- 1Department of Chemical Engineering, Cyprus University of Technology, Limassol, Cyprus
- 2Department of Mechanical Engineering and Materials Science and Engineering, Cyprus University of Technology, Limassol, Cyprus
- 3Research Unit for Nanostructured Materials Systems, Cyprus University of Technology, Limassol, Cyprus
A new approach for CO2 utilization (as a sole carbon source) to acetic acid and other VFAs under ambient conditions using zero valent iron and anaerobic granular sludge was examined by methanogens inhibition. Zero Valent Iron when is anaerobically oxidized generates H2 that can be utilized along with CO2 by homoacetogens in the anaerobic granular sludge for the production of acetic acid or other VFAs. However, methanogens in anaerobic sludge act antagonistically with homoacetogens and therefore the main goal of this study is to examine strategies to inhibit methanogenesis and to enrich homoacetogens. Based on this, several strategies were investigated such as the exposure of (a) anaerobic granular sludge to low pH, (b) the short exposure of anaerobic granular sludge to heat, (c) addition of bromoethanesulfonate under various concentrations and (d) exposure of anaerobic granular sludge to salinity 30–90 g NaCl/L. The highest performance (2,020 mg/L of acetic acid) was found when anaerobic granular sludge was exposed to 50 mM of bromoethanesulfonate at 100 g/L (ZVI), pH 6–6.5, after 12 days whereas, the short exposure of anaerobic granular sludge to heat at 100 g/L ZVI at pH 6–6.5 resulted in 1,290 mg/L of acetic acid. The operation of this system in pH 5–6 generated less VFAs compared to operation at pH 6–6–5. The daily regulation of pH to 3 was not practical as the pH was increased to 6.5 due to abiotic anaerobic oxidation of ZVI and this resulted in CH4 production and propionic acid generation. The exposure of anaerobic granular sludge to ZVI and 30 g NaCl/L as well as to seawater resulted in production of CH4 (around 28% of the headspace) and mainly production of acetic acid (550–850 mg/L). The increased in salinity to 60 and 90 g NaCl/L resulted in reduction of CH4 and VFAs however, a more diverse range of VFAs was produced. Exposure of anaerobic granular sludge to heat and then to CO2 and ZVI resulted in an increase of Clostridium sensu stricto to 65.9% and the same genus was increased when anaerobic sludge was exposed to bromoethanesulfonate and ZVI.
Introduction
Substantial interest has been focused over the past decades to human activities that affect the climate system negatively. It is observed that global resources are overexploited and the consumption of energy increases dramatically. As a consequence, the increased needs for energy have led to excessive use of fossil fuels which result in the increase of CO2 emissions with annual anthropogenic emissions of about 25–35 Gt (Ruiz-Valencia et al., in press). To mitigate this, researchers and engineers examined strategies for the transformation of carbon dioxide (CO2) into commodity chemicals and fuels—also known as carbon capture and utilization (CCU) (Prévoteau et al., 2020). However, this is a challenge as CO2 presents kinetic and thermodynamic stability due to the centrosymmetric structure (O=C=O) and therefore, the use of high-energy substances or processes are required for its reduction (Yang et al., 2017).
In the last decade, there is high interest toward microbial electrosynthesis system for the conversion of CO2 to carboxylic acids using anaerobic mix culture as an inoculum via homoacetogens and methanogenesis inhibition (Vassilev et al., 2018). However, as stated in a recent review (Prévoteau et al., 2020) after a decade of research on CO2 microbial electrosynthesis this process is not yet competitive for synthesis of chemicals and there is plenty of room for improvement.
Recently, Vyrides et al. (2018) using anaerobic granular sludge pointed out that CO2 (as a sole carbon source) can be utilized to CH4 by addition of zero valent iron (ZVI) in anaerobic granular sludge under aquatic ambient conditions. Dong et al. (2019) using nano-ZVI, found similar results. ZVI (Fe0) can be oxidized under abiotic aquatic carbonate anaerobic conditions, generating H2 and siderite as shown in the (Equation 1) (Palacios et al., 2019).
The H2 that was abiotically generated (Equation 1) in combination with CO2 can be consumed by hydrogenotrophic methanogens present in anaerobic granular sludge and can generate CH4 (Equation 2) (Vyrides et al., 2018).
Apart from this, H2 and CO2 can be consumed by homoacetogens for the generation of acetic acid (Equation 3)
Moreover, acetic acid can be utilized via another route driven by acetoclastic methanogens for the formation of CH4 and CO2 associated with the following reaction (Equation 4) (Vyrides et al., 2018).
Vyrides et al. (2018) found that the activity of homoacetogens was suppressed by hydrogenotrophic methanogens under 75 g/L ZVI, 5 gVS/L anaerobic granular sludge and CO2 as a sole carbon source.
In the current study a new proof of concept for CO2 (as the only carbon source) conversion to volatile fatty acids under ambient conditions was investigated using anaerobic granular sludge and ZVI at aquatic mild conditions. For this reason, the main objective was to point out strategies to enrich homoactetogenesis and to inhibit methanogenesis in anaerobic granular sludge in the presence of ZVI. Therefore, the following strategies were independently examined: (a) exposure of anaerobic granular sludge to low pH, (b) short exposure of anaerobic granular sludge to heat, (c) addition of bromoethanesulfonate (BES) under various concentrations, and (d) exposure of anaerobic granular sludge to high salinity (30–90 g NaCl/L). Apart from the volatile fatty acids (VFAs) and gas composition, the microbial profile under different operational conditions was monitored to gain an insight of the process.
Materials and Methods
Anaerobic Sludge and Pre-treatment Preparation
Anaerobic granular sludge was collected from a mesophilic upflow anaerobic sludge blanket reactor (UASB) that was treating dairy wastewater at pH 6.8–7.3. The anaerobic granule sludge was washed with phosphate buffer (20 mM) and then was sieved with a #18 mesh (1,000 μm in pore-diameter). Before to be used as inoculum, the anaerobic granular was placed into a bottle (1L) filled with phosphate buffer solution (20 mM) and closed with a rubber septa and flashed with CO2. Two bottles were prepared and were placed in shaking incubator at 33°C at pH 5–6 and pH 6–6.5 for 3 days.
Batch laboratory scale experiments took place in 250 ml serum bottles with a working volume of 100 ml. To each serum bottles 10 g of pre-treatment wet weight sludge and 10 g of ZVI (Iron ≥99%, reduced, powder (fine)—Sigma Aldrich CAS Number 7439-89-6 and code 12310) was added (final concentration of ZVI was 100 g/L). The remained volume of 100 ml was filled with medium reported at (Angelidaki et al., 2009) (however, no Na2S9H2O was added). The pH was monitored (pH meter Mettler Toledo for small volumes) by withdrawing around 1 ml from each serum bottle. The pH was also adjusted using 2 M HCl or 2 M NaOH. In case that no any pH adjustment was needed the equal volume of anaerobic media was added in order to maintain the working volume. The serum bottles were sealed with rubber septa and a screw cup and flushed with 100% of CO2 for 5 min for creating anaerobic conditions (CO2 as a sole carbon source) to a final absolute pressure of 1.5 bars in order to avoid vacuum pressure due to 1 ml sampling from the headspace for gas analysis and then were placed in an incubator (100 rpm) at 33°C. The pressure was measured and monitored with a manometer at each sampling. In all the experiments, controls with granular sludge and medium without methanogenis inhibitor were included. Moreover, control without adding granular sludge that consisted only with ZVI and medium was prepared.
It was found that the exposure of ZVI (100 g/L) and media at initial pH of 5 and pH 6 under anaerobic conditions (only CO2 in the headspace) generated 95% H2 in the headspace after 40 and 48 h, respectively. Therefore, the overall purpose of those experiments (a–e) was to investigate susistanable strategies to inhibit methanogens and to enhance homoacetogens for utilization of external CO2 and the H2 released from ZVI.
All serum bottles were inoculated with anaerobic granular sludge and CO2 was the sole carbon source. The serum bottles were operated at pH 5–6 and pH 6–6–5. The following strategies were tested for methanogenesis inhibition:
a) Daily reduction of pH to 3 in the system of anaerobic granular sludge and 100 g/L ZVI under batch conditions (15 days). The pH was risen due to the anaerobic oxidation of ZVI and therefore HCl (1 M) was daily added. The bottles were flushed with CO2 on the beginning of the experiment and on day 7.
b) Short exposure of anaerobic granular sludge to heat (95°C for 30 min) was tested prior the inoculation of the sludge to 100 g/L ZVI under batch conditions for 12 days. The bottles were flushed with CO2 on the beginning of the experiment and on day 8. At these conditions two pH were operated at pH 5–6 and at pH 6–6.5.
c) Addition of 50 mM 2-Bromoethanesulfonate (BES) was tested under batch conditions in the system of anaerobic granular sludge and 100 g/L ZVI. At the first batch (duration 15 days), CO2 was flushed in the beginning and on day 7. At the second batch (duration 12 days), 90% of media was replaced with new media (without removing anaerobic granular sludge and ZVI) and CO2 was re-flushed in the beginning of the experiment and on day 8. At these conditions two pH were operated at pH 5–6 and pH 6–6.5.
d) Addition of lower BES concentrations (1–10 mM) in the system of anaerobic granular sludge and 100 g/L ZVI. Under these conditions CO2 was re-injected in the system on day 8, 11, and 15 whereas on day 15 the 90% of the liquid media was replaced with a new media without BES addition or ZVI.
e) Exposure of anaerobic granular sludge to 100 g/L ZVI and operation at (a) 30 g NaCl/L, (b) 60 g NaCL/L and (c) 90 g NaCl/L). The batch tests (duration 24 days) were re-flushed with CO2 (as a sole carbon source) in the beginning of the experiment and on days 3, 10, and 17.
