nasralla et al., 2011).
LSW flooding can alter the physical and chemical properties of reservoir by injecting water with a low concentration of soluble ions. It can reduce the saturation of residual oil and improve oil recovery as a result (Wu et al., 2015; Li et al., 2016; Wu et al., 2016). However, the salinity of LSW has a threshold value. If the salinity is too low, it may cause swelling or flocculation of the clay minerals. And if it is too high, it will lose its function. A large number of experiments have proved the positive effect of LSW on EOR and revealed a lot of relevant mechanisms. However, different testing methods produced different dominant mechanisms, some mechanisms are contradictory to others. Meanwhile, it is generally believed that clay minerals are necessary factors that would affect the result of LSW flooding (Bernard, 1967; Tang and Morrow, 1999; Zhang and Morrow, 2006; Zhang et al., 2007; loahardjo et al., 2010). Lots of studies on mechanisms of clay minerals were conducted. First, in terms of microcosmic mechanism, the main mechanisms include the diffusion of double electron layer (Ligthelm et al., 2009; Cui et al., 2020), cation exchange of clay minerals (Almada et al., 2013; Amirian et al., 2017) and changes in wettability (Nasralla et al., 2011; Amirian et al., 2017). The study of micro percolation mainly focuses on the particle migration of clay minerals. Tang and Morrow (1999) first discovered that the migration of clay mineral particles is important for the flooding of residual oil adsorbed in the pore wall. Song and Kovscek (2016) observed the migration of clay mineral particles with crude oil. There are also many studies through the core flooding experiment. Lebedeva and Fogden (2011) found LSW flooding can increase oil recovery in kaolinite; Kim and Lee (2017) showed the influence of clay type and relative permeability with sandstone cores. Al-Saedi and Flori (2018) prepared sandstone cores with a controllable percentage of clay and carried out the flooding experiment. All of them have investigated the EOR mechanisms of LSW flooding.
From the micro point, most researchers think that the influence of clay minerals is caused by the change of wetting angles and the migration of particles. However, experiment results are quite different under reservoir conditions due to the influence of clay occurrence mode and fluid properties. Also, relevant research on clay minerals is mainly in the qualitative stage, without quantitative evaluation and analysis on the influence of LSW flooding. Moreover, the LSW flooding experiment has special requirements on ion composition and concentration of injected water (Yang et al., 2020). Up to now, there is no study conducted on the optimal ion and concentration of LSW for different types of clay minerals. At the same time, the artificial cores used in the experiments are simply made through the mixing of clay and cores, which can not simulate the cementation state of clay minerals in natural cores. As a result, the accuracy of the experimental data and conclusions is hard to guarantee. In this paper, two kinds of popular clay minerals (kaolinite and montmorillonite) were used to make synthetic sand cores that can simulate the adhesion of clay minerals in natural cores. Through the LSW flooding experiments, the macroscopic flooding effects in different cores were observed, the adaptability evaluation of different clay minerals was obtained and the influence law of clay minerals was summarized. In this way, this paper can provide basic guidance for the use of LSW flooding in the oilfield.
Experimental Section
Equipment
The LSW flooding equipment can be seen in Figure 1. It consists of three systems: reservoir simulation system, fluid injection system and the acquisition and control system for pressure data. The reservoir simulation system includes customized mineral-filled sand filling pipe and thermostat, which can accurately simulate the production process of real reservoir; the fluid injection system can maintain a constant flow rate for the injection of various fluids; the pressure data acquisition and control system can simulate the formation and bottom hole pressure and collect the pressure dring the injection process.
Materials
Materials used in the experiment include quartz sand, clay minerals, crude oil, and LSW with different salinity.
Oil used in the experiment is the heavy oil from Shengli oilfield. The viscosity, resin content and asphaltene of it are pretty high. Its composition and basic physical properties are listed in Table 1.
Two kinds of water, including Na+ and Ca2+ solution, with four different concentration were prepared: 500, 1,000, 5,000, 20000 mg/L. The compositions of these water are listed in Tables 2 and 3. High salinity water (HSW 20,000 mg/L) was used to measure the permeability of sand packs and form the connate water in sand packs before flooding. The salinity of injected water used in LSW is less than 12,000 mg/L.
