- 1NUS Environmental Research Institute, National University of Singapore, Singapore, Singapore
- 2Department of Chemical and Biomolecular Engineering, National University of Singapore, Singapore, Singapore
In the context of accelerating development of renewable and sustainable energy, biogas upgrading has attracted significant attention in order to yield high quality biomethane which can be utilized as an alternative to natural gas. Biogas upgrading techniques have been revolutionized in the past decade with the emergence of new strategies such as enhanced hydrogenotrophic methanogenesis. This review comprehensively summarizes the latest in situ biogas upgrading technologies via enhanced hydrogenotrophic methanogenesis in order to facilitate their development and commercial application: (1) hydrogen injection into anaerobic digesters for in situ enhancement of hydrogenotrophic methanogenic pathways, (2) membrane electrolysis-assisted CO2 extraction and bioelectrochemical removal of CO2, and (3) additives (e.g., ash, zero-valent iron, and biochar). The key findings and technical limitations are summarized and critically analyzed for each in situ biogas upgrading technique. Additionally, potential industrial applications of these in situ biogas upgrading strategies in anaerobic digesters are also discussed, before concluding with their future development.
Introduction
Anaerobic digestion of biodegradable organic waste has become a critical technology in order to simultaneously achieve sustainable waste management and bioenergy production, such as methane-rich biogas (Murphy and Thamsiriroj, 2013; Kougias and Angelidaki, 2018). Nevertheless, the use of methane-rich biogas in natural gas grids is hindered by the presence of impurities including CO2, H2S, and other trace gases (Angelidaki et al., 2019). Hence, biogas upgrading technologies have gained increased interest in recent years in order to achieve higher biomethane grade.
In this context, many biogas upgrading strategies have been developed, such as hydrogen injection in anaerobic digesters for the in situ enhancement of hydrogenotrophic methanogenisis (Luo and Angelidaki, 2012; Luo et al., 2012), CO2 removal via various adsorbents (Mafra et al., 2018; Paolini et al., 2019) and water scrubbing (Noorain et al., 2019), CO2 separation using membranes (Belaissaoui and Favre, 2018; Peppers et al., 2019), or via cryogenic separation techniques (Yousef et al., 2018, 2019). Among these biogas upgrading technologies, the in situ enhancement of hydrogenotrophic methanogenic pathways has particularly attracted attention due to the advantage of converting CO2 into CH4 in situ (Sarker et al., 2018; Zabranska and Pokorna, 2018) via hydrogen injection or microbial electrolysis cells. In the last decade, many breakthroughs were achieved in the field using hydrogen mass transfer in bioreactors (Jensen et al., 2018; Alfaro et al., 2019), membrane electrolysis-assisted CO2 extraction (Verbeeck et al., 2019), bioelectrochemical removal of CO2 (Xu et al., 2014), pH buffer system (Yu D. et al., 2018), modeling and simulations (Lovato et al., 2017), and additives such as ash (Yin et al., 2019), or zero-valent iron (Dykstra and Pavlostathis, 2017). However, much of the related studies are diverse and not consolidated, let alone identify the state-of-the-art.
Therefore, the objective of this review is to offer a comprehensive and up-to-date summary of in situ biogas upgrading technologies via enhanced hydrogenotrophic methanogenesis for high quality biogas production from anaerobic digesters. In addition to summarizing the current state of research in various in situ biogas upgrading technologies, some recommendations for future potential research are also put forth for consideration. The mini review with a special focus on in situ biogas upgrading techniques via enhanced hydrogenotrophic methanogenesis could be an updated supplement to the previous reviews.
Hydrogen Injection for Enhanced Hydrogenotrophic Methanogenesis
Hydrogen injection is one of the most widely studied techniques for in situ biogas upgrading via enhanced hydrogenotrophic methanogenesis (Angelidaki et al., 2018; Sarker et al., 2018). To guarantee the sustainability of the process, the hydrogen injected in the digesters was derived from periodic excess of electrical energy generated from solar energy and/or wind energy (Luo et al., 2012). Figure 1 shows the theoretical basis of enhanced hydrogenotrophic methanogenesis for biogas upgrading.
When anaerobic digestion takes place, complex organic wastes undergo a series of biochemical processes (i.e., hydrolysis, acidogenesis, and acetogenesis) during which they are converted into acetate, H2 and CO2, as shown in Figure 1. During the subsequent hydrogenotrophic methanogenesis process, 4 moles of H2 and 1 mole of CO2 can be converted into 1 mole of CH4. Hydrogenotrophic methanogenesis has been extensively investigated using novel hydrogen injection approaches for elevated mass transfer (Agneessens et al., 2017; Bassani et al., 2017; Jensen et al., 2018) or related microbial communities in hydrogen assisted biogas upgrading process (Corbellini et al., 2018; Wang et al., 2020). One of the major challenges with hydrogen assisted biogas upgrading was the increase of pH value to a relatively high level (e.g. > 8.5) due to bicarbonate consumption, leading to inhibition of methanogenesis (Luo et al., 2012; Angelidaki et al., 2018). In order to mitigate this technical challenge, the anaerobic system with automatically controlled pH-value has been developed (Ravi et al., 2018), which could be integrated into the hydrogen assisted biogas upgrading systems. Another key issue that has to be noted during the in situ biogas upgrading process was the relatively low gas-to-liquid mass transfer rate that limited the availability of hydrogen for methanogens (Bassani et al., 2016; Maegaard et al., 2019). Therefore, an appropriate mass transfer of hydrogen in anaerobic digesters played a significant role in enhancing hydrogenotrophic methanogenesis. Indeed, a relatively low hydrogen concentration was thermodynamically favorable for hydrogenotrophic methanogenesis (Voelklein et al., 2019), whereas elevated hydrogen levels could temporarily inhibit the anaerobic digestion process by promoting the accumulation of acetate (Agneessens et al., 2017).
