- 1Institute of Energy and Sustainable Development, Faculty of Computing, Engineering and Media, De Montfort University, Leicester, United Kingdom
- 2Centre for Academic Innovation, De Montfort University, Leicester, United Kingdom
The symbiosis between smart minigrids and electric mobility has the potential to improve the cost and reliability of energy access for off-grid communities while providing low-carbon transport services. This study explores the commercial viability of using electric vehicles (EVs), recharged by solar minigrids, to provide transport services in off-grid communities. Preliminary findings are presented from a field trial in The Gambia that aims to assess the techno-economic feasibility of integrating sustainable energy and transport infrastructures in sun-rich regions of the Global South. As a dispatchable anchor load, an EV can improve the technical and economic performance of a minigrid by providing demand-side response services. In the developing world, rural communities are often among the poorest, and inadequate transport services remain a major barrier to wealth-creation. Some solutions to this situation may be transferrable to island communities, which share similar challenges in terms of access to energy and fuel. The first of its kind in Africa, this field trial uses an electric minivan, operating from an off-grid village where it has access to a minigrid whose 4.5 kWp of photovoltaic modules form the roof of a parking shelter for the vehicle. While there, the taxi can recharge, ideally during sunny periods when the photovoltaic array produces surplus power, thus allowing the EV’s battery to recharge while bypassing the minigrid’s own accumulator. This improves system reliability and cost effectiveness, while providing pollution-free energy for the taxi. Ultimately, the intention is to test different vehicles in a variety of circumstances, but this paper outlines only the preliminary findings of the first of these trials. Early results provide convincing new evidence that commercial viability of such a concept is possible in Sub-Saharan Africa. Some promising scenarios for commercial viability are identified, which warrant further investigation, since they suggest that a taxi driver’s earnings could be increased between 250 and 1,300% in local operations, and even 20-fold in tourist markets, depending on vehicle type, minigrid configuration and target market. It is hoped that these may encourage the rollout of solar-recharged EVs where the nexus of sustainable energy and transport systems are likely to make the greatest contribution to addressing the UN’s Sustainable Development Goals, by helping to solve the trilemma of providing energy security, social benefit and environmental sustainability in low-income countries.
Introduction
Improving access to affordable and reliable energy supplies is widely considered to be key to tackling the poverty trap across the developing world and thus the United Nations Sustainable Development Goal (SDG) Number 7, which is expressed as ensuring “access to affordable, reliable, sustainable and modern energy for all” (United Nations, 2015: p. 21). This challenge is particularly acute in rural communities where national electricity grids have not yet reached, or in many cases, may never be a viable proposition. Here, off-grid electricity supplies are typically provided through community-level minigrids, stand-alone household systems, or even on a per-device basis.
In an effort to alleviate poverty in the Global South, there is a growing emphasis on providing electricity for “productive uses” that create income (Peters et al., 2011; Mayer-Tasch et al., 2013). However, it is becoming evident that, while an electricity supply may indeed improve the productivity of micro-businesses in rural communities, earning more income is difficult if the extra goods produced cannot be transported to market (Pueyo and Hanna, 2015). Often, the limit of product sales is determined by the amount of goods that can be carried by one person to the nearest market, rather than the amount that can be produced back at home (Starkey et al., 2002). Thus, income growth in rural communities also depends on the transportation of more product to wider markets (Afukaar et al., 2019). Indeed, there is growing evidence that the lack of access to markets can undermine any benefit from increased productivity and can be counterproductive when a higher energy bill is not offset by increased earnings (Pueyo and Hanna, 2015). Unless money comes into the village from outside, there is no net gain to the community, because increased production does not necessarily bring more sales in a very limited market. In a small, relatively isolated community, if one producer of a certain product were to increase productivity thanks to having access to electricity, while another producer of the same goods does not, the competitive advantage of the first can be disruptive to the community because one family thrives while another is plunged deeper into poverty. Conversely, it can make a business unprofitable, by introducing the extra expense of an electricity bill, without necessarily recouping it through additional sales. Access to a market beyond the village, therefore, means that extra productivity can bring additional income into the community, both to the producer of the goods and to the local businesses where he or she spends the extra money earned.
What is particularly innovative about the subject of this study, known as the Solar Taxi project, is that it tests the viability of using electric vehicles (EVs) that are recharged entirely from solar-powered minigrids, for providing transport services. In doing so, it integrates solutions to both electricity access and transport provision into one holistic solution (Mathiesen et al., 2015) by exploiting the symbiosis between a flexible “anchor load” and a minigrid, such that the former provides load-balancing services to the latter.
Extensive research has been carried out on the use of renewable-powered minigrids across the Global South and their potential for alleviating poverty and improving quality of life (Bhattacharyya, 2014; Sakiliba et al., 2015; Baurzhan and Jenkins, 2016). At the same time, the emergence of a significant and rapidly growing EV market (International Energy Agency, 2020) has been underpinned by a vast body of research, as described by (Kumar and Revankar, 2017). However, its focus has generally been on the use of EVs in industrialized economies of Europe, North America and Japan (Poullikkas, 2015), plus China (Wang et al., 2017)—the world’s biggest producer of EVs–and increasingly, India (Mohanty and Kotak, 2017). As summarized by (Richardson, 2013; Daina et al., 2017), much research has addressed the effects that EV recharging has on electricity networks and especially how it can be optimized for demand response services in support of “smart grid” operations. The potential for recharging EVs with solar energy has recently been garnering interest, with several theoretical studies undertaken (Bhatti et al., 2016; García and Jeffrey, 2017), but only a few systems actually implemented, predominantly in the United States (Goldin et al., 2014; Mueller and Mueller, 2014). Having an abundant solar resource, Africa is a good region to consider for solar recharging, as shown by Buresh et al. (2020), but research on EVs and, in particular, the solar recharging of them, has been limited to small field trials on grid-connected urban systems run by the eMobility Technology Innovation Programme at the Nelson Mandela University in South Africa (uYilo, 2020).
The Solar Taxi pilot is therefore a novel demonstration of the intersection of various fields of research to create an integrated PV-to-EV system and presents a ground-breaking and widely-applicable mode of operation that has the potential to be transformative for many communities, especially in some of the poorest regions of the world. It is unique in demonstrating the feasibility of the recharging of EVs from a stand-alone solar minigrid in an off-grid community. The field trial provides a platform for techno-economic and social science research. With the anticipated introduction of remote digital data acquisition capabilities to both vehicle and minigrid in 2021, the ability to capture accurate high-resolution empirical data in real-world operations will enable analysis of technical factors such as energy efficiency, reliability and functional utility, as well as economic aspects, in a range of settings.
There are two major reasons why a flexible, or “dispatchable,” anchor load can be helpful to a minigrid. Firstly, the paltry income obtainable from small domestic electrical loads is not enough to make the building and operation of a minigrid financially viable, so the presence of at least one substantial commercial load is usually required to justify the minigrid’s existence. Such a load is classed as an anchor load, because it is the one that secures the financial sustainability of the minigrid (Robert et al., 2018). Secondly, the “dispatchability” of demand–especially the larger “productive use” loads–is an important characteristic. This is the ability for the load to be adjusted to match the availability of power at any given time. In conventional energy systems, this was not an issue, because variations in demand could easily be matched by adjusting generator output through regulation of fuel input. Hence, we have become accustomed to energy supplies being highly dispatchable because fossil fuels have been such a convenient energy store. However, there is a growing need to shift some dispatchability to the demand side of energy systems, due to the inherent lack of dispatchability in most low-carbon energy sources (Zhang et al., 2013; Mesarić and Krajcar, 2015). Efforts to reduce fossil fuel use, for environmental, health and economic reasons, has led to an increasing reliance upon renewable energy generators, such as photovoltaics (PV), wind and micro-hydro power, including for powering minigrids (Sandwell et al., 2017; Moner-Girona et al., 2018). It is therefore beneficial to have demand loads that can be time-shifted to periods when PV, wind or hydro power is available, otherwise the balancing of supply and demand on the minigrid necessitates a substantial energy storage facility. Batteries can provide this, but are expensive, temperamental, short-lived and often of poor quality and improperly managed or maintained, which frequently leads to the failure of minigrids (Crossland et al., 2015). Dispatchable loads can significantly reduce the reliance on batteries, by effectively bypassing them, and thus lengthening their service life, improving the reliability of the minigrid and lowering the cost of the whole system. Such techniques were developed and tested, for example, in the ESCoBox project, which introduced demand-side response (DSR) techniques into minigrids in the Global South (Harper, 2013; Gammon et al., 2016).
The range of dispatchable demand loads is relatively limited and typically requires the storage of energy or materials to make it possible. Examples include water pumping (where a water tank stores the potential energy of the water), heating or cooling (where thermal energy may be stored through high levels of insulation), or drying, food processing, goods fabrication (where storage of feedstock or product allows the time-shifting of process energy consumption). An EV, having a large onboard energy store, is an ideal facilitator of DSR functionality as long as the scheduling of journeys does not clash with the timing of recharging that harmonizes with renewable energy capture. In situations where this can be successfully coordinated, it has the potential to provide a pollution-free, convenient taxi service to the community, thus fulfilling the beneficial role of being a sizable, commercial, dispatchable anchor load for a renewable-powered minigrid. The Solar Taxi pilot scheme therefore seeks to determine whether an EV recharged from a solar-powered minigrid could provide low-cost mobility services to facilitate the influx of money into the community and, if so, whether a commercial model can be built around this combined mobility provision and minigrid support service to create a viable, sustainable business.
Besides the features of an EV that relate specifically to the aforementioned situation, there are a number of other more general advantages of EVs. Firstly, they do not use fossil fuels, so emit no greenhouse gases or particulates and their drivers are not vulnerable to oil price volatility. Secondly, having fewer moving parts than internal combustion engine vehicles (ICEVs), they are cheaper and easier to maintain and repair and, when the batteries eventually degrade to levels no longer suitable for motive power, they can be repurposed for stationary applications such as being used as a minigrid battery (Jiao and Evans, 2016). Thirdly, the electric drive-train of an EV offers new levels of flexibility in design and application with particular opportunities for the Developing World, where their torque characteristics are well suited to coping with challenging road conditions, their potential as mobile energy supplies may be of particular advantage, and, being able to recharge from any electrical source, they are liberated from the struggle to find an out-of-town refuelling station.
