- 1Instituto de Química, Universidade Federal do Rio de Janeiro, Rio de Janeiro, Brazil
- 2Escola de Química, Universidade Federal do Rio de Janeiro, Rio de Janeiro, Brazil
- 3INCT Energia & Ambiente, Universidade Federal do Rio de Janeiro, Rio de Janeiro, Brazil
Four iron-based catalysts supported on niobium oxide (Nb2O5) were prepared by impregnation and characterized by TPR, FRX, DRX, and textural analysis. The catalysts were tested in a fixed bed continuous flow unit to convert a mixture of CO2 and H2 to hydrocarbons at atmospheric pressure. All catalysts were active in the range of 623–723 K. The selectivity to hydrocarbons and light olefins increases with the use of alkali metal promoters (K or Cs). At 673 K, the Fe-Cr-K/Nb2O5 showed 26% selectivity to light olefins, representing 5% yield with 20% CO2 conversion. The niobium oxide-supported catalysts were more active and selective than the same catalyst formulation supported on SiO2.
Introduction
Over the past century, utilization of carbon-rich fossil fuels like coal, oil, and natural gas has led to a significant improvement of the lifestyle of the human civilization. However, the concentration of CO2 in the atmosphere, emitted upon the burning of fossil fuels, has surpassed 400 ppm in 2013; an increase of more than 40% relative to the pre-industrial era (Monastersky, 2013). Because CO2 is a greenhouse gas, its increasing concentration in the atmosphere is rising the temperature of the planet, leading to global warming and climate changes (Song, 2006).
In recent years, technologies for capturing and storing the CO2 released from the burning of fossil fuels have emerged (Li et al., 2013; Leung et al., 2014; Koytsoumpa et al., 2018). Many are commercially available today, but still poorly implemented. On the other hand, the concept of CO2 utilization, where the captured gas is transformed into useful fuels and chemicals is gaining importance (Centi et al., 2013; Dutta et al., 2017).
Carbon dioxide is thermodynamically stable, with an enthalpy of formation of −393.5 kj.mol−1. Therefore, its transformation into more valuable products usually requires high energy input. Today, CO2 is mostly used in the synthesis of urea and salicylic acid (Aresta et al., 2013). Methanol can also be industrially produced from CO2 (Pérez-Fortes et al., 2016). Nevertheless, organic carbonates, that are valuable chemicals (Aresta and Dibenedetto, 2002; Sakakura and Kohno, 2009; North et al., 2010; Aresta et al., 2015; Ozorio and Mota, 2017), as well as hydrocarbons (Yang et al., 2017), appear as promising products that could be obtained from CO2.
The production of hydrocarbons from CO2 might be essentially viewed as a modification of the Fischer–Tropsch synthesis (FTS), where the CO2 molecule is firstly converted into CO, which then react with H2 to form hydrocarbons (Wang et al., 2011). The first reaction is also called reverse water gas shift (RWGS), being highly endothermic and conducted at elevated temperatures (Dama et al., 2018), whereas the FTS is exothermic (Scheme 1).
Light olefins are key feedstocks in the chemical industry for the manufacture of a wide range of products, like polymers (Amghizar et al., 2017). Ethylene and propylene are the main olefin feedstocks of the chemical industry. They are produced worldwide in huge amounts and their synthesis is the single most energy-consuming process in the chemical industry. Light olefins are usually produced by the steam cracking of naphtha (Ren et al., 2006), but can also be obtained from other sources, such as methanol (Tian et al., 2015) and ethanol (Mohsenzadeh et al., 2017). Producing olefins from CO2 can be viewed as a way of storing CO2 for long periods of time in the form of polymers (Centi et al., 2011). Ideally, the hydrogen needed in the process can come from a renewable source, such as water electrolysis or photocatalysis. The Fischer-Tropsch synthesis is not normally employed in the synthesis of olefins. However, the use of potassium has been shown to significantly enhance the selectivity to olefins in FTS (Arakawa and Bell, 1983; Raje et al., 1998; Cheng et al., 2017). The probable cause is the decrease of hydrogen adsorption, because potassium is an electron donor to the metal catalysts.
Satthawong et al. (2015) studied the conversion of CO2 to light olefins over alumina-supported Fe-Co catalysts. At 573 K and 10 atm, the selectivity to olefin was enhanced by the addition of K to the catalyst. The acid-base properties of the surface hydroxyl groups affect the dispersion of the Fe particles, influencing the activity (Ding et al., 2014). Zhang et al. (2015) reported the preparation of microwave-assisted Fe-Zn-K catalysts to be used in the hydrogenation of CO2 to olefins. At 593 K and 5.0 atm, they observed 51% of CO2 conversion with a selectivity of 54% in light olefins. Visconti and collaborators showed (Visconti et al., 2016) that the selectivity to olefins and the hydrocarbon chain size increase with the promotion of K over Fe-based catalysts.
