- 1Department of Chemical Engineering, University of Birmingham, Birmingham, United Kingdom
- 2Department of Chemistry, Government Sadiq College Women University, Bahawalpur, Pakistan
- 3Fraunhofer Institute for Environmental, Safety, and Energy Technology UMSICHT, Sulzbach Rosenberg, Germany
Being a developing economy, Pakistan is facing a severe energy crisis that limits its economic development. Pakistan relies heavily on energy resources like natural gas, oil, hydropower, nuclear, coal and liquefied petroleum gas (LPG) which contribute as 48.3, 32.1, 11.3, 7.6, and 0.6% of the primary energy supply, respectively. Because of the rapidly growing population and economy, Pakistan's energy needs are huge; to accomplish these energy necessities, Pakistan is continually spending $7–9 billion on the import of fossil fuels. To resolve the serious issues of energy availability, the Alternative Energy Development Board (AEDB) of Pakistan is currently exploring the development of renewable energy technologies in Pakistan that will be beneficial for the developing economy so that Pakistan might be able to minimize the growing energy crisis. Out of all the renewable energy resources, biomass is considered the best and most easily accessible source of energy with its unique environmentally friendly nature, constant supply, wider availability, and ease of integration into existing infrastructure. Despite the presence of an abundance of biomass energy resources, there is still a need for work on the use of these sources to produce energy. This literature review explores the availability of biomass resources in Pakistan and their potential for addressing rapidly growing energy demand in the country, which can assist in the stabilization of a Pakistan's energy demand for challenged economic development.
Introduction
The current population of Pakistan is 198,139,348 (198 million) with an annual growth rate of 1.97% based on the most recent United Nations approximations.1 Within various sectors of, such as domestic consumption, commercial consumption, agriculture, public lighting and bulk supply, the expenditure of energy is 45.7, 7.5, 28.1, 11.8, 0.5, and 6.4%, respectively (Aziz, 2013). Agriculture is the second largest sector of Pakistan's economy, comprising five subsectors with major crops, minor crops, livestock, fisheries, and forestry having a collective share of 21% of the total Gross Domestic Product (GDP) (Raza et al., 2012; Chandio et al., 2016). Major crops, minor crops, livestock, and forestry contribute 5.3, 2.3, 11.8, and 0.4%, respectively, to the total GDP (Chandio et al., 2016). Agriculture is the backbone of Pakistan's economy as most of the exports from Pakistan are based on agricultural products including rice, cotton, wheat, sugarcane, and many other major and minor products (Faridi, 2012). Presently, Pakistan is facing a severe and unmanageable energy crisis that is hindering socio-economic development (Mirza et al., 2008a; Aziz, 2013).
The Energy Situation in Pakistan
The industrial and agricultural sectors are the backbones of the economy, and the progress of the economy is directly linked to the constant availability of energy resources (Abbas, 2015). The main hindrance to the economic development of Pakistan is its poor division of risky energy resources. The power sector of Pakistan remains unsuccessful in overcoming the energy-related issues despite being rich in coal resources (Arif, 2011). Michael Kugelman (Javed et al., 2016) acknowledged in his remarks on Pakistan's energy situation in the National Bureau of Asian Research of America, “Pakistan is mired in an acute energy crisis, one with immense implications for both the nation's floundering economy and its volatile security situation.” At periods of high demand in Pakistan, the electricity supply gap is ~6,000 MW, which accounts for 33–35% of the total expenditures (Abbas, 2015; Saeed et al., 2015). Pakistan has more than 30% of its population (55 million) with no access to electricity (Harijan et al., 2008; Saeed et al., 2015). In urban and rural areas the duration of power outages is 6–12 and 12–18 h per day, respectively (Saeed et al., 2015).2 Pakistan relies heavily on energy resources like natural gas, oil, hydro, nuclear, coal, and liquefied petroleum gas (LPG), each of which contributes 48.3, 32.1, 11.3, 7.6, and 0.6%, respectively, to the primary energy supply (Rehman et al., 2013). According to recent statistics by AEDB, the share of various resources of energy, along with a contribution of 2% from renewable energy, resources involve natural gas (21%), hydropower (31%), Oil (39%), LNG (4%), and nuclear power (3%).2 Moreover, relevant figures for the energy mix have also been supported by the Hydrocarbon Development Institute of Pakistan (HDIP). During 2013, dependence on various sources made up an 80.8% share of natural resources [oil (30.8%), gas (49.5%), coal (87.4%), and liquefied petroleum gas (0.5%)], with a 12.5% share coming from the remaining sources [hydro-power (10.5%), nuclear electricity (1.9%) and imported electricity (0.1%)] (Shaikh et al., 2015). Widening power supply and demand gaps from 1980 to 2012 are shown in Table 1 (Mahmood and Ayaz, 2018).3 Kessides (2013) explored the entire energy scenario of Pakistan, developing a projection of the future stating that electricity outages are expected to increase to 13,000 MW by 2020 (Figure 1).
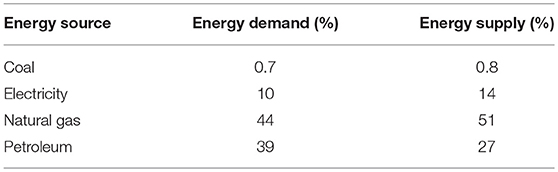
Table 1. Energy Demand and Supply Share in Pakistan for the period 1980–20123.
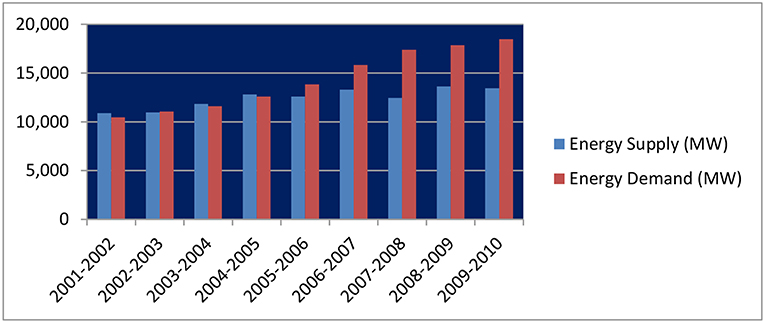
Figure 1. Energy scenario of Pakistan with the supply and demand gap (Kessides, 2013; Khan et al., 2014).
Effects of Energy Crisis
The economic development of any country relies on energy availability as energy is the engine of the economy (Lee and Chang, 2008; Sadorsky, 2010; Kakar et al., 2011). Economical productivity exhibits an increase concomitant with that of the increase in available energy resources. Likewise, energy crisis leads to serious impacts on the economy of a country through its adverse impacts on employment, marketing, and poverty (Cleveland et al., 1984; Kessides, 2013). Because of the heavy shortfall in energy production vs. demand, not only domestic but also various dimensions of the industrial sectors are also badly affected (Khan and Ahmad, 2008; Aziz, 2013; Saeed et al., 2015). Sixty to seventy percent (60–70%) of industrial investors have shifted their businesses to other countries, such as China, India, and Bangladesh, which is attributed to the increase in the energy shortfall, which reaches 40% (Asif, 2009; Saeed et al., 2015). In this period of industrialization and globalization, increasing energy demand cannot be accompanied by only depending on natural resources. Since the mid-1970s, natural energy resources have been running low and considerable efforts have been made to produce energy through renewable energy technologies (Mohan et al., 2006). Not only is the industrial sector affected, but agricultural productivity is also adversely affected by the rapid decrease in affordable energy availability, as the proper functioning of all the tools for agriculture—i.e., for transportation, pesticides and irrigation—are based on the application of energy (Pimentel et al., 1973). Pakistan is not only facing this severe electricity crisis, but a shortage of petroleum products and water scarcity have also become serious issues (Khan et al., 2012). Kessides (2013) in his recent publication described the severe energy crisis in Pakistan. He related severe energy issues with the lack of proper forecasting and planning together with the increasing domestic necessity. Severe blackouts in Pakistan are pushing the country toward concerning levels of poverty and create a negative impact on employment, global competitiveness and exports. The effects of energy disruption and electrical failures are so intensive that they declined the growth rate of the economy by 2% of the original figure of 6.5% per annum (GOP, 2013; Komal and Abbas, 2015). Kugelman (2013) stated that the unavailability of indispensable energy to the various economical sectors has resulted in an annual decline of up to 4% in the total GDP. Siddiqui et al. (2008) reported a survey describing the impacts of power crisis on the employment, production cost, and supply orders. Employment demand for labor in the industrial sector of Pakistan is adversely impacted. The resulting unemployment and labor loss per day varies from industry to industry and the results for different industries are shown in Figure 2.
Energy or Electricity Theft in Pakistan
Of all the electricity generated every year in Pakistan, a large segment is either stolen or lost in the distribution process. Besides the transmission losses and electricity theft, a significant fraction of the electricity consumed remains unpaid for. Islamabad Electricity Supply Company (IESCO), Lahore Electricity Supply Company (LESCO), Gujranwala Electric Power Company (GEPCO), Faisalabad Electricity Supply Company (FESCO), Multan Electric Power Company (MEPCO), Peshawar Electricity Supply Company (PESCO), Quetta Electricity Supply Company (QESCO), Hyderabad Electricity Supply Company (HESCO), and Karachi Electric Supply Company (KESC) are the major power distribution companies in Pakistan (Jamil and Ahmad, 2014). The collective transmission loss of all the distributing companies was reported to be 20.4% by 2010, with the average loss exceeding 20–25% per year. Jamil and Ahmad (2014) submitted a detailed analysis of electricity theft and transmission losses throughout the country. During 201–13, the recorded transmission losses for the IESCO, PESCO, and KESC were 9.5, 36, and 40% respectively. They discovered that 17.8 million consumers were supplied with 92,480 GWh, and ~18,919 GWh remained unpaid for in 2010. The illegal consumption and stealing of electrical power can be prevented by the implementation of strict laws of accountability, strategies and action plans alongside the establishment of good political governance.
Effects of Gas Shortage
In the previous decade, Pakistan was self-reliant in natural gas. However, currently, widespread utilization of natural gas in industry, domestic affairs, and transportation has led to a gas shortage in Pakistan. During 2001–2008, a significant elevation of 10% yearly in gas use was reported. Natural gas provides 31.9% of the overall national energy expenditure and 29% of electricity production (Awan and Rashid, 2012). The Ministry of Petroleum and Natural Resources reported that the growth rate of production of natural gas increased by 2.6%, which occurred alongside a growth rate of 4.4% in the consumption of gas. The consumption of natural gas in various sectors of Pakistan is shown in Figure 3 (Dawood et al., 2013). The textile industry of Pakistan is one of the biggest industries of the country, covering about 60% of total exports and having a net worth of $9 billion. This textile industry is also adversely affected by the gas crisis (Khan and Khan, 2010).
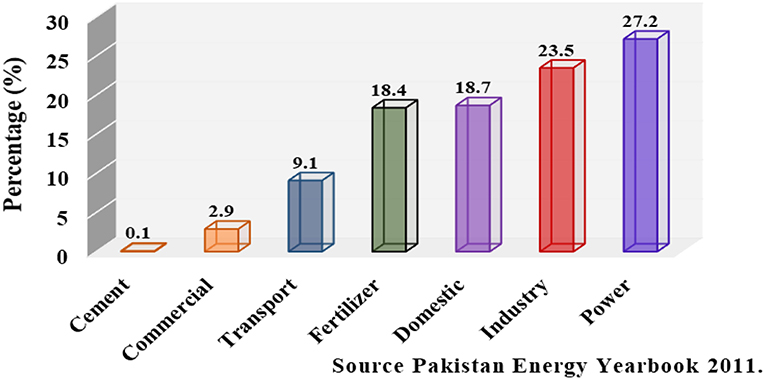
Figure 3. Sector-based division of the consumption of natural gas in Pakistan (Dawood et al., 2013).
