- 1 Beijing Advanced Innovation Center for Tree Breeding by Molecular Design, Beijing Key Laboratory of Lignocellulosic Chemistry, Beijing Forestry University, Beijing, China
- 2 Beijing National Laboratory for Molecular Sciences, CAS Key Laboratory of Colloid and Interface and Thermodynamics, CAS Research/Education Center for Excellence in Molecular Sciences, Institute of Chemistry, Chinese Academy of Sciences, Beijing, China
Understanding lignin structural transformations during DES pretreatment would facilitate to produce lignin with tailor-made properties based on intended applications. To unravel the structural variant of lignin in the DES, the alkali lignin (AL) was pretreated in choline chloride/formic acid (ChCl/FA) (the ratio of 1:2) at 80–120 °C. Characterization of the AL and regenerated lignin samples were determined to understand lignin structure, such as linkage in lignin, phenolic OH content, molecular weight, and aromatic products. ChCl/FA DES pretreatment resulted in large amounts of the dissociation of β-O-4′ bonds, partial cleavage of carbon-carbon bonds (i.e., β-β′, β-5′), dehydration of hydroxyl radicals in lignin’s side-chain, and demethoxylation reaction. Furthermore, various monomeric phenols derived from AL were also determined in spite of low combined yields, which facilitate the production of fine chemicals in the future. Detailed unmasking of lignin structural transformation during the DES process is conducive to the optimizing preparation of homogeneous lignin with low molecular weight.
Introduction
Lignocellulose, mainly consisting of carbohydrates (cellulose and hemicellulose) and aryl-biopolymers (lignin), is the richest, green and renewable bioresource, which can be used to produce various fuels, materials, and fine chemicals (Zhang et al., 2017; Liu et al., 2019b; Dong et al., 2020). The transition from fossil fuels to biomass-based products using renewable lignocellulose has recently been gained considerable interest in biorefinery industries. However, biomass recalcitrance, especially the presence of lignin, would always hinder the successful conversion of lignocellulose to a variety of valuable co-products (Himmel et al., 2007; Ragauskas et al., 2014). Biomass pretreatment, which aims to destroy the inner recalcitrance, is an important step (Rastogi and Shrivastava, 2017; Huang et al., 2019; Soltaniana et al., 2020). Among pretreatment processing, lignin fractionation from lignocellulose remains the key step.
Recently, deep eutectic solvents (DES) have drawn increasing interest, which can be used as promising green solvents for lignocellulosic biomass process and delignification (Chen et al., 2018b; Lin et al., 2019; Lin et al., 2020). DES are considered to be a eutectic mixture made up of hydrogen bond acceptor and hydrogen bond donor through hydrogen bond interactions, and its melting point is much lower than the individual components (Smith et al., 2014). As compared to conventional solvents and ionic liquids, DES owns some superiourities, such as ease of synthesis, availability, cost effectiveness in raw materials, good recyclability, and biocompatibility (Satlewal et al., 2018). Francisco et al. first showed high lignin solubility and low cellulose solubility in a range of DESs (Francisco et al., 2012). After that, various types of DES, such as ChCl/FA, ChCl/lactic acid, and ChCl/glycerol, have been prepared and applied to improve the saccharification efficiency of cellulose and delignification (Zhang et al., 2016; Hou et al., 2018; Tan et al., 2019). Yu and co-workers designed a microwave-assisted DES pretreatment method to achieve ultrafast fractionation of lignocellulose in 3 min (Liu et al., 2017a; Liu et al., 2017b). Wan and co-workers further revealed that ChCl/lactic acid pretreatment can extract 65–72% lignin from lignocellulose for only 45 s with microwave irradiation (Chen and Wan, 2018). Meanwhile, Song group systematically investigated the structural transformation of lignin during ChCl/lactic acid process via the experiments of isolated lignin and a series of β-O-4′ lignin model compounds and unraveled multiple pathways simultaneously occur during ChCl/lactic acid process, such as cleavage of β-O-4′, the repolymerization of active species, the production and derivation of mono products (Wang et al., 2020). Numerous DESs, especially carboxylic acid DES, have achieved the extraction of high-yield lignin among mild conditions. However, a significant amount of DES extracted lignin is still underutilized.