Shi et al. (2018) studied the effect of alkali metals promotion on the performance of CoCu/TiO2 catalysts for the CO2 hydrogenation to long-chain hydrocarbons (C5+). They found that the introduction of alkali metals on the catalyst composition could increase the CO2 adsorption and decrease the H2 chemisorption. Among the promoters studied, sodium provided the highest selectivity to C5+, 42%, with 18.4% of CO2 conversion. The results were explained in terms of the strong basicity of the catalyst.
Ngantsoue-Hoc et al. have investigated the effect of alkali metal ions on metallic iron catalyst in FTS. It was found that the addition of Cs+ improves the activity of Fe in FTS and improves the selectivity to light olefins. Compared with other alkali metals, Cs+ presented the highest selectivity to ethene; ~0.8 in terms of (/ + C2) ratio at 1.308 MPa and 543 K. The unpromoted catalyst showed the (/ + C2) ratio of 0.2. On the other hand, Cs promotion presented the lowest CO conversion (Ngantsoue-Hoc et al., 2002). Furthermore, potassium has been shown to act as an electronic promoter in FTS rather than as a structural one (Saeidi et al., 2014). Choi et al. (1996) studied Fe-K supported on alumina with varying K/Fe ratio, from 0 to 1.0, at 573 K and 20 atm. They found that the olefin to paraffin ratio (O/P) ranged from 0 to 5.8, with CO2 conversions raging between 49 and 68%. Wang et al. (2013) reported the use of iron-based catalysts, aiming the production of light olefins. They tested different supports, finding the best results in terms of selectivity to olefins on ZrO2.
Nb2O5 is used as support in many catalytic reactions (Nowak and Ziolek, 1999). There are some studies on the use of Nb2O5 as support of Fischer-Tropsch catalysts using CO (Soares et al., 1993; Mendes et al., 2006a; Den Otter and De Jong, 2014; Hernández Mejía et al., 2017), but the hydrogenation of CO2 is considerably less studied over metal-supported Nb2O5 catalysts. Nozaki et al. (1987) reported the use of Rh/Nb2O5 catalyst in CO2 hydrogenation to hydrocarbons. At 623K and atmospheric pressure, they found 11% of CO2 conversion, but the main product was methane with 58% selectivity. Hydrocarbons with two or more carbon atoms (C2+) were produced in 37% selectivity of 37%, but the authors did not report the olefin/paraffin ratio. In this work, we report the CO2 hydrogenation over niobium oxide-supported Fe catalysts, aiming to improve the selectivity to light olefins.
Experimental
A sample of Nb2O5, supplied by CBMM (Brazil), was initially calcined at 873 K for 120 min prior to the preparation of the catalysts. This calcination produced a crystalline phase of Nb2O5 (Den Otter and De Jong, 2014), which was then used as support. Previous studies indicated (Mendes et al., 2006a) that metal catalysts supported on non-calcined Nb2O5 are not active in FTS.
The catalysts based on Fe or Fe-Cr, without promotion, were prepared by precipitation. A suspension containing about 8.5 g of the calcined Nb2O5 in 400 mL of deionized water was put in contact with the desired amount of the precursor nitrate salts [7.3 g of Fe(NO3).9H2O, 3.9 g of Cr(NO3).9H2O]. Then, a 1 mol.L−1 solution of NaOH was added drop wise to simultaneously precipitate the metal oxides over the niobium oxide support. After filtration, the resulting solid was washed with deionized water and dried overnight at 403 K. In the case of the catalysts with alkali metal promotion (K or Cs), the impregnation technique was used, due to the solubility of the correspondent bases in aqueous media. The iron and chromium nitrates, as well as the alkali metal salts (KNO3 or CsCl), were dissolved in 500 mL of deionized water and put in contact with the niobium oxide support. In the sequence, water was carefully evaporated at 343 K at reduced pressure, resulting in a solid, which was dried overnight at 403 K. No further calcination of the catalysts was performed. In control experiments, we have observed that calcination of the prepared catalysts significantly altered the TPR profile, leading to less active materials.
The catalysts were named Fe/Nb2O5, Fe-Cr/Nb2O5, Fe-Cr-K/Nb2O5 and Fe-Cr-Cs/Nb2O5. A catalyst supported on SiO2 was also prepared for comparison purpose and named Fe-Cr-K/SiO2.Textural characterization of the catalysts was measured by N2 adsorption using a Micromeritics ASAP 2020 instrument. Powder X-ray diffraction (XRD) patterns were performed using a Shimadzu 6,000 instrument equipped with CuKα target, operating at 40 kV and 30 mA. Temperature-programmed reduction (TPR) was carried out using 50 mg of the catalyst, pretreated on a stream of Ar (30 mL.min−1), at 823 K for 1 h, and then cooled to room temperature. The TPR profile was measured by passing a flow (30 mL.min−1) of 3 vol%H2 and 97 vol% Ar, heating from room temperature to 1,273 K at rate of 10 K.min−1. The chemical composition was measured by X ray Fluorescence (XRF).