In recent years, several new materials (e.g., alumina ceramic sponge), units and reactor configurations were examined in order to enhance the hydrogen mass transfer to the liquid phase. For instance, Alfaro et al. (2019) found that the hydrogen gas-liquid mass transfer was significantly increased through the application of biogas recirculation using a submerged membrane for gas sparging. Similarly, Bassani et al. (2016) reported an innovative setup that consisted of a granular reactor and a separate hydrogen injection chamber. They observed that the maximum methane content in biogas was upgraded to 82% by distributing hydrogen through a metallic diffuser followed by ceramic sponge in a separate chamber (Bassani et al., 2016). Therefore, hydrogen injection could be a promising approach for the intensification of biomethane production in pilot- and full-scale anaerobic digestion plants.
With the development of high-throughput pyrosequencing technology, an increasing number of studies has been investigating the microbial communities involved in hydrogenotrophic methanogenesis, and more specifically, for systems under hydrogen supplementation (Corbellini et al., 2018). Hydrogen injection was reported to selectively enrich reactors in hydrogenotrophic methanogens and syntrophic bacteria (Xu et al., 2020). However, the methanogenic communities in the hydrogen assisted biogas upgrading systems was shown to be affected by several factors including hydrogen concentration (Agneessens et al., 2017), operation temperature (Zhu et al., 2019b), process inhibitors such as ammonia (Wang et al., 2020), and volatile fatty acids (Mulat et al., 2017; Rachbauer et al., 2017), organic loading rate, etc. Supplementing external hydrogen had a strong selective pressure on methanogenic communities due to the critical role of hydrogen concentration on the equilibrium of biological reactions (see Figure 1). Indeed, methanogenic species such as genera Methanoculleus, Methanothermobacter, and Methanoculleus were dominant in hydrogen-supplemented systems (Bassani et al., 2015; Kougias et al., 2017). After hydrogen addition, the produced biogas was upgraded to average methane concentration of 89% in the mesophilic system and 85% in the thermophilic system (Bassani et al., 2015). With hydrogen supplementation at a hydrogen injection rate of 0.66 ml/(min·Vreactor) and an H2:CO2 ratio of 4:1, thermophilic digesters were found to be enriched in some hydrogenotrophic methanogens such as Methanobrevibacter and Methanobacterium (Zhu et al., 2019a), whilst Methanoculleus sp. dominated in mesophilic reactors with a methane content of 95% increased from 50% (Maegaard et al., 2019). Additionally, elevated ammonia concentration (e.g., 1–7 g/L ) induced a shift of pathway, from aceticlastic to hydrogenotrophic methanogenesis, in hydrogen-assisted biogas production (Wang et al., 2020). The enrichment in methanogenic species for hydrogen assisted biogas production systems can help optimizing microbial productivity as well as the performance of AD processes using a bioaugmentation strategy with pure cultures. Moreover, the economic feasibility of hydrogen-assisted methods in various biogas upgrading scenarios should be analyzed in future studies.