As the only renewable aromatic biopolymer in nature, lignin (ca.15–40 wt%) consists of three phenylpropanoid units linked through various ether bonds and carbon-carbon bonds (Sun et al., 2018). To achieve the upgrading of lignin extracted in the DES, it is necessary to unmask lignin structural transformation during DES pretreatment. Zhang and co-workers reported that ChCl/lactic acid pretreatment fractionated a large amount of lignin fragments with high purity from woody biomass, due to the breakage of β-O-4′ bonds in lignin and the suppression of lignin recondensation (Alvarez-Vasco et al., 2016). After that, Shen et al. illustrated that acidic DES pretreatment resulted in not only the depolymerization but also the condensation of the lignin fraction (Shen et al., 2019a; Shen et al., 2019b). However, Wan group found that acidified DES was able to remove more than 76% lignin under mild conditions, and the resulting lignin preserved β-O-4′ bonds and the structure was similar to the native lignin (Chen et al., 2018a, Chen et al., 2018b). As stated above, lignin suffered different chemical and structural changes via diverse DES pretreatment.
To investigate the lignin structural transformation during DES pretreatment, ChCl/FA was applied to pretreat AL at different temperatures (80, 100, and 120°C) for 6 h. The structure of the regenerated lignin was comprehensive analysis by nuclear magnetic resonance (NMR), gel permeation chromatography (GPC), and Fourier Transform Infrared (FT-IR) to verify the structural transformation of lignin in the selected DES. The depolymerized products of lignin were systematically determined via gas chromatography-mass spectrometry (GC-MS).
Methods and Materials
Materials
Triploid of Populus tomentosa Carr (6 years old) were obtained from the Shandong province, China. The poplar was ground, then extracted in a Soxhlet extractor with toluene-ethanol (2:1 v/v) for 6 h, finally milled for 1 h in ZrO2 bowl. Ball-milled wood (100 g) was treated with 1,500 ml of sodium hydroxide (1%) at 75°C for 3 h. The alkali lignin (AL) was separated based on the previous literature (Wen et al., 2014). The chemical composition of AL was analyzed according to NREL standard analytical procedure (Sluiter et al., 2008). The AL is composed of lignin (97.3%), total carbohydrates (1.1%), and others (1.6%). Choline chloride (ChCl) and formic acid (FA), purchased from Sigma-Aldrich, were used to synthesize DES at molar ratios of 1:2.
The Dissolution of Alkali Lignin
0.3 g AL and 6.0 g DESs were loaded in a pressure tube, which was treated at different temperatures (80, 100, and 120°C) for 6 h with 500 rpm. After finishing, samples were cooled down to room temperature. Then, the mixture was slowly added to acidic water (pH = 2) (40 ml) with 800 rpm for 20 min. Finally, the solid was collected via centrifugation at 10,000 rpm for 20 min, and then washed with acidic water for triplicate. The solid sample was lyophilized for 24 h to gain the regenerated lignin sample that was labeled as FA-X (e.g., FA-80). Meanwhile, the 50 ml supernatant was extracted with 400 ml ethyl acetate at room temperature. The mixture was stratified to take the organic phase. Subsequently, after removing the ethyl acetate at 35°C by rotary evaporation, lignin oil was obtained and label as F-X (e.g., F-80). FA and F referred to DESs type. X referred to the DES reaction temperature. All experiments were conducted two times.
Result and Discussion
The alkali lignin (AL) under the mild alkaline treatment was used as lignin model compounds due to the relatively low carbohydrates, no significant structural changes to the polymer backbone, nor condensation, which was close to the structure of protolignin in lignocellulose (Sun et al., 2000). Meanwhile, NMR analysis showed that AL remained various chemical linkages (e.g., 62.0% of β-O-4′ linkages) and no condensation occurred in the mild alkaline condition ( Figures 1 and 2 ), which was similar with Liu group’s AL in mild alkaline treatment (Liu et al., 2019a). ChCl/FA was selected to treat AL, due to its high delignification ability (Muley et al., 2019; Kohli et al., 2020). Herein, AL was pretreated with ChCl/FA DES at different temperatures, and then precipitated as the regenerated lignin. Results suggested that 58% of the regenerated lignin was obtained at 80°C after DES pretreatment (Table 1). The relatively low recovery yield indicated that some lignin fragments were degraded to small and soluble lignin fragment during DES pretreatment. Moreover, with the rise of pretreatment temperature, the recovery yield of lignin after DES pretreatment slightly decreased. The detailed structural transformation of the regenerated lignin was characterized by NMR, GPC, and FT-IR. The depolymerized products acquired after ChCl/FA DES processing were analyzed by GC-MS technology.