Effects of Oil-Shortage
The International Energy Agency has reported that the rise of oil consumption, on average, will be from 85 million barrels to 113 million barrels by 2030 (Howden, 2007). In Pakistan, the energy yearbook describes a 35.5% increase in the share of oil consumption, which surely can be a reason for oil depletion (Figure 4).4 In Pakistan, oil utilization is equivalent to 490 kilograms, with the commercial share of oil in the production of electricity being 19% (Husain, 2010; Javaid et al., 2011). There is a huge gap between oil production and consumption, with production equaling 64,000 barrels per day and consumption surpassing 351,400 barrels per day (Sahir and Qureshi, 2008; Zuberi et al., 2013). The consumption of oil and gas has grown so fast that the remaining reserves are reported to last for 19 and 10 years respectively (Muneer et al., 2006; Asif, 2009). The depletion of natural gas and oil reserves not only affects agriculture and transport, but a reduction in industrial productivity has also been noticed (Asif, 2009). The majority of the natural oil reserves of Pakistan have been consumed, and due to this reason Pakistan is spent $6.7 billion on oil imports during 2005–06 with an increase in growth rate of 1% per year (Ashraf Chaudhry et al., 2009; Awan and Rashid, 2012).5 Currently, studied sources of renewable energy in Pakistan are solar, wind, hydro and biomass. A great number of industries in Pakistan are at present dependent on liquid fuels to fulfill their electricity and heating requirements (Saeed et al., 2015). Unfortunately, accessing universal energy is also a large concern in the country. More than 20,920 villages in the country are so far to be electrified.5 This energy dilemma is further exacerbated because there is no connection to the national electricity grid for some of these remote villages. Thus, decentralized electricity provision is the only cost-effective solution for them.
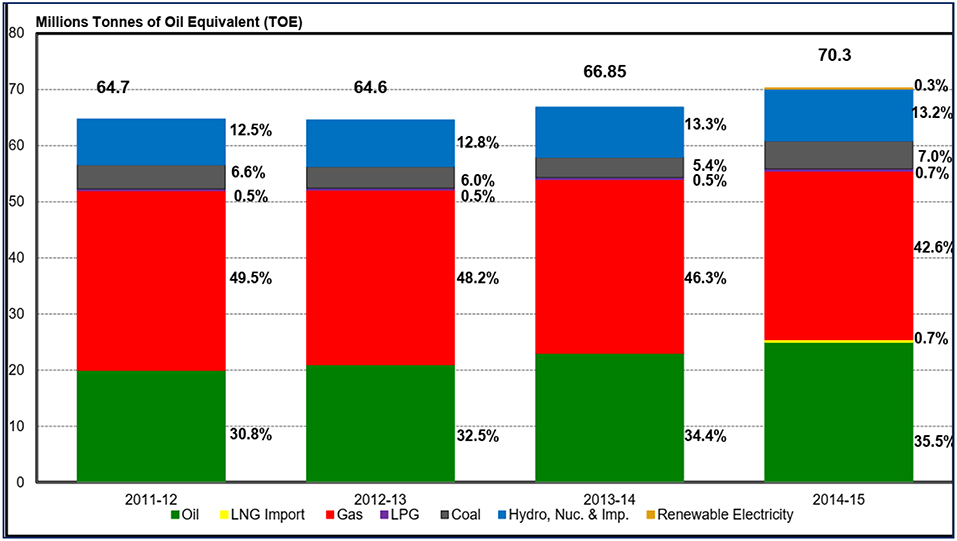
Figure 4. Primary energy supplies by source4.
Reasons for Critical Power Outages
Continuous power interruptions negatively affect economic activities. The obvious reduction in Pakistan's economic productivity is due to power cuts that exceeded 8,000 MW until 2012. Experts analyzed various aspects of the critical power situation in Pakistan and provided reasons for these drastic shortfalls; one of these is that the relevant organizations and institutes have failed to enhance electricity generation and capacity according to supply demands, which leads to drastic energy deficits. Other major reasons include the decline in natural energy reserves, severe water shortfalls, rapidly increasing demand, excessive dependency on imported petroleum products, circular debt and other significant political and governance issues (Malik, 2012; Munir and Khalid, 2012; Nawaz et al., 2013; Rashid Amjad, 2015). Mismanagement and poor administration are also significant factors behind energy issues due to nepotism, corruption, incompetent generation or limited capacity addition, inefficient and outdated transmission systems, financial malefaction, non-optimal tariffs and reliance on expensive fuels (Malik, 2012; Nawaz et al., 2013; Ullah, 2013; Ahmed et al., 2015; Rashid Amjad, 2015). In general, the unskilled and undereducated political leaders and policymakers, low investments by the government, lacking proper vision and accountability, and instability of governance have all led to the power crisis in the country. Delays in payments by the government to the relevant power generation agencies is another huge issue that has lead to the growth of this crisis (Malik, 2012; Munir and Khalid, 2012; Kugelman, 2013; Rashid Amjad, 2015).
The Policy for Energy Production
Efficient policies and proper planning can prevent severe power outages and expanding crisis. Pakistan needs good and efficient governance for broad-based planning to overcome the energy shortfalls. In the designing of reliable policies, many factors are taken into consideration, such as valid and reliable forecast for future demand on the basis of past trends, appropriate development and assessment of technologies, energy conservation, improved policies of long-term and short-term planning to compensate for the energy demand and effective project management (Mathur, 2001; Chikkatur et al., 2009; Østergaard and Sperling, 2014). There are numerous concerns for policy-makers in the formation and management of long-term reliable energy policies, such as energy security, climate change and natural fuel depletion (Turton and Barreto, 2006; Østergaard and Sperling, 2014; Overland, 2016). Energy security refers to the availability of affordable energy without interruptions, which is crucial for economic development (Turton and Barreto, 2006; Hogan et al., 2007).
Reliable energy policy and planning take into account the following important factors, such as the following (Mathur, 2001; Qureshi, 2009; Balat, 2010; Valasai et al., 2017):
• Focusing on renewable energy sources
• Focusing on the cost-effective generation of electricity
• Increasing resources to overcome energy deficits
• Conservation of energy
• Reducing the energy vs. supply demand gaps
• Improving the energy infrastructure to reduce the system losses
Various past governments of Pakistan have also put forward a number of different policies which were reshaped occasionally, including the national energy policies of 1994, 1995, 1998, 2002, 2005, 2006, 2010–2012, and 2013 (Table 2) (Munir and Khalid, 2012; Dawood et al., 2013; Abbas, 2015; Valasai et al., 2017).
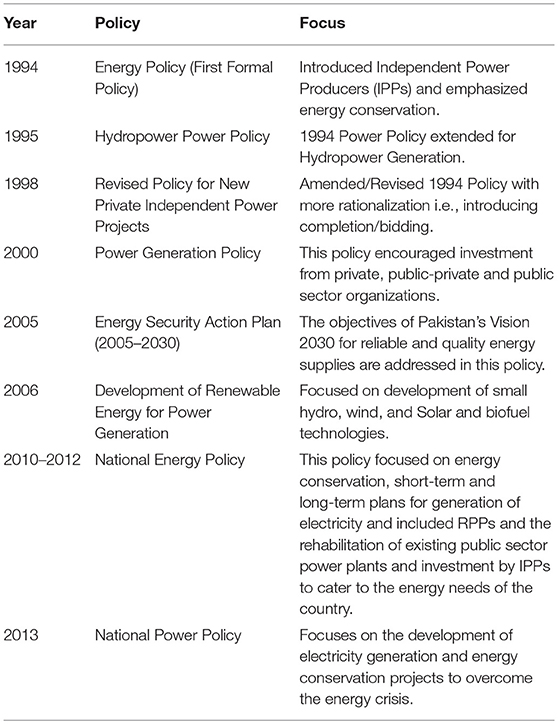
Table 2. National energy policies of Pakistan with their major focus (Abbas, 2015; Valasai et al., 2017).
For bridging the supply and demand gap, the Government of Pakistan (GOP) has recognized alternative and renewable energy (ARE) sources as one of the best preference (Aziz, 2013; Saeed et al., 2015). Pakistan had made a strategy to add a minimum of 9.7 GW of renewable energy by 2030 in the national energy mix. The industrial sector contributes about 40% of the country's GDP. But owing to the deficiency in energy, a large number of these industrial units are dependent on expensive and polluting diesel generation units to meet their energy demand.5 Various industries, such as rice mills, wood processing mills, brick kilns, cement kilns, and metal processing are heavily dependent on heat energy. They frequently burn fossil fuels and some biomass to sustain their energy needs.5 So now, Pakistan is in dire need of advanced conversion technologies, such as biomass combustion, gasification and pyrolysis. Such technologies have been effectively installed in various countries around the globe (Mirza et al., 2008a; Saeed et al., 2015).5 The global daily expenditure of oil currently stands at 85 million barrels, which will be increased to 113 million barrels by 2030 (Howden, 2007). Energy resources that are used at present will not be able to cope with future energy necessities because Pakistan's fossil fuel reserves are diminishing rapidly and the demand of energy is expected to exceed 66,000 MW or 66 GW by 2030 (Howden, 2007; Zuberi et al., 2013). Modern society and economic development would not be realistic without energy; thus, a continuous decline in fossil fuels has forced the relevant agencies to plan alternative ways to produce sustainable energy (Qureshi, 2009; Valasai et al., 2017). The energy produced by renewable sources or inexhaustible natural resources is usually referred to as renewable or sustainable energy (Howden, 2007; Ashraf Chaudhry et al., 2009).2 Renewable energy sources including solar, wind, biomass and others are prevalent in Pakistan (Raja and Abro, 1994; Farooq and Kumar, 2013).2 As stated in a recent survey, the extent of power generation by renewable energy sources in Pakistan is accounted for by wind (340,000 MW), solar (2,900,000 MW), hydro (small/mini, 3,000 MW), and bagasse cogeneration (2,000 MW) (Syed et al., 2014).2
Renewable or Sustainable Energy Resources in Pakistan
Pakistan can resolve energy-related concerns by utilizing renewable energy resources rather than relying on conventional fossil energy resources (non-renewable). Sheikh (2010) described in his study that Pakistan pays 60% of the foreign exchange on the import of expensive fuels, which can be saved by diverting to renewable energy resources. The renewable energy resources of Pakistan are solar, wind, hydro, geothermal and biomass (Sheikh, 2009, 2010; Rafique and Rehman, 2017).
Wind-Power
The coastal area of Pakistan is spread over 1,050 km (250 km in Sindh and 800 km in Balochistan) from the Indian border in the east to the Iranian border in the west (Bhutto et al., 2013).6 According to an estimation of AEDB, Pakistan has the potential to produce 360 GW, but currently, Pakistan ranks 44th in generating wind power, with a total installed capacity of 106 MW (Bhutto et al., 2013; Khahro et al., 2014a). The estimated wind speed on the coastlines of Pakistan ranges between 5 and 7 m/s which leads to wind power generation at the coastal areas of Sindh and Balochistan (Karachi, Ormara, Jivani, Pasni, Baburband, Kati Bandar, Gharo, and many others), and wind power plants have been installed (Ahmed et al., 2006; Sheikh, 2009; Ullah and Chipperfield, 2010; Khahro et al., 2014a,b; Siddique and Wazir, 2016). Wind power plants with energy capacities of 4 KW and 20 KW have been installed at various locations on the coastline of Sindh and Balochistan (Ahmed et al., 2006). Ahmed et al. (2006) reported that the generators of 4 kW capacities work efficiently throughout the year, but there are only a few locations, such as Pasni and Jivani where the 20 KW plants can work because they need high wind speed for operation. The Pakistan Meteorological Department has reported the wind power potential of 45 different Pakistani locations on the coastline. The resulting measures are shown in Table 3 (Harijan, 2008; Harijan et al., 2009, 2011). Despite having considerable wind power generation capacity, regrettably the wind power share has no significant contribution to the total energy mix, but wind energy can be employed in the development of various rural and remote areas in Pakistan (Ahmed et al., 2006). Wind power generation can be enhanced by the formation and proper implementation of long-term productive policies, significant investment and proper vision (Mirza et al., 2007).