The anaerobic batch experiments with anaerobic granular sludge and ZVI were carried out in triplicate and the average and standard deviation is been reported. The controls (anaerobic granular sludge) were done in duplicate and the average was calculated.
Analytical Techniques
The total solids (TS) and volatile solids (VS) of the sieved anaerobic granular sludge were measured according to Standard Methods from APHA. The gas composition (H2, O2, N2, CH4, and CO2 concentrations) from BMP experiment was determined by withdrawing 1 ml from the headspace and by analyzing it in a gas chromatography thermal conductivity detector (GC-TCD) as described by Vyrides et al. (2018). The volume of gas produced was calculated by multiplying the headspace volume by the gas composition (%). The coefficient of variation for 10 identical samples was ±2%. The concentrations of volatile fatty acids VFAs (C1–C5) were analyzed through a high performance liquid chromatography–HPLC (LC-20AD, Shimadzu, Japan) equipped with a Shimadzu SPD20 A UV/VIS detector (Vyrides et al., 2018). The coefficient of variation for 10 identical samples was ±7%. The absolute pressure was measured using a manometer with a fine needle injected to the rubber stopper in the serum bottle (range 2 bar accuracy ±10 mbars).
X-Ray Crystallography of Iron Substrates
X-Ray diffraction (XRD) patterns of selected ZVI specimens, before and after use in the anaerobic bioreactor, were recorded in a multi-purpose diffractometer (Ultima IV, Rigaku). The diffractometer was equipped with a Cu tube operated at 40 kV and 40 mA and the incident beam has been conditioned to parallel mode by a multilayer mirror and partially monochromatized (Cu Ka λ = 0.15418 nm). The high fluorescence of the iron samples has been suppressed by a graphite monochromator located at the receiving side of the optics, just before the detector. XRD patterns were recorded from 20° to 85° 2theta with 0.05° step and 0.5°/min scanning speed.
Next-Generation Sequencing
For bacteria samples (0.2–0.3 g) for DNA extraction were withdrawn from (a) anaerobic granular sludge that was used as an inoculum; (b) anaerobic granular sludge exposed to BES (c) anaerobic granular sludge previously heated and then exposure to ZVI (day 12) (d) anaerobic granular sludge exposed to 50 mM BES and ZVI at the end of the second batch and operated at pH 5–6 and 6–6–5 (e) anaerobic granular sludge exposed to 90 g NaCl/L and 100 g/L ZVI at day 22. The methanogens analysis took place for samples exposed to (a) anaerobic granular sludge (b) anaerobic granular sludge previously heated and then exposure to ZVI and (c) anaerobic granular sludge exposed to 50 mM BES and ZVI at the end of the second batch and operated at 6–6–5. Then, the total genomic DNA was extracted through the FastDNA Spin kit for soil (MP Biomedicals, USA) and then was sent to DNASense Apps Company in Denmark for 454 pyrosequencing. The procedures for bacterial and archaeal 16S rRNA amplicon sequencing were based on Caporaso et al. (2012) and the same protocol that was descripted in the study of Vyrides et al. (2018) was used.
Results
Regulation of pH to 3
The exposure of anaerobic granular sludge at pH 3 was tested as a strategy to inhibit methanogens. At the first 7 days no any inhibitor was added to anaerobic granular sludge and as can be seen from Figure 1 the CH4 composition reached 50% after 7 days at a final absolute pressure 1.5 atm. The H2 initially rose due to anaerobic ZVI oxidation and then it was utilized by hydrogenotrophic methanogens (Figure 1). The VFAs are lower than 50 mg/L and these findings agree with our previous findings Vyrides et al. (2018) (Figure 2) on CO2 utilization to CH4. Thereafter, on day 7 the anaerobic granular sludge were re-flushed with CO2 and the initial pH was initially set to 3. At this period (days 7–15) the pH was daily risen to around 6.5 because of the anaerobic ZVI oxidation (Equation 1) and then was daily regulated to pH 3. This resulted in generation of relatively high propionic acid 230 mg/L and significantly lower concentration from other VFAs (Figure 2).
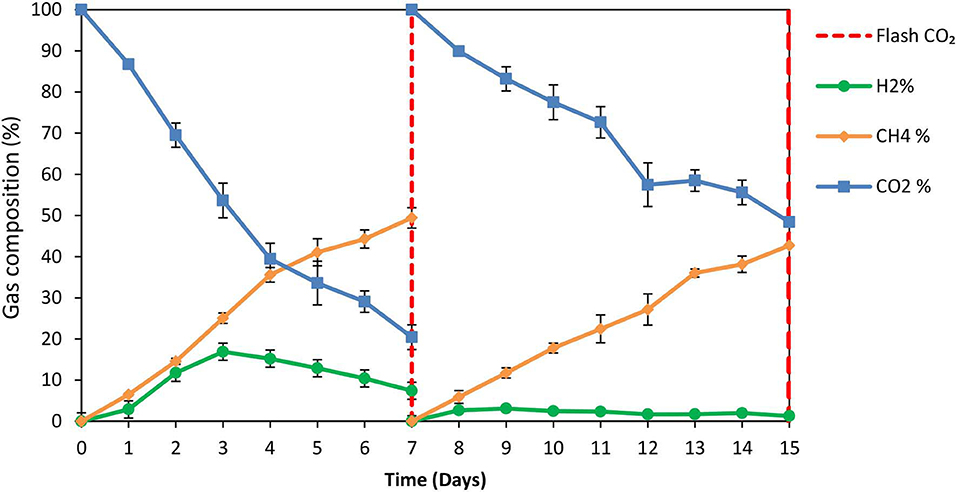
Figure 1. Gas composition over time for anaerobic granular sludge which the pH was daily regulated to pH 3 (after 7th day) in the presence of 100 g/L ZVI. On day 7 the bottles were flushed with CO2.
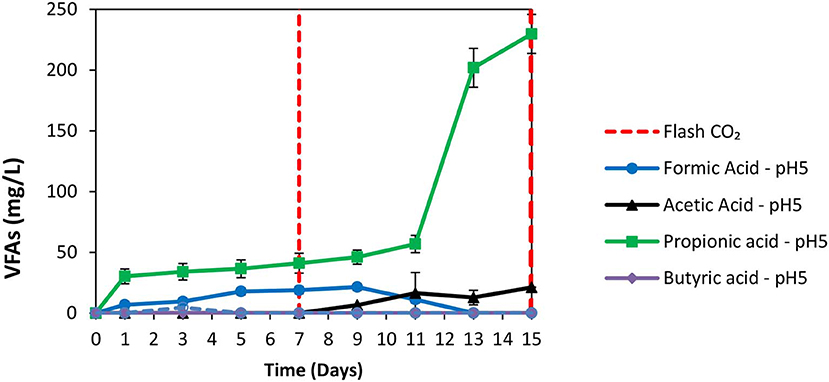
Figure 2. VFAs production over time for anaerobic granular sludge that the pH was daily regulated to pH 3 (after 7th day) in the presence of 100 g/L ZVI.
Heat Pre-treatment of Anaerobic Granular Sludge
The anaerobic granular sludge prior to exposure to ZVI was heated for 30 min to 95°C as strategy to inhibit methanogenesis. During the first 8 days; H2 increased to 60% (for anaerobic granular sludge that the pH regulated to 6–6.5 and to 5–6, respectively) however, after CO2 re flushing (day 8) the H2 (%) at anaerobic granular sludge that the pH regulated to 6–6.5 remained below 1% whereas at the anaerobic sludge that the pH regulated to pH 5–6, H2 increased to 25% on day 11 and reduced to 21% on day 12 (Figure 3). The amount of CH4 was negligible for both conditions and the absolute pressure at day 12 were around 1.4 atm for both conditions. At the end of day 12 the acetic acid for anaerobic sludge exposed to pH 6–6.5 was 1,285 mg/L compared to 650 mg/L for anaerobic sludge that pH was regulated to 5–6 (Figure 4). The other VFAs remained below 130 mg/L for both conditions. On day 12 at the same conditions for anaerobic granular sludge without ZVI (controls) generated in total 395 mg/L and 289 mg/L for pH 6–6–5 and 5–6, respectively. This corresponds to around 26 and 37% of the total VFAs generated in the presence of ZVI and anaerobic granular sludge. The anaerobic granular sludge pre-heated points a potential for this system. However, further experiments need to be done to show the limitations and the frequency for the pre-heat.
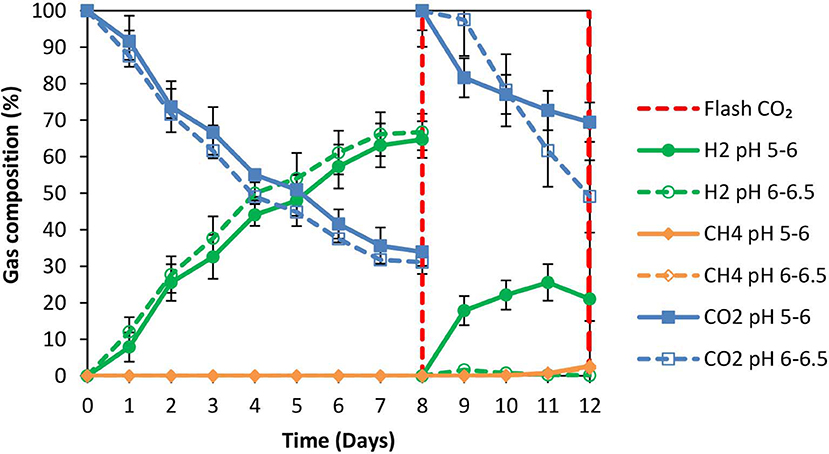
Figure 3. Gas composition over time for anaerobic granular sludge that was pre-heated and then exposed to 100 g/L ZVI and operated at pH 5–6 and pH 6–6.5, respectively.