Structural Analysis of the Regenerated Lignin
Fourier Transform Infrared Analysis
FT-IR spectra were used to assess the lignin structural changes during DES pretreatment (Supplementary Figure S1). The peaks were assigned according to previous literatures (Faix, 1991). All of the regenerated lignin presented a similar peak pattern, while the intensity of these peaks was diminished as compared to that of AL. The wide FT-IR band at 3,448 cm−1 (hydroxyl groups) did not change strikingly, thus needing further analysis by 31P spectra. Supplementary Figure S1 showed that the peak intensity at 2,938 cm−1 (C-H stretch in methyl and methylene group) started to decrease slightly after pretreatment, implying that the DES pretreatment could result in demethoxylation reaction. The intensity of bond at 1,657 cm−1 (conjugated carbonyl absorption) became weak, whereas the newly appeared absorption peak around 1739 cm−1 (unconjugated ketone carbonyl and carboxyl carbonyl groups) was observed in the regenerated lignin. The possibility is that the acidic DES pretreatment resulted in the breakage of β-O-4′ bonds, thus appearing the unconjugated carbonyl groups, which is agreement with the subsequent GC-MS result of forming of Hibbert’s ketone (unconjugated carbonyl chemicals). Recently, Song group proposed reaction pathway of β-O-4′ bonds breakage in lignin using acidic DES, and also found the monoketone compound with the unconjugated carbonyl groups (Wang et al., 2020). Another reason was that esterification may also occur between COOH group in FA and OH groups of γ position in lignin. The typical characteristic peaks at 1,600, 1,502, and 1,459 cm−1 represent aromatic skeletal vibrations, and 1,416 cm−1 corresponds to the C-H deformation combined with aromatic ring vibration. Particularly, the intensity of bonds at 1,330, 1,120, and 835 cm−1 decreased greatly, suggesting that S-type units could be degraded after the DES pretreatment. In addition, the signal at 1,024 cm−1 (aromatic C-H in-plane deformation) gradually got weak after the pretreatment, suggesting that condensation reactions prevented the in-plane deformation of aromatic C-H. The results indicated that DES pretreatment can destroy the lignin structure. However, the detailed transformations of the lignin structure need further confirmation by NMR spectra.
2D-HSQC Analysis
The interunit linkage changes of lignin samples before and after DES pretreatment can be characterized by 2D-HSQC. This technology is able to measure the specific carbon-hydrogen functionalities, which cannot be determined in 13C NMR. According to the previous literatures, the detailed signals of lignin were assigned in Supplementary Table S2 (Marita et al., 2001; Hallac et al., 2010; Sette et al., 2011; Meng et al., 2019).
In the side-chain regions (Figure 1), the peaks of β-O-4′ substructures and methoxyl groups were dominant in the regenerated lignin. After ChCl/FA DES process, the interunit linkages of lignin fragments changed. When comparing the spectra of AL and FA-80, the novel signals (marked with black) were observed, which was attributed to DES components. The signals of Cα-Hα in A substructures got weak at 80°C, and then completely disappeared at the high temperature, indicating that Cα in AL during DES pretreatment was modified. Moreover, the signals of Cβ-Hβ in the A substructures declined with the elevated temperature under DES pretreatment, indicating dissociation of β-O-4′ bonds. Note that the signal of Cγ-Hγ in A′ substructures was observed at 80 °C, suggesting that acylation could occur between γ-OH groups of lignin and COOH groups of FA. Nevertheless, the signal of A′γ substructures entirely disappeared at the harsh condition, possibly due to the deacylation reaction. Besides, the signals of the B and C substructures became weak with the increased temperature, which implied that partial C-C bonds (e.g., β-β′ and β-5′) were degraded. Some studies also reported that C-C bonds could break down during DES pretreatment (Shen et al., 2019a; Hong et al., 2020; Wang et al., 2020). The quantitative analysis of the interunit linkages revealed that amounts of ether bonds and C-C bonds decreased when the pretreatment temperature increased (Figure 2).