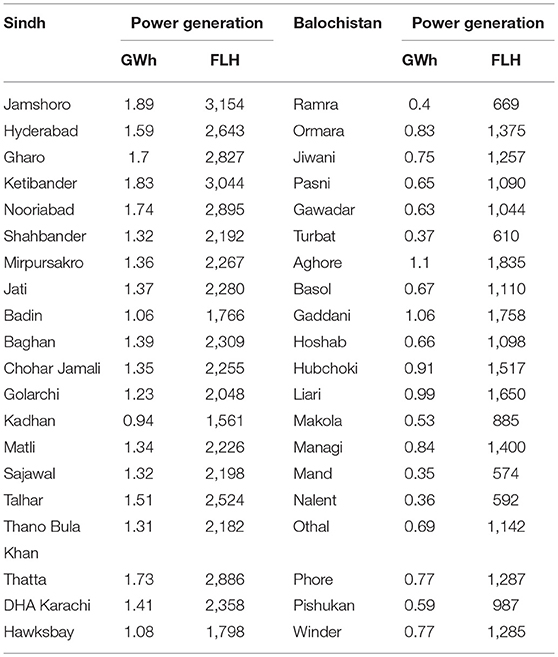
Table 3. Estimated wind power generation capacity of Pakistan (Harijan et al., 2009, 2011).
Solar-Power
Pakistan possesses exceptional solar potential, which is suitable for decentralized commercial utilization of energy. Therefore, solar energy could be the best option to balance the increasing supply and demand energy gap (Khalil and Zaidi, 2014). Solar rays occur on 95% of the surface of Pakistan, which is about 1,500–3,000 h per annum with 5–7 kWh/m2/day of annual average solar radiation, accounting for 200–250 watt/m2 or 6,840–8,280 MJ/m2/day (Mirza et al., 2003; Ashraf Chaudhry et al., 2009; Solangi et al., 2011; Harijan et al., 2015). According to the solar map of Pakistan issued by NREL and USAID, the province of Balochistan shares more than 5–7 kWh/m2/day of annual average global insolation, with an energy potential of 18–25 MJ/m2/day as the sun warms the surface of Balochistan for 6–8 h daily (Sheikh, 2009). Adnan et al. (2012) presented a report stating that solar irradiation intensity was observed to be varied, as the irradiation intensity exceeded 200 W/m from February–October in province of Sindh, March–October in Balochistan, April–September in the various regions of KPK, Gilgit-Baltistan, Azad Kashmir, and March–October in the areas of Punjab. Solar energy resources can be exploited in rural and urban areas for an assortment of applications in two major fields, including photovoltaic (PV) and solar thermal (for instance solar water heating, steam generation, solar cookers, solar dryers and solar desalination) (Mirza et al., 2003; Ashraf Chaudhry et al., 2009). Solangi et al. (2011) reviewed the complete profile of solar power units' installment in Pakistan. They stated that since the 1990s, Pakistan had established 18 photovoltaic projects with 440 kW capacities that afterward diminished due to the inadequacy of technical expertise and mismanagement. Other solar power units of photovoltaic and solar thermal were installed by the relevant companies, with <1,000 kW in photovoltaic and 10,000 kW solar thermal power, which is considerably less than the estimated potential of solar energy generation in Pakistan. There are many reasons behind the current regrettable solar energy scenario in Pakistan, for instance lack of managing and operating proficiency, appropriate policies, awareness in remote areas and lack of sufficient investment together with the extravagant PV technology (Sheikh, 2010; Solangi et al., 2011); (Khan and Pervaiz, 2013).
Hydropower
Hydropower, as the name suggests, is the form of energy that is interconnected with the force of flowing water (potential energy), which can be converted into electricity for diversified applications (Wagner and Mathur, 2011). Globally, hydro-power shares a great deal of about 20% contribution in the total electricity production (Boyle, 2004; Asif, 2009). Total water resources (such as rivers, glaciers, snow melt, etc.) of Pakistan have been estimated to be 400,000 Km2 with an anticipated potential of about 42 GW (Ashraf Chaudhry et al., 2009; Sheikh, 2009). Regardless of being rich in natural water resources, the contribution of hydropower to the total energy mix of the country is declining day after day due to many reasons (such as climate change, short-term policies, proper execution of project, land clearance, resettlement site development, long construction periods and many other financial and political issues). Until now, only 15% of the total hydropower potential, accounting for 6.5 GW, has been installed (Asif, 2009; Bhutto et al., 2012). The generation and exploitation of hydropower have reduced since the 1970s, from 70 to 33% in 2006. Various major hydropower projects are operational, for instance Tarbela, Mangla, Warsak, Chashma, Ghazi, and Barotha contributing about 6,355 MW (Mirza et al., 2008b). The major hydropower projects including Tarbela, Mangla, and Chashma have recently been reported as displaying declines in their storage capacity by 20%, yet alternate undertakings, for instance Ghazi and Barotha (introduced in 2004), are noteworthy contributors toward the complete hydropower capability of Pakistan (Asif, 2009; Bhutto et al., 2012). Hydropower development in Pakistan is shown in Figure 5.7 In the Indus basin of Pakistan, 800 potential sites have been recognized, with a hydroelectric power of 60 GW. Only the hydropower plants at 134 sites are operational, amounting to 11% (6,720 MW) of the total, but the remaining 89% of this project are under implementation and completely undeveloped (Siddiqi et al., 2012). Pakistan can boost the hydroelectric share of the nation's overall energy output by effectively working on the following major projects: Kalabagh, Bhasha, Bunji, Dasu, Kohala, Patan, Neelam-Jhelum, Thakot, Munda, and Ahori, with energy generation capacities of about 3,600–3,800, 4,500–4,600, 5,400, 3,800, 1,100, 2,800, 950, 2,800, 750, and 600 MW, respectively (Asif, 2009; Malik and Sukhera, 2012). Besides these major projects, there are many mini- and micro-hydropower sites in Pakistan, with an identified potential of <5%, which can be employed to harness <1,000 MW of hydroelectric power (Sheikh, 2009, 2010).
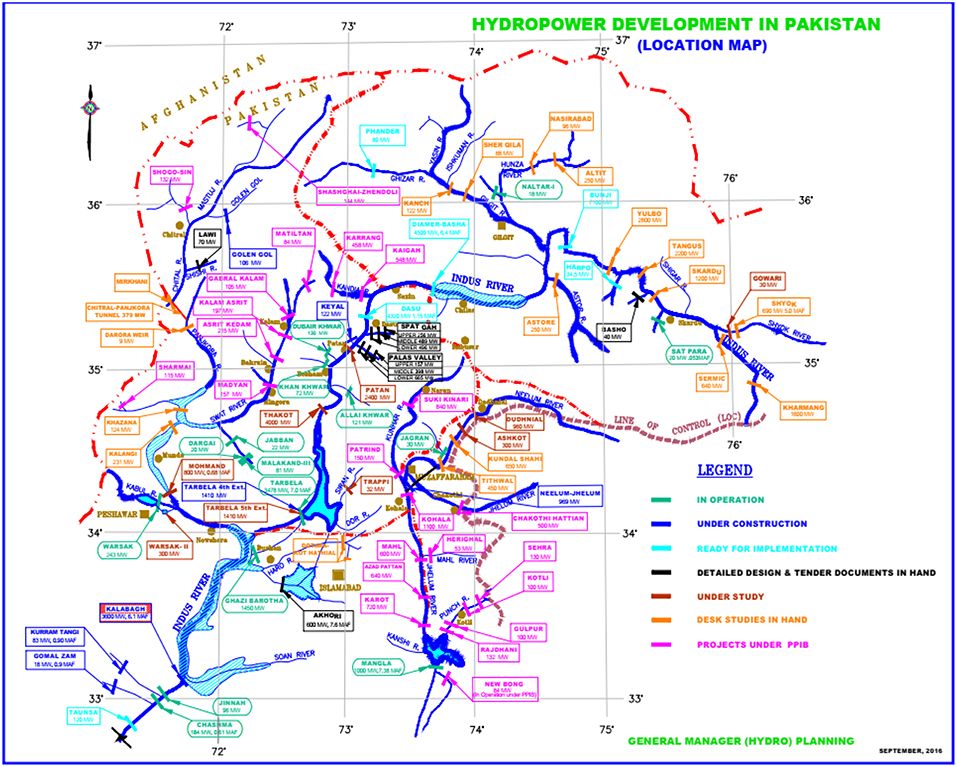
Figure 5. Hydroelectric power development in Pakistan7.
Geothermal
Geothermal energy is another form of clean, renewable energy that is obtained from the heat energy available on the earth. There are various sources of geothermal energy, such as hot springs, volcanoes, fumaroles, and geysers. Pakistan also has great potential for electricity production from geothermal energy. There are numerous hot springs with temperatures of 30–170°C in the regions of Balochistan, Sindh, Karachi, Azad Kashmir, and KPK that can be exploited for the generation of geothermal energy. Despite having plentiful geothermal resources, there is no substantial work or planning to produce usable energy from these resources in Pakistan (Sheikh, 2009, 2010; Awan and Rashid, 2012; Malik and Sukhera, 2012).
Energy From Biomass
Biomass energy has the potential to play a substantial role in combating the expanding energy crisis in Pakistan owing to the massive and diversified biomass potential of the country. Biomass is counted as a clean energy source since it recycles carbon dioxide through photosynthesis during biomass growth (Mirza et al., 2008a). Biomass can be used to produce electricity, thermal energy, and various chemicals. Zuberi et al. (2013) depicted the diverse advantageous aspects of the biomass resources in Pakistan including economic, environmental and employment benefits. Understanding the different properties of the biomass resources is crucial for the success of a biomass-to-energy project. The properties include calorific value, moisture content, ash content, carbon content, hydrogen content, cellulose, hemicellulose and lignin (McKendry, 2002a).
Indigenous Biomass Resources in Pakistan
Pakistan is gifted with biomass resources; however, its overall energy potential is yet to be scientifically determined. Biomass is not only limited to habitat and animal waste but also includes feedstocks, such as agricultural stalk, straw and trash, agro-industrial bagasse, paddy husks and shells, forestry and woodchips, barks and trims, and riverside greens (Farooq and Kumar, 2013; Naqvi et al., 2018).2 Agricultural waste, agro-industrial waste or lignocellulosic waste and wood-based residues are presently estimated to be around 20,494, 25,271, and 1,121 million tons, respectively (Anwar et al., 2014).2 The total estimated biomass potential of Pakistan is 50,000 GW h/year (Farooqui, 2014), which contributes up to 36% of the total nation's energy scenario (Asif, 2009). The various types of biomass feedstock/sources available for power generation are shown in the following Figure 6 (Asif, 2009; Naqvi et al., 2018).2
Potential of Agricultural Residues
Owing to the huge agricultural sector, Pakistan produces a large number of agricultural residues including wheat husks, rice husks, cotton sticks and sugar cane residues. In addition to non-woody agricultural residues, the woody portion also contributes a great share to the production of energy (Mirza et al., 2008a; Aziz, 2013; Zuberi et al., 2013). The total land structure of Pakistan is presented in Figure 7 (Book, 2017).
Cotton is being planted on a large scale with an average crop production of 2,00,000 tons, 2.67 million hectares (11% of the agricultural land) during 2011–12. From 2011 to 2012, the cotton stalks obtained were about 5,898,771 tons, containing an energy potential of 614 GWh. Pakistan is positioned as the fourth largest sugarcane producing country. According to a survey from 2011 to 2012, Pakistan's sugar cane crop cultivation was measured to be 63,920,000 tons, with 5,752,800 tons of residue possessing a power potential of 9,475 GWh (Table 4) (Naqvi et al., 2018). Sugar mills contribute ~2,000 MW in the total national energy (Naqvi et al., 2018).