Although recharging with renewable electricity might seem to utilize free energy from sun, wind, or water, in reality, a tariff must be charged to recover the modest operational costs and comparatively high capital costs of a renewable-based electricity supply. This tariff, however, may be used to incentivize smart recharging, such that it is set at a low price when the renewables are generating enough power to support charging while bypassing the stationary battery, and a high price is charged when it is not. Employing such price structures, a minigrid operator can obtain income from energy that might otherwise be lost and increase both the operational and financial efficiency of the system. At the same time, the EV driver can get a discounted energy price if they choose carefully when they recharge. On the other hand, they still have the choice to pay a premium if they want to recharge at high-tariff periods. An enticing prospect for the longer term is the potential for an EV to provide vehicle-to-grid (or “V2G”) services in which the vehicle’s onboard battery can be used to store energy that can be fed back to the grid if and when needed. Such systems are not yet widely available but are currently being tested in a number of pilot projects (V2G Hub, 2020).
The nascent EV market is growing rapidly across the world, with a burgeoning variety of models on offer from OEMs and niche manufacturers. Although few, if any, existing models are well suited to the demands of driving in the Global South, the simplicity and flexibility of EV design options opens up the possibility for indigenous vehicle development and manufacture of vehicles more suited to conditions there. This might be a significant opportunity for emergence of a new automotive industry in areas of the Global South that have so far been locked out of the automotive market. The key to unleashing this potential will be cost, and the rapid development of automotive battery technologies holds out the promise that this barrier can be overcome. In the meantime, the mode of operation proposed in the Solar Taxi project is being explored as a possible early point of entry into the EV market for the Global South and, in particular, for Africa (Anyanzwa, 2019; uYilo, 2020).
Efforts to eradicate poverty and improve energy access in the Global South often fail where only one facet, such as the purely technical, has been addressed, without consideration of the social context in which it is implemented (Crossland et al., 2015; Gollwitzer et al., 2018). There are numerous examples of failed initiatives and undelivered promises resulting from fragmented and piecemeal approaches to international development that typically offer technical solutions without adequate reference to the social, cultural and behavioral aspects of people’s interactions with the technology (Touboulic and Ejodame, 2016). To avoid repeating such mistakes, the Solar Taxi project incorporates social science alongside engineering practices in an effort to produce socially and economically, as well as technically, viable solutions.
The Solar Taxi project aims to test the idea that using electric vehicles in the developing world would bring a number of benefits to poor communities, chief of which are economic growth and improved quality of life. Specifically, the anticipated benefits include:
• Increased access to markets for locally produced goods and services,
• Greater opportunity for entrepreneurial enterprises,
• Better access to health and education facilities,
• Reduced greenhouse gas and particulate emissions (with associated health benefits),
• Enhanced freedoms and opportunities for women and girls,
• Protection from volatile fuel prices and supply limitations,
• Improved technical and economic performance of community energy networks.
Methodology
A multidisciplinary approach has been taken to the gathering and analysis of data in this field trial such that technical, economic and social/behavioral aspects can be integrated. Hence, a whole-system mixed-methods approach was deemed necessary to this project if it is to result in meaningful positive outcomes and impacts.
The vehicle being used for this initial pilot of the Solar Taxi concept is a Nissan e-NV200 Evalia fully electric five-seater minivan. It features a 24 kWh capacity battery and an 81 kW traction motor that drives the front wheels, a top speed of 123 km/h (76 mph) and a claimed range of 170 km (105.63 miles), although the actual range in real-world conditions is more likely to be around 120–140 km (75–87 miles), depending on load, driving style, topography, etc. The 4.56 m long vehicle has a curb weight of 1,641 kg and gross weight of 2,220 kg, with cargo space of up 2.9 m³ with the three back seats removed. Figure 1 shows the Solar Taxi in operation in The Gambia.
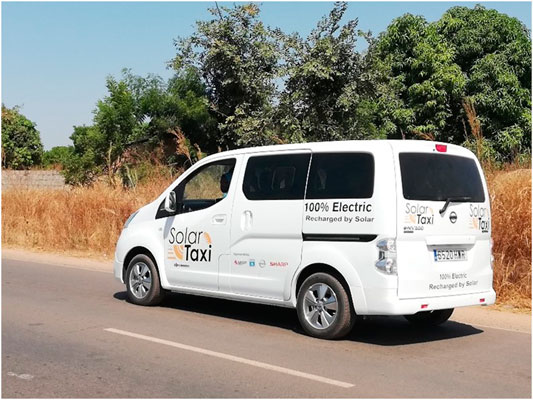
FIGURE 1. The Solar Taxi in operation between Manduar village and the market town of Brikama in The Gambia.
A number of other electric vehicle options were explored for suitability to this research exercise, including small and mid-range hatchbacks, a two-seater quadricycle and a three-wheeled autorickshaw, as modeled, e.g., by Bokopane et al. (2015). The quadricycle and autorickshaw both benefitted from being lightweight, which allows more traveling range for a given amount of energy input, but their load-carrying capacities and limited speed would restrict the range of markets they could serve. At the other end of the scale, the minivan has the advantage of being highly adaptable for the carrying of passengers and/or cargo, which enables it to be used in the testing of a variety of potential operational regimes. Shipping restrictions on certain vehicles, plus budgetary constraints also favored the e-NV200, which, on this occasion, was made available to the project at a highly-discounted nominal price.
Figure 2 illustrates how quickly the vehicle can be fully recharged after each day’s shift using a variety of standard charging rates. To recharge the vehicle from any 13 A 230 V socket, a “Mode 2” cable with a protection device, as shown in Figure 3, is needed since a 13 A circuit is only designed to accommodate that current over a period of a few minutes, e.g., when boiling a kettle, but is not able to operate at that level for several hours, as may be the case when recharging an EV. Thus, the control box on the cable (Figure 3) limits the charging current to 10 A to reduce the risk of overheating any wiring. Hence, the slowest standard charging rate for an EV is 10 A, which is currently the case for the Solar Taxi. To charge at 13, 16, or 32 A, a special recharging power outlet and cable is required, plus the installation of suitable wiring from the building’s main consumer distribution unit. In the case of a PV-powered minigrid, it also requires an inverter of higher AC output capacity, since a 10 A recharging rate equates to 2.3 kW, 13 A draws 2.99 kW and a 32 A recharger uses 7.36 kW. It is clear from Figure 2 that the rate at which the EV is recharged has a dramatic effect on the practicality of fitting a full recharge into the short period of high insolation during the middle of the day, and the ability for the taxi to quickly get back onto the streets to earn more money.
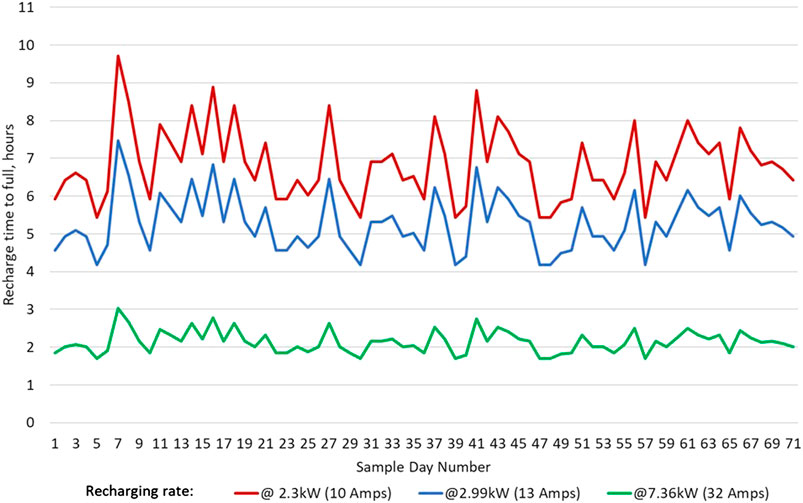
FIGURE 2. Comparison of recharging time needed to fully refill the battery following each day’s shift using different charging rates.
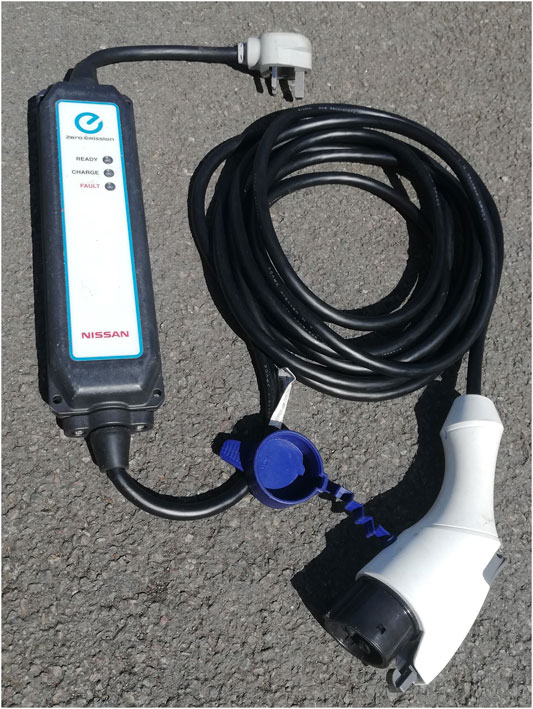
FIGURE 3. This Mode 2 cable has a protection device that allows an EV to be safely charged from a standard 13 Amp socket
The minigrid from which the Solar Taxi is recharged each day (as the weather allows) is powered by a 4.5 kWp array of PV panels that form part of the roof of a car port where the vehicle is parked while not in use (Figure 4). Aside from the recharging of the Solar Taxi, which is its main anchor load, the minigrid supplies the electricity needed to run the Global Hands Community Development Hub in the off-grid village of Manduar, which is situated approximately 3 km (two miles) from the local market town of Brikama in The Gambia (see map in Figure 5). Other loads on the minigrid include lighting, refrigeration, laptops, phone charging, cooling fans, and occasionally power tool recharging, a sound system or small cooker. Figure 6 shows a schematic of the minigrid system. A separate PV system is used to pump water for the site from a borehole. As a focus for community activity in the village, just off the main road to Brikama, the Hub is an excellent location for basing the Solar Taxi.