In aromatic regions (Figure 2), signals of syringyl (S) and guaiacyl (G) substructures were detected. After DES pretreatment, the chemical shift of S units were altered, which implied that condensation could occur. Meanwhile, the signal of C2-H2 in G units gradually diminished as the increased temperature, indicating the possible formation of the condensed G units. Furthermore, S/G ratio of lignin was also a reliable method to evaluate the structural variant of the regenerated lignin samples. Supplementary Table S1 clearly showed that S/G ratio in the regenerated lignin was relatively higher as compared to that in AL, and sharply raise when the temperature elevated. The possible reason for this result was that G-type units in lignin were prone to degraded or removed during DES pretreatment. Besides, it probably indicated that G-type units in lignin degraded more quickly than the S-type units. However, under the acidic lithium bromide trihydrate system at 110 °C, native lignin was depolymerized in the solid state with minimal condensation (Li et al., 2018). To effectively separate depolymerized lignin with a less condensed form from biomass, it is necessary to optimize DES pretreatment conditions.
31P-NMR Analysis
The depolymerization and condensation reaction in lignin significantly influence the content of hydroxyl groups (OH) that have a significant effect on lignin valorization. The variations of OH content in lignin before and after pretreatment can be probed by 31P NMR (Table 2; Supplementary Figure S2). It was found that the COOH amount in the regenerated lignin samples slightly increased after ChCl/FA DES pretreatment, suggesting that aliphatic OH may be oxidized into COOH. On the contrary, the content of aliphatic OH declined as the temperature increased, because of the dehydration and acylation reaction. For AL, the amount of guaiacyl OH was more than that of syringyl OH, which indicated that S-type substructures were inclined to form ether bonds. In addition, the significant increase of condensed guaiacyl OH was clearly observed. The result indicated that lignin experienced repolymerization, being in line with 2D-HSQC result. Furthermore, the amounts of phenolic OH in the regenerated lignin were more than that of AL. This was mainly because of the dissociation of β-O-4′ bonds, which was consistent with the result of 2D-HSQC analysis. However, the temperature did not significantly influence on the content of phenolic OH. This implied that significant amount of ether bonds was cleaved at the low temperature. In short, depolymerization and repolymerization of lignin could simultaneously occur during ChCl/FA DES processing, likewise observed in 2D-HSQC analysis.

TABLE 2. Quantification of the functional groups in the lignin samples by quantitative 31P-NMR method.
13C-NMR Analysis
To deeply explore the structural change of lignin in DES system, 13C NMR was used for the determination of AL and the regenerated lignin. The distinct peaks were identified as follows, such as 119.4 ppm (G6), 115.1 ppm (G5), 111.3 ppm (G2) and 104.4 ppm (S2,6), confirming that the lignin from poplar is S-G type lignin (Supplementary Figure S3. The Cα, Cβ, and Cγ of β-O-4′ bonds in lignin were located in 86.2, 72.4, and 59.8 ppm. These signal intensity of β-O-4′ bonds, etherified S4 (138.4 ppm) and S3,5 (152.7–152.3 ppm) units declined after DES pretreatment, while the increased intensity of non-etherified S3,5 and G3 units (147.2–147.4 ppm) were observed as compared to that of AL. The results proved that the ether linkages were dissociated during DES pretreatment. It was speculated that the signal located at 162.7 ppm was assigned to FA according to 2D-HSQC analysis. Quantification analysis of 13C NMR showed that the content of aromatic C-C in AL was relatively lower (1.66/Ar) than that in FA-120 (1.98/Ar), while the content of aromatic C-H was also observed to reduce from 2.40/Ar in AL to 2.13/Ar in FA-120 (Table 3). This result proved that ChCl/FA DES pretreatment also can result in repolymerization reaction, which was according to 2D-HSQC and 31P NMR analysis. Besides, the content of -OCH3 in FA-120 was relatively lower (1.40/Ar) than that in AL (1.65/Ar). The reduced content of -OCH3 indicated that demethoxylation reaction occurred during DES pretreatment.
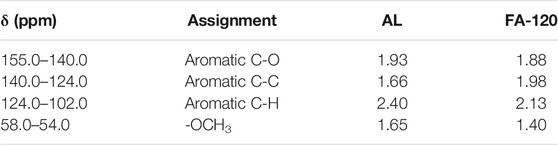
TABLE 3. The assignment and quantification of the signals of the 13C-NMR spectra (results expressed per Ar).