Synthetic sand packs are made of quartz sand and clay minerals. The ratio of the 10–20 mesh coarse quartz sand, the 40–60 mesh fine quartz and clay is 4.5:4.5:1. First, mixed the clay and deionized water with a ratio of 1:15, then degraded by a 15 Hz acoustic wave for 30 min. After that, added NaOH to adjust the pH value to 9.8 (consistent with the injected water in the research oil field), centrifuged the solution for 3 min, removed the sediment, took out the suspension, quickly stirred and degraded for 10 min, centrifuged 3 min again, repeated the above step for three times. Took the suspension attached to the surface of quartz sand and placed it in the oven at 120 C. Finally, the quartz sand with clay was filled in the sand filling pipe to complete the sand pack production process. The scanning electron microscope (SEM) of the sand pack surface is shown in Figure 2. The physical properties and experimental parameters of the sand packs are listed in Table 4.
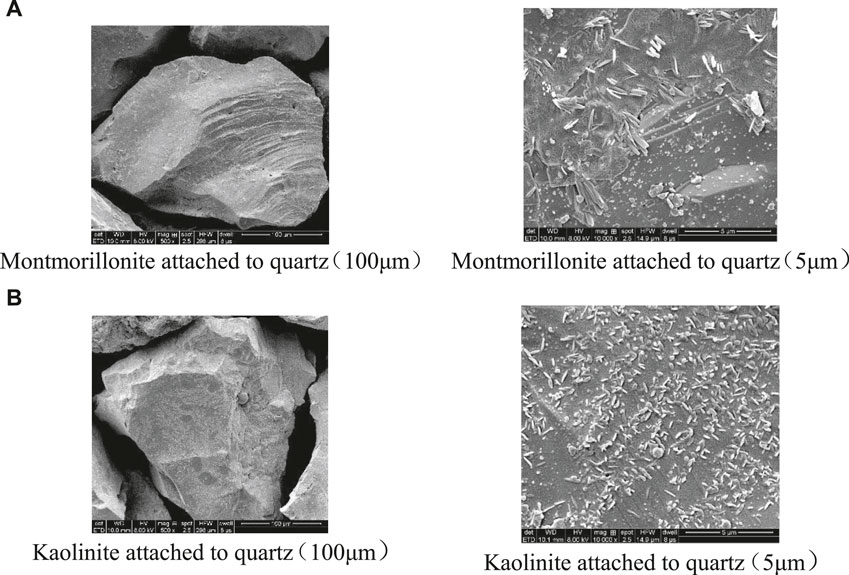
FIGURE 2. Scanning electron micrograph (SEM) of (A) montmorillonite and (B) kaolinite attached to quartz.
Procedure
1) Make different kinds of synthetic sand packs: 100% quartz sand; 90%/10% quartz sand/kaolinite or montmorillonite. 2) Measure the porosity of each sand pack using the weighing method. 3) Test the permeability of each sand pack under simulated reservoir conditions. 4) Saturate HSW and crude oil in sequence under the pressure of 10 MPa and reservoir temperature of 60°C with a liquid injection rate of 0.2 ml/min, after that, leave the sand packs in the holder for 1 weak to make the oil, water and rock contact sufficiently. 5) Inject water at 0.2 ml/min according to designed scheme. During the sand pack flooding experiments, produced oil and water were recorded at each 0.2 PV. 6) Calculate the oil recovery factor and water content in produced fluid. When the water content became 98%, the sand pack flooding process was finished.
Schemes
A single variable was controlled in the experiment. Clay minerals were divided into kaolinite, montmorillonite and non-clay minerals, the ion type of the flooding fluid was set to Ca2+ and Na+. First, 18 groups of experiments were designed with LSW with a concentration of 5,000, 1,000, and 500 mg/L, respectively. Then, each kind of sand pack was flooded by HSW-LSW (20,000, 5,000, 1,000, 500 mg/L) in sequence to see the effect of flooding mode on oil recovery.
Experiment Results and Discussion
Performance of Enhanced Oil Recovery
Results of sand pack flooding are shown in Table 5, including injection water, flooding mode and oil recovery. During the successive LSW injection, more and more oil was produced with the decrease of salinity of the injected water. This phenomenon successfully proves the EOR performance of LSW injection.