Carbon dioxide hydrogenation was carried out in a continuous flow stainless-steel reactor. Typically, 0.5 g of the catalyst was loaded in the reactor and firstly pretreated under H2 gas flow (30 mL.min−1) at 773 K for 1 h. After this procedure, the reactor was cooled down and a mixture of H2/CO2, with a molar ratio of 3:1, respectively, was passed (30 mL.min−1) over the catalyst at 623, 673, and 723 K. The pressure was kept at 1 atm in all tests. The products were separated and analyzed using an on-line gas chromatograph equipped with flame ionization detector (FID) and thermal conductivity detector (TCD).
Results and Discussion
The metal composition of the prepared catalysts and the textural characterization are shown in Table 1. The results indicated that the experimental chemical compositions are close to the nominal ones. Some deviation has occurred in the Fe content of the Fe/Nb2O5 and on the K content of the Fe-Cr-K/Nb2O5. In general, the composition of all prepared catalysts are similar in terms of Fe and Cr content, considering the experimental errors of the method. The specific area of the niobium support significantly reduces upon calcination. This is due to the formation of a crystalline phase of niobium oxide. The area decreased upon impregnation of the metals, except in the case of Fe-Cr/Nb2O5. The catalyst supported on SiO2 presented a significantly higher surface area, characteristic of the silica support.
The temperature programmed reduction profiles (TPR) of the catalysts are shown in Figure 1. The profile of Fe/Nb2O5 shows four peaks, suggesting three steps of iron reduction. Niobium oxide presents a broad peak above 1,173 K. Nevertheless, this peak is not clear or is shifted to higher temperatures in the impregnated catalysts. This point has already been observed in other works and may be due to metal-support interaction, which may affect the reducibility of the Nb2O5 (Eleutério et al., 1998; Noronha et al., 2000; Rezende et al., 2012; Hernández Mejía et al., 2017).
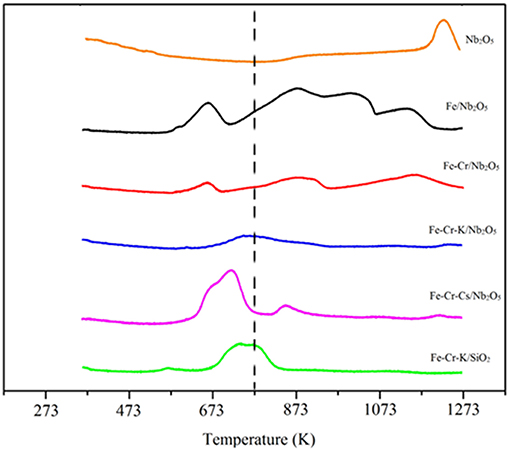
Figure 1. TPR profiles of the catalysts. The dotted line indicates the temperature of reduction inside the reactor, prior to CO2 hydrogenation.
The Fe-Cr/Nb2O5 catalyst shows a broad peak around 673 K, probably associated with the concomitant reduction of Fe3+ and Cr3+. A broad peak starts appearing around 773 K, with maximum at 873 K, and can be attributed to the reduction of Fe2+. Fe-Cr-K/Nb2O5 and Fe-Cr-Cs/Nb2O5 catalysts have main reductions at similar temperature ranges.
Figure 2 shows the X-ray diffractograms of the catalysts. The patterns are associated with the crystalline Nb2O5 phases. The most intense peaks, at 2θ = 23 and 29°, correspond to the TT-phase or T-phase. The catalyst supported on silica showed no appreciable X-ray diffraction, indicative of an amorphous structure.
The conversion and selectivity of the different catalysts in the CO2 hydrogenation, at three different temperatures, are shown in Tables 2–4. The selectivity to CO usually increases with temperature. This may be explained by the thermodynamics of the two reactions. Whereas, the RWGS is endothermic and favored at higher temperatures, the FTS is exothermic and less favored when the temperature goes up. The CO2 conversion was significantly lower on the catalyst promoted with Cs. The same behavior was observed on Ru supported over graphite, where lower CO conversion was observed in the FTS upon Cs promotion (Eslava et al., 2017). The possible explanation is the strong CO adsorption on Cs, due to its more basic nature. Considering the acid character of CO2, a similar behavior would be expected, explaining the lower CO2 conversions upon the Cs-promoted catalyst. The catalyst supported on SiO2 was slightly less active than the catalyst supported on Nb2O5 of similar chemical composition, in spite of the significantly higher surface area. A similar conclusion was reached on Fischer-Tropsch studies over Co/Al2O3 and Co/Nb2O5 (Den Otter and De Jong, 2014). Both catalysts presented almost the same activity per unit of weight, but the Co content impregnated on the niobium oxide support was 5-fold lower than over Al2O3.