Molecular Weights Analysis
The changes of molecular weights in lignin have the great effect on the structure of lignin. The depolymerization reactions are conducive to decreasing molecular weights of lignin, while the formation of heterogeneous lignin structure with the increasing molecular weight is mainly attributed to the condensation reaction. In the present study, the weight average molecular weights (M w), number average molecular weight (M n), and the polydispersity index (PDI) in lignin were showed in Table 1. Herein, M w of AL was 5,080 g mol−1and PDI was 1.59. However, the M w of the lignin samples after ChCl/FA DES pretreatment sharply declined (2,230–1,640 g mol−1) with respect to that of AL, implying that depolymerization of lignin during DES pretreatment was the dominating reaction. Furthermore, PDI of lignin fragments after pretreatment was lower (1.17–1.20) with relation to that of AL, which showed the formation of more homogeneous lignin structure. In brief, ChCl/FA DES pretreatment can obtain the homogeneous lignin with the relatively low molecular weight.
Analysis of Lignin Oil
To improve the understanding of lignin structural transformation during DES pretreatment, the depolymerized compounds generated at the different temperature were identified by GC-MS analysis. After separating the regenerated lignin, the leftover liquid was extracted by ethyl acetate. After removing ethyl acetate, lignin oil containing degradation products of lignin was obtained (Supplementary Figure S4). The results were summarized in Supplementary Table S3. The depolymerized products were determined based on the previous literatures (Xiao et al., 2017; Lan et al., 2019; Li et al., 2019). These lignin oil included guaiacylacetone, guaiacyldiketone, syringaldehyde, syringylacetone, syringyldiketone and methylparaben, which were mainly composed of syringyl and guaiacyl derived phenolic compounds. Further, guaiacyldiketone and syringyldiketone were generated, indicating that the cleavage of ether bonds and oxidation reaction occurred after DES process. As depicted in Fig S5, the identified compounds enormously contained guaiacyl units with few syringyl units. This result indicated that G-type lignin could be prone to depolymerize than S-type lignin. However, the yield of depolymerized compounds was still low overall, due to the weak depolymerization of lignin in DES pretreatment. In addition, it was found that the reaction temperature notably impacted on the degraded products. The results showed that few degraded products were formed at a mild reaction pretreatment (i.e., 80°C), while the amount and kinds of degraded products increased when the temperature rose. This result implied that the higher temperature favored the depolymerization of lignin.
Conclusions
The present study deeply investigated the impact of the temperatures of ChCl/FA DES pretreatment on the structure and depolymerized products of lignin. The recovery yield of the regenerated lignin samples was around 44–75%, indicating a portion of lignin fraction was decomposed after pretreatment. Large amounts of β-O-4′ bonds with a fraction of carbon-carbon bonds (i.e., β-β′, β-5′) were cleaved when the reaction temperature increased, thereby leading to the increased phenolic OH groups and the decreased molecular weights. Besides, the decrease in aliphatic OH and OCH3 groups was attributed to dehydration and demethoxylation, respectively. Furthermore, the γ-OH in regenerated lignin can be reacted with formic acid into γ-acetylated groups in the mild condition; however, high temperature led to further deacylation reaction. Various monomeric phenols derived from AL were also determined in spite of low combined yields, which facilitate the production of fine chemicals in future. The homogeneous lignin with the high phenolic OH and low molecular weight was obtained after DES pretreatment, which is readily transformed into high value-added materials and chemicals. This study provided a systematic and comprehensive knowledge on lignin transformation during DES pretreatment, which would be conducive to lignin extraction and valorization.
Data Availability Statement
All datasets generated for this study are included in the article/Supplementary Material.
Conflict of Interest
The authors declare that the research was conducted in the absence of any commercial or financial relationships that could be construed as a potential conflict of interest.
Author Contributions
SH carried out all experiments. SH, X-JS, ZS wrote and revised the manuscript. T-QY designed the work and revised the manuscript. All authors discussed the results.
Funding
This work was supported by the National Natural Science Foundation of China (31971613 and 31670587), the Fundamental Research Funds for the Central Universities (2015ZCQ-CL-02), and the Beijing Forestry University Outstanding Young Talent Cultivation Project (2019JQ03005).
Supplementary Material
The Supplementary Material for this article can be found online at: https://www.frontiersin.org/articles/10.3389/fenrg.2020.573198/full#supplementary-material
References
Alvarez-Vasco, C., Ma, R., Quintero, M., Guo, M., Geleynse, S., and Ramasamy, K. K. (2016). Unique low-molecular-weight lignin with high purity extracted from wood by deep eutectic solvents (DES): a source of lignin for valorization. Green Chem. 18, 5133–5141. doi:10.1039/C6GC01007E.