Microbial Electrochemical and Membrane Electrolysis-Assisted In situ Biogas Upgrading
In addition to direct injection of hydrogen in anaerobic digesters, microbial and membrane electrolysis cells could be integrated to digesters in order to generate hydrogen via electrolysis of water, hence facilitating hydrogenotrophic methanogenesis for in situ biogas upgrading (Jin et al., 2017; Yu Z. et al., 2018). Figure 2 shows the schematic reactor setup for microbial electrochemical and membrane electrolysis-assisted in situ biogas upgrading. Xu et al. (2014) reported that after CO2 removal using a bioelectrochemical method, hydrogenotrophic methanogenesis and alkali production were the two major contributors to in situ biogas upgrading. Furthermore, species involved in direct interspecies electron transfer, such as methanogenic genus Methanobacterium, were dominant on all biocathodes, thus playing a significant role in enhancing hydrogenotrophic methanogenesis. Membrane electrolysis could simultaneously remove CO2 and produce hydrogen, hence was reported as an innovative treatment method for biogas upgrading (Verbeeck et al., 2019). An ideal 4:1 H2: CO2 ratio in the off gas could be obtained with system optimization and could be further upgraded through chemoautotrophic microbial conversion of CO2-CH4. Electrochemical biogas upgrading was shown to be a promising approach as it can yield a biomethane purity of 98.9% in addition to making better use of excess renewable energy. Furthermore, the bio-electrochemically assisted in situ biomethanation technology can also be integrated to wastewater treatment or resource recovery processes (Kokkoli et al., 2018; Fu et al., 2020). In a study conducted by Yanuka-Golub et al. (2019), hydrogen bioavailability was increased using an electrode-assisted anaerobic digestion process, which led to the enrichment of hydrogenotrophic methanogens (e.g., Methanomicrobiales). Another example of electrolysis-assisted in situ biogas upgrading was the conversion of CO2 into methane using a bioelectrochemical system integrated to a wastewater treatment process (Batlle-Vilanova et al., 2019). This technology enhanced biomethane production by 17.4% and produced chlorine compounds in sufficient quantity to disinfect the wastewater from treatment plants. More recently, a novel three-chamber microbial electrolysis cell was developed and utilized for nutrient recovery and biogas upgrading by Zeppilli et al. (2019). This example demonstrated the potential of bioelectrochemical systems which can combine in situ biogas upgrading with nutrient recovery and wastewater treatment. Nonetheless, most of these electrolysis-assisted in situ biogas upgrading technologies are in their early stages of industrial development; more pilot-scale studies should be conducted to optimize system stability and practicability. In addition, the microbial electrochemical methods for in situ biogas upgrading are generally expensive, thereby their economic feasibility should be evaluated together with the corresponding methane yields in the future studies.
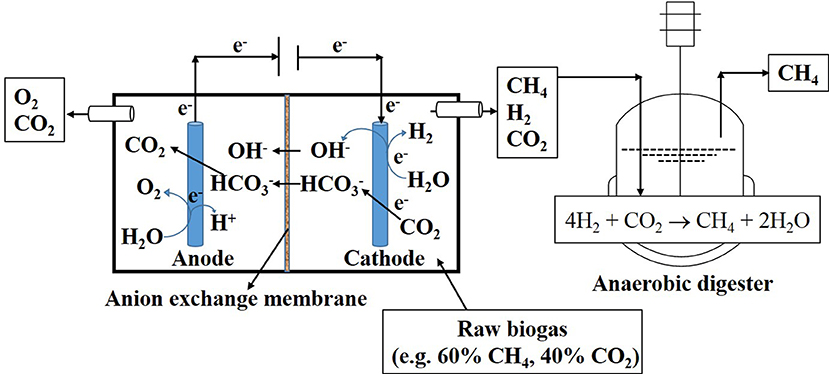
Figure 2. Schematic reactor setup for microbial electrochemical and membrane electrolysis-assisted in situ biogas upgrading. Modified based on Verbeeck et al. (2019) with permission/license granted by the publisher.
Additives for Enhanced Hydrogenotrophic Methanogenesis
Anaerobic digestion of organic wastes relies on a series of reactions catalyzed by enzymes from complex microbial communities (Zhang et al., 2019a), for which trace metals act as essential co-factors. Additionally, the use of external additives can help enrich digesters in functional methanogenic species (Arif et al., 2018). Based on this premise, some researchers proposed the supplementation of digesters with additives such as zero-valent iron, ash and biochar to enhance hydrogenotrophic methanogenesis. For instance, Dykstra and Pavlostathis (2017) explored the influence of zero valent iron (ZVI) on a methanogenic bioelectrochemical system, and reported a 123–231% increase in total methane production compared to the control system without ZVI amendment. The enhancement of hydrogenotrophic methanogenesis could be directly correlated to the enrichement in hydrogenotrophic methanogens such as Methanobrevibacter arboriphilus. Even though ZVI supplementation is mainly associated with positive effects, it is a relatively costly enhancement technique. Furthermore, the post-treatment of ZVI-containing digestate can be a critical issue if large quantity of heavy metals are present in the digesters (Zhang et al., 2019b). Therefore, more environmental-friendly and cheaper alternatives have also been explored. For instance, it has been reported that addition of ash in anaerobic digesters treating wasted activated sludge increased methane content to 79.4%, due to the increased abundance of Methanomassiliicoccus and improved CO2 capture (Yin et al., 2019). Indeed, hydrogen was confirmed as a necessary substrate for methanogenesis process of Methanomassiliicoccus (Dridi et al., 2012). In the ash amended digester, CO2 sequestration as calcite sediment was facilitated, leading to a relatively high hydrogen percentage in the biogas (Yin et al., 2019). Therefore, the enriched Methanomassiliicoccus converted more hydrogen into methane via enhanced hydrogenotrophic methanogenesis. Recently, doping granular activated carbon particles with magnetite was an efficient method to attain high-performance anaerobic digestion (Barua et al., 2019). Further cost reduction was achieved by using biochar as an additive for anaerobic digestion. Shen et al. (2015) reported that pipeline-quality biomethane (e.g., CH4 > 90%) was obtained from the anaerobic digestion of sludge amended with corn stover biochar. Biochar supplementation provided substantial micro- and macro-nutrients (e.g., K, Ca, P, Mg, and Fe) to anaerobic microbial communities, hence facilitating the growth of functional bacteria (e.g., Clostridia) and methanogens (e.g., Methanothermobacter). It has been validated that biochar amendment altered the abundance of functional methanogenic species by enhancing the selective enrichment of different species (Zhang et al., 2020a). Therefore, in the biochar amended anaerobic digesters, the enriched hydrogenotrophic methanogenic species facilitated the hydrogenotrophic methanogenesis process, leading to a higher methane yield (Zhang et al., 2020b). For instance, the selective colonization of Methanosarcina contributed to the in situ biogas upgrading of biochar-amended digesters with a maximum methane content of 95% (Shen et al., 2017). More recently, it was confirmed that biochar acted as a good carrier for immobilized hydrogenotrophic methanogens in anaerobic digesters, thus accelerating the bioconversion of CO2 to methane and increasing the overall rate of methanogenesis (Yang et al., 2020). However, due to the environmental challenges associated with the use of ZVI, ash or biochar in anaerobic digesters, more cost-effective and environmentally-friendly additives should be developed to enhance methanogenesis whilst keeping the treatment cost of the digestate to a minimum.