Analysis of Montmorillonite Flooding Results
The comparison of oil recovery in different sand pack-flooding runs can be seen in Figures 2 and 3. With the rise of injected volume of LSW, the recovery degree increases rapidly at first, then gradually tends to be stable. The result of oil recovery with a salinity of 1,200 mg/L is similar to that of 2,400 mg/L, which is much higher than that of 12,000 mg/L.
The final oil recovery of montmorillonite is shown in Figure 4. For Na+, when the salinity of injected water decreased from 12,000 to 2,400 mg/L, the final oil recovery increased from 53.82 to 58.91%, which increased by 5.09%. When the salinity of injected water decreased from 2,400 to 1,200 mg/L, the final oil recovery increased from 58.91 to 59.03%, which increased by only 0.21%. It can be seen that the increase is pretty obvious in the range of 12,000–2,400 mg/L, however, the recovery in the range of 2,400–1,200 mg/L had little change. The Ca2+ flooding had a similar situation. When the salinity of injected water decreased from 12,000 to 2,400 mg/L, the final oil recovery increased from 51.15 to 57.68%, which increased by 6.53%. When the salinity decreased from 12,400 to 1,200 mg/L, the final oil recovery increased from 57.68 to 58.06%, which increased by only 0.38%.
This indicates that under the condition of montmorillonite sand pack, the oil recovery increases with the decrease of salinity, but there is a limit for the salinity content. As a result, the oil recovery cannot keep increasing with the decrease of the salinity of LSW. And there exists an optimum salinity to maximize oil recovery. This is because montmorillonite is pretty expansive and has great expansibility under acidic conditions. When the ion concentration becomes higher, the diffusion electron layer of montmorillonite will be compressed and the expansion volume will decrease (Zadaka et al., 2010), which is helpful to increase the permeability of the reservoir. However, when the ion concentration is too low, the compression capacity of metal cation ions on the electric double layer decreases rapidly, and the expansion capacity of montmorillonite increases rapidly, which leads to the expansion and dispersion of clay and block the seepage channel.
Analysis of Kaolinite Flooding Results
The comparison of oil recovery of kaolinite is shown in Figures 4 and 5. Like montmorillonite, the oil recovery increases rapidly first, then tends to be stable slowly. However, what is different from the montmorillonite sand pack is that the oil recovery increases with the decrease of salinity of injected water.
The final oil recovery of kaolinite is shown in Figure 7. For Na+, when the salinity of injected water decreased from 12,000 to 2,400 mg/L, the final oil recovery increased from 52.32 to 60.14%, which increased by 7.82%. When the salinity of injected water decreased from 2,400 to 1,200 mg/L, the final oil recovery increased from 60.14 to 65.82%, which increased by 5.86%. The Ca2+ flooding had a similar situation. When the salinity of injected water decreased from 12,000 to 2,400 mg/L, the final oil recovery factor increased from 50.59 to 55.73%, which increased by 5.14%. When the salinity decreased from 12,400 to 1,200 mg/L, the final oil recovery factor increased from 55.73 to 60.27%, which increased by 4.54%.
Regardless of the replacement of the kaolinite sand packs by NaCl solution or CaCl2 solution, the increase of oil recovery in the range of 1,200–2,400 mg/L is higher than that in the range of 2,400–12,000 mg/L. It shows that the oil recovery increase with the decrease of salinity, and a lower salinity will have a faster increase rate. This is because kaolinite is non-expansive, which will not expand and migrate when meets water, and does not need the inhibition of cations. When the salinity of the displacement fluid decreases in pore throat, the diffusion of double electron layer between clay particles promotes the migration of clay particles, and the oil droplets adsorbed on the surface of clay particles migrate as well. With the increase of negative zeta potential and electrostatic repulsion, the oil droplets on the surface of clay particles gradually move from the surface of clay particles, eventually leading to the enhancement of water wettability to enhance oil recovery.
Analysis of Quartz Sand Flooding Results
Figures 8 and 9 show the change of oil recovery with the change of injection volume for sand packs that are only made from quartz sand. From these two figures, we can see that the quartz sand packs also underwent three stages: rapid growth, slow growth and stabilization. However, the recovery degree of these quartz sand packs is pretty high even under low salinity conditions. This phenomenon means that the low salinity condition does not affect the recovery factor. The ultimate recovery factor of the quartz sand packs is about 70%, which cannot be achieved by water flooding with low salinity water.