Chen, Z., Bai, X. L., and Wan, C. X. (2018a). High-solid lignocellulose processing enabled by natural deep eutectic solvent for lignin extraction and industrially relevant production of renewable chemicals. ACS Sustain. Chem. Eng. 6, 12205–12216. doi:10.1021/acssuschemeng.8b02541.
Chen, Z., Reznicek, W. D., and Wan, C. (2018b). Deep eutectic solvent pretreatment enabling full utilization of switchgrass. Bioresour. Technol. 263, 40–48. doi:10.1016/j.biortech.2018.04.058.
Chen, Z., and Wan, C. (2018). Ultrafast fractionation of lignocellulosic biomass by microwave-assisted deep eutectic solvent pretreatment. Bioresour. Technol. 250, 532–537. doi:10.1016/j.biortech.2017.11.066.
Dong, H., Zheng, L., Yu, P., Jiang, Q., Wu, Y., Huang, C., et al. (2020). Characterization and application of lignin-carbohydrate complexes from lignocellulosic materials as antioxidants for scavenging in vitro and in vivo reactive oxygen species. ACS Sustain. Chem. Eng. 8, 256–266. doi:10.1021/acssuschemeng.9b05290.
Faix, O. (1991). Condensation indices of lignins determined by FTIR-spectroscopy. Holz als Roh-und Werkstoff. 49, 356. doi:10.1007/BF02662706.
Francisco, M., van den Bruinhorst, A., and Kroon, M. C. (2012). New natural and renewable low transition temperature mixtures (LTTMs): screening as solvents for lignocellulosic biomass processing. Green Chem. 14, 2153–2157. doi:10.1039/c2gc35660k.
Hallac, B. B., Pu, Y., and Ragauskas, A. J. (2010). Chemical transformations of Buddleja davidii lignin during ethanol Organosolv pretreatment. Energy Fuels. 24, 2723–2732. doi:10.1021/ef901556u.
Himmel, M. E., Ding, S.-Y., Johnson, D. K., Adney, W. S., Nimlos, M. R., and Brady, J. W. (2007). Biomass recalcitrance: engineering plants and enzymes for biofuels production. Science 315, 804–807. doi:10.1126/science.1137016.
Hong, S., Shen, X.-J., Pang, B., Xue, Z. M., Cao, X.-F., and Wen, J.-L. (2020). In-depth interpretation of the structural changes of lignin and formation of diketones during acidic deep eutectic solvent pretreatment. Green Chem. 22, 1851–1858. doi:10.1039/D0GC00006J.
Hou, X.-D., Li, A.-L., Lin, K.-P., Wang, Y.-Y., Kuang, Z.-Y., and Cao, S.-L. (2018). Insight into the structure-function relationships of deep eutectic solvents during rice straw pretreatment. Bioresour. Technol. 249, 261–267. doi:10.1016/j.biortech.2017.10.019.
Huang, C. X., Lin, W. Q., Lai, C. H., Li, X., Jin, Y. C., and Yong, Q. (2019). Coupling the post-extraction process to remove residual lignin and alter the recalcitrant structures for improving the enzymatic digestibility of acid-pretreated bamboo residues. Bioresour. Technol. 285, 121355. doi:10.1016/j.biortech.2019.121355.
Kohli, K., Katuwal, S., Biswas, A., and Sharma, B. K. (2020). Effective delignification of lignocellulosic biomass by microwave assisted deep eutectic solvents. Bioresour. Technol. 303, 122897. doi:10.1016/j.biortech.2020.122897.
Lan, W., de Bueren, J. B., and Luterbacher, J. S. (2019). Highly selective oxidation and depolymerization of α,γ‐diol‐protected lignin. Angew. Chem. Int. Ed. 58, 2649–2654. doi:10.1002/anie.201811630.
Li, H., Bunrit, A., Lu, J., Gao, Z., Luo, N., and Liu, H. (2019). Photocatalytic cleavage of aryl ether in modified lignin to non-phenolic aromatics. ACS Catal. 9, 8843–8851. doi:10.1021/acscatal.9b02719.
Li, N., Li, Y., Yoo, C. G., Yang, X., Lin, X., and Ralph, J. (2018). An uncondensed lignin depolymerized in the solid state and isolated from lignocellulosic biomass: a mechanistic study. Green Chem. 20, 4224–4235. doi:10.1039/C8GC00953H.