Potential Industrial Applications of In situ Biogas Upgrading
Although the aforementioned in situ biogas upgrading techniques (i.e., hydrogen injection, bioelectrochemical technique, and additive addition) have been widely researched in the past decade, most of the studies were based on lab-scale anaerobic digesters, hence pilot-, and full-scale test-bedding would be worth exploring. Although it is challenging to precisely estimate the number of existing biogas plants which adopted an in situ biogas upgrading strategy, it is believed to be growing steadily due to the objectives set by the agreement of the Paris Climate Summit in 2015 in terms of greenhouse gas emissions. Hydrogen injection is the most widely industrialized in situ biogas upgrading technique among the three processes reviewed here. For instance, Jensen et al. (2018) investigated the hydrogen mass transfer potential of a venturi-type injector for biogas upgrading of a large-scale (1,200 m3) anaerobic digester. The experimental results demonstrated that hydrogen consumption rates increased with hydrogen injection rates. Moreover, the injection of large bubbles was shown to limit the gas-liquid mass transfer rate and could lead to incomplete hydrogen consumption. Thus, optimizing gas flow rates for full-scale in situ biogas upgrading systems should facilitate the injection of smaller gas bubbles and improve the hydrogen transfer efficiency of the system.
In comparison to hydrogen injection, bioelectrochemical technique and additive supplementation are two new strategies, but their examination in existing pilot-scale digesters are extremely limited. The use of additives in existing biogas plants treating solid organic wastes would increase the hydrolysis rate and the biogas methane content of the anaerobic digestion process without requiring major modification to the existing infrastructure. In the short term, the in situ biogas upgrading technique with the best investment potential might be the hydrogen injection technology, but the development of an injection equipment providing high mass transfer efficiency would be necessary. The supplementation of additives should be considered as a medium-term investment due to its potential to promote microbial growth and increase process efficiency at a relatively low cost. Nevertheless, the environmental and economic costs of additives (e.g., biochar, zero valent iron, etc.) need to be evaluated before it can be applied to existing commercial-scale biogas plants. Although engineered additives are still under investigation, they hold great potentials in revolutionizing the upgraded biogas industry (Linville et al., 2017; Pan et al., 2019). On the longer term, integrated in situ biogas upgrading technology derived from aforementioned techniques might be attractive to investors, as the integrated approaches present the benefits of multiple technologies. Generally, more efforts should be focused on the scale-up of the aforementioned in situ biogas upgrading techniques before they are efficient enough to be used in industrial installations.
Conclusions and Recommendations for Future Work
In situ biogas upgrading techniques via enhanced hydrogenotrophic methanogenesis, including hydrogen injection, bioelectrical methods, and supplementation of additives, are rapidly developing worldwide in order to provide new strategies for clean and sustainable biogas production. In this mini-review, we summarized the latest research progress for in situ biogas upgrading via enhanced hydrogenotrophic methanogenesis, which would improve the quality of biogas. Nonetheless, several limitations need to be overcome before the aforementioned in situ biogas upgrading approaches could be applied in industrial anaerobic digestion plants for biomethane production.
Among the three in situ biogas upgrading techniques reviewed in this article, hydrogen injection is the most mature method. However, the hydrogen used in this approach was generally obtained from water electrolysis using the excess electricity of wind energy. As excess electricity is not widely available, this approach would not be economically suitable for all regions of the world. Therefore, economic feasibility should be evaluated together with excess power availability when considering the use of hydrogen assisted in situ biogas upgrading. Although a number of novel hydrogen injection approaches have been shown to increase gas-to-liquid mass transfer rate at the lab scale, more research to enhance the availability of hydrogen to methanogens in pilot- and commercial-scale digesters are required. Furthermore, the parameters (e.g., hydrogen concentration, temperature, feedstock, etc.) which contribute to the change in microbial communities in hydrogen-assisted hydrogenotrophic methanogenesis should be further investigated.