The conversion of CO2 to hydrocarbons is expected to occur through two consecutive reactions: RWGS, where CO2 is reduced to CO, and FTS, where CO and H2 reacts to yield the hydrocarbons. The first reaction is endothermic, whereas the second is exothermic. The results at 723 K indicated that the Cr-containing Nb2O5-supported catalysts showed higher conversion than the catalyst containing only Fe over the same support. The exception was the Cs-promoted catalyst, which showed the lowest conversion, probably due to the more basic nature, as previously discussed.
Lohitharn et al. (2008) observed that chromium improved the activity of the supported iron catalyst in the Fischer-Tropsch synthesis. The authors ascribed the higher activity to the better Fe dispersions. The improvement of the activity of iron-based catalysts with chromium addition was also evidenced in this work, but it seems to be related to the fact that Cr is a good catalyst for RWGS and this may be the main reason for the higher conversion over Cr-containing catalysts, especially at higher temperatures. On the other hand, the selectivity to CO was higher on Fe-Cr/Nb2O5 catalyst than on Fe/Nb2O5. Although the different conversion level impairs a more precise discussion, the results suggest that the higher amount of Fe in the Fe/Nb2O5 favors FTS, decreasing the amount of CO in the reaction medium. Thus, a proper balance of the metals is important to control the activity and selectivity of the catalyst. Promotion with K (Fe-Cr-K/ Nb2O5) did not alter much the conversion and CO selectivity in relation to Fe-Cr/Nb2O5 at 723K. The main effect is on the selectivity to olefins. In fact, it seems that at 723 K, RWGS is becoming more important and seems to be governing the whole process.
The effect of K promotion is clearer at lower temperatures. Data at 623 and 673K suggest that besides influencing the selectivity to olefins, alkali promotion may have a role in the conversion of CO (FTS), as the selectivity for this product is lower on Fe-Cr-K/Nb2O5 than on Fe-Cr/Nb2O5. In fact, it has been reported that K promotion has a positive role in the formation of hydrocarbons in the hydrogenation of CO2 (Ning et al., 2018).
Methane was the main hydrocarbon produced over all catalysts, except on Fe-Cr-Cs/Nb2O5 at 623 and 673 K, where light olefins were higher in selectivity. As expected, the promotion with K and Cs significantly increased the selectivity to light olefins and decreased the paraffin to olefin ratio. Promotion also decreased the formation of CO, yielding more hydrocarbon products. The selectivity to light olefins passes to a maximum at 673 K for the Fe-Cr-K/Nb2O5 and Fe-Cr-Cs/Nb2O5 catalysts, being 25 and 26%, respectively. The non-promoted, Fe-Cr/Nb2O5 catalyst, showed the highest selectivity to olefins (13%) at 723 K. At lower temperatures, more paraffinic hydrocarbons were formed, whereas at higher temperatures the selectivity to methane increases. These trends are normally observed in traditional Fischer-Tropsch process. However, when CO2 is used as feedstock, the first reaction is the endothermic RWGS and a compromise between conversion and selectivity to methane and CO must be pursued.
The promotion with Cs gave the highest selectivity to olefins, reaching 26% at 673 K, but with a conversion of only 6%, considerably lower when compared with the other catalysts. This behavior has already been observed in studies with CO hydrogenation and was associated with the higher basicity of the catalyst due to the presence of Cs (Eslava et al., 2017). In contrast, Fe-Cr-K/Nb2O5 presented a selectivity to light olefins of 25% at 673 K, but with a conversion of 20%, indicating a more favorable balance between activity and selectivity. No hydrocarbon with more than four carbon atoms was observed in all experiments, which might be due to the conditions of low pressure and high temperature used in the experiments.
The performance of the niobium-supported catalysts was superior than the silica-supported one, especially at higher temperatures, where formation of olefins is more favored. For instance, at 673 K the CO2 conversion was 20% over Fe-Cr-K/Nb2O5 and the selectivity to light olefins was 26%, whereas over Fe-Cr-K/SiO2 the conversion and selectivity to olefins were 13%. Since both catalysts have similar chemical compositions, it may be assumed that niobium oxide support leads to a more active and selective catalyst. Nevertheless, it is not completely clear why iron catalysts supported over Nb2O5 are more active toward FTS than similar catalyst formulation supported on SiO2. Strong metal support interaction has been reported on Co/Nb2O5 and Co-Pd/Nb2O5 Fischer-Tropsch catalysts, and associated with good catalyst performance (Mendes et al., 2006a,b; Schmal and Freund, 2009). The TPR results of the impregnated catalysts did not clearly show the reduction of the Nb2O5 support, which occurs in temperatures above 1,173 K. This may be an indication of such strong interaction, as already reported for Fe/Nb2O5, through XPS and Mösbauer spectroscopy (Cagnoli et al., 2007), leading to the reduction of the niobium oxide support at higher temperatures.