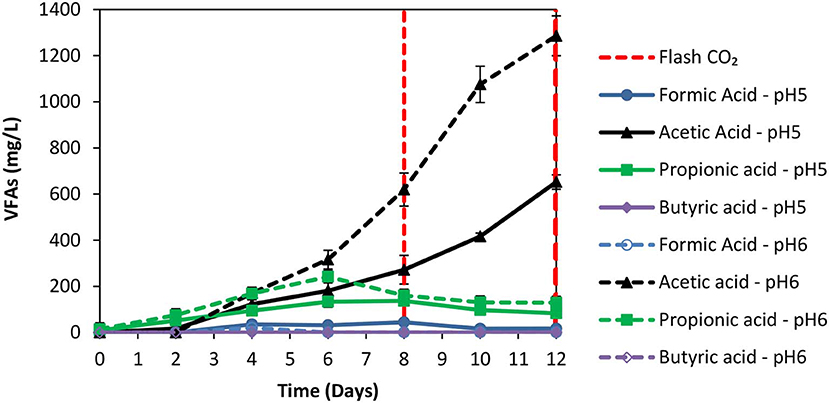
Figure 4. VFAs production over time over time for anaerobic granular sludge that was pre-heated and then exposed to 100 g/L ZVI and operated at pH 5–6 and pH 6–6.5, respectively.
Addition of 50 mM of BES
2-Bromoethanesulfonate (BES) was used as a strategy to inhibit methanogenesis and to enrich homoacetogens in anaerobic granular sludge within the system (ZVI, anaerobic granular sludge and CO2 as a sole carbon source). As was stated in the material and methods 50 mM of BES and 100 g/L ZVI were only added at the beginning of the experiment and anaerobic granular sludge was exposed to pH 5–6 and 6–6.5, respectively. As shown in Figure 5; during the first 15 days, methanogenesis was fully inhibited and no significant amount of CH4 was detected for both pHs (pH 5–6 and 6–6.5). From day 0 to day 7, the CO2 (%) decreased and at the same period the H2 increased to 70–85%. The increase of H2 could be attributed to the anaerobic oxidation of ZVI (Equation 1). Then, after CO2 flushing on day 7; the H2 increased (for anaerobic sludge exposed to pH 6–6.5) from day 7 to day 12 and then decreased probably due to the consumption of H2 and CO2 by homoacetogens (Equation 3). On the other hand, at pH 5–6 the H2 has risen to 70% possibly due to more favorable thermodynamic condition for the abiotic production of H2 form ZVI (Equation 1) compared to the activity of homoacetogens (Equation 3). The absolute pressure was 1.3 and 1.5 atm for sample exposed to pH 6–6.5 and pH 5–6, respectively. At this period, the concentration of the VFAs was increased over time for both conditions (pH regulated to 5–6 and pH regulated to 6–6.5) (Figure 6A). In the first 7 days, for both conditions formic acid was the dominant VFAs. However, after CO2 re-flushing (day 7) a high production of acetic acid (Figure 6A) was detected (1,736 mg/L for anaerobic sludge at pH 6–6.5 and 600 mg/L for anaerobic sludge at pH 5–6). The 2nd higher VFA for anaerobic sludge exposed to pH 6–6.5 was propionic acid (500 mg/l) whereas, butyric acid was 157 mg/L and formic acid was almost reduced to zero. The same trend but in lower concentrations of VFAs (Figure 6A) was found for anaerobic sludge exposed to pH 5–6.
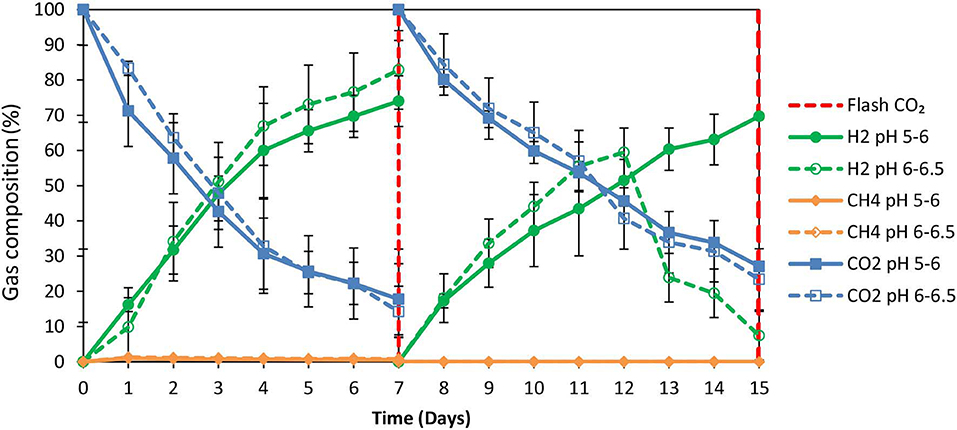
Figure 5. Gas composition (%) over time for anaerobic granular sludge with 50 mM BES, 100 g/L ZVI and operation at pH 5–6 and pH 6–6.5, respectively.
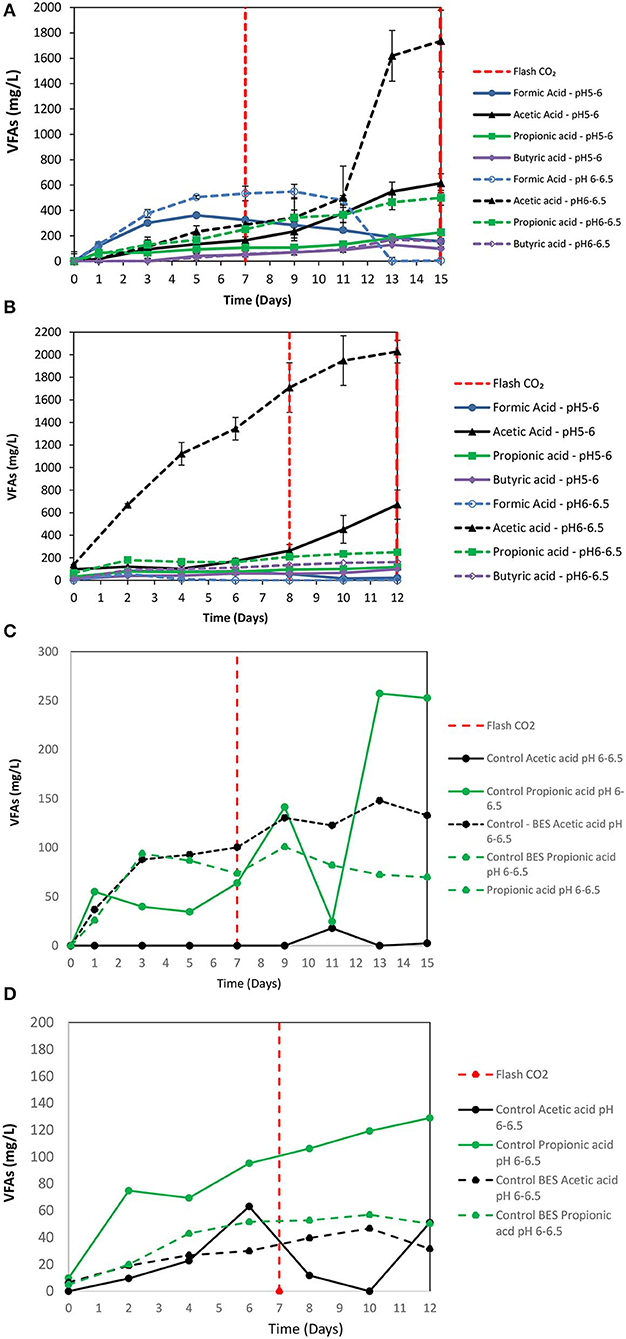
Figure 6. (A) First batch: VFAs production over time for anaerobic granular sludge with 50 mM BES, 100 g/L ZVI and operation at pH 5–6 and pH 6–6.5, respectively. (B) second batch: VFAs production over time for anaerobic granular sludge with 50 mM BES, 100 g/L ZVI and operation at pH 5–6 and pH 6–6.5, respectively. (C) VFAs production over time for anaerobic granular sludge (control) and anaerobic granular sludge with BES and operation at pH 6–6.5. No ZVI were added in the controls. (D) VFAs production over time for anaerobic granular sludge (control) and anaerobic granular sludge with BES and operation at pH 6–6.5. No ZVI were added in the controls.
At the 2nd batch were 90% of media was replaced (without removing anaerobic granular sludge and ZVI) and CO2 was re-flushed. During this period, no CH4 was detected and H2 followed the same patent as the first bath (data not shown). As shown in Figure 6B, anaerobic granular sludge exposed to pH 6–6.5 (in BES and 100 g/L ZVI) the acetic acid reached 2,020 mg/L on day 12 whereas, other VFAs was in substantially lower concentrations (Figure 6B). At these conditions, at lower pH 5–6 the same trend regarding the VFAs was found however, acetic acid was at significantly lower concentration (670 mg/L). Two controls were operated in parallel without addition of ZVI to test if the generated VFAs was due to the addition of ZVI and not due to cell lysis of anaerobic granular sludge (Figures 6C,D). The 1st control contained only anaerobic granular sludge and media (no ZVI and BES were added) and the 2nd control contained anaerobic granular sludge and BES and media with no ZVI. The results showed that at the 1st cycle the VFAs from the controls were lower than 250 mg/L whereas the VFAs at the 2nd cycle were lower than 150 mg/L. Based on this, it was calculated that the VFAs that was due to soluble microbial products were around 10% of the total VFAs produced in the presence of ZVI, anaerobic granular sludge and CO2 as the only external carbon source. The CO2 (%) for control and control with BES under these conditions were around 95 and 99%, respectively. Minor amounts of H2 (<0.5%) were detected for both controls. Similar results (VFAs and gas composition) were found for the controls operated at pH 5–6. These findings points out that the main caused of VFAs generation by anaerobic granular sludge from CO2 was due to ZVI addition and not due to cell lysis of anaerobic granular sludge.