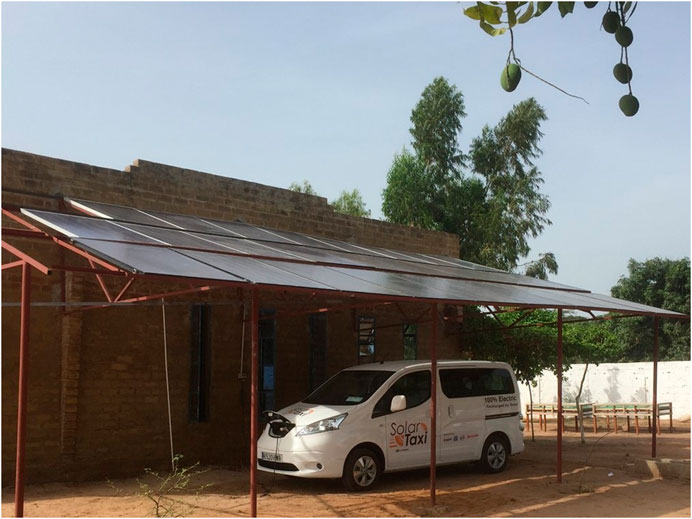
FIGURE 4. The Solar Taxi recharging from the minigrid at Manduar that is powered by a 4.5 kW PV array that forms part of the roof of its car port (Source: Gammon, 2019).
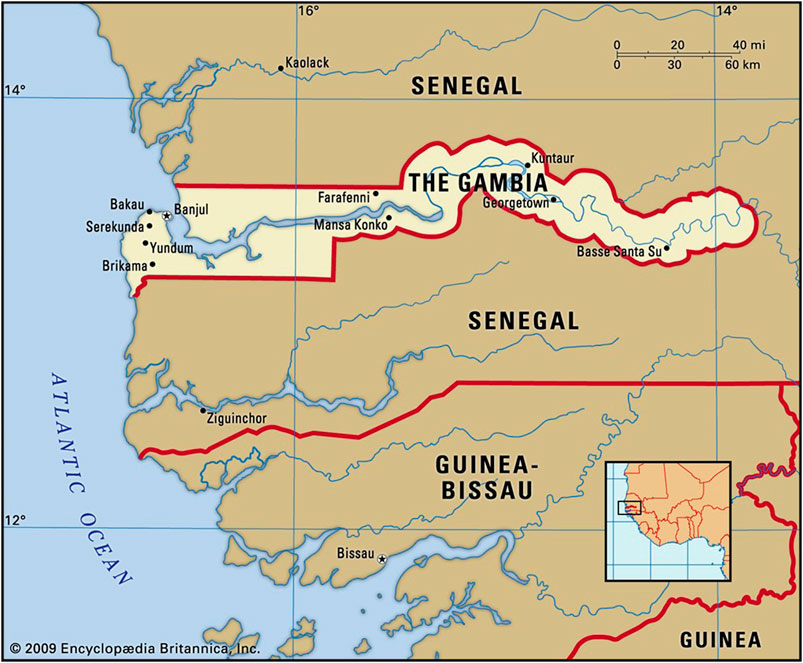
FIGURE 5. Map showing the location of Brikama in The Gambia, West Africa (By courtesy of Encyclopædia Britannica, Inc., copyright 2009; used with permission).
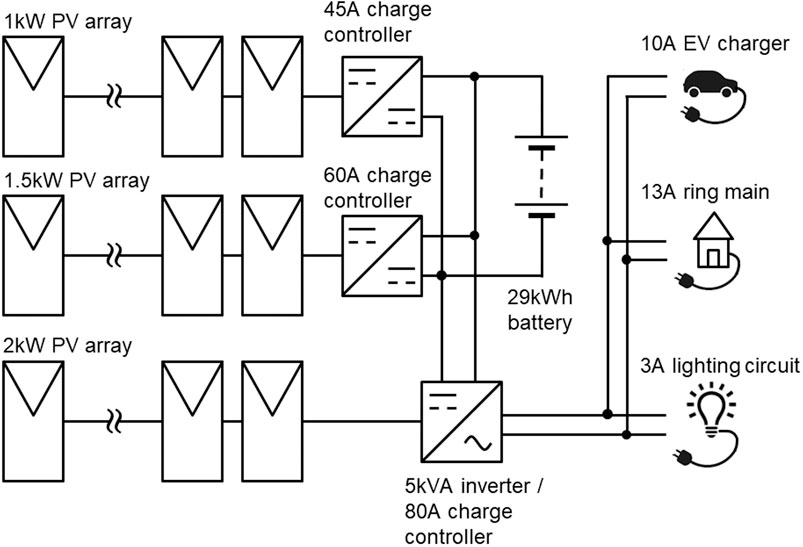
FIGURE 6. Schematic of the solar powered minigrid at the Global Hands Community Development Hub where the Solar Taxi is recharged.
As the Solar Taxi field trial was established with the support of donors who provided the vehicle and the PV modules essentially free of charge, the analysis of the current situation as it stands provides results that would be unrepresentative of normal economic reality. Nevertheless, this case is presented as a comparison with other more realistic scenarios and is the foundation upon which those scenarios are built. In all the scenarios analyzed, the capital expenditure (CAPEX) and operational expenditure (OPEX) of setting up and running the taxi service, with PV-only recharging, is used. Anticipated incomes are derived from the data obtained in the initial focus group meeting, plus the daily reporting from the field trial. Along with a range of electric taxi scenarios, the current situation with conventional internal combustion engine vehicles (ICEVs) is assessed for comparison, so that it is clear what the EVs must compete with in the taxi market. To assist in defining the scenarios, the ESCoBox decision Support Tool (DST) was used. This open-source software can be downloaded from (ESCoBox, 2017). This tool was originally developed to assist in the design and management of renewables-based minigrids, using incentivization through time of use (TOU) tariffs to encourage consumers to shift demand away from the hours of darkness to periods of high solar insolation to reduce costs and improve the reliability of minigrid systems. In this study, it was used to find the lowest-cost option for creating a solar-recharged taxi service in a range of scenarios and for optimizing the recharging schedule, which in turn dictates the taxi’s operational routine (see example screenshot in Figure 7).
The settings used in this analysis were fairly conservative to ensure some headroom in development of the business case for each scenario. This means that the cloud cover parameter was set slightly pessimistically and the date chosen was in the rainy season, so that the technical design covered the worst-case scenario for energy capture. The intention of this preliminary study is not to provide an accurate economic analysis or in-depth feasibility study. Instead, it is an outline assessment to ascertain which options deserve further detailed investigation on the potential for development and optimization of a full business case.
This pilot scheme benefitted from donations of the major components at negligible cost, so the results displayed in Table 1 reflect this current situation. However, all the other financial analysis undertaken for this study fully incorporates the cost of the donated items as if they were purchased at current market prices. References are provided in Table 2 for the sources of pricing information for each component. Thus, the CAPEX for the PV system is the sum of the cost of the PV modules, the inverter-charger (or just a charge controller, in the case of DC charging), the main battery accumulator, wiring and other minor electrical components, plus the cost of installation. Since operation and maintenance (O and M) costs for the PV system are negligible, the OPEX for it is essentially the annual cost of repaying any loan on its procurement and installation.

TABLE 1. Financial summary of current operating conditions for the Solar Taxi and a typical conventional taxi in the Brikama area of The Gambia.
The electricity cost, in Gambian Dalasi (GMD) per kWh, is calculated as the tariff required to pay off the CAPEX of the system over 36 months, based on daily energy consumption patterns recorded in the taxi operations.
For the EV, CAPEX is taken as the cost of buying the vehicle and shipping it to The Gambia (including import tax), while the OPEX is the cost of electricity to recharge it, maintenance costs and taxi operator’s license fee. In the case of the ICEV equivalent, the calculations are similar except that cost of repaying the loan is substituted with that of renting the vehicle from the owner as it is common practice for drivers rent their taxi from a third party. We have therefore assumed the usual rates for this when calculating the OPEX for a conventional ICEV taxi.
In all cases, loans are assumed to be repaid over 36 months with an exchange rate of 3.1% APR, which is a typical United Kingdom rate. The Gambian base lending rate of 12% is considered too demanding for most of the scenarios analyzed in this study, so an assumption is made that a sensible commercial strategy would be for the operation to be run through a United Kingdom-based parent company that could obtain financing at more favourable rates, while at the same time delivering jobs and capacity-building in The Gambia.
The drivers’ income is obtained entirely from taxi fares and, for simplicity, it is assumed that there are on average three passengers per journey (four leaving Brikama, one, two, three, or four going to Brikama for part or all of the journey). Tariffs are as indicated during the focus group meeting of local taxi drivers. Tourist rates tend to be considerably higher and are fairly variable, but the rates included in this assessment are at the very conservative (low) end of the range.
The results in Table 1 reflect the current situation, which is the only case in which equipment donations affect the results. In Tables 3, 4, these donations are ignored and current market prices are assumed for these items instead so as to provide a more realistic comparison.
Ahead of the technical trial of the Solar Taxi service, investigations were carried out to try to ascertain the potential viability of the system from the taxi drivers’ point of view, as they are the stakeholders most qualified to assess it. With an intimate knowledge of the local taxi market, the customer base and the practical aspects for providing the service, their experience would be invaluable in exploring the possibilities for employing this method of delivering an environmentally and commercially sustainable taxi business. Hence, on the July 2, 2017, a focus group was convened with five participants who all had several years’ experience as taxi drivers in the immediate locality. In the 2-h semi-structured discussion, the technical, economic and social aspects of the proposed Solar Taxi concept were explored and critically assessed by the taxi drivers. The main findings of this meeting, which are outlined in the Results section of this paper, provided the confidence to proceed to the technical trial and also helped to shape its implementation.