Regarding the cultivation of wheat, Pakistan is listed as the world's third largest country in the production of wheat, with its total share of the agricultural sector reaching 10.1%, and it has been identified as a potential source to produce clean energy, such as bio-oil (Iqbal et al., 2018). Together with these major crops, other crop residues (maize, rice, gram, etc.) also encompass a significant share of the total of agricultural residues that are available for energy conversion (Farooq and Kumar, 2013). The total residue production from the agriculture sector was estimated to be 62 million tons, with 2% growth rate per annum since 2000, which became 81 million tons per annum in 2012–13 (Farooq and Kumar, 2013; Ghafoor et al., 2016). Woody residues are utilized extensively in the household and cottage industries. Forest covers 5.2% (4.224 million hectares) of the available land and provides about 80% of the total generated bio-energy (Sheikh, 2009; Naqvi et al., 2018). Banana production is also considered as a source of renewable energy in Pakistan. According to one estimation, there are 173,000 banana trees per km2 countrywide, with an average caloric value of 17.8 MJ/k. Cutting of the banana trees is usually carried out three times per annum, resulting 7 kg of heavy residues per tree (Saeed et al., 2015). Saeed et al. (2015) presented a comprehensive study on the estimated energy production from banana cultivation in Pakistan (Table 5). They stated that the share of banana tree remains in the annual consumption of electricity is 2.4% and, by efficiently processing agricultural waste materials, 56% of the total electricity requirement of the nation can be achieved.

Table 5. Energy production from banana crop per year (Saeed et al., 2015).
Potential of Animal Manure
The bovine population of Pakistan is estimated to be 67,294,000 (Table 6) with an annual growth rate of 4% (Amjid et al., 2011; Aziz, 2013; Sakib Sherani, 2009-10). The resulting animal manure measures at 368,434,650 kg, which is deployed in the production of biogas with an energy potential of 23,654 GWh (Aziz, 2013; Naqvi et al., 2018). Livestock animals (such as cows, buffaloes, sheep, cattle, etc.) account for 55.3% of the total agricultural sector, which was elevated to 55.4% a year later, with total contributions of 11.9 and 12.1% to the GDP, respectively (Uddin et al., 2016).
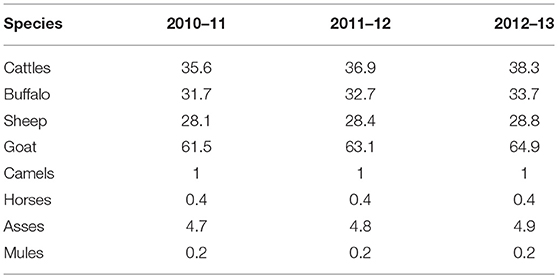
Table 6. Livestock population (in millions) in Pakistan from 2010 to 2013 (Uddin et al., 2016).
Potential of Municipal Solid Waste (MSW)
Being a populous country, urban areas of Pakistan produce more than 64,000 tons of municipal solid waste daily, which comprises both organic and inorganic components and can be efficiently processed into various energy forms with an average calorific value of 6.89 MJ/kg (Harijan et al., 2008; Aziz, 2013; Valasai et al., 2017). In the major cities of Pakistan (such as Karachi, Islamabad, Lahore, Multan, Faisalabad, Peshawar, Gujranwala, Rawalpindi, and Quetta), the estimated MSW production is about 7,121,626 tons (Aziz, 2013). MSW can contribute 13,900 GWh of energy production (Naqvi et al., 2018). Pakistan is confronting issues of waste (damped papers, plastics, metals, rubbers, glass, textile effluents, cardboards, bones, leaves, grass, straws, wood, fodder, and so on) mismanagement as the effluent is not disposed of properly. The typical composition of the MSW is shown in Figure 8 (Zuberi and Ali, 2015). According to an approximation, 9.8 million tons of MSW were produced in 2005, 57% of which was collected for utilization in the production of electricity (Farooq and Kumar, 2013). Muhammad Khalid Farooq and S. Kumar (Farooq and Kumar, 2013) reported the complete scenario of MSW in Pakistan shown in Table 7.
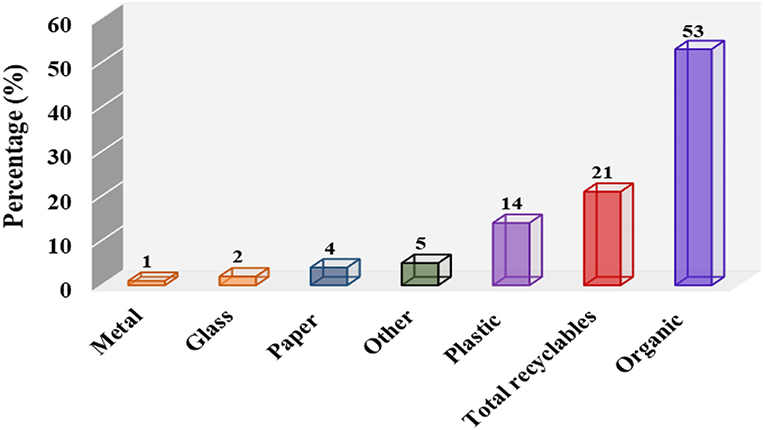
Figure 8. Composition of municipal waste (Zuberi and Ali, 2015).
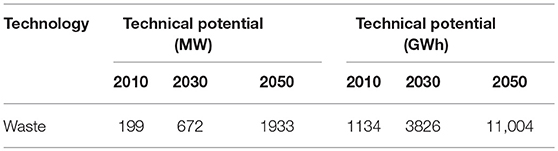
Table 7. The potential of Municipal Solid Waste (MSW) in Pakistan (Farooq and Kumar, 2013; Valasai et al., 2017).
Methodologies for Biomass-to-Energy Conversion
There are two major classes of the techniques for processing of biomass into bioenergy, such as (i) thermo-chemical conversion techniques including direct combustion, liquefaction, trans-esterification, gasification and pyrolysis; and (ii) biochemical decomposition techniques including anaerobic decomposition and fermentation, as shown in Figure 9 (McKendry, 2002a,b; Naqvi et al., 2018). Another unique set of techniques receiving attention is bioelectrochemical systems (BES). In bioelectrochemical techniques, microbes are utilized to yield valuable inorganic and organic byproducts along with power generation by oxidization of biological substratum (Pant et al., 2012; Kelly and He, 2014; Wang et al., 2015; Bajracharya et al., 2016; Khan et al., 2017). BES is a potential platform for producing sustainable bio-energy by minimum power consumption and it can also be applied as a robust tool for remediation of contaminants, such as carbon dioxide, nitrates, phosphates, and micropollutants in wastewater, etc. (Jin and Fallgren, 2014; Kelly and He, 2014; Sultana et al., 2015; Wang et al., 2015). Amongst various thermo-chemical processes, the most preferable are gasification and pyrolysis. Biomass pyrolysis has drawn much consideration recently owing to the production of bio-oil, biochar, and hydrogen-rich fuel gas (Ferdous et al., 2001; Li et al., 2004; Goyal et al., 2008). Pyrolysis of biomass takes place at elevated temperatures above 400°C in the absence of oxygen or air, and the nature of the resulting products is determined by the chemical composition of the biomass and reaction conditions of the pyrolysis (Li et al., 2004; Naqvi et al., 2018). The efficiency of the pyrolysis process mainly depends upon various factors, such as particle size, temperature, heating rate, residence time, biopolymer composition, and type of catalyst (Li et al., 2004). The process of pyrolysis has certain limitations including the production of unstable crude bio-oil, which is comprised of high contents of water and oxygen and needs to be further upgraded for direct use in combustible engines. Moreover, an air decontamination installation is essential as the pyrolysis process generates a high concentration of detrimental gases, such as carbon dioxide, carbon monoxide, etc. As a result of pyrolysis, the delivered ashes are reported to have high heavy metal content that is regarded as hazardous waste (Zaror and Pyle, 1982; Serio et al., 2001; Yaman, 2004; Jahirul et al., 2012). Sometimes, biochemical conversion methodology is applied to wet biomass (anaerobic digestion, ethanol fermentation, lignocellulosic conversions) for biomass energy conversions (Goyal et al., 2008; Naqvi et al., 2018).2 The anaerobic digestion process is well-recognized in the generation of biogas from biomass including MSW, remnants of fruits, vegetables, leaves, grasses, woods, weeds, as well as marine and freshwater biomass. As an outcome of this process, the biogas obtained is rich in methane (60%) together with hydrogen and carbon dioxide, which can be utilized for numerous applications, such as transportation fuel, heating fuel, as a substitute to natural gas and so on (Jones and Ogden, 1984; Chynoweth, 1987; Gunaseelan, 1997; Chynoweth et al., 2001). Almost all types of dry biomass can be effectively utilized as fuel in combustion-based processes with the limited end-use of products. For gasification, woody biomass—for example husks and wood chips—are good fuels in dry form. Gasification of biomass is carried out in the presence of oxygen, air and/or steam at temperatures exceeding 700°C with or without a catalyst, which subsequently generates a syngas consisting of hydrogen, methane, carbon dioxide, carbon monoxide, nitrogen and water vapor (Nunes et al., 2016). Various reactor configurations are utilized, such as fixed bed (updraft or downdraft) and fluidized bed (bubbling or circulation) reactors. Moreover, with the application of gas turbines or diesel generators, the generated gases can be converted into electricity (Chynoweth et al., 2001). The complete process of biomass conversion to syngas consists of different steps (Figure 10) (Kumar et al., 2009). Some of the semi-woody biomass, such as corncobs and corn stalks, can also be used for gasification. Leafy biomass, such as straw and stalks need to be densified (pellet) for gasification (Demirbas et al., 2009; Kumar et al., 2009; Farooq and Kumar, 2013).2 An alternative sustainable solution of solid waste management including agricultural waste materials and animal manure is composting, which is frequently practiced in various underdeveloped countries including Pakistan. In the process of composting, the organic matter is subjected to aerobic or anaerobic decomposition by microbes, which act as an effective fertilizer or soil conditioner for the agricultural land. Moreover, composting results in the production of harmful greenhouse gases—for instance, methane, ammonia, nitrous oxide, and carbon dioxide—which, together with deteriorating the atmosphere, emits an unpleasant odor and causes contamination of the underground water sources (Inbar et al., 1993; Smet et al., 1999; Alfano et al., 2008; Ngoc and Schnitzer, 2009; Shen et al., 2011).
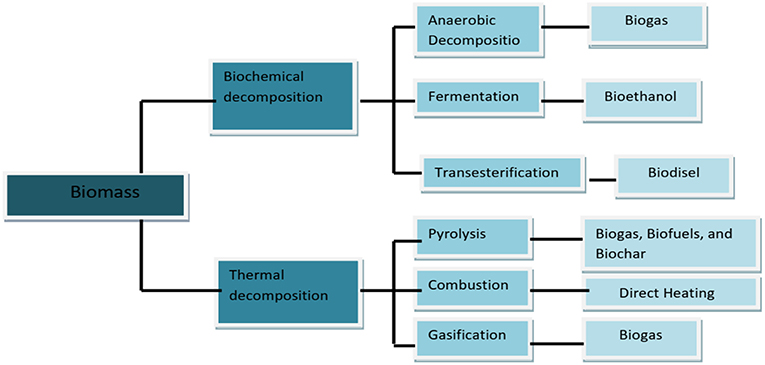
Figure 9. Various techniques for biomass conversion into bio-fuels (Naqvi et al., 2018).
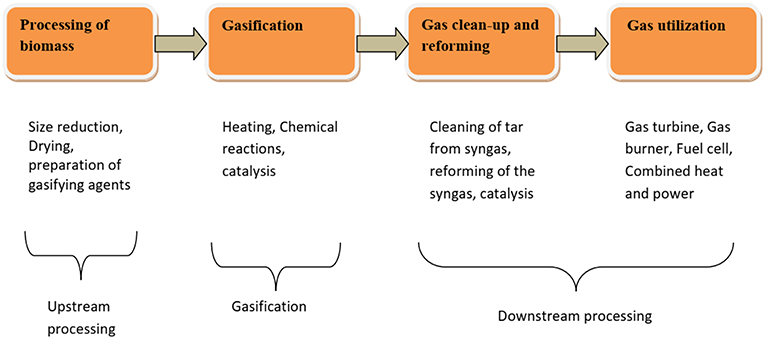
Figure 10. Steps involved in gasification of biomass (Kumar et al., 2009).