Similarly, various novel fermentation systems have been developed at the lab scale for electrolysis-assisted in situ biogas upgrading technologies, but more pilot-scale studies should be conducted in the future to optimize system stability and practicability. Finally, the supplementation of digesters with engineered additives holds great potentials. However, the environmental and economic costs of additives should be evaluated prior to application in existing biogas plants. In parallel, advanced reactor systems combining different in situ biogas upgrading techniques for enhanced hydrogenotrophic methanogenesis can be developed. Moreover, net energy gain should also be taken into consideration when evaluating the technical feasibility.
The modeling and simulations of in situ enhanced hydrogenotrophic methanogenesis, which have been explored by a pioneering study from Lovato et al. (2017), should be further investigated. Indeed, reliable mathematical models can reduce experimental cost and risk, predict system performance, optimize operational parameters, and help analyze essential methanogenic pathways.
Author Contributions
LZ and AK wrote the manuscript. YT performed supervision. All authors reviewed the manuscript.
Funding
This research project was funded by the National Research Foundation, Prime Minister's Office, Singapore under its Campus for Research Excellence and Technological Enterprise (CREATE) Programme. The open access publication fee for this manuscript came from LZ.
Conflict of Interest
The authors declare that the research was conducted in the absence of any commercial or financial relationships that could be construed as a potential conflict of interest.
Acknowledgments
This research project was funded by the National Research Foundation, Prime Minister's Office, Singapore under its Campus for Research Excellence and Technological Enterprise (CREATE) Programme.
References
Agneessens, L. M., Ottosen, L. D. M., Voigt, N. V., Nielsen, J. L., de Jonge, N., Fischer, C. H., et al. (2017). In-situ biogas upgrading with pulse H2 additions: the relevance of methanogen adaption and inorganic carbon level. Bioresour. Technol. 233, 256–263. doi: 10.1016/j.biortech.2017.02.016
Alfaro, N., Fdz-Polanco, M., Fdz-Polanco, F., and Díaz, I. (2019). H2 addition through a submerged membrane for in-situ biogas upgrading in the anaerobic digestion of sewage sludge. Bioresour. Technol. 280, 1–8. doi: 10.1016/j.biortech.2019.01.135
Angelidaki, I., Treu, L., Tsapekos, P., Luo, G., Campanaro, S., Wenzel, H., et al. (2018). Biogas upgrading and utilization: current status and perspectives. Biotechnol. Adv. 36, 452–466. doi: 10.1016/j.biotechadv.2018.01.011
Angelidaki, I., Xie, L., Luo, G., Zhang, Y., Oechsner, H., Lemmer, A., et al. (2019). “Biogas Upgrading: Current and Emerging Technologies.” in Biofuels: Alternative Feedstocks and Conversion Processes for the Production of Liquid and Gaseous Biofuels, eds A. Pandey, C. Larroche, E. Gnansounou, S. K. Khanal, C. G. Dussap, and S. Ricke (London: Elsevier), 817–843. doi: 10.1016/B978-0-12-816856-1.00033-6
Arif, S., Liaquat, R., and Adil, M. (2018). Applications of materials as additives in anaerobic digestion technology. Renew. Sust. Energ. Rev. 97, 354–366. doi: 10.1016/j.rser.2018.08.039
Barua, S., Zakaria, B. S., Lin, L., and Dhar, B. R. (2019). Magnetite doped granular activated carbon as an additive for high-performance anaerobic digestion. Mater. Sci. Energ. Technol. 2, 377–384. doi: 10.1016/j.mset.2019.04.002
Bassani, I., Kougias, P. G., and Angelidaki, I. (2016). In-situ biogas upgrading in thermophilic granular UASB reactor: key factors affecting the hydrogen mass transfer rate. Bioresour. Technol. 221, 485–491. doi: 10.1016/j.biortech.2016.09.083
Bassani, I., Kougias, P. G., Treu, L., and Angelidaki, I. (2015). Biogas upgrading via hydrogenotrophic methanogenesis in two-stage continuous stirred tank reactors at mesophilic and thermophilic conditions. Environ. Sci. Technol. 49, 12585–12593. doi: 10.1021/acs.est.5b03451
Bassani, I., Kougias, P. G., Treu, L., Porté, H., Campanaro, S., and Angelidaki, I. (2017). Optimization of hydrogen dispersion in thermophilic up-flow reactors for ex situ biogas upgrading. Bioresour. Technol. 234, 310–319. doi: 10.1016/j.biortech.2017.03.055