Technical trials of the Solar Taxi commenced a year later when the solar minigrid at the Manduar Community Development Hub was upgraded with the installation of a larger (5 kV A) inverter and the of construction of a car port with a 7.5 kWp solar roof, of which 4.5 kWp is connected to the solar minigrid that serves the EV charging and other loads at the Hub. The vehicle had been procured in the intervening year after the focus group meeting with the taxi drivers, and was shipped from the United Kingdom to The Gambia in time for the launch of the Solar Taxi service on the July 3, 2018. A local taxi driver was recruited to operate the service and to report on the distance traveled, fares collected and timing of working shifts for each day of operation. Approximate recharging times were deduced from this data and the incomes calculated on a temporal (per hour) and spatial (per km) basis. Days where recharging was not possible, due to extensive cloud cover, were also noted.
During the technical trial, the driver of the Solar Taxi recorded data for 71 days, spread across a 3-week period in August and September 2018 (toward the end of the rainy season), the whole of November and December 2018, plus a week in early February 2019 (in the dry season), followed by 11 days spanning the last week in 2019 and first few days of 2020. All except two (sample day numbers five and seven) were carried out as a single shift and most started in late afternoon/early evening and lasted, typically, from 4 to 6 h each. The start time, finish time, fare takings and distance traveled were recorded for each shift. The number of days sampled is modest and more robust data would be obtained from a larger sample size. However, the practicalities of the situation, predominantly relating to personnel issues and financial constraints, limited the scope of this initial pilot. Plans to improve on this in future, including the use of remote digital data collection techniques, which would, among other benefits such as improved accuracy and the recording of more parameters, be of particular value in allowing a meaningful energy efficiency analysis of the system to be carried out.
Results
The initial data-gathering exercise of the focus group with five local taxi drivers revealed the following key points.
• 50–60% of the drivers’ income from taxi fares is spent on paying for fuel. This was later corroborated with the help of online sources (Numbeo, 2020).
• The vehicles require regular (sometimes weekly) maintenance, due to the age and condition of the vehicles and the demanding conditions (long hours, poor roads) they must endure. Typically, taxis in The Gambia are old vehicles, imported second-hand from Europe and, since arriving in the country, have driven hundreds of thousands of kilometres and have had their engines (and other major components) replaced several times over.
• As this particular group of drivers was representative of a large cohort of local taxi operators working between the village of Manduar and the local market town of Brikama, most of their journeys were shuttling between these two locations that are 3 km apart, so the majority of journeys only last 10 min. Typically, a driver will wait until the taxi is full (with four passengers) before setting off from Brikama. However, traveling in the opposite direction, they could start off with as few as one passenger, but would be highly likely to pick up others on the way, often ending up with a full car by the time they have arrived in Brikama. Thus, it would be a reasonable assumption that the average passenger load would be three people.
• The fare to travel by taxi between these two locations is 10 GMD, equivalent to £0.25 in UK currency (GBP), which works out at 3.33 GMD per person, per kilometre. A taxi driver’s take-home pay in the Brikama area is around 30,000–36,000 GMD per year. Income can be significantly more than this in the main tourist and commercial areas of The Gambia, where, as an article in the local press would suggest (Secka, 2019), around 90,000 GMD is more typical.
• There is a predictable lull in demand for taxi services during the middle of the day, i.e., between morning and evening rush hours. This, of course, provides a suitable opportunity for recharging an EV from PV-derived electricity while the resource is at its most abundant and with minimal need for energy storage in another (off-board/stationary) battery.
• Many taxi drivers do not own their vehicle, but rent it from the owner for around 400–500 GMD per day, depending on its condition. Some are salaried and tasked with bringing in about 500 GMD a day.
• It was also noted that driving an EV, especially in heavy urban traffic, would be less physically demanding for the taxi drivers, since there are no gears or clutch pedal.
• The drivers believed, too, that the novelty of riding in a fully electric taxi that uses no fuel and is powered by the sun might mean that customers may be willing to pay a premium for the Solar Taxi service. This, however, was not put to the test during the trial as standard fares were charged throughout.
Figure 8 shows the time for which the Solar Taxi was operating and the distance it covered each day of the trial. It also indicates the rated range (as specified by the manufacturer) and the more meaningful “real-world” range that is typically experienced in use. The two large distance values arise from the driver carrying out a double shift with a recharge in between on days 5 and 7.
In Figure 9, the earning efficiency is expressed in terms of income per hour and per kilometre for each day in the local currency of the Gambian Dalasi. Currently, a Gambian Dalasi (GMD) is equivalent to £0.0158 (GBP), or $0.0198 (USD). It is evident from this graph that the double shifts in day 5 and particularly day 7 were not a good strategy in terms of earnings per hour or per kilometre, and this is why the driver decided against repeating the experience over the rest of the trial.
No distinction was made between weekdays and weekends because there is little difference in working routine over the weekly cycle. The mean rate of earnings across the Solar Taxi trial was 131.31 GMD per hour and 5.61 GMD per kilometre. It is not clear, however, how many passengers were traveling at any given time, as it would be too complex a task to expect of the taxi driver to report on this, given that there is plenty of ride-sharing in The Gambia, but often it might be for only part of the journey. From the focus group discussion with the taxi drivers, plus direct personal experience, it was determined that the average taxi fare in the Brikama/Manduar area was 3.33 GMD/km per person (although this could vary significantly in different areas across the country). This implies that the Solar Taxi was carrying an average of 1.7 passengers per kilometre traveled. The discrepancy with the assumed average of three passengers in a conventional taxi arises primarily because the Solar Taxi was not able to begin its journey from Brikama at the town's taxi rank, which is where a vehicle would usually wait until it has a full complement of passengers before departing. Missing out on this opportunity clearly had a negative impact on the Solar Taxi’s load factor. This also raises the issue of the taxi drivers’ fear of competition from this new technology, which seems, from evidence reported by the Solar Taxi’s driver, to have been part of the motivation to exclude it from the taxi rank. This is a significant issue in the transition to sustainable mobility, especially in economically vulnerable regions where it is particularly important that there is a “just transition” (McCauley and Heffron, 2018) and that “no-one should be left behind.” However, these matters are not discussed in this paper. Suffice to say, throughout this pilot of sustainable transport services, it has been stressed that the Solar Taxi is not trying to compete with conventional taxis, but is paving the way for those same drivers to ply their trade in a more sustainable, comfortable, healthy, and potentially profitable, manner. For the purposes of the following techno-economic analyses, this disparity is ignored and it is assumed that, in a future market, both technologies will be able to operate on a more even basis. Thus, in all scenarios, the same passenger load factor is assumed for equivalent vehicles.
In addition to the 71 operational days, the Solar Taxi driver reported on days when cloud cover prevented the PV from supplying enough power to recharge the vehicle and also on days when insolation was sufficient but he had to take a day off for other reasons. Thus, the total number of days reported were 124, of which 90 (73%) were sunny enough to recharge the taxi, but 19 were of these were taken as days off. With the trial taking place across both wet and dry seasons in similar proportions to the lengths of the respective seasons, the percentage of sunny days in the trial can be treated as fairly representative of that of a whole year. This was then taken into account when analyzing the results to assess the potential for commercial operations of a Solar Taxi service with a variety of vehicle types. It was also assumed that the driver would normally work 6 days a week, 52 weeks of the year, which is typical of a taxi driver’s routine in The Gambia.
Only four morning shifts were undertaken during the trial, twice in the first week and once each in the following 2 weeks. This is because it quickly became evident that these were not as lucrative or as practical as the evening shifts. This could be due to traffic congestion in the morning rush hour, where the benefits of operating when there is high demand for taxis (supplier’s market) might be outweighed by the slow travel speeds if charging fares on a per-mile (rather than per minute) basis. Alternatively, it could be a result of the smart recharging regime required for the EV taxi. In the latter case, serving the morning rush-hour market demands that some energy be left in the EV’s battery overnight since it was recharged during the previous day. So, after recharging during the hours of peak solar irradiation, the driver must choose whether to expend the stored energy on that day’s evening shift, the next morning’s shift, or to divide it between both, before the opportunity comes for the next recharging session in the middle of the day. In terms of saving the time and distance that might be traveled without picking up passengers on the way to or from the Hub, there is an incentive to use up the battery’s capacity in a single shift. This then forces a choice between morning or evening shift, as doing both is not considered worthwhile, which leads to a simple assessment of which is the most profitable of the two. This situation may vary for different vehicles, at different locations and different distances from their key markets, and optimization of such factors will be undertaken in future work.
The mean earnings for the Solar Taxi service during this trial was 5.61 GMD/km (£0.87/km), which equated to an average of 131 GMD/h (£2.07/h). This is not the same as the tariff per person, per km, as the number of passengers for each journey was not recorded. If the standard local tariff were charged, this would suggest that the Solar Taxi tended to have a lower load factor (average number of passengers) than a conventional taxi, which would typically expect 10 GMD/km.
Analysis and Discussion
The core question that the Solar Taxi pilot seeks to answer is whether an electric taxi service–particularly one that is recharged entirely from solar energy (and is therefore genuinely providing zero-emission mobility)—is a viable proposition in sun-rich parts of the world. It is clear that it is technically viable, but the following analysis explores whether it is also commercially feasible, based on the data obtained in a field trial that uses The Gambia, in West Africa, as its case study. As discussed previously, there are particular reasons why a Sub-Saharan location should be chosen for this pilot study and chief among these is the presumed benefits of providing affordable and sustainable mobility to some of the world’s poorest and most disadvantaged regions. In such straitened circumstances, it is self-evident that driving down costs is critical to meeting this target. Hence, our techno-economic analysis focusses on defining circumstances in which commercial viability may be achieved.