Biomass Composition
The woody biomass is categorized into three types—softwood, hardwood, and eucalypt. Lignocellulosic biomass primarily consists of three natural polymers (lignin, cellulose, and hemicelluloses). The chemical composition of different biopolymers of wood is given in Table 8 (Papari and Hawboldt, 2015).
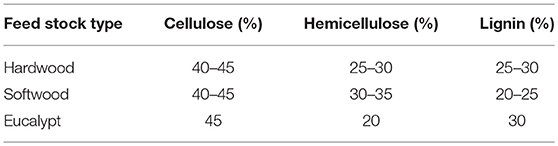
Table 8. Chemical composition of different biopolymers of wood (Papari and Hawboldt, 2015).
Biomass Products
The final product of pyrolysis of biomass depends on feedstock that can be sourced from agricultural waste, such as rice, wheat, sugar cane, and forestry residues, such as sawdust, bark, wood chips, shavings, and algae. Three main products obtained from biomass are bio-oil, biochar, and non-condensable fuel gas, in addition to solid pellets, chemicals and biofuels (Papari and Hawboldt, 2015).
Bio-Oils
During pyrolysis, the breakage of bonds between the biopolymers (lignin, cellulose, and hemi-cellulose) leads to volatile vapors being produced. These vapors are condensed to form a liquid called bio-oil. Fast pyrolysis leads to higher bio-oil yields and slow pyrolysis to lower yields. Bridgwater (2012) and Duman et al. (2011) describe the bio-oil as a complex mixture of different organic compounds, which requires blending with conventional fuels or hydro-deoxygenation to make it usable as a liquid fuel. Bio-oil is a complex mixture of around 300 different compounds as stated by Zhang (Zhang et al., 2007). Various researchers (Oasmaa and Kuoppala, 2003; Oasmaa and Meier, 2005; Mullen et al., 2010) have described the weighted percentage of compounds present in bio-oil to include water (10–30%), aldehydes (1–17%), acids (3–10%), carbohydrates (3–34%), phenolics (2–15%), alcohols (<4%), ketones (2–11%), and other unclassified compounds (5–58%). Calorific value, pH and viscosity of bio-oil are shown by Zhang (Zhang et al., 2007) to vary between 16 and 30 MJ/kg, 2.5–3.4 and 40–100 (cP), which makes it an inferior fuel compared to diesel.
Pyrolysis of biomass generates bio-oil which is a CO2-neutral fuel with limited contents of sulfur oxide gases and can be readily stored and transported (Mohan et al., 2006). Bio-oils have been catalytically improved to high-quality hydrocarbon fuels and can be used as an alternative to petroleum fuels, which are of exceptionally high cost (Czernik and Bridgwater, 2004; Mohan et al., 2006). Iqbal et al. (2018) presented a study based on the production of bio-oil from the wheat residues by using the fast pyrolysis methodology. They studied the energy products of the fast pyrolysis of wheat stalks under a temperature range of 300–650 degree Celsius, which resulted in the phenolics, linear ketones, anhydrosugars, ketones, furan, anhydrosugar and acids, with percentage shares of 27.32, 8.53, 7.66, 8.53, 10.74, and 11.12%, respectively (Iqbal et al., 2018).
Biofuels
Biofuels are harnessed from food crops that require, for instance, bio-ethanol from corn, wheat or sugar beet, and biodiesel from oil seeds (such as rapeseed, soybean, palm, sunflower, and so forth) (Demirbas, 2011; Naqvi et al., 2018). Some of the biofuels are highly controversial as they use land that is used for growing food. Forestry and agricultural remains along with the MSW can also be exploited in the production of biofuels (Balat, 2007). Another classification of bio-fuels presented based on the conversion technology including first generation biofuels, second-generation biofuels, third generation biofuels, and fourth generation biofuels as shown in Figure 11. Variant products obtained by using first-generation biofuels are bio-alcohols, vegetable oil, biodiesel, bio-syngas, and biogas. Bio-alcohols, bio-oil, bioDMF, biohydrogen, and bio-Fischer–Tropsch diesel are generated by employing the second-generation biofuel technology. Third-generation biofuel technology results in the production of vegetable oil and biodiesel, and the fourth-generation technology gives bio-gasoline (Demirbas, 2011).
Vegetable oils and animal fats are also frequently deployed nowadays in the production of biodiesels (a biofuel) through trans-esterification, which is used in compression–ignition engines or diesel engines. Low sulfur content, flash point, aromatic content, and biodegradability make biodiesel superior to diesel fuel (Mirza et al., 2008a). Currently, Pakistan possesses the largest stocks of the methanol and ethanol, which react with vegetable oil to form bio-diesels. Pakistan is estimated to have the potential to produce 56 million tons of bio-diesels, which is far greater than the required amount (8.5 million tons). It has been reported in the literature that Pakistan has the potential to produce ~930,000 tons of edible oils, which is expected to be elevated in the future (Khan and el Dessouky, 2009).
Biogas
Biogas is generated by using the microbially-controlled anaerobic digestion process from waste byproducts including animal dung, urban waste, and crop residues with high moisture content. On average, biogas contains methane (50 to 70%), carbon dioxide (30 to 50%) and traces of other gases, such as hydrogen, hydrogen sulfides, moisture, and siloxanes. Biogas plants of varying scales are deployed in domestic biogas production for cooking and in industrial installations to generate electricity. In the literature, the reported calorific value of biogas is 21–24 MJ/m3 (Sheikh, 2010; Amjid et al., 2011; Jiang et al., 2011; Saleh, 2012). Rural areas of Pakistan are replete with a variety of biomass resources (animal dung waste, MSW, agri-industrial, and agricultural waste), which can be used to produce about 12 million cubic meters of biogas per day, which is enough to fulfill the energy requirements of 28 million rural people. Currently, Pakistan possesses ~5,357 operational biogas units, with a varying production capacity of 3–15 m3/day; however, the targeted total biogas potential is 12–16 million m3/day (Mirza et al., 2008a; Sheikh, 2010; Javed et al., 2016; Naqvi et al., 2018). In Pakistan, the installment of biogas plants was started in 1974, and from then 4,137 plants were installed until 1987, but the growth rate of this technology became extremely slow, resulting in an increase of the figure to only 6,000 installations by 2006. Nevertheless, new policies are being developed by the government to install 3,00,000 biogas units throughout Pakistan within 10 years. This program was initiated in 2009, but only 5,360 plants have been successfully installed as of 2014 (Saleh, 2012).8,9 Biogas is also used to produce electricity in Pakistan on a small scale, as 92 biogas plants are working in this regard resulting in the generation of 790 KW electricity (Uddin et al., 2016). There is significant potential that remains to be explored to install biogas plants for electricity production.
Biochar
Biochar is a charcoal-like substance (Chynoweth et al., 2001) produced by the thermochemical conversion of organic matter, such as crop residues, various grasses, and agricultural plant residues. Char can be produced either by heating biomass with or without air or oxygen (pyrolysis) by processes known as gasification and pyrolysis (run in the regime that leaves charcoal residue) (Jones and Ogden, 1984; Chynoweth et al., 2001).
Carbon is found in all living organisms. It is the major building block for life on Earth. Carbon exists in many forms, predominately as plant biomass, soil organic matter and as the gas carbon dioxide (CO2) in the atmosphere and dissolved in seawater (Demirbas et al., 2009). Carbon dioxide (CO2) is a potent greenhouse gas along with other gases, such as methane (CH4) and nitrous oxide (NOx) (Jones and Ogden, 1984; Demirbas et al., 2009; Kumar et al., 2009; Ngoc and Schnitzer, 2009; Nunes et al., 2016). CO2 is released through natural processes; for example via respiration and volcano eruptions or by human activities, such as the burning of fossil and biomass fuels. It has been reported that in the last 150 years, the amount of carbon in the atmosphere has increased by 30% (Alfano et al., 2008). According to the World Meteorological Organization (WMO) “concentrations of carbon dioxide in the atmosphere surged at a record-breaking speed in 2016 to the highest level in 800,000 years.” This high level of CO2 in the atmosphere affects the global climate. The scientists believe that the rising global temperature has a direct relationship with the increased level of CO2 (Alfano et al., 2008). It is estimated that global temperatures will rise for decades to come, largely due to greenhouse gases emitted by human activities. At present, Pakistan contributes <1% of the total global greenhouse gas emission on a per-capita basis. However, due to the geographical location of Pakistan, average temperatures are predicted to rise faster than elsewhere, increasing 7.2°F (4°C) by the year 2100 (2012 World Wildlife Fund report) (Inbar et al., 1993). Pakistan's per capita energy consumption and cumulative CO2 emissions are extremely low (Inbar et al., 1993). However, the situation will not remain so as Pakistan is projected to add nearly 100 million people by 2050, causing great strains on its resources. According to projections, the country will increase carbon emissions by 300 percent in the next 15 years due to more cars which clog roads and an increased demand for electricity (Inbar et al., 1993). Hence, it is high time for Pakistan to focus on using renewable sources for energy production that will lead the country to a low-carbon pathway. In this direction, biochar production via pyrolysis of biomass is a carbon-negative system that leads to the production of energy products and biochar for carbon sequestration. This technology is carbon-negative as it removes net carbon dioxide from the atmosphere and can store CO2 in the soil via a process known as carbon sequestration. Electrochemical reduction of the carbon dioxide is also actualized by deploying modified microbial electrochemical technologies (METs) including microbial fuel cells (MFCs), which aim to convert of biomass into electrical power using micro-organisms (Rabaey and Verstraete, 2005; Logan et al., 2006; Oh and Logan, 2007; Wang et al., 2010). In low-cost microbial electrochemical technologies (METs) or bioelectrochemical systems (BES), such as microbial electrolysis cells (MECs), the biological substrate is oxidized at the surface of the anode by utilizing bacteria that act as exoelectrogens, leading to electron generation and also CO2 (Aelterman et al., 2006; Logan, 2009; Qiao et al., 2010; Villano et al., 2010; Zhao et al., 2012). Then, these electrons are captured by the cathode. On the cathode surface, the electron and diffused protons cause a reduction process of CO2 and produce valuable products; for example, hydrogen gas or water and methane [CO2 + 8H+ + 9e−1 → CH4 + 2H2O] (Call and Logan, 2008; Sun et al., 2008; Cheng et al., 2009; Villano et al., 2010; Wang et al., 2010; Kim and Logan, 2011; Logan and Rabaey, 2012; Zhao et al., 2012; Khan et al., 2017). It is estimated that biochar has the potential to sequester almost 400 billion tons of carbon by 2100 and to lower atmospheric CO2 concentrations by 37 parts per million (Ngoc and Schnitzer, 2009). Overall, it seems reasonable to conclude that biochar production from biomass has the potential to offset the entire annual CO2 increase in the atmosphere (4.8 vs. 4.1 GtC/yr), as shown in Table 9 (Jones and Ogden, 1984).
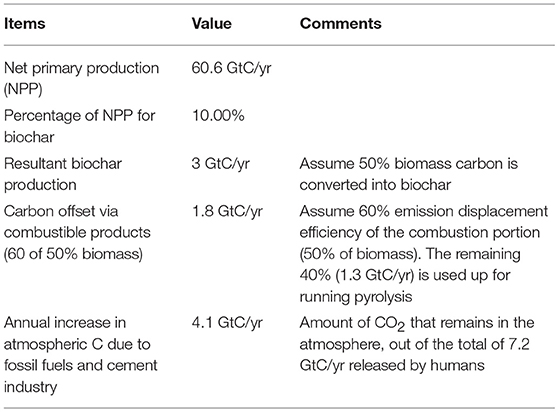
Table 9. Potentials for worldwide carbon sequestration via biochar production and dispersion over agricultural land (Jones and Ogden, 1984).