Analysis of the Influence of Ion
In this study, different kinds of NaCl and CaCl2 solutions are used to flood the montmorillonite and kaolinite, respectively. The comparison of the oil recovery is shown in Figure 10. It can be seen from Figure 10 that when the CaCl2 solution displacement montmorillonite, the result is better, and the NaCl solution is better for kaolinite.
Under same condition, using different kinds of LSW for the flooding of the same type of sand packs, the result of univalent ions (Na+) is always better than that of divalent ions (Ca2+). The reason is that the Ca2+ just like a bridge between clay particles and crude oil, which is not conducive to crude oil desorption. However, Na+ can replace the high valence ions for the water in the pore, and the substitution of the high valence ions will behave as a bridge making the crude oil desorb from the surface of clay particles easily. Univalent ions can induce the diffusion of double electron layers on the surface of clay particles and oil-water interface, increase the negative zeta potential on the surface of clay particles and the oil-water interface, and enhance the electrostatic repulsion between the interfaces, thus promote the desorption of crude oil on the surface of clay particles. The change of zeta potential at the interface also makes the wettability of clay particles to be more hydrophilic. Therefore, under different valence states, if ions have an impact on the recovery of LSW flooding, the displacement effect of the solution containing monovalent ion is more obvious than that of the solution containing divalent ion.
Additionally, it can be seen that when the montmorillonite is flooded by LSW, the increasing rate of CaCl2 solution is faster and its result is more obvious. Compared with monovalent ions, divalent ion needs a lower concentration to compress the dielectronic layer. This is mainly due to the characteristic absorption of divalent ions (Ma et al., 2011), which reacts with hydroxyl groups on the surface of montmorillonite to form hydroxyl compounds and enters into the Stern layer. So Ca2+ still has a better compression effect on the double electron layer than Na+ when the salinity of the water is low.
Analysis of the Influence of Displacement Mode
Figure 11 shows the results of kaolinite sand packs under different displacement modes. When the Na+ concentration of LSW is 5,000, 1,000, 500 mg/L, the oil recovery is 10.95, 18.77, and 24.45% higher than that of HSW. The final oil recovery of continuous injection of high-low salinity water (H-LSW) can reach 69.42%, which is 28.05% higher than that of direct injection of HSW, and 17.1, 9.28, 3.6% higher than the direct injection of 5,000, 1,000, 500 mg/L.
The oil recovery curve of the montmorillonite sand pack under different displacement modes is shown in Figure 12. When the concentration of LSW is 5,000, 1,000, 500 mg/L, the recovery is 10.57, 15.66, 15.78%, respectively, higher than that of HSW with the concentration of 20,000 mg/L. However, the oil recovery can be as high as 63.75% with continuous injection of H-LSW, which is 20.5% higher than that of direct injection of high salinity water with a concentration of 5,000 mg/L. The oil recovery of low salinity water with a concentration of 5,000, 1,000, 500 mg/L, is 9.93, 4.84 and 2.96% higher than the direct injection of 5,000, 1,000, 500 mg/L.
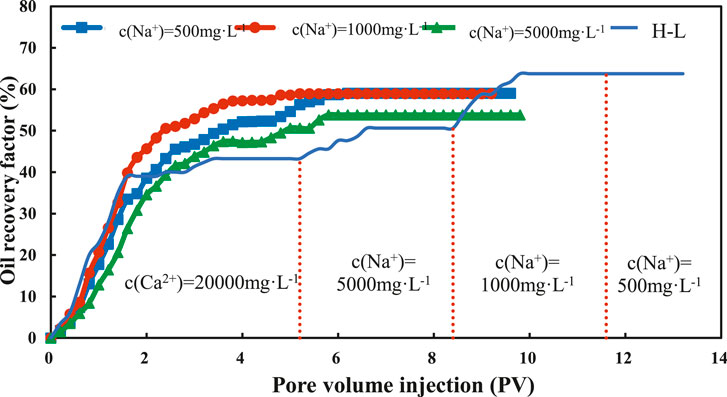
FIGURE 12. Variation curve of the recovery degree of kaolinite sand packs under different displacement methods.