The financial overview provided in Table 1 compares the operating conditions of the Solar Taxi with a typical conventional taxi working between Brikama and Manduar during the period of the field trial. The electricity cost is calculated on the basis of repaying the CAPEX for the solar energy system over a 36-month period using compound interest. For the current situation, this does not include the purchase of the PV modules, which were donated free of charge for the pilot, but does include the minigrid’s inverter and battery, plus wiring, materials and minor equipment costs, and labor for installation (estimated to be about £1,000). The OPEX includes normal O and M costs, plus an amount that represents repayment of the cost of shipping the vehicle to The Gambia from the United Kingdom, where relevant. Since the vehicle was donated almost free of charge for this pilot scheme, there is of course no financing cost included in this instance for the purchase of the vehicle. In all other cases, where the CAPEX of PV and vehicle are included, loan repayments are calculated as outlined above. Fuel costs and fare tariffs are as reported by the taxi drivers and verified through Numbeo (2020).
From the comparison in Table 1, it appears that the major impediment to making a profit for the conventional ICEV taxi drivers is the cost of fuel, whereas the EV taxi incurs a much lower energy cost. However, due to the distorting effects of the donations of both the vehicle and the PV modules to the Solar Taxi pilot project, the first financial analysis was heavily skewed in favor of the EV taxi service. Therefore, further analysis was undertaken to provide a more realistic and informative comparison that includes the purchase cost of both the EV and PV elements under more normal circumstances.
To explore the potential commercial viability of EVs for providing public transport services, it is necessary to consider a range of vehicles and journey types. This first trial of the Solar Taxi, for example, was focused on (but not exclusive to) a particular route, yet the experience of running this pilot strongly indicates that, without the substantial donations of expensive equipment, this would not be a viable proposition with this particular vehicle. The Nissan e-NV200 five-seater minivan with substantial luggage or spare seating capacity, would be much better suited to ferrying tourists between the airport and their hotels, or taking them to visit local attractions. Tourists, being much richer than the vast majority of local people, are able and willing to pay a significantly higher price for mobility than local people. This proposition has been key to the establishment of Opibus, which is a company in Kenya that converts rugged safari vehicles from ICEVs to EVs (Opibus, 2017). Furthermore, during the Solar Taxi field trial, the diver did not adhere closely to the principle of only recharging during the hours of maximum solar insolation, but instead relied frequently on the storage capacity of the Manduar Community Development Hub’s substantial minigrid battery to prolong the recharging period beyond what would have been possible with a system optimized purely for EV taxi operations. This meant sacrificing the full utility of the Hub’s electricity supply for other users, which is usually not a problem, except when there is a large group of visitors already placing high demands on its minigrid system.
The Nissan e-NV200 is a large, comparatively heavy vehicle, designed for the industrialized economies and temperate climates of the Global North. It consequently has a high energy demand, which necessitated a large minigrid and PV system at the Hub to support it. The Brikama-Manduar shuttle could equally well be served, e.g., by tuk-tuks (autorickshaws). These are small, lightweight, 3-wheeled vehicles that are well suited to the local conditions as they originated in India, are in widespread use across Asia, and are now gaining a foothold in certain parts of Sub-Saharan Africa (SSA) too (Bokopane et al., 2015). With a significantly lower energy consumption per kilometre traveled than a car or van, an electric tuk-tuk would require a much smaller PV system to recharge it. Most tuk-tuks can carry three passengers in addition to the driver, but there are versions available that can carry six passengers, and this is the version that will be considered in the following analysis. Also under consideration are a Nissan Leaf, which is a mid-sized hatchback car, a Renault Zoe, which is a smaller hatchback, a three-wheeled cargo bike (which can be configured to carry goods or up to six passengers) and a two-wheeled motorbike. All are EVs that are readily available on the market and, for the purposes of this study, the smaller vehicles are assumed to be new, whereas the cars would be more likely to be shipped from Europe as second-hand vehicles, in accordance with common practice in SSA. Figure 10 illustrates the vehicles that are considered in this study as potential alternatives to the Nissan e-NV200 minivan.
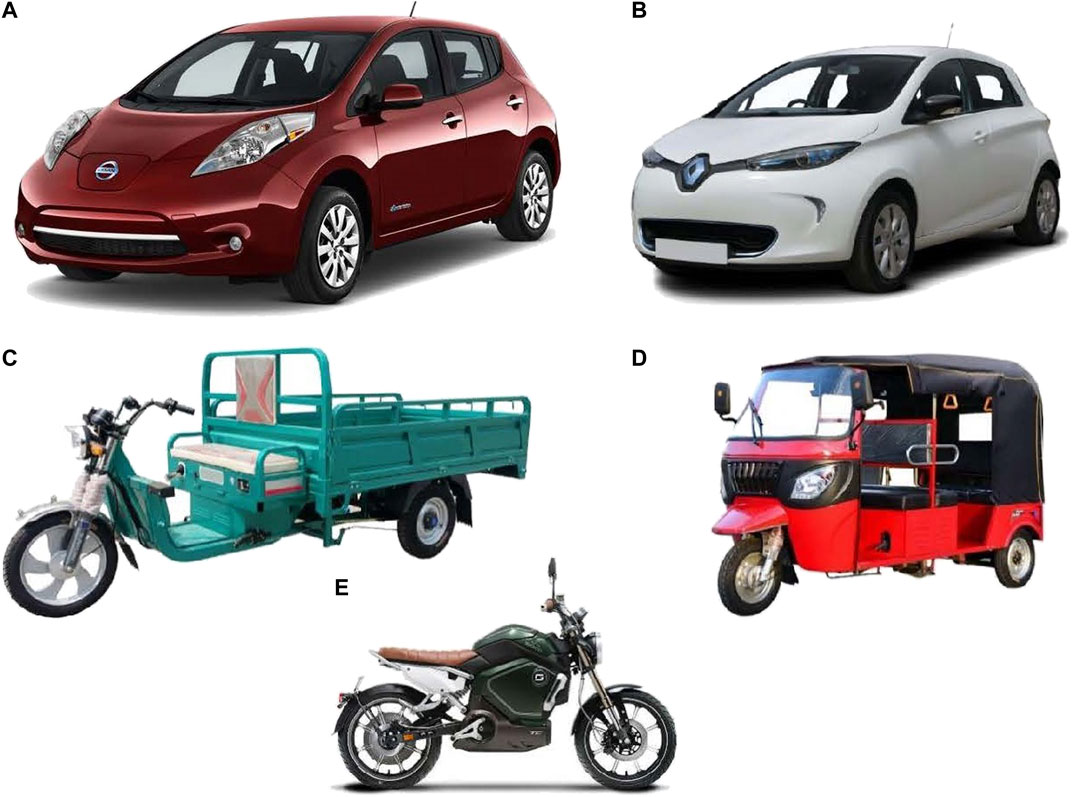
FIGURE 10. Illustration of vehicles being assessed as alternatives to the Nissan e-NV200 minivan used in the Solar Taxi field trial (Source: Nissan, 2017; Renault, 2016; Henan Zipstar Tricycle Manufacturing Co. Ltd., 2020; Xinxiang Siristar Import and Export Co. Ltd., 2020; Vmoto United Kingdom Distribution Ltd., 2019; used with permission).
An option that is also possible to consider in the case of the smaller vehicles (e.g., cargo bikes and tuk-tuks) is DC charging. With much smaller batteries operating at lower voltages, they could be recharged directly from the PV through a DC charge controller rather than via an inverter that converts it to AC before reconverting it back to DC to go into the battery. Dispensing with the inverter saves both electrical losses and unnecessary cost. This is not so easy to achieve with the larger vehicles and those smaller ones with sophisticated proprietary AC charging systems (e.g., the motorbike used as an example here, which has a swappable lithium-ion battery).
The two-wheeled e-motorbike is particularly suitable for remote villages where it can cope more easily with extremely poor road conditions than bigger vehicles, but can also be operated as a cheaper taxi service in areas that are likely to be more economically impoverished, where fuel is scarcer and more expensive, where there are minigrids that need an appropriate dispatchable anchor load. Confidence in this approach is what underpins the business model for Ampersand, which is a Rwandan company that has tested 20 e-motorbikes in Kigali and is now reportedly making 500 of them a year (Peters, 2020). Conversely, with tourism being one of The Gambia’s main sources of income, the e-NV200 is well suited to serving the numerous, comparatively wealthy, foreign visitors to the country in the busy commercial and tourist centers. In Kenya, Opibus is building its business on tourist dollars to underpin its planned rollout of EVs into the wider public transport arena (Opibus, 2017), while taxi operator Nopia is already running a handful of Nissan Leaf taxis in Nairobi with ambitions to have a fleet of 50 EVs by the end of 2020 (Kuhudzai, 2019).
EVs with a lower energy demand enable lower-cost PV systems to be used to recharge them, thus making the whole PV-to-EV operation more cost effective and potentially more commercially viable. Of particular importance is their potential to be recharged relatively quickly. An EV that can be fully recharged within 3–4 h, during the mid-day sunshine, places a much smaller demand on the PV-powered minigrid than one that takes six or more hours to do so, because the latter would inevitably require the minigrid to have its own large accumulator. The battery is often the most expensive component of the minigrid and, being the most susceptible to stress and misuse, is usually also its most short-lived. It is therefore advantageous to keep its size and operational fatigue to a minimum. Thus, each of the following scenarios is predicated upon designing the minigrid most suitable for the particular EV type and model in question, taking into account the EV’s battery size, recharging rate and anticipated daily range. Each also takes advantage of the drop-off in demand for taxi services during the middle of the day, as reported by the taxi drivers, as this provides an ideal opportunity to stop and recharge at the time of highest insolation.
For this study, a range of PV-powered minigrid configurations were designed, using combinations of the main building blocks of the system (i.e., PV array, inverter, battery), as outlined in Figure 11. In each case, an average achievable daily range for the Solar Taxi (Nissan e-NV200) is indicated. A basic cost-benefit analysis of each option clearly indicates that it is worth paying more for a PV system that allows greater range, because the returns are better. This is because the CAPEX is high and the OPEX low for both the solar minigrid and the EV, so it is important to operate at a high capacity factor to obtain good returns on the initial investment. In this way, a modest increment in initial setup costs leads to higher sustained income over the longer term. In the case of solar recharging of an EV, the additional PV and inverter capacities allow the EV to recharge more fully in a shorter space of time. The difference between a 5-h charge and a 6-h charge is more significant in terms of annual earning potential than is the saving achieved in purchasing a smaller system in the first place. Of the options outlined, only the 3.5-h configuration allows the e-NV200 to recharge fully on an average day and thus make use of its full traveling range.