Batlle-Vilanova, P., Rovira-Alsina, L., Puig, S., Balaguer, M. D., Icaran, P., Monsalvo, V. M., et al. (2019). Biogas upgrading, CO2 valorisation and economic revaluation of bioelectrochemical systems through anodic chlorine production in the framework of wastewater treatment plants. Sci. Total Environ. 690, 352–360. doi: 10.1016/j.scitotenv.2019.06.361
Belaissaoui, B., and Favre, E. (2018). Novel dense skin hollow fiber membrane contactor based process for CO2 removal from raw biogas using water as absorbent. Sep. Purif. Technol. 193, 112–126. doi: 10.1016/j.seppur.2017.10.060
Corbellini, V., Kougias, P. G., Treu, L., Bassani, I., Malpei, F., and Angelidaki, I. (2018). Hybrid biogas upgrading in a two-stage thermophilic reactor. Energy Convers. Manag. 168, 1–10. doi: 10.1016/j.enconman.2018.04.074
Dridi, B., Fardeau, M. L., Ollivier, B., Raoult, D., and Drancourt, M. (2012). Methanomassiliicoccus luminyensis gen. nov., sp. nov., a methanogenic archaeon isolated from human faeces. Int. J. Syst. Evol. Microbiol. 62, 1902–1907. doi: 10.1099/ijs.0.033712-0
Dykstra, C. M., and Pavlostathis, S. G. (2017). Zero-valent iron enhances biocathodic carbon dioxide reduction to methane. Environ. Sci. Technol. 51, 12956–12964. doi: 10.1021/acs.est.7b02777
Fu, X.-Z., Li, J., Pan, X.-R., Huang, L., Li, C.-X., Cui, S., et al. (2020). A single microbial electrochemical system for CO2 reduction and simultaneous biogas purification, upgrading and sulfur recovery. Bioresour. Technol. 297:122448. doi: 10.1016/j.biortech.2019.122448
Jensen, M. B., Kofoed, M. V. W., Fischer, K., Voigt, N. V., Agneessens, L. M., Batstone, D. J., et al. (2018). Venturi-type injection system as a potential H2 mass transfer technology for full-scale in situ biomethanation. Appl. Energy 222, 840–846. doi: 10.1016/j.apenergy.2018.04.034
Jin, X., Zhang, Y., Li, X., Zhao, N., and Angelidaki, I. (2017). Microbial electrolytic capture, separation and regeneration of CO2 for biogas upgrading. Environ. Sci. Technol. 51, 9371–9378. doi: 10.1021/acs.est.7b01574
Kokkoli, A., Zhang, Y., and Angelidaki, I. (2018). Microbial electrochemical separation of CO2 for biogas upgrading. Bioresour. Technol. 247, 380–386. doi: 10.1016/j.biortech.2017.09.097
Kougias, P. G., and Angelidaki, I. (2018). Biogas and its opportunities-A review. Front. Env. Sci. Eng. 12, 1–12. doi: 10.1007/s11783-018-1037-8
Kougias, P. G., Treu, L., Benavente, D. P., Boe, K., Campanaro, S., and Angelidaki, I. (2017). Ex-situ biogas upgrading and enhancement in different reactor systems. Bioresour. Technol. 225, 429–437. doi: 10.1016/j.biortech.2016.11.124
Linville, J. L., Shen, Y., Ignacio-de Leon, P. A., Schoene, R. P., and Urgun-Demirtas, M. (2017). In-situ biogas upgrading during anaerobic digestion of food waste amended with walnut shell biochar at bench scale. Waste Manag. Res. 35, 669–679. doi: 10.1177/0734242X17704716
Lovato, G., Alvarado-Morales, M., Kovalovszki, A., Peprah, M., Kougias, P. G., Rodrigues, J. A. D., et al. (2017). In-situ biogas upgrading process: modeling and simulations aspects. Bioresour. Technol. 245, 332–341. doi: 10.1016/j.biortech.2017.08.181
Luo, G., and Angelidaki, I. (2012). Integrated biogas upgrading and hydrogen utilization in an anaerobic reactor containing enriched hydrogenotrophic methanogenic culture. Biotechnol. Bioeng. 109, 2729–2736. doi: 10.1002/bit.24557
Luo, G., Johansson, S., Boe, K., Xie, L., Zhou, Q., and Angelidaki, I. (2012). Simultaneous hydrogen utilization and in situ biogas upgrading in an anaerobic reactor. Biotechnol. Bioeng. 109, 1088–1094. doi: 10.1002/bit.24360
Maegaard, K., Garcia-Robledo, E., Kofoed, M. V. W., Agneessens, L. M., de Jonge, N., Nielsen, J. L., et al. (2019). Biogas upgrading with hydrogenotrophic methanogenic biofilms. Bioresour. Technol. 287:121422. doi: 10.1016/j.biortech.2019.121422
Mafra, L., Cendak, T., Schneider, S., Wiper, P. V., Pires, J., Gomes, J. R., et al. (2018). Amine functionalized porous silica for CO2/CH4 separation by adsorption: Which amine and why. Chem. Eng. J. 336, 612–621. doi: 10.1016/j.cej.2017.12.061