Conclusion
Being a developing country, Pakistan is facing a severe energy crisis that limits its economy. Because of the rapidly growing population and economy, Pakistan's energy needs become potentially huge; to resolve these serious issues, AEDB is currently functioning on the development of recent renewable energy technologies in Pakistan, which will be beneficial for the developing economy of Pakistan to minimize the growing energy crisis. The sources of renewable energy are hydel, solar, wind and biomass, which has significant potential to compete with growing energy requirements. Thus far, Pakistan envisions setting up plants to generate 10,000 MW through renewable resources by 2030. These plants will be installed with a 50% share of the beneficiary. Out of all the renewable energy resources, biomass is considered the best and most easily accessible source of energy with its unique environmentally friendly nature. Despite the bountiful presence of biomass energy resources, there is still a need to work on the use of these sources to produce energy.
Author Contributions
All authors listed have made a substantial, direct and intellectual contribution to the work, and approved it for publication.
Conflict of Interest Statement
The authors declare that the research was conducted in the absence of any commercial or financial relationships that could be construed as a potential conflict of interest.
Footnotes
1. ^Worldometer: Population. http://www.worldometers.info/world-population/pakistan-population/ 2017
2. ^Alternative-Energy-Development-Board, Ministry of Water and Power, Government of Pakistan [http://www.aedb.org/].
3. ^https://www.eia.gov/beta/international
4. ^Pakistan Energy Year Book 2015 published by Hydrocarbon Development Institute of Pakistan (HDIP) MoPNR, Government of Pakistan.
5. ^An overview of Fossil Fuels in Pakistan PEY, 2004 by Ministry of Petroleum and Natural Resources, Government of Pakistan.
6. ^Wildlife of Pakistan. Introduction to Pakistan: Coastline WoP: Available online at: http://www.wildlifeofpakistan.com/IntroductiontoPakistan/coastlineofPakistan.htm
7. ^Hydropower Development in Pakistan [http://www.wapda.gov.pk/index.php/projects/hydropower-development-in-pakistan].
8. ^EKN-RSPN Pakistan Domestic Biogas Programme (PDBP): [http://www.rspn.org/index.php/projects/completed/ekn-pdbp/].
9. ^(PDBP) E-RPDBP: [http://www.rspn.org/wp-content/uploads/2014/09/Newsletter-Jan-Mar-2012.pdf].
References
Adnan, S., Hayat Khan, A., Haider, S., and Mahmood, R. (2012). Solar energy potential in Pakistan. J. Renew. Sustain. Energy 4:032701. doi: 10.1063/1.4712051
Aelterman, P., Rabaey, K., Pham, H. T., Boon, N., and Verstraete, W. (2006). Continuous electricity generation at high voltages and currents using stacked microbial fuel cells. Environ. Sci. Technol. 40, 3388–3394. doi: 10.1021/es0525511
Ahmed, M., Riaz, K., Khan, A. M., and Bibi, S. (2015). Energy consumption–economic growth nexus for Pakistan: taming the untamed. Renew. Sustain. Energy Rev. 52, 890–896. doi: 10.1016/j.rser.2015.07.063
Ahmed, M. A., Ahmed, F., and Akhtar, M. W. (2006). Assessment of wind power potential for coastal areas of Pakistan. Turk. J. Phys. 30, 127–135.
Alfano, G., Belli, C., Lustrato, G., and Ranalli, G. (2008). Pile composting of two-phase centrifuged olive husk residues: technical solutions and quality of cured compost. Bioresour. Technol. 99, 4694–4701. doi: 10.1016/j.biortech.2007.09.080
Amjid, S. S., Bilal, M. Q., Nazir, M. S., and Hussain, A. (2011). Biogas, renewable energy resource for Pakistan. Renew. Sustain. Energy Rev. 15, 2833–2837. doi: 10.1016/j.rser.2011.02.041
Anwar, Z., Gulfraz, M., and Irshad, M. (2014). Agro-industrial lignocellulosic biomass a key to unlock the future bio-energy: a brief review. J. Radiat. Res. Appl. Sci. 7, 163–173. doi: 10.1016/j.jrras.2014.02.003
Ashraf Chaudhry, M., Raza, R., and Hayat, S. A. (2009). Renewable energy technologies in Pakistan: prospects and challenges. Renew. Sustain. Energy Rev. 13, 1657–1662. doi: 10.1016/j.rser.2008.09.025
Asif, M. (2009). Sustainable energy options for Pakistan. Renew. Sustain. Energy Rev. 13, 903–909. doi: 10.1016/j.rser.2008.04.001
Awan, K. Y., and Rashid, A. (2012). Overview of Pakistan's Electricity Crisis, Generation-Mix and Renewable Energy Scenarios.
Bajracharya, S., Sharma, M., Mohanakrishna, G., Benneton, X. D., Strik, D. P., Sarma, P. M., et al. (2016). An overview on emerging bioelectrochemical systems (BESs): technology for sustainable electricity, waste remediation, resource recovery, chemical production and beyond. Renew. Energy 98, 153–170. doi: 10.1016/j.renene.2016.03.002
Balat, M. (2007). Global bio-fuel processing and production trends. Energy Explor. Exploit. 25, 195–218. doi: 10.1260/014459807782009204
Balat, M. (2010). Security of energy supply in Turkey: challenges and solutions. Energy Conv. Manage. 51, 1998–2011. doi: 10.1016/j.enconman.2010.02.033
Bhutto, A. W., Bazmi, A. A., and Zahedi, G. (2012). Greener energy: issues and challenges for Pakistan-hydel power prospective. Renew. Sustain. Energy Rev. 16, 2732–2746. doi: 10.1016/j.rser.2012.02.034
Bhutto, A. W., Bazmi, A. A., and Zahedi, G. (2013). Greener energy: issues and challenges for Pakistan—wind power prospective. Renew. Sustain. Energy Rev. 20, 519–538. doi: 10.1016/j.rser.2012.12.010
Book, S. Y. (2017). Statistical Year Book. Available online at: www.instat.gov.al/media/3658/statistical-yearbook-2017.pdf
Boyle, G. (2004). Renewable Energy, Power for a Sustainable Future. Oxford: Oxford University Press, The Open University.
Bridgwater, A. V. (2012). Review of fast pyrolysis of biomass and product upgrading. Biomass Bioenergy 38, 68–94. doi: 10.1016/j.biombioe.2011.01.048
Call, D., and Logan, B. E. (2008). Hydrogen production in a single chamber microbial electrolysis cell lacking a membrane. Environ. Sci. Technol. 42, 3401–3406. doi: 10.1021/es8001822
Chandio, A. A., Yuansheng, J., and Magsi, H. (2016). Agricultural sub-sectors performance: an analysis of sector-wise share in agriculture GDP of Pakistan. Int. J. Econ. Finance 8:156. doi: 10.5539/ijef.v8n2p156
Cheng, S., Xing, D., Call, D. F., and Logan, B. E. (2009). Direct biological conversion of electrical current into methane by electromethanogenesis. Environ. Sci. Technol. 43, 3953–3958. doi: 10.1021/es803531g
Chikkatur, A. P., Sagar, A. D., and Sankar, T. (2009). Sustainable development of the Indian coal sector. Energy 34, 942–953. doi: 10.1016/j.energy.2008.12.014
Chynoweth, D. P., Owens, J. M., and Legrand, R. (2001). Renewable methane from anaerobic digestion of biomass. Renew. Energy 22, 1–8. doi: 10.1016/S0960-1481(00)00019-7
Cleveland, C. J., Costanza, R., Hall, C. A., and Kaufmann, R. (1984). Energy and the US economy: a biophysical perspective. Science 225, 890–897. doi: 10.1126/science.225.4665.890
Czernik, S., and Bridgwater, A. (2004). Overview of applications of biomass fast pyrolysis oil. Energy Fuels 18, 590–598. doi: 10.1021/ef034067u
Dawood, Y., Saeed, A., Ata, M. G., and Nawaz, M. T. (2013). Impact of International Politics on Pakistans Energy Crisis. Vol. 3, International Journal of Developing Country Studies.
Demirbas, A. (2011). Competitive liquid biofuels from biomass. Appl. Energy 88, 17–28. doi: 10.1016/j.apenergy.2010.07.016
Demirbas, M. F., Balat, M., and Balat, H. (2009). Potential contribution of biomass to the sustainable energy development. Energy Conv. Manage. 50, 1746–1760. doi: 10.1016/j.enconman.2009.03.013
Duman, G., Okutucu, C., Ucar, S., Stahl, R., and Yanik, J. (2011). The slow and fast pyrolysis of cherry seed. Bioresour. Technol. 102, 1869–1878. doi: 10.1016/j.biortech.2010.07.051
Faridi, M. Z. (2012). Contribution of agricultural exports to economic growth in Pakistan. Pak. J. Comm. Soc. Sci. 6, 133–146. Available online at: https://www.econstor.eu/bitstream/10419/188047/1/pjcss079.pdf
Farooq, M. K., and Kumar, S. (2013). An assessment of renewable energy potential for electricity generation in Pakistan. Renew. Sustain. Energy Rev. 20, 240–254. doi: 10.1016/j.rser.2012.09.042
Farooqui, S. Z. (2014). Prospects of renewables penetration in the energy mix of Pakistan. Renew. Sustain. Energy Rev. 29, 693–700. doi: 10.1016/j.rser.2013.08.083
Ferdous, D., Dalai, A., Bej, S., Thring, R., and Bakhshi, N. (2001). Production of H2 and medium Btu gas via pyrolysis of lignins in a fixed-bed reactor. Fuel Process. Technol. 70, 9–26. doi: 10.1016/S0378-3820(00)00147-8
Ghafoor, A., ur Rehman, T., Munir, A., Ahmad, M., and Iqbal, M. (2016). Current status and overview of renewable energy potential in Pakistan for continuous energy sustainability. Renew. Sustain. Energy Rev. 60, 1332–1342. doi: 10.1016/j.rser.2016.03.020
Goyal, H., Seal, D., and Saxena, R. (2008). Bio-fuels from thermochemical conversion of renewable resources: a review. Renew. Sustain. Energy Rev. 12, 504–517. doi: 10.1016/j.rser.2006.07.014
Gunaseelan, V. N. (1997). Anaerobic digestion of biomass for methane production: a review. Biomass and bioenergy 13, 83–114. doi: 10.1016/S0961-9534(97)00020-2
Harijan, K. (2008). Mode Lung and Analysis of Tile Potential Demand for Renewable Sources of Energy in Pakistan. Jamshoro: Mehran University of Engineering and Technology.
Harijan, K., Uqaili, M. A., and Memon, M. (2008). “Renewable energy for managing energy crisis in Pakistan,” in International Multi Topic Conference: 2008 (Berlin; Heidelberg: Springer), 449–455. doi: 10.1007/978-3-540-89853-5_48
Harijan, K., Uqaili, M. A., Memon, M., and Mirza, U. K. (2009). Assessment of centralized grid connected wind power cost in coastal area of Pakistan. Renew. Energy 34, 369–373. doi: 10.1016/j.renene.2008.05.001
Harijan, K., Uqaili, M. A., Memon, M., and Mirza, U. K. (2011). Forecasting the diffusion of wind power in Pakistan. Energy 36, 6068–6073. doi: 10.1016/j.energy.2011.08.009
Harijan, K., Uqaili, M. A., and Mirza, U. K. (2015). Assessment of solar PV power generation potential in Pakistan. J. Clean Energy Technol. 3, 54–56. doi: 10.7763/JOCET.2015.V3.168
Hogan, L., Curtotti, R., and Austin, A. (2007). APEC Energy Security and Sustainable Development Through Efficiency and Diversity: Economic Issues in Technology RandD, Adoption and Transfer. Canberra, ACT: ABARE.
Howden, D. (2007). World Oil Supplies Are Set to Run Out Faster Than Expected, Warn Scientists. The Independent.