The effect of different supports on the catalytic performance of Fe promoted with 1wt% of K (Wang et al., 2013) is shown in Table 5, for comparison purpose. One can see that ZrO2 presented the highest yield of light olefins among the tested supports, with Al2O3 and CNT coming in second and third places. Nevertheless, all catalysts produced C5+ hydrocarbons, probably due to the high pressure used in the experiments.
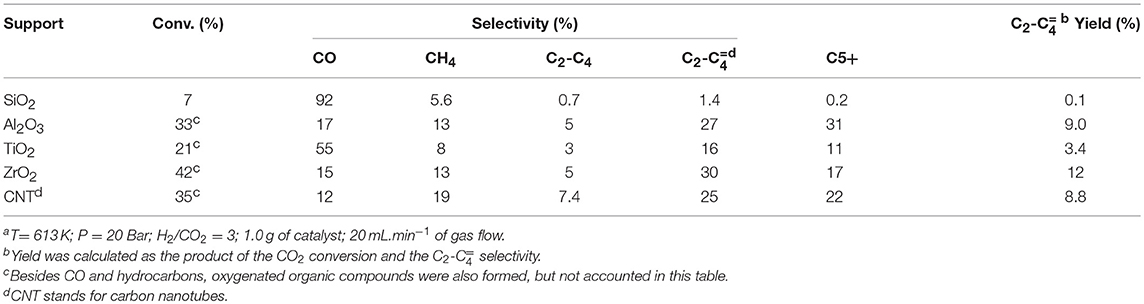
Table 5. Catalytic performance of Fe(10 wt%)–K(1 wt%) supported catalyst on the CO2 hydrogenation to hydrocarbonsa (Wang et al., 2013).
The Fe-Cr-K/Nb2O5 catalyst showed 5.0 % yield of light olefins at 673 K and 1.0 bar, with 20% CO2 conversion. The difference in temperature and pressure does not allow a fair comparison; in addition, the space velocity used in this studied was three times faster than the space velocity used by Wang et al. Our results indicate that Nb2O5 may have a potential to be exploited as support in the hydrogenation of CO2 to hydrocarbons, showing better selectivity to light olefins than SiO2 and TiO2, which are traditional supports of Fischer-Tropsch synthesis. On the other hands, our results indicate that a proper metal composition should still be pursued, in order for Nb2O5 competitive as support of catalysts for CO2 hydrogenation.
Comparison of the Fe-Cr-K/Nb2O5 catalyst with Rh/Nb2O5 used by Nozaki et al. (1987) for the CO2 hydrogenation at 623 K indicated that our catalyst was less selective to methane and slightly more selective to C2+, although the authors did not report the results in terms of olefin formation. This is because iron could better contribute to chain growth than rhodium. Nevertheless, in a general trend, the iron-based catalysts that we studied were less selective to hydrocarbons, producing significantly more CO.
The maximum yield of light olefins, 5%, was observed over the Fe-Cr-K/Nb2O5 catalyst at 673 K. Although the catalyst promoted with Cs showed a slightly higher selectivity to olefins at this temperature, the significantly lower CO2 conversion led to a yield of only 1.6%. The Fe-Cr-Cs/Nb2O5 catalyst formulation showed the highest yield of olefins, 2.4%, at 723 K, but with considerable formation of CO and methane. Fe-Cr/Nb2O5 showed the maximum yield of olefin, 4.6%, also at 723 K, but together with significant formation of CO and methane. The catalyst supported on SiO2 also showed the highest yield of olefins, 2.6%, at 723 K. Thus, it becomes clear that Nb2O5 has a beneficial effect as support on the performance of K-promoted iron catalyst, concerning the production of light olefins in FTS.
Conclusions
The hydrogenation of CO2 to light olefins was studied over Fe-Cr catalysts, promoted with K or Cs, and supported on calcined Nb2O5. A Fe-Cr catalyst promoted with K and supported on SiO2 was also prepared for comparison purposes.
The catalysts supported on Nb2O5 showed significantly lower surface areas than the catalyst supported on SiO2. Nevertheless, the Fe-Cr catalyst, promoted with K and supported on niobium oxide was more active and selective to olefins than the catalyst of same composition but supported on silica. At 673 K, the selectivity to light olefins was 25% over Fe-Cr-K/Nb2O5 and 26% on Fe-Cr-Cs/Nb2O5, but the later showed a considerably lower conversion. Therefore, the yield of olefins was 5 and 1.6% for both catalyst, respectively, at this temperature.
Alkali metal promotion increases the selectivity of olefins, similarly to what has been observed in FTS with CO, probably due to electron-donor effects. Cs promotion led to lower CO2 conversion, probably because of the higher basicity.
The results indicate the potential of the Nb2O5 in the formulation of catalysts for CO2 hydrogenation to hydrocarbons, especially light olefins. Compared with traditional SiO2, niobium oxide support gives higher activity and selectivity to olefins in CO2 hydrogenation, probably due to strong metal-support interactions.
Author Contributions
IdS was a Ph.D. student working in the project of CO2 hydrogenation to hydrocarbons. CM was the advisor. Both authors contributed to the writing of the paper.