Mulat, D. G., Mosbæk, F., Ward, A. J., Polag, D., Greule, M., Keppler, F., et al. (2017). Exogenous addition of H2 for an in situ biogas upgrading through biological reduction of carbon dioxide into methane. Waste Manag. 68, 146–156. doi: 10.1016/j.wasman.2017.05.054
Murphy, J. D., and Thamsiriroj, T. (2013). “Fundamental science and engineering of the anaerobic digestion process for biogas production,” in The Biogas Handbook, eds A. Wellinger, J. Murphy, and D. Baxter (Cambridge: Woodhead Publishing), 104–130. doi: 10.1533/9780857097415.1.104
Noorain, R., Kindaichi, T., Ozaki, N., Aoi, Y., and Ohashi, A. (2019). Biogas purification performance of new water scrubber packed with sponge carriers. J. Clean. Prod. 214, 103–111. doi: 10.1016/j.jclepro.2018.12.209
Pan, J., Ma, J., Zhai, L., Luo, T., Mei, Z., and Liu, H. (2019). Achievements of biochar application for enhanced anaerobic digestion: a review. Bioresour. Technol. 292:122058. doi: 10.1016/j.biortech.2019.122058
Paolini, V., Torre, M., Giacopini, W., Pastori, M., Segreto, M., Tomassetti, L., et al. (2019). CO2/CH4 separation by hot potassium carbonate absorption for biogas upgrading. Int. J. Greenh. Gas Con. 83, 186–194. doi: 10.1016/j.ijggc.2019.02.011
Peppers, J., Li, Y., Xue, J., Chen, X., Alaimo, C., Wong, L., et al. (2019). Performance analysis of membrane separation for upgrading biogas to biomethane at small scale production sites. Biomass Bioenergy 128:105314. doi: 10.1016/j.biombioe.2019.105314
Rachbauer, L., Beyer, R., Bochmann, G., and Fuchs, W. (2017). Characteristics of adapted hydrogenotrophic community during biomethanation. Sci. Total Environ. 595, 912–919. doi: 10.1016/j.scitotenv.2017.03.074
Ravi, P. P., Lindner, J., Oechsner, H., and Lemmer, A. (2018). Effects of target pH-value on organic acids and methane production in two-stage anaerobic digestion of vegetable waste. Bioresour. Technol. 247, 96–102. doi: 10.1016/j.biortech.2017.09.068
Sarker, S., Lamb, J. J., Hjelme, D. R., and Lien, K. M. (2018). Overview of recent progress towards in-situ biogas upgradation techniques. Fuel 226, 686–697. doi: 10.1016/j.fuel.2018.04.021
Shen, Y., Forrester, S., Koval, J., and Urgun-Demirtas, M. (2017). Yearlong semi-continuous operation of thermophilic two-stage anaerobic digesters amended with biochar for enhanced biomethane production. J. Clean. Prod. 167, 863–874. doi: 10.1016/j.jclepro.2017.05.135
Shen, Y., Linville, J. L., Urgun-Demirtas, M., Schoene, R. P., and Snyder, S. W. (2015). Producing pipeline-quality biomethane via anaerobic digestion of sludge amended with corn stover biochar with in-situ CO2 removal. Appl. Energy 158, 300–309. doi: 10.1016/j.apenergy.2015.08.016
Verbeeck, K., De Vrieze, J., Biesemans, M., and Rabaey, K. (2019). Membrane electrolysis-assisted CO2 and H2S extraction as innovative pretreatment method for biological biogas upgrading. Chem. Eng. J. 361, 1479–1486. doi: 10.1016/j.cej.2018.09.120
Voelklein, M. A., Rusmanis, D., and Murphy, J. D. (2019). Biological methanation: strategies for in-situ and ex-situ upgrading in anaerobic digestion. Appl. Energy 235, 1061–1071. doi: 10.1016/j.apenergy.2018.11.006
Wang, H., Zhu, X., Yan, Q., Zhang, Y., and Angelidaki, I. (2020). Microbial community response to ammonia levels in hydrogen assisted biogas production and upgrading process. Bioresour. Technol. 296:122276. doi: 10.1016/j.biortech.2019.122276
Xu, H., Wang, K., and Holmes, D. E. (2014). Bioelectrochemical removal of carbon dioxide (CO2): an innovative method for biogas upgrading. Bioresour. Technol. 173, 392–398. doi: 10.1016/j.biortech.2014.09.127
Xu, H., Wang, K., Zhang, X., Gong, H., Xia, Y., and Holmes, D. E. (2020). Application of in-situ H2-assisted biogas upgrading in high-rate anaerobic wastewater treatment. Bioresour. Technol. 299:122598. doi: 10.1016/j.biortech.2019.122598
Yang, H.-J., Yang, Z.-M., Xu, X.-H., and Guo, R.-B. (2020). Increasing the methane production rate of hydrogenotrophic methanogens using biochar as a biocarrier. Bioresour. Technol. 302:122829. doi: 10.1016/j.biortech.2020.122829
Yanuka-Golub, K., Baransi-Karkaby, K., Szczupak, A., Reshef, L., Rishpon, J., Shechter, R., et al. (2019). An electrode-assisted anaerobic digestion process for the production of high-quality biogas. Water Sci. Technol. 79, 2145–2155. doi: 10.2166/wst.2019.214