Husain, T. (2010). Pakistan's energy sector issues: energy efficiency and energy environmental links. Lahore J. Econ. 15, 33–59. Available online at: http://www.lahoreschoolofeconomics.edu.pk/EconomicsJournal/Journals/Volume%2015/Issue%20SP/03%20Dr%20Tariq%20Husain%20EDITED%20TTC%2011-10-10.pdf
Inbar, Y., Hadar, Y., and Chen, Y. (1993). Recycling of cattle manure: the composting process and characterization of maturity. J. Environ. Qual. 22, 857–863. doi: 10.2134/jeq1993.00472425002200040032x
Iqbal, T., Lu, Q., Dong, C.-Q., Zhou, M.-X., Arain, Z., Ali, Z., et al. (2018). “A study of product distribution under fast pyrolysis of wheat stalk while producing bio-oil,” in Computing, Mathematics and Engineering Technologies (iCoMET), 2018 International Conference on: 2018 (Sukkur: IEEE), 1–6.
Jahirul, M. I., Rasul, M. G., Chowdhury, A. A., and Ashwath, N. (2012). Biofuels production through biomass pyrolysis—a technological review. Energies 5, 4952–5001. doi: 10.3390/en5124952
Jamil, F., and Ahmad, E. (2014). An empirical study of electricity theft from electricity distribution companies in Pakistan. Pak. Dev. Rev. 239–254. doi: 10.30541/v53i3pp.239-254
Javaid, M. A., Hussain, S., Maqsood, A., Arshad, Z., Arshad, A., and Idrees, M. (2011). Electrical energy crisis in Pakistan and their possible solutions. Int. J. Basic Appl. Sci. 11, 26–35. Available online at: http://climateinfo.pk/frontend/web/attachments/data-type/Javaid%20et%20al%20(2011)%20Electrical%20Energy%20Crisis%20in%20Pakistan%20and%20Their%20Possible%20Solutions.pdf
Javed, M. S., Raza, R., Hassan, I., Saeed, R., Shaheen, N., Iqbal, J., et al. (2016). The energy crisis in Pakistan: a possible solution via biomass-based waste. J. Renew. Sustain. Energy 8:043102. doi: 10.1063/1.4959974
Jiang, X., Sommer, S. G., and Christensen, K. V. (2011). A review of the biogas industry in China. Energy Policy 39, 6073–6081. doi: 10.1016/j.enpol.2011.07.007
Jin, S., and Fallgren, P. H. (2014). “Feasibility of using bioelectrochemical systems for bioremediation,” in Microbial Biodegradation and Bioremediation, ed S. Das (Rourkila: Elsevier), 389–405.
Jones, H. B. Jr., and Ogden, E. (1984). Biomass energy potential from livestock and poultry wastes in the Southern United States. Biomass 6, 25–35. doi: 10.1016/0144-4565(84)90005-2
Kakar, Z. K., Khilji, B. A., and Khan, M. J. (2011). Financial development and energy consumption: empirical evidence from Pakistan. Int. J. Trade Econ. Finance 2:469. doi: 10.7763/IJTEF.2011.V2.150
Kelly, P. T., and He, Z. (2014). Nutrients removal and recovery in bioelectrochemical systems: a review. Bioresour. Technol. 153, 351–360. doi: 10.1016/j.biortech.2013.12.046
Kessides, I. N. (2013). Chaos in power: Pakistan's electricity crisis. Energy Policy 55, 271–285. doi: 10.1016/j.enpol.2012.12.005
Khahro, S. F., Tabbassum, K., Soomro, A. M., Dong, L., and Liao, X. (2014b). Evaluation of wind power production prospective and Weibull parameter estimation methods for Babaurband, Sindh Pakistan. Energy Conv. Manage. 78, 956–967. doi: 10.1016/j.enconman.2013.06.062
Khahro, S. F., Tabbassum, K., Soomro, A. M., Liao, X., Alvi, M. B., Dong, L., et al. (2014a). Techno-economical evaluation of wind energy potential and analysis of power generation from wind at Gharo, Sindh Pakistan. Renew. Sustain. Energy Rev. 35, 460–474. doi: 10.1016/j.rser.2014.04.027
Khalil, H. B., and Zaidi, S. J. H. (2014). Energy crisis and potential of solar energy in Pakistan. Renew. Sustain. Energy Rev. 31, 194–201. doi: 10.1016/j.rser.2013.11.023
Khan, A. A., and Khan, M. (2010). Pakistan textile industry facing new challenges. Res. J. Int. Stud. 14, 21–29. Available online at: https://www.researchgate.net/profile/Aftab_Alam18/publication/258351787_Pakistan_textile_industry_facing_new_challenges/links/0deec52808bf9271ff000000.pdf
Khan, A. A., Mehmood, Z., Shahzad, A., Chughtai, K. A., and Javed, A. (2014). Evaluation of wind energy potential alongside motorways of Pakistan. Asian J. Appl. Sci. Eng. 3, 375–381. doi: 10.15590/ajase
Khan, H. A., and Pervaiz, S. (2013). Technological review on solar PV in Pakistan: scope, practices and recommendations for optimized system design. Renew. Sustain. Energy Rev. 23, 147–154. doi: 10.1016/j.rser.2013.02.031
Khan, M., Nizami, A., Rehan, M., Ouda, O., Sultana, S., Ismail, I., et al. (2017). Microbial electrolysis cells for hydrogen production and urban wastewater treatment: a case study of Saudi Arabia. Appl. Energy 185, 410–420. doi: 10.1016/j.apenergy.2016.11.005
Khan, M. A., and Ahmad, U. (2008). Energy demand in Pakistan: a disaggregate analysis. Pak. Dev. Rev. 47, 437–455. doi: 10.30541/v47i4IIpp.437-455
Khan, N. A., and el Dessouky, H. (2009). Prospect of biodiesel in Pakistan. Renew. Sustain. Energy Rev. 13, 1576–1583. doi: 10.1016/j.rser.2008.09.016
Kim, Y., and Logan, B. E. (2011). Microbial reverse electrodialysis cells for synergistically enhanced power production. Environ. Sci. Technol. 45, 5834–5839. doi: 10.1021/es200979b
Komal, R., and Abbas, F. (2015). Linking financial development, economic growth and energy consumption in Pakistan. Renew. Sustain. Energy Rev. 44, 211–220. doi: 10.1016/j.rser.2014.12.015
Kumar, A., Jones, D., and Hanna, M. (2009). Thermochemical biomass gasification: a review of the current status of the technology. Energies 2, 556–581. doi: 10.3390/en20300556
Lee, C.-C., and Chang, C-P. (2008). Energy consumption and economic growth in Asian economies: a more comprehensive analysis using panel data. Resour. Energy Econ. 30, 50–65. doi: 10.1016/j.reseneeco.2007.03.003
Li, S., Xu, S., Liu, S., Yang, C., and Lu, Q. (2004). Fast pyrolysis of biomass in free-fall reactor for hydrogen-rich gas. Fuel Process. Technol. 85, 1201–1211. doi: 10.1016/j.fuproc.2003.11.043
Logan, B. E. (2009). Exoelectrogenic bacteria that power microbial fuel cells. Nat. Rev. Microbiol. 7:375. doi: 10.1038/nrmicro2113
Logan, B. E., Hamelers, B., Rozendal, R., Schröder, U., Keller, J., Freguia, S., et al. (2006). Microbial fuel cells: methodology and technology. Environ. Sci. Technol. 40, 5181–5192. doi: 10.1021/es0605016
Logan, B. E., and Rabaey, K. (2012). Conversion of wastes into bioelectricity and chemicals by using microbial electrochemical technologies. Science 337, 686–690. doi: 10.1126/science.1217412
Mahmood, T., and Ayaz, M. T. (2018). Energy security and economic growth in Pakistan. Pak. J. Appl. Econ. 28, 47–64. Available online at: http://www.aerc.edu.pk/wp-content/uploads/2018/04/Paper-759-TAHIR-IV-1.pdf
Malik, A. (2012). Power Crisis in Pakistan: A Crisis in Governance? Islamabad: Pakistan Institute of Development Economics.
Malik, S. N., and Sukhera, O. R. (2012). Management of natural gas resources and search for alternative renewable energy resources: a case study of Pakistan. Renew. Sustain. Energy Rev. 16, 1282–1290. doi: 10.1016/j.rser.2011.10.003
Mathur, J. (2001). Development of a Modified Dynamic Energy and Greenhouse Gas Reduction Planning Approach Through the Case of Indian Power Sector. Essen: Universität GH Essen.
McKendry, P. (2002a). Energy production from biomass (part 1): overview of biomass. Bioresour. Technol. 83, 37–46. doi: 10.1016/S0960-8524(01)00118-3
McKendry, P. (2002b). Energy production from biomass (part 2): conversion technologies. Bioresour. Technol. 83, 47–54. doi: 10.1016/S0960-8524(01)00119-5
Mirza, U. K., Ahmad, N., and Majeed, T. (2008a). An overview of biomass energy utilization in Pakistan. Renew. Sustain. Energy Rev. 12, 1988–1996. doi: 10.1016/j.rser.2007.04.001
Mirza, U. K., Ahmad, N., Majeed, T., and Harijan, K. (2007). Wind energy development in Pakistan. Renew. Sustain. Energy Rev. 11, 2179–2190. doi: 10.1016/j.rser.2006.03.003
Mirza, U. K., Ahmad, N., Majeed, T., and Harijan, K. (2008b). Hydropower use in Pakistan: past, present and future. Renew. Sustain. Energy Rev. 12, 1641–1651. doi: 10.1016/j.rser.2007.01.028
Mirza, U. K., Maroto-Valer, M. M., and Ahmad, N. (2003). Status and outlook of solar energy use in Pakistan. Renew. Sustain. Energy Rev. 7, 501–514. doi: 10.1016/j.rser.2003.06.002
Mohan, D., Pittman, C. U., and Steele, P. H. (2006). Pyrolysis of wood/biomass for bio-oil: a critical review. Energy Fuels 20, 848–889. doi: 10.1021/ef0502397
Mullen, C. A., Boateng, A. A., Goldberg, N. M., Lima, I. M., Laird, D. A., and Hicks, K. B. (2010). Bio-oil and bio-char production from corn cobs and stover by fast pyrolysis. Biomass Bioenergy 34, 67–74. doi: 10.1016/j.biombioe.2009.09.012
Muneer, T., Maubleu, S., and Asif, M. (2006). Prospects of solar water heating for textile industry in Pakistan. Renew. Sustain. Energy Rev. 10, 1–23. doi: 10.1016/j.rser.2004.07.003
Naqvi, S. R., Jamshaid, S., Naqvi, M., Farooq, W., Niazi, M. B. K., Aman, Z., et al. (2018). Potential of biomass for bioenergy in Pakistan based on present case and future perspectives. Renew. Sustain. Energy Rev. 81, 1247–1258. doi: 10.1016/j.rser.2017.08.012
Nawaz, S., Iqbal, N., and Anwar, S. (2013). Electricity demand in Pakistan: a nonlinear estimation. Pak. Dev. Rev. 52, 479–491. doi: 10.30541/v52i4Ipp.479-492
Ngoc, U. N., and Schnitzer, H. (2009). Sustainable solutions for solid waste management in Southeast Asian countries. Waste Manage. 29, 1982–1995. doi: 10.1016/j.wasman.2008.08.031
Nunes, L., Matias, J., and Catalão, J. (2016). Biomass combustion systems: a review on the physical and chemical properties of the ashes. Renew. Sustain. Energy Rev. 53, 235–242. doi: 10.1016/j.rser.2015.08.053
Oasmaa, A., and Kuoppala, E. (2003). Fast pyrolysis of forestry residue. 3. Storage stability of liquid fuel. Energy Fuels 17, 1075–1084. doi: 10.1021/ef030011o
Oasmaa, A., and Meier, D. (2005). Norms and standards for fast pyrolysis liquids: 1. Round robin test. J. Anal. Appl. Pyrol. 73, 323–334. doi: 10.1016/j.jaap.2005.03.003
Oh, S.-E., and Logan, B. E. (2007). Voltage reversal during microbial fuel cell stack operation. J. Power Sources 167, 11–17. doi: 10.1016/j.jpowsour.2007.02.016
Østergaard, P. A., and Sperling, K. (2014). Towards sustainable energy planning and management. Int. J. Sustain. Energy Plan. Manage. 1, 1–5. doi: 10.5278/ijsepm.2014.1.1
Pant, D., Singh, A., Van Bogaert, G., Irving Olsen, S., Singh Nigam, P., Diels, L., et al. (2012). Bioelectrochemical systems (BES) for sustainable energy production and product recovery from organic wastes and industrial wastewaters. RSC Adv. 2, 1248–1263. doi: 10.1039/C1RA00839K
Papari, S., and Hawboldt, K. (2015). A review on the pyrolysis of woody biomass to bio-oil: focus on kinetic models. Renew. Sustain. Energy Rev. 52, 1580–1595. doi: 10.1016/j.rser.2015.07.191
Pimentel, D., Hurd, L., Bellotti, A., Forster, M., Oka, I., Sholes, O., et al. (1973). Food production and the energy crisis. Science 182, 443–449. doi: 10.1126/science.182.4111.443
Qiao, Y., Bao, S.-J., and Li, C. M. (2010). Electrocatalysis in microbial fuel cells—from electrode material to direct electrochemistry. Energy Environ. Sci. 3, 544–553. doi: 10.1039/b923503e
Rabaey, K., and Verstraete, W. (2005). Microbial fuel cells: novel biotechnology for energy generation. Trends Biotechnol. 23, 291–298. doi: 10.1016/j.tibtech.2005.04.008
Rafique, M. M., and Rehman, S. (2017). National energy scenario of Pakistan–current status, future alternatives, and institutional infrastructure: an overview. Renew. Sustain. Energy Rev. 69, 156–167. doi: 10.1016/j.rser.2016.11.057
Raja, I. A., and Abro, R. S. (1994). Solar and wind energy potential and utilization in Pakistan. Renew. Energy 5, 583–586. doi: 10.1016/0960-1481(94)90439-1
Rashid Amjad, S. J. B. (Ed.). (2015). Pakistan Moving the Economy Forward. New Delhi: Cambridge University Press.