Addition of Lower BES Concentrations (1–10 mM)
Based on the results of 50 mM BES (section Addition of 50 mM of BES) on this system (anaerobic granular sludge, ZVI, BES, and CO2 as a sole carbon source) we have examined lower BES concentrations 1–10 mM on VFAs generation over time. As shown in Figure 7, the VFAs for all the samples exposed to BES increased from day 1 to 15. The highest VFAs (2,066 mg/L) were generated at day 15 by anaerobic granular sludge exposed to 4 mM BES whereas at 2 mM BES and 6 mM BES the VFAs were 1,904 and 1,784 mg/L, respectively. On day 15 after replacing the 90% of the media with a new media, the anaerobic granular sludge that was initially exposed to 4 mM BES generated the highest amount of VFAs 1,572 mg/L (day 22) where the rest of samples (2–10 mM BES) generated between 750 and 984 mg/L VFAs. The main VFA generated by the samples that was exposed to BES was the acetic acid (Figure 7) which has significantly increased after CO2 re-flushing on day 8. Under these conditions, formic acid reached its highest value (140–360 mg/L) on day 9 and then gradually decreased (Figure 7). The production of propionic acid gradually increased for all the samples at around 220–360 mg/L and after the media was replaced; the remained at 150 mg/L. Butyric acid was not detected during the first 8 days and then was gradually increase to 100–150 mg/L for all the samples exposed to BES (Figure 7). In the first 8 days CH4 was generated by all samples with the highest composition (27% CH4) at 1 mM BES (Figure 8A). The low acetic acid during the first 8 days could be due to the utilization of acetic acid by acetoclastic methanogens. However, after CO2 re flushing on days 8 and 11 only the anaerobic sludge subjected to 1 mM generated higher than 2% CH4. The replacement of the media on day 15 resulted in rapid CH4 generation from anaerobic sludge initially exposed to 1 mM BES and 2 mM BES. At the first 8 days, the samples exposed to 4 mM BES −10mM pointed out higher absolute pressure compared to the rest of the samples as well as higher H2 (%) (Figure 8B). This could be attributed due to severe inhibition of hydrogenotrophic methanogens by BES and as a result less H2 (%) was consumed (Figure 8B). Form day 8 to 15 the H2 decrease over time (for samples exposed to 4 mM BES) and this is an indication of the activity of homoacetogens. The samples that pointed out the highest VFAs during this period had the fastest decreased of absolute pressure over time due to the activity of homoacetogens.
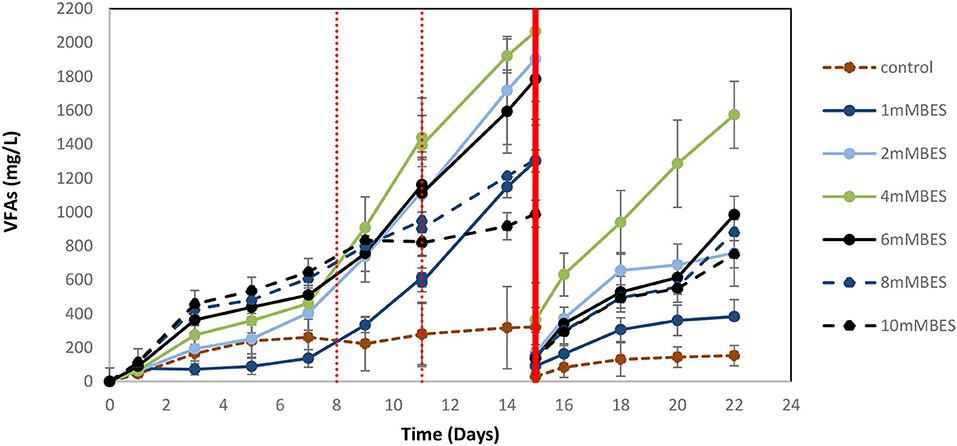
Figure 7. VFAs production over time for anaerobic granular sludge exposed to 1–10 mM BES, 100 g/L ZVI and operation at pH 6–6.5. On day 15, 90% of the media was re-placed.
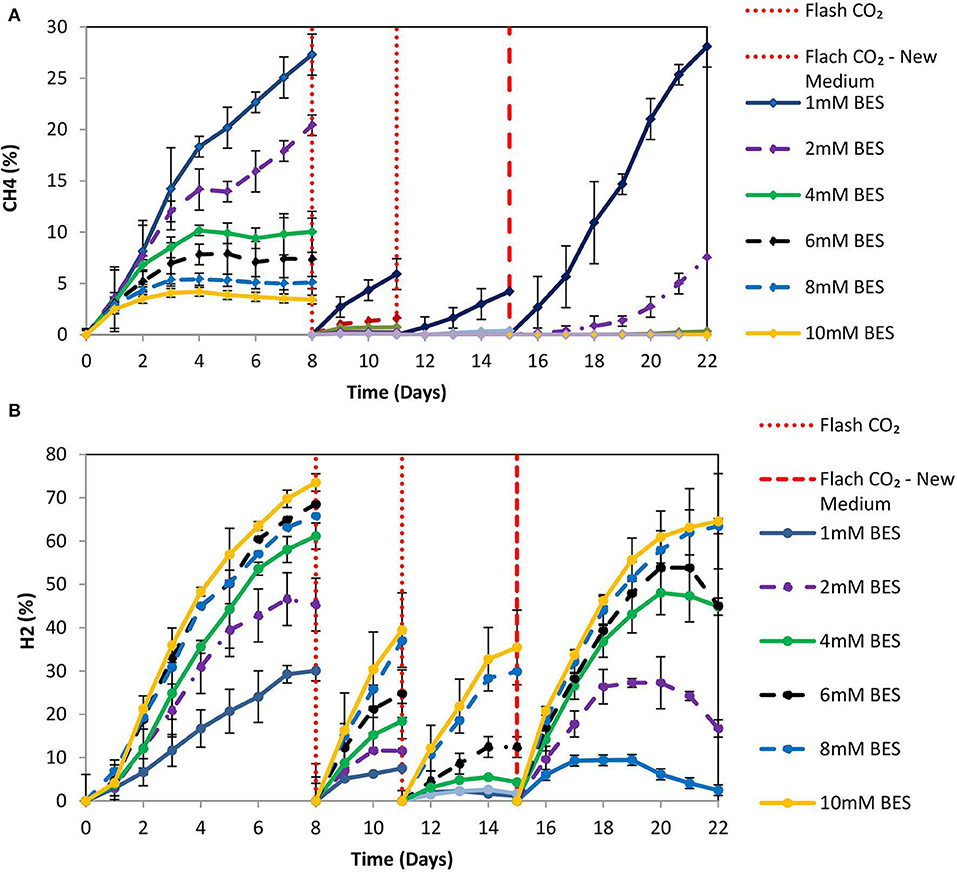
Figure 8. (A) CH4 production over time for anaerobic granular sludge exposed to 1–10 mM BES, 100 g/L ZVI and operation at pH 6–6.5. On day 15, 90% of the media was replaced with a new media. (B) H2 production over time for anaerobic granular sludge exposed to 1–10 mM BES, 100 g/L ZVI and operation at pH 6–6.5. On day 15, 90% of the media was replaced with a new media.
Exposure of Anaerobic Granular Sludge at 30, 60, and 90 g NaCl/L
Vyrides and Stuckey (2009) found that when anaerobic sludge fed with glucose at salinity level higher than 20 g NaCl/L resulted in methnaogensis inhibition and accumulation of VFAs. Based on the higher inhibition of methanogens to high salinity compared to acetogens we have individually tested this system (anaerobic granular sludge and 100 g ZVI/L) at 30, 60, and 90 g NaCl/L as another strategy to inhibit methanogenesis without severely inhibit homoacetogens.
On day 10 at 30 g NaCl/L the VFAs were at their higher concentration (848 mg/L) and then gradually declined to 510 mg/L (Figure 9A). At these conditions, acetic acid was the main VFA although on day 24 butyric acid, propionic acid and acetic had around the same concentration (150 mg/L) (Figure 9A). The gradual decrease of acetic acid could be due to the adaptation of acetoclastic methanogens to salinity and the utilization of acetic acid. As shown in Figure 10A after each CO2 re-flushing, the CH4 was around 28%. Therefore, at 30 g NaCl/L simultaneous generation of CH4 and VFAs from CO2 as a sole carbon source was obtained by anaerobic sludge in the presence of ZVI. Interestingly, the same trend (in both VFAs and gas composition) was found when seawater was used instead of 30 g NaCl/L. Potentially this system could be used in sea vessel for CO2 utilization, first by adsorption of CO2 in reactor with seawater and then the saturated with CO2 seawater can be used in a system of anaerobic granular sludge and ZVI.