Funding
Faperj–cientista do nosso estado–Proc. E-26/202.928/2015 CNPq–Proc. 442797/2014–Edital Universal–Catalisadores para a conversõo de dióxido de carbono (CO2).
Conflict of Interest Statement
The authors declare that the research was conducted in the absence of any commercial or financial relationships that could be construed as a potential conflict of interest.
Acknowledgments
The authors gratefully acknowledge financial support from Capes, Faperj, and CNPq. The laboratory of X-Ray Diffraction of the Institute of Chemistry is also acknowledged.
References
Amghizar, I., Vandewalle, L. A., Van Geem, K. M., and Marin, G. B. (2017). New trends in olefin production. Engineering 3, 171–178. doi: 10.1016/J.ENG.2017.02.006
Arakawa, H., and Bell, A. T. (1983). Effects of potassium promotion on the activity and selectivity of iron fischer-tropsch catalysts. Indus. Eng. Chem. Process Design Dev. 22, 97–103. doi: 10.1021/i200020a017
Aresta, M., and Dibenedetto, A. (2002). Carbon dioxide as building block for the synthesis of organic carbonates. J. Mol. Catal. A. 182–183, 399–409. doi: 10.1016/S1381-1169(01)00514-3
Aresta, M., Dibenedetto, A., and Angelini, A. (2013). The changing paradigm in CO2 utilization. J. CO2 Utilization 3–4, 65–73. doi: 10.1016/j.jcou.2013.08.001
Aresta, M., Dibenedetto, A., Angelini, A., and Pápai, I. (2015). Reaction mechanisms in the direct carboxylation of alcohols for the synthesis of acyclic carbonates. Topics Catal. 58, 2–14. doi: 10.1007/s11244-014-0342-0
Cagnoli, M. V., Alvarez, A. M., Gallegos, N. G., Bengoa, J. F., de Souza, C. D. D., Schmal, M., et al. (2007). Mössbauer and XPS spectroscopies studies of SMSI effect on Fe/Nb2O5catalysts for the fischer-tropsch synthesis. Appl. Catal. A Gen. 326, 113–119. doi: 10.1016/j.apcata.2007.04.005
Centi, G., Iaquaniello, G., and Perathoner, S. (2011). Can we afford to waste carbon dioxide? Carbon dioxide as a valuable source of carbon for the production of light olefins. Chem. Sus. Chem. 4, 1265–1273. doi: 10.1002/cssc.201100313
Centi, G., Quadrelli, E. A., and Perathoner, S. (2013). Catalysis for CO2 conversion: a key technology for rapid introduction of renewable energy in the value chain of chemical industries. Energy Environ. Sci. 6, 1711. doi: 10.1039/c3ee00056g
Cheng, Y., Lin, J., Wu, T., Wang, H., Xie, S., Pei, Y., et al. (2017). Mg and K dual-decorated Fe-on-reduced graphene oxide for selective catalyzing CO hydrogenation to light olefins with mitigated CO2emission and enhanced activity. Appl. Catalys. B Environ. 204, 475–485. doi: 10.1016/j.apcatb.2016.11.058
Choi, P. H., Jun, K. W., Lee, S. J., Choi, M. J., and Lee, K. W. (1996). Hydrogenation of carbon dioxide over alumina supported Fe-K catalysts. Catal. Lett. 40, 115–118. doi: 10.1007/BF00807467
Dama, S., Ghodke, S. R., Bobade, R., Gurav, H. R., and Chilukuri, S. (2018). Active and durable alkaline earth metal substituted perovskite catalysts for dry reforming of methane. Appl. Catal. B Environ. 224, 146–158. doi: 10.1016/j.apcatb.2017.10.048
Den Otter, J. H., and De Jong, K. P. (2014). Highly selective and active niobia-supported cobalt catalysts for fischer-tropsch synthesis. Topics Catal. 57, 445–450. doi: 10.1007/s11244-013-0200-5
Ding, F., Zhang, A., Liu, M., Zuo, Y., Li, K., Guo, X., et al. (2014). CO2 hydrogenation to hydrocarbons over iron-based catalyst: effects of physicochemical properties of Al2O3 supports. Indus. Eng. Chem. Res. 53, 17563–17569. doi: 10.1021/ie5031166
Dutta, A., Farooq, S., Karimi, I. A., and Khan, S. A. (2017). Assessing the potential of CO2 utilization with an integrated framework for producing power and chemicals. J. CO2 Utilization 19, 49–57. doi: 10.1016/j.jcou.2017.03.005