Lin, W. Q., Chen, D. F., Yong, Q., Huang, C. X., and Huang, S. L. (2019). Improving enzymatic hydrolysis of acid-pretreated bamboo residues using amphiphilic surfactant derived from dehydroabietic acid. Bioresour. Technol. 293, 122055. doi:10.1016/j.biortech.2019.122055.
Lin, W. Q., Xing, S., Jin, Y. C., Lu, X. M., Huang, C. X., and Yong, Q. (2020). Insight into understanding the performance of deep eutectic solvent pretreatment on improving enzymatic digestibility of bamboo residues. Bioresour. Technol. 306, 123163. doi:10.1016/j.biortech.2020.123163.
Liu, X. R., Li, Y. D., Ewulonu, C. M., Ralph, J., Xu, F., Zhang, Q., et al. (2019a). Mild alkaline pretreatment for isolation of native-like lignin and lignin-containing cellulose nanofibers (LCNF) from crop waste. ACS Sustain. Chem. Eng. 7, 14135–14142. doi:10.1021/acssuschemeng.9b02800.
Liu, Y. R., Nie, Y., Lu, X. M., Zhang, X. P., He, H. Y., Pan, F. J., et al. (2019b). Cascade utilization of lignocellulosic biomass to high-value products. Green Chem. 21, 3499–3535. doi:10.1039/C9GC00473D.
Liu, Y. Z., Chen, W. Z., Xia, Q. Q., Guo, B. T., Wang, Q. W., Liu, S. X., et al. (2017a). Efficient cleavage of lignin-carbohydrate complexes and ultrafast extraction of lignin oligomers from wood biomass by microwave-assisted treatment with deep eutectic solvent. ChemSusChem 10, 1692–1700. doi:10.1002/cssc.201601795.
Liu, Y. Z., Guo, B. T., Xia, Q. Q., Meng, J., Chen, W. S., Liu, S. X., et al. (2017b). Efficient cleavage of strong hydrogen bonds in cotton by deep eutectic solvents and facile fabrication of cellulose nanocrystals in high yields. ACS Sustain. Chem. Eng. 5, 7623–7631. doi:10.1021/acssuschemeng.7b00954.
Marita, J. M., Ralph, J., Lapierre, C., Jouanin, L., and Boerjan, W. (2001). NMR characterization of lignins from transgenic poplars with suppressed caffeic acid O-methyltransferase activity. J. Chem. Soc., Perkin Trans. 1, 2939–2945. doi:10.1039/b107219f.
Meng, X. Z., Crestini, C., Ben, H. X., Hao, N. J., Pu, Y. Q., Ragauskas, A. J., et al. (2019). Determination of hydroxyl groups in biorefinery resources via quantitative 31P NMR spectroscopy. Nat. Protoc. 14, 2627–2647. doi:10.1038/s41596-019-0191-1.
Muley, P. D., Mobley, J. K., Tong, X., Novak, B., Stevens, J., Moldovan, D., et al. (2019). Rapid microwave-assisted biomass delignification and lignin depolymerization in deep eutectic solvents. Energy Convers. Manag. 196, 1080–1088. doi:10.1016/j.enconman.2019.06.070.
Ragauskas, A. J., Beckham, G. T., Biddy, M. J., Chandra, R., Chen, F., Davis, M. F., et al. (2014). Lignin valorization: improving lignin processing in the biorefinery. Science 344, 1246843. doi:10.1126/science.1246843.
Rastogi, M., and Shrivastava, S. (2017). Recent advances in second generation bioethanol production: an insight to pretreatment, saccharification and fermentation processes. Renew. Sustain. Energy Rev. 80, 330–340. doi:10.1016/j.rser.2017.05.225.
Satlewal, A., Agrawal, R., Bhagia, S., Sangoro, J., and Ragauskas, A. J. (2018). Natural deep eutectic solvents for lignocellulosic biomass pretreatment: recent developments, challenges and novel opportunities. Biotechnol. Adv. 36, 2032–2050. doi:10.1016/j.biotechadv.2018.08.009.
Sette, M., Wechselberger, R., and Crestini, C. (2011). Elucidation of lignin structure by quantitative 2D NMR. Chem. Eur J. 17, 9529–9535. doi:10.1002/chem.201003045.
Shen, X.-J., Chen, T., Wang, H.-M., Mei, Q., Yue, F., Sun, S., et al. (2019a). Structural and morphological transformations of lignin macromolecules during bio-based deep eutectic solvent (DES) pretreatment. ACS Sustain. Chem. Eng. 8, 2130. doi:10.1021/acssuschemeng.9b05106.