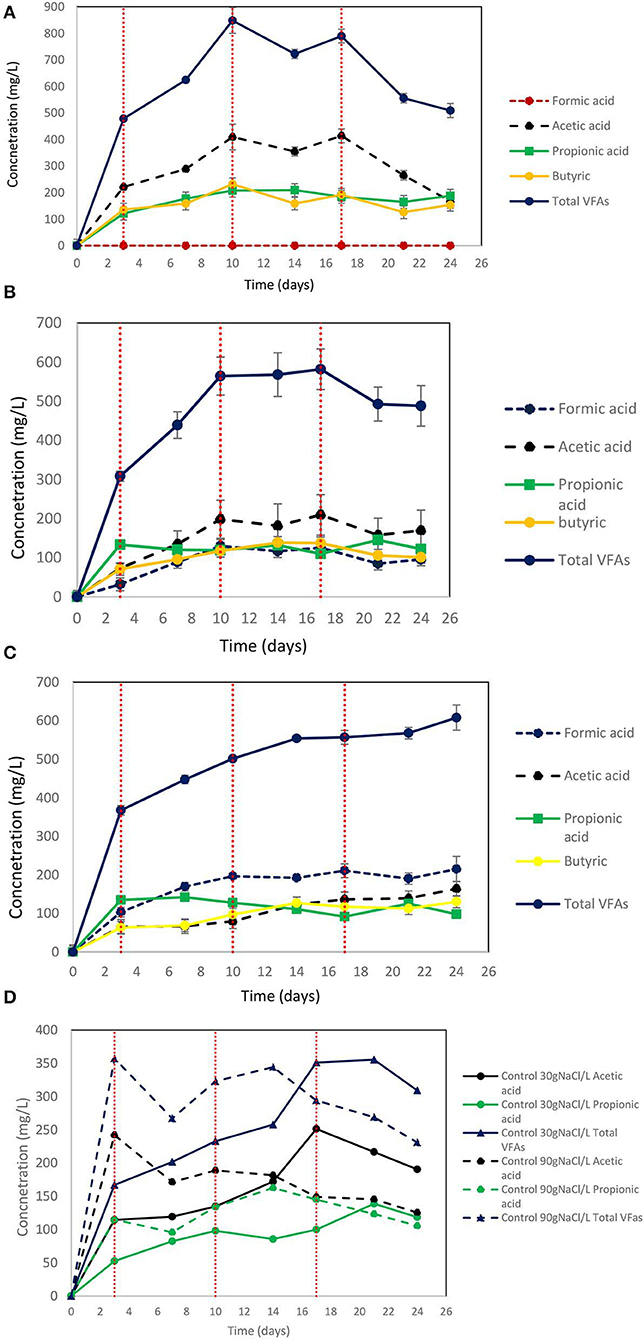
Figure 9. (A) Production of VFAs by anaerobic granular sludge exposed to 30 g NaCl/L, 100 g ZVI/L and CO2 as a sole carbon source. CO2 re flushing took place on days 3, 10, and 17. (B) Production of VFAs by anaerobic granular sludge exposed to 60 g NaCl/L, 100 g ZVI/L and CO2 as a sole carbon source. CO2 re flushing took place on days 3, 10, and 17. (C) Production of VFAs by anaerobic granular sludge exposed to 90 g NaCl/L, 100 g ZVI/L and CO2 as a sole carbon source. CO2 re flushing took place on days 3, 10, and 17. (D) Production of VFAs by control anaerobic granular sludge exposed to 30 and 90 g NaCl/L and CO2 as a sole carbon source. No ZVI were added to controls. CO2 re flushing took place on days 3,10, and 17.
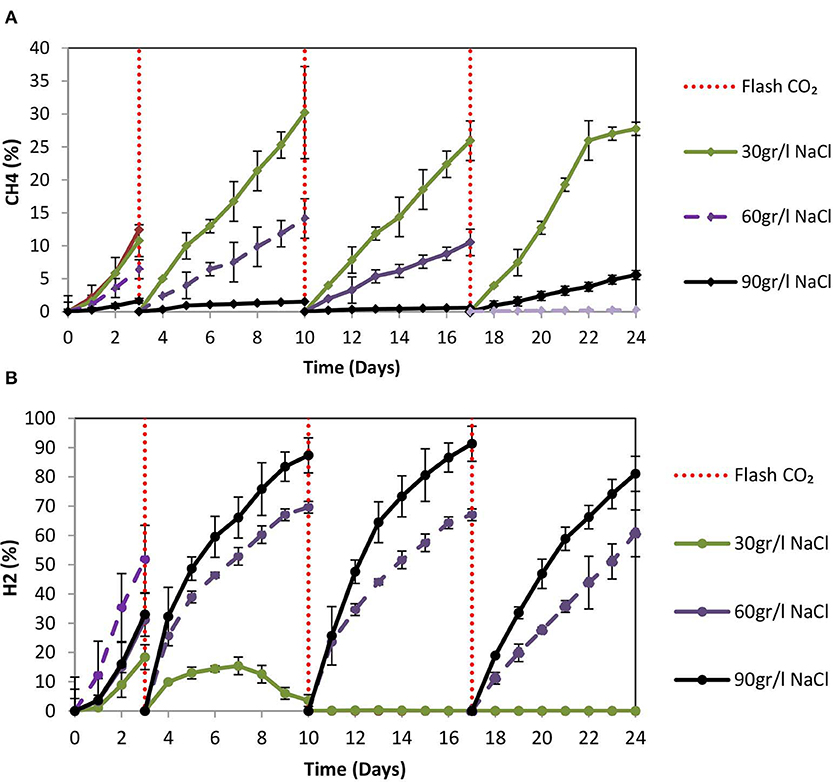
Figure 10. (A) CH4 (%) production over time by anaerobic granular sludge exposed to 100 g ZVI/L and salinity of 30, 60, and 90 g NaCL/L. (B) H2 (%) production over time by anaerobic granular sludge exposed to 100 g ZVI/L and salinity of 30, 60, and 90 g NaCL/L.
On day 24, at salinity of 60 and 90 g NaCl/L the VFAs were around 488 and 608 mg/L, respectively (Figures 9B,C). At 60 g NaCl/L the acetic acid was slightly higher compared to other VFAs (Figure 9B) however at 90 g NaCL/L formic acid was around 100 mg/L higher compared to the other VFAs (Figure 9C). At control 30 g NaCl/L without ZVI addition the anaerobic granular sludge generated maximum 355 mg/L VFAs which this consist of 42% of the VFAs produced in the presence of ZVI (Figure 9D). The control anaerobic granular sludge exposed to 90 g NaCL/L generated maximum VFAs 357 mg/L which is the 58% of the VFAs generated in the same conditions in the presence of ZVI.
At 60 g NaCl/L the CH4 (%) on day 24 was around 4% whereas at 90 g NaCL/L the CH4 was <0.5% (Figure 10A). On day 24, under these conditions H2 (%) was around 74 and 61% for samples exposed to 90 and 60 g NaCl/L (Figure 10B). On day 24, the absolute pressure for samples exposed to 30, 60, and 90 g NaCL/L was 1.3, 1.4, and 1.5 atm, respectively.
Microbial Profile of Anaerobic Granular Sludge at ZVI, CO2 as a Sole Carbon Source and Methanogenesis Inhibition
The relative abundance of each bacterial phylum and genera were calculated based on the average of the two OTUs of the replicates. The bacteria microbial profile at phylum and genera level can be seen in Figure 11. When anaerobic granular sludge was pre-heated and then exposed to CO2 and ZVI resulted in an increase of Firmicutes from 7 to 65.9% detected in the control (anaerobic granular sludge). Specifically Clostridium sensu stricto was the genera that detected under these conditions and was not detected at all at the control. The Clostridium sensu stricto also detected in anaerobic granular sludge exposed to BES, ZVI and pH 5–6 and 6–6.5 at relative high abundance of 22 and 24%, respectively (Figure 11). Vyrides et al. (2018) also identified Clostridium sensu stricto as one of the most dominant genus in anaerobic granular sludge exposed to ZVI and CO2. Interestingly, Philips et al. (2018) found Clostridium sensu stricto at 10% relative abundance from an anoxic corrosion crust sample enriched with ZVI. In addition, studies that used micron-sized zero valent iron (ZVI) (Huang et al., 2019) and ZVI nanoparticles (Yin and Wang, 2019) in anaerobic digester treating swine manure and macroalgae identified Clostridium sensu stricto in anaerobic bacteria.