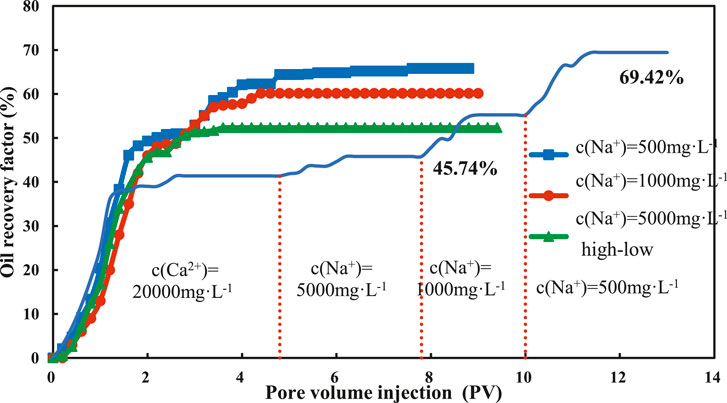
FIGURE 13. Variation curve of the recovery degree of montmorillonite sand packs under different displacement methods.
To sum up, the final oil recovery under different displacement modes is in order of H-LSW > LSW > HSW. This is because when the H-LSW is injected continuously, the concentration of brine reduces gradually, besides, the long inject time and large injection volume are conducive to the interaction among brine, crude oil and clay minerals. Therefore, to achieve maximum oil production, the continuous injection of H-LSW is the best choice. However, this method requires a large amount of brine, and its injection volume is larger than the volume required by the direct injection of LSW to achieve optimal production. As a result, the displacement mode of direct injection of LSW can achieve an ideal recovery in a short time.
Conclusions
In this paper, experiments were conducted with synthetic sand packs that contain different types of clay and different kinds of LSW to determine the relationship between clay minerals and LSW. From the results, the following conclusions have been drawn:
(1) LSW flooding can improve the oil recovery of sand packs made from clay minerals, including montmorillonite and kaolinite, but it cannot increase the oil recovery of sand packs that do not contain clay minerals.
(2) For expansive clays such as montmorillonite, the oil recovery is higher with CaCl2 solution. And the best displacement concentration of Ca2+ is between 500 mg/L and 1,000 mg/L. The reason is that the cations in LSW can inhibit the expansion and migration of expansive clay minerals. If the concentration is too low, it will lead the migration of clay mineral particles, block the pores and increase the residual oil.
(3) For non-expansive clays, such as kaolinite, the oil recovery is higher with the CaCl2 solution. And when the ion concentration is lower, the recovery will be higher. That is because when the ion concentration is lower, the water wettability of the sand pack will be higher, and the oil displacement efficiency will be higher.
(4) No matter whether the montmorillonite or kaolinite is contained in the sand packs, the final oil recovery under different displacement modes is in the order of H-LSW > LSW > HSW.
(5) As for field production, for sand packs rich in montmorillonite, CaCl2 solution should be used as displacement fluid, and ion concentration should be 1,000 mg/L. For sand packs rich in kaolinite, NaCl solution should be used as displacement fluid, and solution with lower ion concentration will have better results. In terms of displacement modes, from the perspective of maximizing oil production, continuous injection of H-LSW is the best choice; from the perspective of achieving ideal recovery in a short time, the direct LSW flooding mode is more attractive.
Data Availablity Statement
All datasets presented in this study are included in the article.
Author Contributions
XW: Conceptualization, methodology, validation, formal analysis and writing - original draft. YZ: Supervision, guidance and resources. WJ: Writing - original draft and data graph drawing. ZW: Experiment operation and data graph drawing. XL: Investigation and resources. FW: Methodology, validation and formal analysis.
Funding
This study was supported by the Natural Science Foundation of China (Project Number: 51874339).
Conflict of Interest
The authors declare that the research was conducted in the absence of any commercial or financial relationships that could be construed as a potential conflict of interest.
References
Al-Saedi, H. N., and Flori, R. E. (2018). Enhanced oil recovery of low salinity water flooding in sandstone and the role of clay. Petrol. Explor. Dev. 45 (5), 927–931. doi:10.1016/s1876-3804(18)30096-x
Almada, M. B. P., Pieterse, S. G. J., Marceliset, A. H. M., Brussee, N. J., and van der Linde, H. A. (2013). “Experimental investigation on the effects of very low salinity on Middle Eastern sandstone core floods,” in SPE European formation damage conference and exhibition, Noordwijk, The Netherlands, June 5–7, 2013 (Society of Petroleum Engineers), SPE165180. Available at: https://www.onepetro.org/conference-paper/SPE-165180-MS (Accessed 2013).