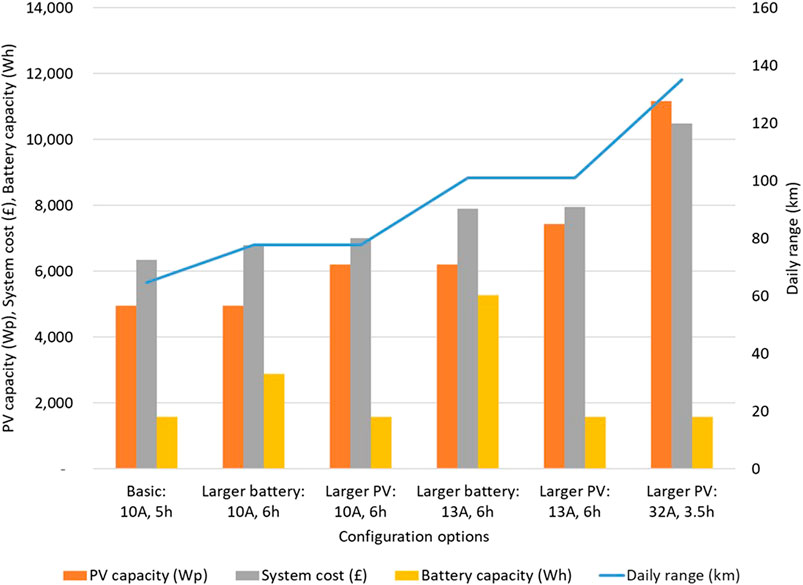
FIGURE 11. Different PV system configurations are assessed for commercial viability. For each, the mean daily EV range achievable is based on the Nissan e-NV200 used in the Solar Taxi pilot.
The first configuration in Figure 11 allows only 5 h of recharging through the current-limiting Mode two cable at 10 A without recourse to charging from the minigrid battery. Extending the recharge time to 6 h, while still using the 10-A cable, is possible by either increasing the PV capacity or the minigrid’s battery capacity. Recharging at 13 A is possible using an alternative standard (Mode 3) cable and specialist socket and so a 6-h charging period at this power produces a longer daily range. Again, the two options for modifying the PV system to accommodate this is either by increasing PV and inverter capacity or by increasing the stationary battery size. The final option illustrated is to recharge for 3.5 h at 32 A, which is the only configuration that enables a full recharge without resorting to the use of the stationary battery. Although the specific vehicle used for the Solar Taxi trial does not have this capability, newer versions of the same model are available with it included, so it is instructive to explore this option for this and other vehicles, such as the Nissan Leaf, which shares many of the e-NV200’s attributes, but in a hatchback car format.
The key assumptions that are used in the techno-economic analysis of a variety of vehicle and minigrid configurations are presented in Table 2. In the scenarios summarized in Tables 3, 4, the PV-powered minigrid has been treated as if it is owned and operated by a different party to the taxi operator. This is to accommodate the situations in which the taxi driver may simply be plugging into a minigrid that is not exclusively dedicated to, or owned by, the taxi operator, in which case the minigrid operator is going to want to make a profit in selling power to the taxi driver. If, however, the minigrid operator is also the taxi operator, their total revenue would be the sum of both the PV system’s profit and the EV taxi’s profit. CO2 emissions are included in the parameters listed for ICEVs, so that a rough estimate can be made of the greenhouse gases that would be avoided by substituting an ICEV with an EV. Assuming that the ICEV travels the same distance each day as the EV, the CO2 savings from a car would be 23.6 kg a year, 5.9 kg from a cargo bike, 7.0 kg from a tuk-tuk and 6.2 kg from a motorbike.
With the caveat that it is only a simple high-level assessment, the analysis presented here suggests that there are a variety of circumstances in which EVs that are recharged entirely with solar power could provide a commercially viable taxi service in the case study location of The Gambia and, by implication, the prospects would look similarly promising in many other sun-rich regions of the Global South. Most notable of the commercially viable markets identified are those serving the large tourism sector in The Gambia in which the minivan appears the most lucrative, with the potential for a taxi driver to earn nearly twenty times more than they would by working the Brikama-Manduar route in a conventional ICEV taxi. Depending on the model, a car might earn 12 or 13 times more, and a tuk-tuk two and a half times the Brikama taxi. The biggest barrier to a Gambian being able to grasp such an opportunity is the difficulty in getting credit and the high interest rate on loans. With such a high up-front cost to establish an operation such as this, finance would be essential to all but the wealthiest Gambians who wished to set up such a business.
In serving local people, rather than tourists, the returns look far less lucrative, but are nonetheless more promising than the current status quo for the taxi operators. The electric tuk-tuk appears especially attractive as it is a lightweight vehicle, and therefore more energy efficient than a heavy car, and is ideally suited to the conditions of most of the Global South. It is perhaps a lesson in the shortcomings of trying to adapt products from different worlds to try to fit them into an environment to which they are less suited, as is often the case with vehicles imported from the Global North. However, even in doing so, electric vehicles imported from Europe are shown by this analysis to offer a taxi driver the chance of improving their earning potential by up to a third, while imported lightweight EVs from the emerging economies of Asia could provide double the income of a conventional taxi. The motorbike looks the most marginal of options; however, it is important to note that this analysis is based very much on a specific peri-urban route, whereas the conditions in more remote locations (i.e., most of the rest of the country) are much more favourable to this type of transport. It would therefore be instructive to undertake a study on this particular market using more specifically targeted data and in greater depth than this brief overview has provided. This service is the most important in addressing the poverty trap experienced by many of the world’s most disadvantaged communities and, even just for that reason, deserves further exploration. Ampersand’s apparent success in Rwanda gives a strong indication that this approach could deliver the viable solutions that are needed (Ampersand, 2020).
While this study already provides some optimism for the future of EVs in the Developing World, it also offers some insight into the circumstances that might improve the prospects yet further. These include:
• Easier access to loans, with lower interest rates and longer payback periods;
• Government support through subsidies or reduced license fees or taxes for EVs, training and education in low-carbon transport systems, stronger emission regulations, removal of subsidies to the fossil fuel sector;
• Continued volatility of fossil fuel markets, or a return to higher prices;
• Further advances in low-carbon energy technologies such as batteries, power electronics and photovoltaics, with a continuation of the rapid downward trend in their cost;
• Increased opportunities for battery swapping systems in EVs and/or low-voltage DC charging directly from PV systems;
• Take-up of additional services that EVs can provide (e.g., V2G services, portable/mobile power supplies, roof-mounted PV on tuk-tuks, onboard solar-powered refrigeration in enclosed cargo bikes, etc.);
• Use of electric boats for fishing and tourism (especially for birdwatching, which is a major attraction for tourists in The Gambia);
• Increased choice of vehicle designs, especially those that are more appropriate for the Global South. For example, they can be simpler, with a smaller parts inventory and no need for unnecessary features like heating systems, making them cheaper to build and maintain and to run, but they must be rugged with strong suspension, good ground clearance, and be tolerant of heat, dust and mud;
• The introduction of TOU tariffs that incentivize recharging at times of high energy supply from renewables and low energy demand (and, potentially, incentivizes V2G operations when the opposite conditions arise);
• Increased demand diversity and improved economies of scale as minigrids grow, such that dispatchable EV charging loads can operate increasingly in harmony with other loads on the system using demand-side management techniques.
While many of the aforementioned factors cannot be addressed through the future work of the Solar Taxi project, there are several that we do intend to pursue. To tackle these effectively, the first and most important task is to install instrumentation on the e-NV200, and any other vehicles that are to be tested, to enable comprehensive data capture for a variety of parameters concerning the technical performance of the vehicle and its interaction with the solar minigrid. In particular, this would enable an analysis of the energetic efficiency of the system. This is another feature that draws on the experience of the ESCoBox project that developed data acquisition techniques for remote monitoring of off-grid energy systems (Boait et al., 2017). With this implemented, the vehicle will continue to work for some time predominantly in its current Manduar-based operations so as to verify the self-reported data so far supplied by the driver.
The preliminary findings of the Solar Taxi pilot reported in this paper indicate strongly that a thorough investigation should be made of smaller EVs operating as taxis in the Gambian context, and so the intention is to undertake trials of an electric tuk-tuk as soon as possible, which would allow the e-NV200 to transfer to the more lucrative tourism-focused market.
Having smaller vehicles involved in the Solar Taxi project would then open up the opportunities to explore directly-coupled DC charging and battery-swapping methods of replenishing the EV’s energy store. In such a case, batteries can be recharged during the day on the minigrid and quickly loaded directly onto the vehicle, while the discharged batteries are offloaded for recharge on the minigrid allowing the EV to immediately return to service. This avoids one cycle of charge and discharge losses each day and could be accommodated by judicious configuration of battery packs that can fit with local working practices and ergonomic factors. It does, however necessitate an extra battery for the EV, but with a fleet of several such EVs, there are economies of scale that mean the ratio of batteries to EV can reduce to less than 2:1 as the fleet grows.
Again, using the ESCoBox system, the sophistication of charge scheduling can be improved, such that it becomes more responsive to changing weather conditions, demand variations or energy price signaling, which can increase both technical and economic efficiency while improving the reliability of energy services. These iterations of the current system can be advanced through continuation of the Solar Taxi initiative. In the longer term, V2G could be implemented and even rapid charging that could operate at up to around 50 kW.
In the meantime, capacity-building in the local community and wider host country is considered key to realizing the aims of poverty alleviation, and the provision of good quality and sustainable employment. Consequently, we are working with Gambian education and training establishments to embed the growing knowledge about low-carbon energy and transport systems in their curriculum as a key part of this project.