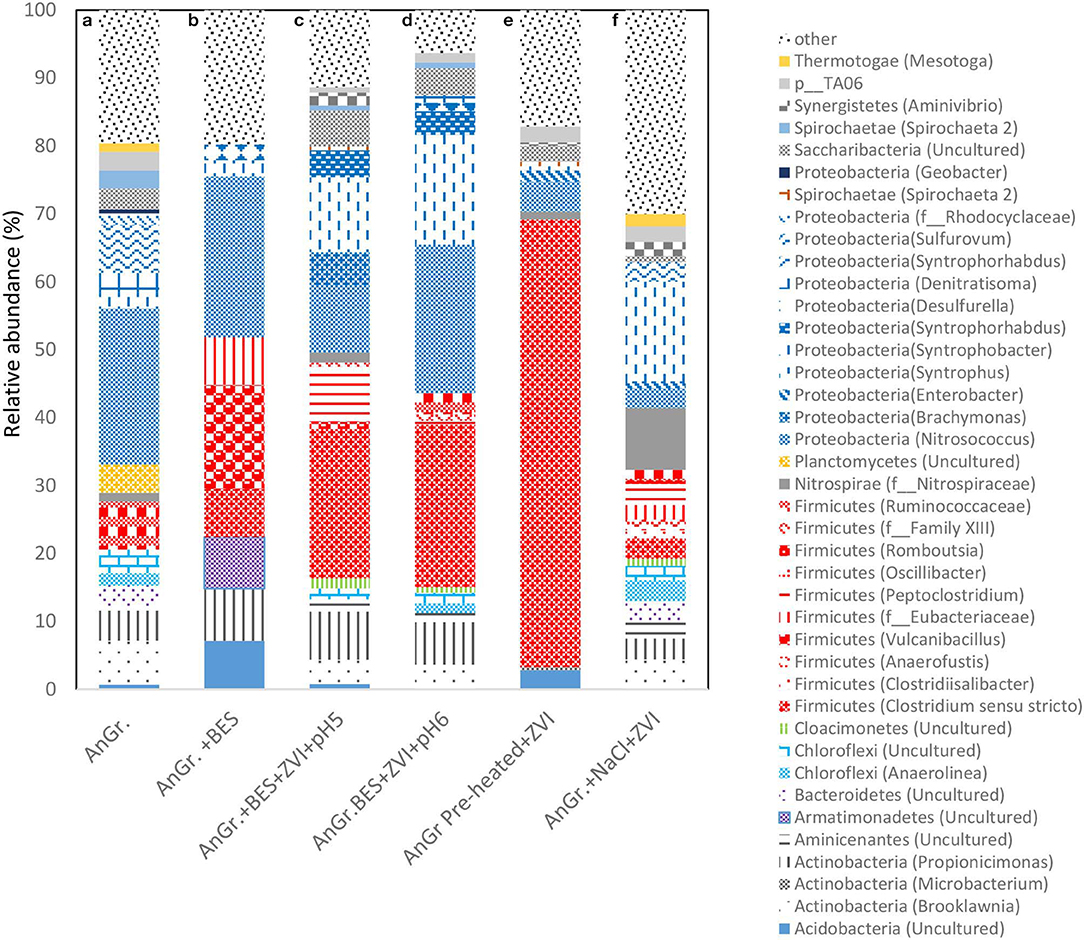
Figure 11. Relative abundance of bacterial community at the phylum and genus level at the end of batch experiment for (a) anaerobic granular sludge (AnGr.), (b) anaerobic granular sludge and BES (AnGr. + BES), (c) anaerobic granular sludge, BES, ZVI and operation at pH 5–6 (AnGr. + BES + ZVI + pH 5), (d) anaerobic granular sludge, BES, ZVI and operation at pH 6–6.5 (AnGr. + BES + ZVI + pH 6), (e) anaerobic granular sludge previously heated, ZVI and operation at pH 6–6.5 (AnGr. pre-heated + ZVI), (f) anaerobic granular sludge exposed to 90 g NaCl/L and ZVI (AnGr. NaCl + ZVI).
Moreover, substantial increase of Syntrophobacter genus was detected in samples that were exposed in anaerobic granular sludge ZVI and BES operated at pH 6–6.5 (8.7%), pH 5–6 (14.3%) as well as to anaerobic granular sludge exposed to salinity and ZVI (14%) (Figure 11). The relative abundance of this species in anaerobic granular sludge and anaerobic granular sludge exposed to BES was around 2%. Syntrophobacter can grow on propionate in syntrophic association with methanogens for growth. These syntrophic propionate oxidizers require hydrogen-consuming partners to keep the hydrogen partial pressure low enough for propionate oxidation to be thermodynamically favorable. It is possible under ZVI the high H2 partial pressure could lead to the microbial reduction of CO2 to butyric and/or propionic acid; and then these products (butyric and propionic acid) can be further degraded with Syntrophobacter and homoacetogenic bacteria (Clostridium sensu stricto or others) to acetic acid. Noteworthy, representatives of the genus Shewanella and/or Acetobacterium, Sporomusa that were previously detected in anaerobic environment and ZVI (Kato et al., 2015; Philips et al., 2018) were below the detection limit (0.01% relative abundance) in all samples and this could be due to the origin of the inoculum (anaerobic granular sludge).
The methanogens were also analyzed at the end of batch experiment in order to point out the genus that survive under these conditions and can reduce the performance of the system by consumption of acetic acid and/or H2. As one can see in Figure 12; the exposure of anaerobic granular sludge to ZVI and BES resulted in Methanosaeta genera decreased to 29.7% compared to the control 52.1% whereas, the Methanobacterium genera significantly increased to 38.6% compared to 3.3% of the control. This is in line with the findings of Xu et al. (2010) that found that acetoclastic methanogens were more sensitive to BES compared to hydrogenotrophic methanogens. On the other hand, the pre-exposure to heat resulted in significant increase of Methanosaeta species to 74.4% compared to the control. This could be due to availability of high concentrations of acetic acid under this conditions and the resistance to heat of Methanosaeta genus due to thick, relatively impervious S-layers that form a sheath which encompasses the cell wall (Bhattad et al., 2017).
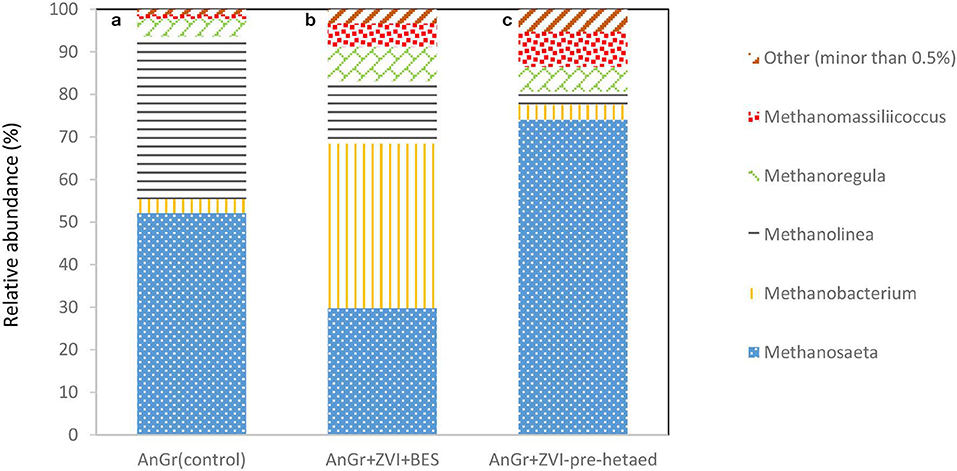
Figure 12. Relative abundance of archaeal at the genus level at the end of batch experiment for (a) anaerobic granular sludge (control) (AnGrControl), (b) anaerobic granular sludge exposed to BES and ZVI (AnGr+ZVI+BES), and (c) pre-heated anaerobic granular sludge and then exposed to BES and ZVI (AnGr+ZVI+pre−heated).
Structural Characterization of ZVI Before and After Exposure to Anaerobic Granular Sludge and CO2
Figure 13 shows the XRD patterns of (a) the as received ZVI, and of the ZVI after exposure to anaerobic granular sludge with (b) BES inhibitor (27 days) and (c) NaCl inhibitor (24 days). The ZVI powder exhibits three diffraction peaks at 2θ = 44.7°, 2θ = 65.0°, and 2θ = 82.3° which correspond to the body-centered cubic (BCC) crystalline alpha phase of iron (α-Fe), commonly referred to as ferrite (Figure 13A, black line). The absence of any other peaks confirms the purity of iron and the lack of any iron oxides or any other crystalline phases (impurities) within the initial as received material. On the other hand, the ZVI sample with BES inhibitor after 27 days of the anaerobic process (Figure 13B, red line) exhibits peaks that can be primarily allocated to siderite (FeCO3) and pure α-Fe. The siderite peaks can be attributed to the oxidization products under aquatic carbonate anaerobic conditions, in line with the reaction of Equation (1), whereas the presence of Fe relates to the unreacted part of ZVI suggesting that the transformation is not yet complete after 27 days. Furthermore, a minor quantity of magnetite (Fe3O4) is also present as shown by the peak at 2θ = 35.4° which is suggested as a secondary product of the oxidation reaction. The XRD pattern of the ZVI sample with NaCl inhibitor (Figure 13C, green line), exhibits peaks of siderite and pure Fe, similarly to the sample with BES inhibitor. It appears that the magnetite formation within this reaction is insignificant. Beyond that, two minor NaCl peaks are also observed which could relate to remnants of NaCl crystals that were left undiluted/unreacted.
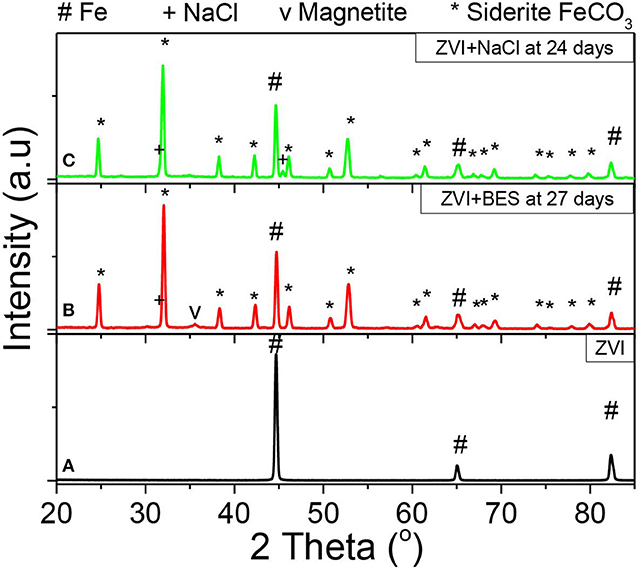
Figure 13. XRD data from the surfaces of (A) ZVI (black line), (B) ZVI after 27 days exposure to anaerobic granular sludge, BES and CO2 (red line), and (C) ZVI after exposure for 24 days to 60 g NaCl/L anaerobic granular sludge and CO2 (green line).
Discussion
A new approach for CO2 conversion to acetic acid and other VFAs under ambient conditions using ZVI and anaerobic granular sludge was examined. For this reason, several strategies for methanogenesis inhibition was tested such as exposure to low pH, heat pre-treatment of anaerobic granular sludge, addition of BES and operation at high salinity.