Amirian, T., Haghighi, M., and Mostaghimi, P. (2017). Pore scale visualization of low salinity water flooding as an enhanced oil recovery method. Energy Fuels. 31 (12), 13133–13143. doi:10.1021/acs.energyfuels.7b01702
Amirian, T., Haghighi, M., and Mostaghimi, P. (2017). Pore scale visualization of low salinity water flooding as an enhanced oil recovery method. Energy Fuels. 31 (12), 13133–13143. doi:10.1021/acs.energyfuels.7b01702
Bernard, G. G. (1967). “Effect of floodwater salinity on recovery of oil from cores containing clays,” in SPE California regional meeting, Los Angeles, California. October 26–27, 1967 (Society of Petroleum Engineers), SPE1725. Available at: https://www.onepetro.org/conference-paper/SPE-1725-MS (Accessed, 1967).
Cui, C. Z., Wei, Z. Z., and Wu, Z. J. (2020). Influence of low salinity water flooding on rock wettability based on DLVO theory. J. China Univ. Petrol. 44 (01), 106–114. doi:10.3969/j.issn.1673-5005.2020.01.012
Guo, S. (2020). Application and development analysis of EOR flooding technology in oilfield. Chem. Eng. Equip. (04), 40–41.
Idowu, J., Somervile, J., Adebari, D., and Meshioye, O. (2011). “Effect of salinity changes of the injected water on water flooding performance in carbonate reservoirs,” in Nigeria annual international conference and exhibition, Abuja, Nigeria. July 30–August 3, 2011 (Society of Petroleum Engineers), SPE 150816. Available at: https://www.onepetro.org/conference-paper/SPE-150816-MS (Accessed 2011).
Kim, C., and Lee, J. (2017). Experimental study on the variation of relative permeability due to clay minerals in low salinity water-flooding. J. Petrol. Sci. Eng. 151, 292–304. doi:10.1016/j.petrol.2017.01.014
Lebedeva, E. V., and Fogden, A. (2011). Micro-CT and wettability analysis of oil recovery from sand packs and the effect of waterflood salinity and kaolinite. Energy Fuels. 25 (12), 5683–5694. doi:10.1021/ef201242s
Li, H. T., Ma, Q. R., and Li, D. H. (2017). Micro mechanism of low salinity water injection to improve oil recovery of sandstone reservoir. J. Pet. Explor. Drill. Prod. Technol. 39 (2), 151–157. doi:10.13639/j.odpt.2017.02.005
Li, Y. J., Xia, N., and Li, Y. P. (2016). Oil displacement of low salinity water and hexaalky hydroxypropyl sulfobetaine surfactant. Oilfield Chem. 33 (03), 487–491. doi:10.19346/j.cnki.1000-4092.2016.03.022
Ligthelm, D. J., Gronsvled, J., Hofman, J., Brussee, N., Marceils, F., and van der Linde, H. (2009). “Novel waterflooding strategy by manipulation of injection brine composition,” in EUROPEC/EAGE conference and exhibition, Amsterdam, The Netherlands, June 8–11, 2009 (Society of Petroleum Engineers), SPE119835. Available at: https://www.onepetro.org/conference-paper/SPE-119835-MS (Accessed 2009).
loahardjo, N., Xie, X., and Morrow, N. R. (2010). Oil recovery by sequential waterflooding of mixed-wet sandstone and limestone. Energy Fuels. 24 (9), 5073–5080. doi:10.1021/ef100729b
Ma, L., Peng, C. S., and Fan, C. (2011). Effect of water chemical properties on swelling property of montmorillonite suspension. J. Ocean Univ. China. (S1), 379–385.
Nasralla, R. A., Alotaibi, M. B., and Nasr-El-Din, H. A. (2011). “Efficiency of oil Recovery by low salinity water flooding in sandstone reservoirs,” in SPE Western North American region meeting, Alaska, United States, 7–11 May (Society of Petroleum Engineers), SPE 144602. Available at: https://www.onepetro.org/conference-paper/SPE-144602-MS (Accessed 2011).