Conclusion
The aim of the Solar Taxi project is to determine whether it is both technically and commercially feasible to provide a taxi service in sun-rich parts of the world using an electric vehicle that is recharged entirely from solar energy, thereby offering genuine zero-emission mobility. This has relevance to several of the UN’s Sustainable Development Goals, particularly in the fights against poverty, ill health and climate change, and in the provision of decent employment and sustainable communities. This paper has presented the preliminary findings of the Solar Taxi project based on initial results obtained in a field trial carried out in the West African country of The Gambia. The emphasis in this project has been on wealth-creation in remote and off-grid communities, especially in the Developing World, but it offers insights that are also relevant to island communities (Kuang et al., 2016).
The technical feasibility of the Solar Taxi concept is demonstrated by this field trial and, while it is only intended as a brief overview, the techno-economic analysis presented here provides confidence that a number of modes of operation are possible in which solar-recharged electric taxis can be commercially viable in The Gambia and, by implication, across much of the Global South. The most optimistic scenarios arise from the use of lightweight vehicles, such as tuk-tuks (autorickshaws) and cargo bikes, but electric cars and minivans also show commercial promise. In the effort to support the most remote and impoverished communities, electric motorbikes appear highly suitable and are already being successfully deployed in some parts of Sub-Saharan Africa. These findings suggest that the most promising options identified in this preliminary analysis warrant a full feasibility study that could lead to the development of a viable business case. The summary analysis presented here suggests that a taxi driver could double their income by transferring from a conventional combustion vehicle to an all-electric one and, in the case of providing taxi services to tourists, could even raise it nearly twentyfold.
Data Availability Statement
The raw data supporting the conclusions of this article will be made available by the authors, without undue reservation.
Ethics Statement
The studies involving human participants were reviewed and approved by International Board of Directors of Global Hands. The patients/participants provided their written informed consent to participate in this study.
Author Contributions
The contributions made by each of the authors of the paper entitled ‘Preliminary findings from a pilot study of electric vehicle recharging from a stand-alone solar minigrid’ were as follows: RG: Conceptualization; funding and equipment acquisition; technical methodology; data curation; investigation; formal analysis; supervision; original draft. MS: Funding and equipment acquisition; social science methodology; project administration; resources; data curation; review and editing.
Funding
The authors declare that this study received funding from Nissan Europe. The funder was not involved in the study design, collection, analysis, interpretation of data, the writing of this article or the decision to submit it for publication.
Disclaimer
Frontiers Media SA remains neutral with regard to jurisdictional claims in published maps and institutional affiliations.
Conflict of Interest
The authors declare that the research was conducted in the absence of any commercial or financial relationships that could be construed as a potential conflict of interest.
Acknowledgments
The authors wish to gratefully acknowledge the significant contribution made to the Solar Taxi project by Nissan Europe who kindly donated a nearly-new e-NV200 minivan in support of the field trial. Similarly, we are grateful to Sharp, who donated 30 ex-laboratory photovoltaic modules to the project and to the Manduar Community Development Hub. Our thanks also go to De Montfort University, who provided funding for minigrid components, such as batteries and an inverter, funded the shipping of the vehicle to The Gambia and paid traveling expenses for researchers to work there. We also extend our gratitude to the staff of Global Hands in the United Kingdom and The Gambia, who manage the Manduar Community Development Hub, and our friends and colleagues in Manduar village. They have given a great deal of in-kind support and, by hosting the Solar Taxi, have been instrumental to making this initiative possible. Finally, we should like to thank the Government of The Gambia, who have supported this research by waiving import taxes and by enabling the Solar Taxi to operate as a research vehicle in The Gambia.
References
Afukaar, F., Damsere-Derry, J., Peters, K., and Starkey, P. (2019). Rural transport services indicators: using a new mixed-methods methodology to inform policy in Ghana. Trans. Res. Interdiscip. Perspect. 3, 100074. doi:10.1016/j.trip.2019.100074
Ampersand (2020). Available at: https://ampersand.solar/ (Accessed April 24, 2020).
Anyanzwa, J. (2019). Uganda and Rwanda lead East Africa in switch to electric cars, the East African. Available at: https://www.theeastafrican.co.ke/meta/2562-2554-36ij67/index.html (Accessed May 12, 2020).
AutoScout24 (2020a). Nissan E-NV200 evalia 24KW comfort inkl.-batterie navi SH. Available at: https://www.autoscout24.com/offers/nissan-e-nv200-evalia-24kw-comfort-inkl-batterie-navi-sh-electric-white-a7c0a8b8-d154-40b1-b991-b6e6da10becd?cldtidx=21 (Accessed April 16, 2020).
AutoScout24 (2020b). Renault ZOE intens charge rapide. Available at: https://www.autoscout24.com/offers/renault-zoe-intens-charge-rapide-electric-white-3faa97c3-74ed-4664-85ac-a7ad7c2b0951?cldtidx=10&cldtsrc=listPage (Accessed April 16, 2020).
AutoScout24 (2020c). Nissan leaf visia 24 kWh (inkl. Batterie). Available at: https://www.autoscout24.com/offers/nissan-leaf-visia-24-kwh-inkl-batterie-electric-white-ddda231c-5593-4dcd-a253-3d301bfdf593?cldtidx=5&cldtsrc=listPage (Accessed April 16, 2020).
BatteryMasters (2016). Ultramax NP33-12C, 12V 33Ah 20hr high capacity Deep cycle sealed lead acid battery. Available at: https://www.batterymasters.co.uk/ultramax-np33-12c-12v-33ah-20hr-as-30ah-32ah-35ah-36ah-high-capacity-deep-cycle-sealed-lead-acid-battery.html (Accessed April 15, 2020).
Battery Megastore (2019a). Victron energy Quattro 48/15000/200-100/100 VE.Bus—QUA483150000. Available at: https://www.batterymegastore.co.uk/product/victron-energy-quattro-48-15000-200-100-100-ve-bus-qua483150000/ (Accessed April 15, 2020).
Battery Megastore (2019b). Victron energy EasySolar 48/5000/70-100 with MPPT 150/100 & color control—PMP485027010. Available at: https://www.batterymegastore.co.uk/product/victron-energy-easysolar-48-5000-70-100-with-mppt-150-100-color-control-pmp485027010/ (Accessed April 15, 2020).
Battery Megastore (2019c). Victron energy MultiPlus 48/3000/35-16 VE.Bus—PMP483020001. Available at: https://www.batterymegastore.co.uk/product/victron-energy-multiplus-48-3000-35-16-ve-bus-pmp483020001/ (Accessed April 15, 2020).
Battery Megastore (2019d). Victron energy SmartSolar MPPT 250/60-Tr—SCC125060221. Available at: https://www.batterymegastore.co.uk/product/victron-energy-smartsolar-mppt-250-60-tr-scc125060221/ (Accessed April 15, 2020).
Baurzhan, S., and Jenkins, G. P. (2016). Off-grid solar PV: is it an affordable or appropriate solution for rural electrification in sub-Saharan African countries? Renew. Sustain. Energy Rev. 60, 1405–1418. doi:10.1016/j.rser.2016.03.016
Bhattacharyya, S. (2014). Rural electrification through decentralised off-grid systems in developing countries. United Kingdom: Springer Science and Business Media, London.
Bhatti, A. R., Salam, Z., Aziz, M., Yee, K., and Ashique, R. H. (2016). Electric vehicles charging using photovoltaic: status and technological review, Renew. Sustain. Energy Rev. 54, 34–47. doi:10.1016/j.rser.2015.09.091
Boait, P., Gammon, R., Advani, V., Wade, N., Greenwood, D., and Davison, P. (2017). ESCoBox: a set of tools for mini-grid sustainability in the developing world. Sustainability 9(5), 738. doi:10.3390/su9050738
Bokopane, L., Kusakana, K., and Vermaak, H. J. (2015). “Optimal energy management of an isolated electric Tuk-Tuk charging station powered by hybrid renewable systems,” in 2015 international conference on the domestic use of energy (DUE), Cape Town, 2015, 193–201.
Buresh, K. M., Apperley, M. D., and Booysen, M. J. (2020). Three shades of green: perspectives on at-work charging of electric vehicles using photovoltaic carports. Energy Sustain. Dev. 57, 132–140. doi:10.1016/j.esd.2020.05.007
CarGambia (2020). Mercedes-benz C-klasse serekunda. Available at: https://www.cargambia.com/en/vehicle_listings/ad-mercedes-benz-c-klasse-kanifing-serekunda-8 (Accessed April 17, 2020).
CCL Components (2019a). Victron energy SmartSolar MPPT charge controller 100V/20A_48V. Available at: https://www.cclcomponents.com/victron-energy-smartsolar-mppt-charge-controller-100v-20a-48v (Accessed April 15, 2020).
CCL Components (2019b). Victron energy 12V 60Ah (C20) GEL deep cycle battery. Available at: https://www.cclcomponents.com/victron-energy-12v-60ah-c20-gel-deep-cycle-battery (Accessed April 15, 2020).
CCL Components (2019c). Victron energy 12V 110Ah (C20) GEL deep cycle battery. Available at: https://www.cclcomponents.com/victron-energy-12v-110ah-c20-gel-deep-cycle-batteryCCL (Accessed April 15, 2020).
Crossland, A., Anuta, O., and Wade, N. (2015). A socio-technical approach to increasing the battery lifetime of off-grid photovoltaic systems applied to a case study in Rwanda. Renew. Energy 83, 30–40. doi:10.1016/j.renene.2015.04.020
Daina, N., Sivakumar, A., and Polak, J. W. (2017). Electric vehicle charging choices: modelling and implications for smart charging services. Transport. Res. C Emerg. Technol. 81, 36–56. doi:10.1016/j.trc.2017.05.006
ESCoBox (2017). Link to download the ESCoBox PV DST. Available at: http://hal.dmu.ac.uk/documents/2017/08/ESCoBox_DST_PV_and_battery_R2.xlsm0 (Accessed March 23, 2020).
Gammon, R., Boait, P., and Advani, V. (2016). Management of demand profiles on minigrids in developing countries using timeslot allocation,” in 2016 IEEE PES Power Africa Conference, Editor U. Onyewuchi (Livingstone, Zambia: IEEE), 41–45.
García, I. I., and Jeffrey, M. (2017). Recharging of electric cars by solar photovoltaics, electric vehicles: prospects and challenges. Edinburgh, United Kingdom: Elsevier, 415–487.