Up to date, there are several physicochemical technologies for CO2 mitigation (Muñoz et al., 2015). On the other hand, using chemoautotrophic microbes; CO2 can be converted into CH4 or other high value added products at mild operational and environmental conditions (Angelidaki et al., 2018). Specifically, the power-to-gas technologies are based on the conversion of H2 and CO2 to CH4 by hydrogenotrophic methanogens (Equation 2) at an in-situ biological process or at ex-situ biological process. In addition, many researchers used microbial electrosynthesis (MES) for CO2 reduction to carboxylic acids (Jiang et al., 2019; Prévoteau et al., 2020) however as was reported in recent reviews (Bajracharya et al., 2017; Jiang et al., 2019; Prévoteau et al., 2020) no pilot or full scale MES study has been reported to date and one of the main challenge in the MES system is the cost reduction (Jiang et al., 2019). On the other hand, as was stated in this proof of concept study using ZVI and anaerobic granular sludge; the CO2 can be converted to carboxylic acids without the complicate configuration of microbial electrosynthesis (electrodes, proton exchange membrane, and connection with power supply). The findings of our study show that 2,200 mg/L (after 12 days) can be produced using anaerobic granular sludge, ZVI and BES whereas the anaerobic sludge previous exposure to heat can generate around 1,300 mg/L after 12 days. The anaerobic granular sludge exposure to ZVI and 30 g NaCl/L generated 848 mg/L of VFAs after 12 days and around 30% of the headspace consisted of CH4. The same trend (in both VFAs and gas composition) was detected when seawater was used instead of 30 g NaCl/L. This system could be used in sea vessel for CO2 utilization or in industries that generate high CO2 close to the coast. The CO2 can be firstly absorb in reactor with seawater and then the saturated with CO2 seawater can be used in a system of anaerobic granular sludge and ZVI. By increasing the salinity to 60 and 90 g NaCl/L a decreased in the CH4 content and the concentrations of VFAs was found. However, at a higher salinity a more diverse range of VFAs were produced and this can potentially increase the economic benefit of the system.
This is a “proof of concept study” and factors that need to be consider prior the application of this process at larger scale could be: the cost of BES or the sludge pre-treatment cost, the recovery cost of VFAs, and a crucial factor could also be the regeneration of ZVI. Interestingly, in the current experiments the ZVI was added in relatively high concentration at the beginning of the experiment and it shows that can retain its activity over the CO2 re-feeding over 27 days. This agrees with our previous study (Vyrides et al., 2018) which it was found that ZVI was only added once in anaerobic granular sludge and was sufficient for the production of the same amount of CH4 over 2 CO2 re-feedings for a total of 67 days. This is in line with the findings in Figure 13; initially high concentration of ZVI was used (100 g/L) and the ZVI was not fully oxidized to siderite therefore its retain part of its activity. Nevertheless, the conversion of oxidized ZVI (mainly siderite) to ZVI is an important factor that need to be investigated in future studies in order the process to become more sustainable. So far few studies has examined this; Jin et al. (2011) used glycerin at 280°C in the presence of NaOH to regenerate the ZVI that was previously oxidized to Fe(OH)3. In addition, several studies (Steinfeld, 2002; Jin et al., 2014) proposed the use of concentrated solar energy for the reduction of oxidized metal (ZnO) to native metal (Zn0). Several researchers examined the synthesis of nano ZVI from iron ions using polyphenols derived from plant extracts or agrowaste. This process occurs under ambient conditions and is a low cost sustainable method for synthesis of nano ZVI (Hoag et al., 2009; Herlekar et al., 2014; Stefaniuk et al., 2016). However, according to Mystrioti et al. (2015) the process of synthesis of nano-ZVI from plant or biomass extracts can be limited due to the formation of mixture of other iron such as iron oxides of iron hydroxides. As another strategy to reduce the cost of this process (conversion of CO2 to VFAs using ZVI and anaerobic sludge) the ZVI can be replace by scrap ZVI. Scrap ZVI which is 5–10 times cheaper than ZVI (Alibaba.com, 2020) has been used added in laboratory anaerobic bioreactors for waste and wastewater treatment and an increase of methane were found (Zhen et al., 2015; Wang et al., 2017).
An additional benefit of the use of ZVI in this system could be related with H2 solubilization. The H2 is naturally released from ZVI inside the liquid phase whereas, in several power-to-gas systems must cross the interface between the gas and the liquid phase in order to be available for the microorganisms (Tirunehe and Norddahl, 2016; Angelidaki et al., 2018; Maegaard et al., 2019). At the examined system anaerobic microorganisms could create biofilm on the ZVI or could be attached Philips et al. (2018) and therefore a high H2 utilization could be obtained at a higher efficiency compared to power-to-gas system. Philips et al. (2018) stated that the main mechanisms of Fe(0) corrosion under microbial anaerobic condition is hydrogen depended and could be due to extracellular components (possibly hydrogenase enzymes) as was reported by Deutzmann et al. (2015) or by the maintenance of low H2 concentrations by attached cells on Fe(0). In our study, in samples that low H2 composition (in the headspace) was detected, a high concentration of acetic acid was also found; and this most likely was due to the utilization of H2 and CO2 by homoacetogens. Apart from H2 utilization several researchers reported that anaerobic microorganisms could potentially directly utilize electrons from ZVI for the generation of acetic acid from CO2 (Kato, 2016) although a detailed understanding of the extracellular electron mechanism(s) of acetogens remains lacking (Kato, 2016; Philips et al., 2018).
Noteworthy, to increase the efficiency and to implement this system at larger scale (ZVI, anaerobic granular sludge and CO2 as a sole carbon source) strategies for methanogenesis inhibition over long period should be established. In addition, other products such as butyric acid and or ethanol that have higher value than acetic acid (Jiang et al., 2019) can also be targeted within this system in order to increase the profit from the system. Moreover, a comparison of the performance and on the cost for this system with a system that H2 is provided externally to anaerobic biomass and/or with microbial electrosynthesis could be done to evaluate the strength and limitation of this metal bio process.
Conclusions
A new approach for CO2 utilization (as a sole carbon source) to acetic acid and other VFAs under ambient conditions using zero valent iron and anaerobic granular sludge was investigated by methanogens inhibition and homoactetogenesis enrichment. In this proof of concept study using ZVI and anaerobic granular sludge; the CO2 can be converted to carboxylic acids without the complicate configuration of microbial electrosynthesis (electrodes, proton exchange membrane, and connection with power supply). To inhibit methanogenesis and to enrich homoacetogens the following strategies were examined such as the exposure of (a) anaerobic granular sludge to low pH, (b) the short exposure of anaerobic granular sludge to heat, (c) addition of bromoethanesulfonate under various concentrations, and (d) exposure of anaerobic granular sludge to salinity 30–90 g NaCl/L. The highest performance (2,020 mg/L of acetic acid) was found when anaerobic granular sludge was exposed to 50 mM of BES at 100 g/L (ZVI), pH 6–6.5, after 12 days whereas, the short exposure of anaerobic granular sludge to heat at 100 g/L ZVI at pH 6–6.5 resulted in 1,290 mg/L of acetic acid after 12 days. The daily regulation of pH to 3 was not practical as the pH was increased to 6.5 due to abiotic anaerobic oxidation of ZVI and this resulted in CH4 production and propionic acid generation. The exposure of anaerobic granular sludge to ZVI and 30 g NaCl/L or to seawater resulted in production of CH4 (around 28% of the headspace) and mainly production of acetic acid (550–850 mg/L). The increased in salinity to 60 and 90 g NaCl/L resulted in reduction of CH4 and VFAs however, a more diverse range of VFAs was produced. Exposure of anaerobic granular sludge to heat and then to CO2 and ZVI resulted in an increase of Clostridium sensu stricto to 65.9% and the same genus was increased when anaerobic sludge was exposed to bromoethanesulfonate and ZVI.
Data Availability Statement
All datasets generated for this study are included in the article/supplementary material.
Author Contributions
CS carried out the main experimental activities and wrote the first draft of the manuscript. GC and LK contacted the XRD analysis, contributed to the discussion section regarding the role of ZVI oxidized products, and revised the manuscript. IV designed the methodology of the work and revised the manuscript.
Funding
The authors would like to thank Cyprus University of Technology for covering the open access fees. The authors acknowledge the financial support for this work by Research Promotion Foundation (RPF) (Cyprus) under the project P2P/M-ERA.NET/0317/0008 (BElectroCathode).
Conflict of Interest
The authors declare that the research was conducted in the absence of any commercial or financial relationships that could be construed as a potential conflict of interest.
References
Alibaba.com (2020). Available online at: https://www.alibaba.com (accessed February 8, 2020).