Nasralla, R. A., Bataweel, M. A., and Nasr-El-Din, H. A. (2011). Investigation of wettability alteration by low salinity water,” in SPE Offshore Europe oil and gas conference and exhibition, Aberdeen, United Kingdom. September 6–8, 2011 (Society of Petroleum Engineers), SPE146322. Available at: https://www.onepetro.org/conference-paper/SPE-146322-MS (Accessed 2011).
Song, W., and Kovscek, A. R. (2016). Direct visualization of pore-scale fines migration and formation damage during low-salinity waterflooding. J. Nat. Gas Sci. Eng. 34, 1276–1283. doi:10.1016/j.jngse.2016.07.055
Tang, G. Q., and Morrow, N. R. (1999). Influence of brine composition and fines migration on crude oil/brine/rock interactions and oil recovery. J. Petrol. Sci. Eng. 24 (2-4), 99–111. doi:10.1016/s0920-4105(99)00034-0
Wu, J., Chang, Y. W., and Li, J. (2015). Mechanism and application conditions of low salinity water drive technology. J. Southwest Petrol. Univ. 37 (05), 145–151.
Wu, X. Y., Lv, P., and Wang, C. S. (2016). Research on the influence of injection timing on low salinity water flooding effect. Sci. Technol. Eng. 16 (04), 12–15.
Yang, Z. H., Yin, T. H., Kang, S., and Shen, H. (2020). Influence mechanism of ionic composition of brine on rock surface wettability. J. Northeast Petrol. Univ. 44(02):113–118.
Zadaka, D., Radian, A., and Mishael, Y. G. (2010). Applying zeta potential measurements to characterize the adsorption on montmorillonite of organic cations as monomers, micelles, or polymers. J. Colloid Interface Sci. 352 (1), 171–177. doi:10.1016/j.jcis.2010.08.010
Zhang, Y. S., and Morrow, N. R. (2006). “Comparison of secondary and tertiary recovery with change in injection brine composition for crude-oil/sandstone combinations,” in SPE/DOE symposium on improved oil recovery, Oklahoma, United States, April 22–26, 2006 (Society of Petroleum Engineers), SPE99757, Available at: https://www.onepetro.org/conference-paper/SPE-99757-MS (Accessed 2006).
Zhang, Y. S., Xie, X. N., and Morrow, N. R. (2007). “Waterflood performance by injection of brine with different salinity for reservoir cores,” in SPE annual technical conference and exhibition, California, United States, November 11–14, 2007 (Society of Petroleum Engineers), SPE109849, Available at: https://www.onepetro.org/conference-paper/SPE-109849-MS (Accessed 2007).
Zhang, Y., Xie, X., and Morrow, N. R. (2007). “Waterflood performance by injection of brine with different salinity for reservoir cores,” in SPE annual technical conference and exhibition, California, United States, November11–14, 2007 (Society of Petroleum Engineers), SPE 109849. Available at: https://www.onepetro.org/conference-paper/SPE-109849-MS (Accessed 2007).
Keywords: sandstone reservoir, low salinity water flooding, enhanced oil recovery, clay minerals, sandpack displacement
Citation: Wei X, Zhang Y, Jiang W, Wang Z, Li X and Wu F (2020) Experimental Investigation on the Role of Clays in Low Salinity Water Flooding. Front. Energy Res. 8:593639. doi: 10.3389/fenrg.2020.593639
Received: 11 August 2020; Accepted: 22 September 2020;
Published: 22 October 2020.
Edited by:
Wei Yu, University of Texas at Austin, United StatesReviewed by:
Hongna Ding, Northeast Petroleum University, ChinaLei Zhang, China University of Geosciences Wuhan, China
Xiao Wang, Southwest Petroleum University, China
Copyright © 2020 Wei, Zhang, Jiang, Wang, Li and Wu. This is an open-access article distributed under the terms of the Creative Commons Attribution License (CC BY). The use, distribution or reproduction in other forums is permitted, provided the original author(s) and the copyright owner(s) are credited and that the original publication in this journal is cited, in accordance with accepted academic practice. No use, distribution or reproduction is permitted which does not comply with these terms.
*Correspondence: Yanyu Zhang, eXl6aGFuZ0B1cGMuZWR1LmNu Xuemei Wei, MzA3NzI1Njg3QHFxLmNvbQ==