Eleutério, A., Santos, J. F., Passos, F. B., Aranda, D. A. G., and Schmal, M. (1998). The effect of preparation method on Pt/Nb2O5 catalysts. Braz. J. Chem. Eng. 15. doi: 10.1590/S0104-66321998000200014
Eslava, J. L., Sun, X., Gascon, J., Kapteijn, F., and Rodríguez-Ramos, I. (2017). Ruthenium particle size and cesium promotion effects in Fischer-Tropsch synthesis over high-surface-area graphite supported catalysts. Catal. Sci, Technol. 7, 1235–1244. doi: 10.1039/c6cy02535h
Hernández Mejía, C., den Otter, J. H., Weber, J. L., and de Jong, K. P. (2017). Crystalline niobia with tailored porosity as support for cobalt catalysts for the Fischer–Tropsch synthesis. Appl. Catal. A Gen. 548, 143–149. doi: 10.1016/j.apcata.2017.07.016
Koytsoumpa, E. I., Bergins, C., and Kakaras, E. (2018). The CO2 economy: review of CO2 capture and reuse technologies. J. Supercritical Fluids 132, 3–16. doi: 10.1016/j.supflu.2017.07.029
Leung, D. Y. C., Caramanna, G., and Maroto-Valer, M. M. (2014). An overview of current status of carbon dioxide capture and storage technologies. Renew. Sustain. Energy Rev. 39, 426–443. doi: 10.1016/j.rser.2014.07.093
Li, B., Duan, Y., Luebke, D., and Morreale, B. (2013). Advances in CO2 capture technology: a patent review. Appl. Energy 102, 1439–1447. doi: 10.1016/j.apenergy.2012.09.009
Lohitharn, N., Goodwin, J. G., and Lotero, E. (2008). Fe-based Fischer-Tropsch synthesis catalysts containing carbide-forming transition metal promoters. J. Catal. 255, 104–113. doi: 10.1016/j.jcat.2008.01.026
Mendes, F. M. T., Perez, C. A. C., Noronha, F. B., Souza, C. D. D., Cesar, D. V., Freund, H. J., et al. (2006a). Fischer–tropsch synthesis on anchored Co/Nb2O5/Al2O3 catalysts: the nature of the surface and the effect on chain growth. J. Phys. Chem. B 110, 9155–9163. doi: 10.1021/jp060175g
Mendes, F. M. T., Uhl, A., Starr, D. E., Guimond, S., Schmal, M., Kuhlenbeck, H., et al. (2006b). Strong metal support interaction on Co/niobia model catalysts. Catal. Lett. 111, 35–41. doi: 10.1007/s10562-006-0127-6
Mohsenzadeh, A., Zamani, A., and Taherzadeh, M. J. (2017). Bioethylene production from ethanol: a review and techno-economical evaluation. Chem. Bio. Eng. Rev. 4, 75–91. doi: 10.1002/cben.201600025
Monastersky, R. (2013). Global carbon dioxide levels near worrisome milestone. Nature 497, 13–14. doi: 10.1038/497013a
Ngantsoue-Hoc, W., Zhang, Y., Oandapos Brien, R. J., Luo, M., and Davis, B. H. (2002). Fischer-tropsch synthesis: activity and selectivity for group I alkali promoted iron-based catalysts. Appl. Catal. A Gen. 236, 77–89. doi: 10.1016/S0926-860X(02)00278-8
Ning, W., Li, B., Wang, B., Yang, X., and Jin, Y. (2018). Enhanced production of -C5+ Hydrocarbons from -CO2 hydrogenation by the synergistic effects of Pd and K on γ-Fe 2O3 catalyst. Catal. Lett. 149, 431–440. doi: 10.1007/s10562-018-2622-y
Noronha, F. B., Aranda, D. A. G., Ordine, A. P., and Schmal, M. (2000). The promoting effect of Nb2O5 addition to Pd/Al2O3 catalysts on propane oxidation. 57, 275–282. doi: 10.1016/S0920-5861(99)00337-5
North, M., Pasquale, R., and Young, C. (2010). Synthesis of cyclic carbonates from epoxides and CO2. Green Chem. 12, 1514–1539. doi: 10.1039/c0gc00065e
Nowak, I., and Ziolek, M. (1999). Niobium compounds: preparation, characterization, and application in heterogeneous catalysis. Chem. Rev. 99, 3603–3624. doi: 10.1021/cr9800208
Nozaki, F., Sodesawa, T., and Satoh, S. (1987). Hydrogenation of carbon dioxide into light hydrocarbons at atmospheric pressure over Rh/Nb205 or Cu/Si02-Rh/Nb205 catalyst. J. Catal. 346, 339–346.