Yin, C., Shen, Y., Yu, Y., Yuan, H., Lou, Z., and Zhu, N. (2019). In-situ biogas upgrading by a stepwise addition of ash additives: methanogen adaption and CO2 sequestration. Bioresour. Technol. 282, 1–8. doi: 10.1016/j.biortech.2019.02.110
Yousef, A. M., El-Maghlany, W. M., Eldrainy, Y. A., and Attia, A. (2018). New approach for biogas purification using cryogenic separation and distillation process for CO2 capture. Energy 156, 328–351. doi: 10.1016/j.energy.2018.05.106
Yousef, A. M., El-Maghlany, W. M., Eldrainy, Y. A., and Attia, A. (2019). Upgrading biogas to biomethane and liquid CO2: a novel cryogenic process. Fuel 251, 611–628. doi: 10.1016/j.fuel.2019.03.127
Yu, D., Meng, X., Liu, J., Dian, L., Sui, Q., Zhang, J., et al. (2018). Formation and characteristics of a ternary pH buffer system for in-situ biogas upgrading in two-phase anaerobic membrane bioreactor treating starch wastewater. Bioresour. Technol. 269, 57–66. doi: 10.1016/j.biortech.2018.08.072
Yu, Z., Leng, X., Zhao, S., Ji, J., Zhou, T., Khan, A., et al. (2018). A review on the applications of microbial electrolysis cells in anaerobic digestion. Bioresour. Technol. 255, 340–348. doi: 10.1016/j.biortech.2018.02.003
Zabranska, J., and Pokorna, D. (2018). Bioconversion of carbon dioxide to methane using hydrogen and hydrogenotrophic methanogens. Biotechnol. Adv. 36, 707–720. doi: 10.1016/j.biotechadv.2017.12.003
Zeppilli, M., Simoni, M., Paiano, P., and Majone, M. (2019). Two-side cathode microbial electrolysis cell for nutrients recovery and biogas upgrading. Chem. Eng. J. 370, 466–476. doi: 10.1016/j.cej.2019.03.119
Zhang, L., Li, F., Kuroki, A., Loh, K.-C., Wang, C.-H., Dai, Y., et al. (2020a). Methane yield enhancement of mesophilic and thermophilic anaerobic co-digestion of algal biomass and food waste using algal biochar: semi-continuous operation and microbial community analysis. Bioresour. Technol. 302:122892. doi: 10.1016/j.biortech.2020.122892
Zhang, L., Lim, E. Y., Loh, K.-C., Ok, Y.-S., Lee, J.-T., Shen, Y., et al. (2020b). Biochar enhanced thermophilic anaerobic digestion of food waste: focusing on biochar particle size, microbial community analysis and pilot-scale application. Energ. Convers. Manage. 209:112654. doi: 10.1016/j.enconman.2020.112654
Zhang, L., Loh, K.-C., Lim, J. W., and Zhang, J. (2019a). Bioinformatics analysis of metagenomics data of biogas-producing microbial communities in anaerobic digesters: a review. Renew. Sust. Energ. Rev. 100, 110–126. doi: 10.1016/j.rser.2018.10.021
Zhang, L., Loh, K.-C., and Zhang, J. (2019b). Enhanced biogas production from anaerobic digestion of solid organic wastes: current status and prospects. Bioresour. Technol. Rep. 5, 280–296. doi: 10.1016/j.biteb.2018.07.005
Zhu, X., Cao, Q., Chen, Y., Sun, X., Liu, X., and Li, D. (2019a). Effects of mixing and sodium formate on thermophilic in-situ biogas upgrading by H2 addition. J. Clean. Prod. 216, 373–381. doi: 10.1016/j.jclepro.2019.01.245
Keywords: anaerobic digestion, biomethane production, hydrogenotrophic methanogen, hydrogen injection, additive supplementation, microbial fuel cell, CO2 removal
Citation: Zhang L, Kuroki A and Tong YW (2020) A Mini-Review on In situ Biogas Upgrading Technologies via Enhanced Hydrogenotrophic Methanogenesis to Improve the Quality of Biogas From Anaerobic Digesters. Front. Energy Res. 8:69. doi: 10.3389/fenrg.2020.00069
Received: 26 February 2020; Accepted: 09 April 2020;
Published: 28 April 2020.
Edited by:
Richen Lin, University College Cork, IrelandReviewed by:
Gang Luo, Fudan University, ChinaGopalakrishnan Kumar, University of Stavanger, Norway
Copyright © 2020 Zhang, Kuroki and Tong. This is an open-access article distributed under the terms of the Creative Commons Attribution License (CC BY). The use, distribution or reproduction in other forums is permitted, provided the original author(s) and the copyright owner(s) are credited and that the original publication in this journal is cited, in accordance with accepted academic practice. No use, distribution or reproduction is permitted which does not comply with these terms.
*Correspondence: Le Zhang, erizle@nus.edu.sg; Yen Wah Tong, chetyw@nus.edu.sg