Angelidaki, I., Alves, M., Bolzonella, D., Borzacconi, L., Campos, J. L., Guwy, A. J., et al. (2009). Defining the biomethane potential (BMP) of solid organic wastes and energy crops: a proposed protocol for batch assays. Water sci technol. 59, 927–934. doi: 10.2166/wst.2009.040
Angelidaki, I., Treu, L., Tsapekos, P., Luo, G., Campanaro, S., Wenzel, H., et al. (2018). Biogas upgrading and utilization: current status and perspectives. Biotechnol. Adv. 36, 452–466. doi: 10.1016/j.biotechadv.2018.01.011
Bajracharya, S., Srikanth, S., Mohanakrishna, G., Zacharia, R., Strik, D. P., and Pant, D. (2017). Biotransformation of carbon dioxide in bioelectrochemical systems: state of the art and future prospects. J. Power Sources 356, 256–273. doi: 10.1016/j.jpowsour.2017.04.024
Bhattad, U., Venkiteshwaran, K., Cherukuri, K., Maki, J. S., and Zitomer, D. H. (2017). Activity of methanogenic biomass after heat and freeze drying in air. Environ. Sci. Water Res. Tech. 3, 462–471. doi: 10.1039/C7EW00049A
Caporaso, J. G., Lauber, C. L., Walters, W. A., Berg-Lyons, D., Huntley, J., Fierer, N., et al. (2012). Ultra-high-throughput microbial community analysis on the Illumina HiSeq and MiSeq platforms. ISME J. 6, 1621–1624. doi: 10.1038/ismej.2012.8
Deutzmann, J. S., Sahin, M., and Sportmann, A. M. (2015). Extracellular enzymes facilitate electron uptake in biocorrosion and bioelectrosynthesis. mBio 6, 1–15 doi: 10.1128/mBio.00496-15
Dong, D., Aleta, P., Zhao, X., Choi, O. K., Kim, S., and Lee, J. W. (2019). Effects of nanoscale zero valent iron (nZVI) concentration on the biochemical conversion of gaseous carbon dioxide (CO2) into methane (CH4). Bioresour. Technol. 275, 314–320. doi: 10.1016/j.biortech.2018.12.075
Herlekar, M., Barve, S., and Kumar, R. (2014). Plant-mediated green synthesis of iron nanoparticles. J. Nanoparticles 2014:140614. doi: 10.1155/2014/140614
Hoag, G. E., Collins, J. B., Holcomb, J. L., Hoag, J. R., Nadagouda, M. N., and Varma, R. S. (2009). Degradation of bromothymol blue by ‘greener'nano-scale zero-valent iron synthesized using tea polyphenols. J. Mater. Chem. 19, 8671–8677. doi: 10.1039/b909148c
Huang, W., Yang, F., Huang, W., Wang, D., Lei, Z., and Zhang, Z. (2019). Weak magnetic field significantly enhances methane production from a digester supplemented with zero valent iron. Bioresour. Technol. 282, 202–210. doi: 10.1016/j.biortech.2019.03.013
Jiang, Y., May, H. D., Lu, L., Liang, P., Huang, X., and Ren, Z. J. (2019). Carbon dioxide and organic waste valorization by microbial electrosynthesis and electro-fermentation. Water Res. 149, 42–55. doi: 10.1016/j.watres.2018.10.092
Jin, F., Gao, Y., Jin, Y., Zhang, Y., Cao, J., Wei, Z., et al. (2011). High-yield reduction of carbon dioxide into formic acid by zero-valent metal/metal oxide redox cycles. Energy Environ. Sci. 4, 881–884. doi: 10.1039/c0ee00661k
Jin, F., Zeng, X., Liu, J., Jin, Y., Wang, L., Zhong, H., et al. (2014). Highly efficient and autocatalytic H2O dissociation for CO2 reduction into formic acid with zinc. Sci. Rep. 4:4503. doi: 10.1038/srep04503
Kato, S. (2016). Microbial extracellular electron transfer and its relevance to iron corrosion. Microb. Biotechnol. 9, 141–148. doi: 10.1111/1751-7915.12340
Kato, S., Yumoto, I., and Kamagata, Y. (2015). Isolation of acetogenic bacteria that induce biocorrosion by utilizing metallic iron as the sole electron donor. Appl. Environ. Microbiol. 81, 67–73. doi: 10.1128/AEM.02767-14
Maegaard, K., Garcia-Robledo, E., Kofoed, M. V. W., Agneessens, L. M., de Jonge, N., Nielsen, J. L., et al. (2019). Biogas upgrading with hydrogenotrophic methanogenic biofilms. Bioresour. Technol. 287:121422. doi: 10.1016/j.biortech.2019.121422
Muñoz, R., Meier, L., Diaz, I., and Jeison, D. (2015). A review on the state-of-the-art of physical/chemical and biological technologies for biogas upgrading. Rev. Environ. Sci. Bio. 14, 727–759. doi: 10.1007/s11157-015-9379-1
Mystrioti, C., Sparis, D., Papasiopi, N., Xenidis, A., Dermatas, D., and Chrysochoou, M. (2015). Assessment of polyphenol coated nano zero valent iron for hexavalent chromium removal from contaminated waters. Bull. Environ. Contam. Tox. 94, 302–307. doi: 10.1007/s00128-014-1442-z
Palacios, P. A., Snoeyenbos-West, O., Löscher, C. R., Thamdrup, B., and Rotaru, A. E. (2019). Baltic Sea methanogens compete with acetogens for electrons from metallic iron. ISME J. 13, 3011–3023. doi: 10.1038/s41396-019-0490-0
Philips, J., Monballyu, E., Georg, S., De Paepe, K., Prévoteau, A., Rabaey, K., et al. (2018). An Acetobacterium strain isolated with metallic iron as electron donor enhances iron corrosion by a similar mechanism as Sporomusa sphaeroides. FEMS Microbiol. Ecol. 95:fiy222. doi: 10.1093/femsec/fiy222
Prévoteau, A., Carvajal-Arroyo, J. M., Ganigu,é, R., and Rabaey, K. (2020). Microbial electrosynthesis from CO2: forever a promise? Curr. Opin. Biotechnol. 62, 48–57. doi: 10.1016/j.copbio.2019.08.014
Ruiz-Valencia, A., Benmeziane, D., Pen, N., Petit, E., Bonniol, V., Belleville, M. P., et al. (in press). CO2 valorization by a new microbiological process. Catal Today doi: 10.1016/j.cattod.2019.03.053
Stefaniuk, M., Oleszczuk, P., and Ok, Y. S. (2016). Review on nano zerovalent iron (nZVI): from synthesis to environmental applications. Chem. Eng. J. 287, 618–632. doi: 10.1016/j.cej.2015.11.046
Steinfeld, A. (2002). Solar hydrogen production via a two-step water-splitting thermochemical cycle based on Zn/ZnO redox reactions. Int. J. Hydrogen Energy 27, 611–619. doi: 10.1016/S0360-3199(01)00177-X
Tirunehe, G., and Norddahl, B. (2016). The influence of polymeric membrane gas spargers on hydrodynamics and mass transfer in bubble column bioreactors. Bioprocess Biosyst. Eng. 39, 613–626. doi: 10.1007/s00449-016-1543-7
Vassilev, I., Hernandez, P. A., Batlle-Vilanova, P., Freguia, S., Krömer, J. O., Keller, J., et al. (2018). Microbial electrosynthesis of isobutyric, butyric, caproic acids, and corresponding alcohols from carbon dioxide. ACS Sustain. Chem. Eng. 6, 8485–8493. doi: 10.1021/acssuschemeng.8b00739
Vyrides, I., Andronikou, M., Kyprianou, A., Modic, A., Filippeti, A., Yiakoumis, C., et al. (2018). CO2 conversion to CH4 using Zero Valent Iron (ZVI) and anaerobic granular sludge: optimum batch conditions and microbial pathways. J. CO2 Util. 27, 415–422. doi: 10.1016/j.jcou.2018.08.023
Vyrides, I., and Stuckey, D. C. (2009). Effect of fluctuations in salinity on anaerobic biomass and production of soluble microbial products (SMP s). Biodegradation 20, 165–175. doi: 10.1007/s10532-008-9210-6
Wang, D., Ma, W., Han, H., Li, K., and Hao, X. (2017). Enhanced treatment of Fischer–Tropsch (FT) wastewater by novel anaerobic biofilm system with Scrap Zero Valent Iron (SZVI) assisted. Biochem. Eng. J. 117, pp.66–76. doi: 10.1016/j.bej.2016.09.012
Xu, K., Liu, H., and Chen, J. (2010). Effect of classic methanogenic inhibitors on the quantity and diversity of archaeal community and the reductive homoacetogenic activity during the process of anaerobic sludge digestion. Bioresour. Technol. 101, 2600–2607. doi: 10.1016/j.biortech.2009.10.059
Yang, H., Zhang, C., Gao, P., Wang, H., Li, X., Zhong, L., et al. (2017). A review of the catalytic hydrogenation of carbon dioxide into value-added hydrocarbons. Catal. Sci. Technol. 7, 4580–4598. doi: 10.1039/C7CY01403A
Yin, Y., and Wang, J. (2019). Enhanced biohydrogen production from macroalgae by zero-valent iron nanoparticles: Insights into microbial and metabolites distribution. Bioresour. Technol. 110–117. doi: 10.1016/j.biortech.2019.121808
Keywords: anaerobic granular sludge, CO2 utilization, Clostridium sensu stricto, homoacetogenesis, hydrogenotrophic methanogens, zero valent iron, volatile fatty acids
Citation: Samanides CG, Koutsokeras L, Constantinides G and Vyrides I (2020) Methanogenesis Inhibition in Anaerobic Granular Sludge for the Generation of Volatile Fatty Acids from CO2 and Zero Valent Iron. Front. Energy Res. 8:37. doi: 10.3389/fenrg.2020.00037
Received: 23 November 2019; Accepted: 25 February 2020;
Published: 13 March 2020.
Edited by:
Greeshma Gadikota, Cornell University, United StatesReviewed by:
Wei Liu, Molecule Works Inc., United StatesBo Fu, Jiangnan University, China
Deepika Malhotra, Pacific Northwest National Laboratory (DOE), United States
Copyright © 2020 Samanides, Koutsokeras, Constantinides and Vyrides. This is an open-access article distributed under the terms of the Creative Commons Attribution License (CC BY). The use, distribution or reproduction in other forums is permitted, provided the original author(s) and the copyright owner(s) are credited and that the original publication in this journal is cited, in accordance with accepted academic practice. No use, distribution or reproduction is permitted which does not comply with these terms.
*Correspondence: Ioannis Vyrides, ioannis.vyrides@cut.ac.cy