Goldin, E., Erickson, L., Natarajan, B., Brase, G., and Pahwa, A. (2014). Solar powered charge stations for electric vehicles. Environ. Prog. Sustain. Energy 33 (4), 1298–1308.
Gollwitzer, L., Ockwell, D., Muok, B., Ely, A., and Ahlborg, H. (2018). Rethinking the sustainability and institutional governance of electricity access and mini-grids: electricity as a common pool resource. Energy Res. Soc. Sci. 39, 152–161. doi:10.1016/j.erss.2017.10.033
Harper, M. (2013). Review of strategies and technologies for demand-side management on isolated mini-grids. Berkeley, CA: Lawrence Berkeley National Laboratory (LBNL), Vol. 3, 1–26.
International Energy Agency (2020). Global EV outlook 2020, IEA, Paris. Available at: https://www.iea.org/reports/global-ev-outlook-2020 (Accessed September 06, 2020).
Jiao, N., and Evans, S. (2016). Business models for sustainability: the case of second-life electric vehicle batteries. Proc. CIRP. 40, 250–255. doi:10.1016/j.procir.2016.01.114
Kavaki (2020). Chinese three wheel cargo petrol tricycle 200cc water cooled 3 wheel motorcycle/scooter tricycle for sales. Available at: https://www.alibaba.com/product-detail/Chinese-three-wheel-cargo-petrol-tricycle_60790634878.html (Accessed April 17, 2020).
Kuang, Y., Zhang, Y., Li, B. Z. K., Cao, Y., Li, L., and Zeng, L. (2016). A review of renewable energy utilization in islands, Renew. Sustain. Energy Rev. 59, 504–513. doi:10.1016/j.rser.2016.01.014
Kuhudzai, R. (2019). All electric taxi-hailing service nopia looks to scale up in Kenya, CleanTechnica, 22/11/2019. Available at: https://cleantechnica.com/2019/11/22/all-electric-taxi-hailing-service-nopia-looks-to-scale-up-in-kenya/ (Accessed May 16, 2020).
Kumar, M. S., and Revankar, S. T. (2017). Development scheme and key technology of an electric vehicle: an overview. Renew. Sustain. Energy Rev. 70, 1266–1285. doi:10.1016/j.rser.2016.12.027
Mathiesen, B., Lund, H., Connolly, D., Wenzel, H., Ostergaard, P., Möller, B., et al. (2015). Smart energy systems for coherent 100% renewable energy and transport solutions. Appl. Energy 145, 139–154. doi:10.1016/j.apenergy.2015.01.075
Mayer-Tasch, L., Mukherjee, M., and Reiche, K. (2013). Report No. 156. Productive use of energy—PRODUSE measuring impacts of electrification on small and micro-enterprises.
McCauley, D., and Heffron, R. (2018). Just transition: integrating climate, energy and environmental justice. Energy Pol. 119, 1–7. doi:10.1016/j.enpol.2018.04.014
Mesarić, P., and Krajcar, S. (2015). Home demand side management integrated with electric vehicles and renewable energy sources, Energy Build. 108, 1–9. doi:10.1016/j.enbuild.2015.09.001
Mohanty, P., and Kotak, Y. (2017). Electric vehicles: status and roadmap for India, electric vehicles: prospects and challenges. Edinburgh, United Kingdom: Elsevier, 387–414.
Moner-Girona, M., Solano-Peralta, M., Lazopoulou, M., Ackom, E. K., Vallve, X., and Szabó, S. (2018). Electrification of sub-Saharan Africa through PV/hybrid mini-grids: reducing the gap between current business models and on-site experience. Renew. Sustain. Energy Rev. 91, 1148–1161. doi:10.1016/j.rser.2018.04.018
Mueller, O. M., and Mueller, E. K. (2014). “Off-grid, low-cost, electrical sun-car system for developing countries,” in IEEE global humanitarian technology conference (GHTC 2014), San Jose, CA, 2014, 14–17.
Numbeo (2020). Gas prices in Brikama, Gambia. Numbeo website. Available at: https://www.numbeo.com/gas-prices/in/Brikama-Gambia (Accessed March 27, 2020).
Opibus (2017). Opibus website. Available at: https://www.opibus.se/ (Accessed February 18, 2020).
Peters, A. (2020). This electric motorcycle startup is transforming the Rwandan taxi industry, Fast Company, 06/02/2020. Available at: https://www.fastcompany.com/90460273/this-electric-motorcycle-startup-is-transforming-the-rwandan-taxi-industry (Accessed May 16, 2020).
Peters, J., Vance, C., and Harsdorff, M. (2011). Grid extension in rural Benin: micro-manufacturers and the electrification trap. World Develop. 39 (5), 773–783. doi:10.1016/j.worlddev.2010.09.015
Poullikkas, A. (2015). Sustainable options for electric vehicle technologies, renewable and sustainable energy reviews. Limassol, Cyprus: Elsevier.
Pueyo, A., and Hanna, R. (2015). Practical Action Consulting Report. What level of electricity access is required to enable and sustain poverty reduction? Annex 1—literature review.
Richardson, D. B. (2013). Electric vehicles and the electric grid: a review of modeling approaches, impacts, and renewable energy integration. Renew. Sustain. Energy Rev. 19, 247–254. doi:10.1016/j.rser.2012.11.042
Robert, F., Sisodia, G., and Gopalan, S. (2018). “The critical role of anchor customers in rural microgrids: impact of load factor on energy cost,” in 6th international conference on computation of power, energy, information and communication, ICCPEIC 2017, Melmaruvathur, India, January 2018, 398–403.
Sakiliba, K., Sani Hassan, A., Wu, J., Saja Sanneh, E. A., and Ademi, S. (2015). Assessment of stand-alone residential solar photovoltaic application in sub-Saharan Africa: a case study of Gambia. J. Renew. Energy 2015, 1–10. doi:10.1155/2015/640327
Sandwell, P., Ekins-Daukes, N., and Nelson, J. (2017). What are the greatest opportunities for PV to contribute to rural development? Energy Proc. 130, 139–146. doi:10.1016/j.egypro.2017.09.416
Secka, M. (2019). Hand to mouth: why taxi driving in the Gambia is no picnic, the Chronicle Gambia, 7th May 2019. Available at: www.chronicle.gm/hand-to-mouth-why-taxi-driving-in-the-gambia-is-no-picnic/ (Accessed May 10, 2020).
Siristar (2020). 6 seater tuk tuk rickshaw electric used adult tricycle for sale. Available at: https://siristar.en.alibaba.com/product/60741870519-806232145/6_seater_tuk_tuk_rickshaw_electric_used_adult_tricycle_for_sale.html (Accessed April 16, 2020).
Starkey, P., Ellis, S., Hine, J., and Ternell, A. (2002). Improving rural mobility: options for developing motorized and nonmotorized transport in rural areas. World Bank Technical Paper, 1–69.
Touboulic, A., and Ejodame, E. (2016). Are we really doing the “right thing”? From sustainability imperialism in global supply chains to an inclusive emerging economy perspective. J. Innov. Impact. 8, 630. doi:10.9774/gleaf.9781783533527_3
United Nations (2015). Transforming our world: the 2030 agenda for sustainable development, A/RES/70/1, 4th plenary meeting 25 September 2015. Available at: https://sustainabledevelopment.un.org/content/documents/21252030%20Agenda%20for%20Sustainable%20Development%20web.pdf.
Urban eBikes (2020). Super soco TC 1500W—45mph. Available at: https://urbanebikes.com/collections/electric-motorbikes/products/super-soco-tc (Accessed April 16, 2020).
Used Bike UK (2020). Yamaha Sr125 for sale in gloucester. Available at: https://www.usedbikesuk.co.uk/bikes-for-sale/yamaha/sr125/44990.htm (Accessed April 17, 2020).
uYilo (2020). Website of uYilo eMobility technology innovation programme. Available at: http://uyilo.org.za/ (Accessed May 10, 2020).
V2G Hub (2020). Available at: https://www.v2g-hub.com/ (Accessed May 12, 2020).
Wang, Y., Sperling, D., and Tal, G., and Fang, H. (2017). China’s electric car surge. Energy Pol. 102, 486–490. doi:10.1016/j.enpol.2016.12.034
Yinjian (2020). 6 passenger gasoline tricycle taxi for Africa, southeast Asia tuk Tuk, Motorcycle, gasoline motorized tricycle. Available at: https://www.alibaba.com/product-detail/6-Passenger-Gasoline-Tricycle-Taxi-for_60540341165.html?spm=a2700.7724857.normalList.103.31047ab4aPY9qh (Accessed April 17, 2020).
Zhang, Y., and Gatsis, N., and Giannakis, G. B. (2013). Robust energy management for microgrids with high-penetration renewables. IEEE Trans. Sustain. Energy 4 (4), 944–953. doi:10.1109/TSTE.2013.2255135
Zhengzhou Known Imp (2020). Promotion sales adult electric cargo tricycle motorcycle three wheel bicycle for adults/mobility scooter dumper. Available at: https://www.aliexpress.com/item/4000149103258.html (Accessed April 16, 2020).
Keywords: low-carbon transport, international development, minigrids, solar energy, electric vehicles, smart recharging, sub-Saharan Africa, developing world
Citation: Gammon R and Sallah M (2021) Preliminary Findings From a Pilot Study of Electric Vehicle Recharging From a Stand-Alone Solar Minigrid. Front. Energy Res. 8:563498. doi: 10.3389/fenrg.2020.563498
Received: 19 May 2020; Accepted: 02 December 2020;
Published: 25 January 2021.
Edited by:
Goran Krajacic, University of Zagreb, CroatiaCopyright © 2021 Gammon and Sallah. This is an open-access article distributed under the terms of the Creative Commons Attribution License (CC BY). The use, distribution or reproduction in other forums is permitted, provided the original author(s) and the copyright owner(s) are credited and that the original publication in this journal is cited, in accordance with accepted academic practice. No use, distribution or reproduction is permitted which does not comply with these terms.
*Correspondence: Rupert Gammon, cmdhbW1vbkBkbXUuYWMudWs=