Ozorio, L. P., and Mota, C. J. A. (2017). Direct carbonation of glycerol with CO2 catalyzed by metal oxides. ChemPhysChem 18, 3260–3265. doi: 10.1002/cphc.201700579
Pérez-Fortes, M., Schöneberger, J. C., Boulamanti, A., and Tzimas, E. (2016). Methanol synthesis using captured CO2 as raw material: techno-economic and environmental assessment. Appl. Energy 161, 718–732. doi: 10.1016/j.apenergy.2015.07.067
Raje, A. P., O'Brien, R. J., and Davis, B. H. (1998). Effect of potassium promotion on iron-based catalysts for Fischer-Tropsch synthesis. J. Catal. 180, 36–43. doi: 10.1006/jcat.1998.2259
Ren, T., Patel, M., and Blok, K. (2006). Olefins from conventional and heavy feedstocks: energy use in steam cracking and alternative processes. Energy 31, 425–451. doi: 10.1016/j.energy.2005.04.001
Rezende, C. C., Neto, J. L., Silva, A. C., Lima, V. M., Pereira, M. C., and Oliveira, L. C. A. (2012). Synthesis and characterization of iron / niobium composites : catalyst for dye wastewater treatments. CATCOM 26, 209–213. doi: 10.1016/j.catcom.2012.06.006
Saeidi, S., Amin, N. A. S., and Rahimpour, M. R. (2014). Hydrogenation of CO2 to value-added products—a review and potential future developments. J. CO2 Util. 5, 66–81. doi: 10.1016/j.jcou.2013.12.005
Sakakura, T., and Kohno, K. (2009). The synthesis of organic carbonates from carbon dioxide. Chem. Commun. 11, 1312–1330. doi: 10.1039/b819997c
Satthawong, R., Koizumi, N., Song, C., and Prasassarakich, P. (2015). Light olefin synthesis from CO2 hydrogenation over K-promoted Fe-Co bimetallic catalysts. Catal. Today 251, 34–40. doi: 10.1016/j.cattod.2015.01.011
Schmal, M., and Freund, H. J. (2009). Towards an atomic level understanding of niobia based catalysts and catalysis by combining the science of catalysis with surface science. Anais Acad. Brasil. Ciencias 81, 297–318. doi: 10.1590/S0001-37652009000200016
Shi, Z., Yang, H., Gao, P., Chen, X., Liu, H., and Zhong, L. (2018). Effect of alkali metals on the performance of CoCu/TiO2 catalysts for CO2 hydrogenation to long—chain hydrocarbons. Chin. J. Catal. 39, 1294–1302. doi: 10.1016/S1872-2067(18)63086-4
Soares, R. R., Frydman, A., and Schmal, M. (1993). Effect of preparation method on 5% Co/Nb2O5 in fischer-tropsch syntesis (FTS). Catal. Today 16, 361–370. doi: 10.1016/0920-5861(93)80075-C
Song, C. (2006). Global challenges and strategies for control, conversion and utilization of CO2for sustainable development involving energy, catalysis, adsorption and chemical processing. Catal. Today 115, 2–32. doi: 10.1016/j.cattod.2006.02.029
Tian, P., Wei, Y., Ye, M., and Liu, Z. (2015). Methanol to olefins (MTO): from fundamentals to commercialization. ACS Catal. 5, 1922–1938. doi: 10.1021/acscatal.5b00007
Visconti, C. G., Martinelli, M., Falbo, L., Fratalocchi, L., and Lietti, L. (2016). CO2 hydrogenation to hydrocarbons over Co and Fe-based Fischer-Tropsch catalysts. Catal. Today 277, 161–170. doi: 10.1016/j.cattod.2016.04.010
Wang, J., You, Z., Zhang, Q., Deng, W., and Wang, Y. (2013). Synthesis of lower olefins by hydrogenation of carbon dioxide over supported iron catalysts. Catal. Today 215, 186–193. doi: 10.1016/j.cattod.2013.03.031
Wang, W., Wang, S., Ma, X., and Gong, J. (2011). Recent advances in catalytic hydrogenation of carbon dioxide. Chem. Soc. Rev. 40, 3703–3727. doi: 10.1039/c1cs15008a
Yang, H., Zhang, C., Gao, P., Wang, H., Li, X., Zhong, L., et al. (2017). A review of the catalytic hydrogenation of carbon dioxide into value-added hydrocarbons. Catal. Sci. Technol. 7, 4580–4598. doi: 10.1039/c7cy01403a
Keywords: CO2, hydrocarbons, olefins, hydrogenation, niobium oxide
Citation: da Silva IA and Mota CJA (2019) Conversion of CO2 to Light Olefins Over Iron-Based Catalysts Supported on Niobium Oxide. Front. Energy Res. 7:49. doi: 10.3389/fenrg.2019.00049
Received: 11 January 2019; Accepted: 07 May 2019;
Published: 28 May 2019.
Edited by:
Peter Styring, University of Sheffield, United KingdomReviewed by:
M. Hassan Beyzavi, University of Arkansas, United StatesWei Liu, Molecule Works Inc., United States
Copyright © 2019 da Silva and Mota. This is an open-access article distributed under the terms of the Creative Commons Attribution License (CC BY). The use, distribution or reproduction in other forums is permitted, provided the original author(s) and the copyright owner(s) are credited and that the original publication in this journal is cited, in accordance with accepted academic practice. No use, distribution or reproduction is permitted which does not comply with these terms.
*Correspondence: Claudio J. A. Mota, cmota@iq.ufrj.br