Shen, X.-J., Wen, J.-L., Mei, Q.-Q., Chen, X., Sun, D., Yuan, T.-Q., et al. (2019b). Facile fractionation of lignocelluloses by biomass-derived deep eutectic solvent (DES) pretreatment for cellulose enzymatic hydrolysis and lignin valorization. Green Chem. 21, 275–283. doi:10.1039/C8GC03064B.
Sluiter, A., Hames, B., Ruiz, R., Scarlata, C., Sluiter, J., Templeton, D., et al. (2008). Determination of structural carbohydrates and lignin in biomass. Golden: CO: National Renewable Energy Laboratory (NREL).
Smith, E. L., Abbott, A. P., and Ryder, K. S. (2014). Deep eutectic solvents (DESs) and their applications. Chem. Rev. 114, 11060–11082. doi:10.1021/cr300162p.
Soltanian, S., Aghbashlo, M., Almasi, F., Hosseinzadeh-Bandbafha, H., Nizami, A.-S., Ok, Y. S., et al. (2020). A critical review of the effects of pretreatment methods on the exergetic aspects of lignocellulosic biofuels. Energy Convers. Manag. 212, 112792. doi:10.1016/j.enconman.2020.112792.
Sun, R., Tomkinson, J., Sun, X. F., and Wang, N. J. (2000). Fractional isolation and physico-chemical characterization of alkali-soluble lignins from fast-growing poplar wood. Polymer 41, 8409–8417. doi:10.1016/S0032-3861(00)00190-7.
Sun, Z., Fridrich, B., de Santi, A., Elangovan, S., and Barta, K. (2018). Bright side of lignin depolymerization: toward new platform chemicals. Chem. Rev. 118, 614–678. doi:10.1021/acs.chemrev.7b00588.
Tan, Y. T., Ngoh, G. C., and Chua, A. S. M. (2019). Effect of functional groups in acid constituent of deep eutectic solvent for extraction of reactive lignin. Bioresour. Technol. 281, 359–366. doi:10.1016/j.biortech.2019.02.010.
Wang, S., Li, H., Xiao, L.-P., and Song, G. (2020). Unraveling the structural transformation of wood lignin during deep eutectic solvent treatment. Front. Energy Res. 8, 48. doi:10.3389/fenrg.2020.00048.
Wen, J.-L., Yuan, T.-Q., Sun, S.-L., Xu, F., and Sun, R.-C. (2014). Understanding the chemical transformations of lignin during ionic liquid pretreatment. Green Chem. 16, 181–190. doi:10.1039/C3GC41752B.
Xiao, L.-P., Wang, S., Li, H., Li, Z., Shi, Z.-J., Xiao, L., et al. (2017). Catalytic hydrogenolysis of lignins into phenolic compounds over carbon nanotube supported molybdenum oxide. ACS Catal. 7, 7535–7542. doi:10.1021/acscatal.7b02563.
Zhang, C.-W., Xia, S.-Q., and Ma, P.-S. (2016). Facile pretreatment of lignocellulosic biomass using deep eutectic solvents. Bioresour. Technol. 219, 1–5. doi:10.1016/j.biortech.2016.07.026.
Keywords: lignin, deep eutectic solvents, pretreatment, nuclear magnetic resonance, structural transformation
Citation: Hong S, Shen X-J, Sun Z and Yuan T-Q (2020) Insights into Structural Transformations of Lignin Toward High Reactivity During Choline Chloride/Formic Acid Deep Eutectic Solvents Pretreatment. Front. Energy Res. 8:573198. doi: 10.3389/fenrg.2020.573198
Received: 16 June 2020; Accepted: 21 August 2020;
Published: 30 October 2020.
Edited by:
Li Shuai, Fujian Agriculture and Forestry University, ChinaReviewed by:
Yanding Li, Massachusetts Institute of Technology, United StatesSomnath D. Shinde, Sandia National Laboratories (SNL), United States
Copyright © 2020 Hong, Shen, Sun and Yuan. This is an open-access article distributed under the terms of the Creative Commons Attribution License (CC BY). The use, distribution or reproduction in other forums is permitted, provided the original author(s) and the copyright owner(s) are credited and that the original publication in this journal is cited, in accordance with accepted academic practice. No use, distribution or reproduction is permitted which does not comply with these terms.
*Correspondence: Tong-Qi Yuan, ytq581234@bjfu.edu.cn