Raza, S. A., Ali, Y., and Mehboob, F. (2012). Role of Agriculture in Economic Growth of Pakistan. Euro Journals.
Rehman, M. S. U., Rashid, N., Saif, A., Mahmood, T., and Han, J-I. (2013). Potential of bioenergy production from industrial hemp (Cannabis sativa): Pakistan perspective. Renew. Sustain. Energy Rev. 18, 154–164. doi: 10.1016/j.rser.2012.10.019
Sadorsky, P. (2010). The impact of financial development on energy consumption in emerging economies. Energy Policy 38, 2528–2535. doi: 10.1016/j.enpol.2009.12.048
Saeed, M., Irshad, A., Sattar, H., Andrews, G., Phylaktou, H., and Gibbs, B. (2015). “Agricultural waste biomass energy potential in Pakistan,” in Proceedings of the International Conference held in Shanghai, PR China: 2015 (Leeds).
Sahir, M. H., and Qureshi, A. H. (2008). Assessment of new and renewable energy resources potential and identification of barriers to their significant utilization in Pakistan. Renew. Sustain. Energy Rev. 12, 290–298. doi: 10.1016/j.rser.2006.07.002
Sakib Sherani, M. O. F. (2009-10). Growth and Development, Pakistan Economic Survey. Government of Pakistan.
Saleh, A. (2012). Biogas Potential in Pakistan. Lahore: Biomass Conversion Research Centre, Department of Chemical Engineering, COMSATS Institute of information Technology.
Serio, M. A., Kroo, E., Bassilakis, R., Wójtowicz, M. A., and Suuberg, E. M. (2001). A Prototype Pyrolyzer for Solid Waste Resource Recovery in Space. SAE Technical Paper. doi: 10.4271/2001-01-2349
Shaikh, F., Ji, Q., and Fan, Y. (2015). The diagnosis of an electricity crisis and alternative energy development in Pakistan. Renew. Sustain. Energy Rev. 52, 1172–1185. doi: 10.1016/j.rser.2015.08.009
Sheikh, M. A. (2009). Renewable energy resource potential in Pakistan. Renew. Sustain. Energy Rev. 13, 2696–2702. doi: 10.1016/j.rser.2009.06.029
Sheikh, M. A. (2010). Energy and renewable energy scenario of Pakistan. Renew. Sustain. Energy Rev. 14, 354–363. doi: 10.1016/j.rser.2009.07.037
Shen, Y., Ren, L., Li, G., Chen, T., and Guo, R. (2011). Influence of aeration on CH4, N2O and NH3 emissions during aerobic composting of a chicken manure and high C/N waste mixture. Waste Manage. 31, 33–38. doi: 10.1016/j.wasman.2010.08.019
Siddiqi, A., Wescoat, J. L Jr, Humair, S., and Afridi, K. (2012). An empirical analysis of the hydropower portfolio in Pakistan. Energy Policy 50, 228–241. doi: 10.1016/j.enpol.2012.06.063
Siddique, S., and Wazir, R. (2016). A review of the wind power developments in Pakistan. Renew. Sustain. Energy Rev. 57, 351–361. doi: 10.1016/j.rser.2015.12.050
Siddiqui, R., Jalil, H. H., Nasir, M., Malik, W. S., and Khalid, M. (2008). The cost of unserved energy: evidence from selected industrial cities of Pakistan. Pak. Dev. Rev. 47, 227–246. Available online at: https://www.jstor.org/stable/23234709?seq=1#page_scan_tab_contents
Smet, E., Van Langenhove, H., and De Bo, I. (1999). The emission of volatile compounds during the aerobic and the combined anaerobic/aerobic composting of biowaste. Atmos. Environ. 33, 1295–1303. doi: 10.1016/S1352-2310(98)00260-X
Solangi, K., Islam, M., Saidur, R., Rahim, N., and Fayaz, H. (2011). A review on global solar energy policy. Renew. Sustain. Energy Rev. 15, 2149–2163. doi: 10.1016/j.rser.2011.01.007
Sultana, S., Khan, M. D., Sabir, S., Gani, K. M., Oves, M., and Khan, M. Z. (2015). Bio-electro degradation of azo-dye in a combined anaerobic–aerobic process along with energy recovery. New J. Chem. 39, 9461–9470. doi: 10.1039/C5NJ01610J
Sun, M., Sheng, G. P., Zhang, L., Xia, C. R., Mu, Z. X., Liu, X. W., et al. (2008). An MEC-MFC-coupled system for biohydrogen production from acetate. Environ. Sci. Technol. 42, 8095–8100. doi: 10.1021/es801513c
Syed, M. S., Chaudhry, I. A., Farooq, M., and Qamar, A. (2014). Modeling and forecasting of energy scenario in Pakistan with application of decentralized energy planning. J. Faculty Eng. Technol. 21, 11–24. Available online at: http://111.68.103.26/journals/index.php/jfet/article/view/399
Turton, H., and Barreto, L. (2006). Long-term security of energy supply and climate change. Energy Policy 34, 2232–2250. doi: 10.1016/j.enpol.2005.03.016
Uddin, W., Khan, B., Shaukat, N., Majid, M., Mujtaba, G., Mehmood, A., et al. (2016). Biogas potential for electric power generation in Pakistan: a survey. Renew. Sustain. Energy Rev. 54, 25–33. doi: 10.1016/j.rser.2015.09.083
Ullah, I., and Chipperfield, A. J. (2010). An evaluation of wind energy potential at Kati Bandar, Pakistan. Renew. Sustain. Energy Rev. 14, 856–861. doi: 10.1016/j.rser.2009.10.014
Ullah, K. (2013). Electricity infrastructure in Pakistan: an overview. Int. J. Energy Inform. Commun. 4, 11–26. Available online at: https://www.researchgate.net/profile/Kafait_Ullah/publication/304826505_Electricity_infrastructure_in_Pakistan_an_overview/links/59b0d4efa6fdcc3f8889b977/Electricity-infrastructure-in-Pakistan-an-overview.pdf
Valasai, G. D., Uqaili, M. A., Memon, H. R., Samoo, S. R., Mirjat, N. H., and Harijan, K. (2017). Overcoming electricity crisis in Pakistan: a review of sustainable electricity options. Renew. Sustain. Energy Rev. 72, 734–745. doi: 10.1016/j.rser.2017.01.097
Villano, M., Aulenta, F., Ciucci, C., Ferri, T., Giuliano, A., and Majone, M. (2010). Bioelectrochemical reduction of CO2 to CH4 via direct and indirect extracellular electron transfer by a hydrogenophilic methanogenic culture. Bioresour. Technol. 101, 3085–3090. doi: 10.1016/j.biortech.2009.12.077
Wagner, H.-J., and Mathur, J. (2011). Introduction to Hydro Energy Systems: Basics, Technology and Operation. Heidelberg; Dordrecht; London; New York, NY: Springer Science and Business Media.
Wang, H., Luo, H., Fallgren, P. H., Jin, S., and Ren, Z. J. (2015). Bioelectrochemical system platform for sustainable environmental remediation and energy generation. Biotechnol. Adv. 33, 317–334. doi: 10.1016/j.biotechadv.2015.04.003
Wang, X., Feng, Y., Liu, J., Lee, H., Li, C., Li, N., et al. (2010). Sequestration of CO2 discharged from anode by algal cathode in microbial carbon capture cells (MCCs). Biosens. Bioelectron. 25, 2639–2643. doi: 10.1016/j.bios.2010.04.036
Yaman, S. (2004). Pyrolysis of biomass to produce fuels and chemical feedstocks. Energy Conv. Manage. 45, 651–671. doi: 10.1016/S0196-8904(03)00177-8
Zaror, C., and Pyle, D. (1982). The pyrolysis of biomass: a general review. Proc. Indian Acad. Sci. Sect. C Eng. Sci. 5:269.
Zhang, Q., Chang, J., Wang, T., and Xu, Y. (2007). Review of biomass pyrolysis oil properties and upgrading research. Energy Conv. Manage. 48, 87–92. doi: 10.1016/j.enconman.2006.05.010
Zhao, H., Zhang, Y., Zhao, B., Chang, Y., and Li, Z. (2012). Electrochemical reduction of carbon dioxide in an MFC–MEC system with a layer-by-layer self-assembly carbon nanotube/cobalt phthalocyanine modified electrode. Environ. Sci. Technol. 46, 5198–5204. doi: 10.1021/es300186f
Zuberi, M. J. S., and Ali, S. F. (2015). Greenhouse effect reduction by recovering energy from waste landfills in Pakistan. Renew. Sustain. Energy Rev. 44, 117–131. doi: 10.1016/j.rser.2014.12.028
Zuberi, M. J. S., Hasany, S. Z., Tariq, M. A., and Fahrioglu, M. (2013). “Assessment of biomass energy resources potential in Pakistan for power generation,” in Power Engineering, Energy and Electrical Drives (POWERENG), 2013 Fourth International Conference on: 2013 (Istanbul: IEEE), 1301–1306. doi: 10.1109/PowerEng.2013.6635801
Keywords: biomass, bioenergy, pyrolysis, gasificaiton, energy, biochar
Citation: Saghir M, Zafar S, Tahir A, Ouadi M, Siddique B and Hornung A (2019) Unlocking the Potential of Biomass Energy in Pakistan. Front. Energy Res. 7:24. doi: 10.3389/fenrg.2019.00024
Received: 12 November 2018; Accepted: 14 February 2019;
Published: 22 March 2019.
Edited by:
Muhammad Raza Naqvi, Karlstad University, SwedenReviewed by:
Mohammad Zain Khan, Aligarh Muslim University, IndiaLucian Toma, Politehnica University of Bucharest, Romania
Copyright © 2019 Saghir, Zafar, Tahir, Ouadi, Siddique and Hornung. This is an open-access article distributed under the terms of the Creative Commons Attribution License (CC BY). The use, distribution or reproduction in other forums is permitted, provided the original author(s) and the copyright owner(s) are credited and that the original publication in this journal is cited, in accordance with accepted academic practice. No use, distribution or reproduction is permitted which does not comply with these terms.
*Correspondence: Muhammad Saghir, bS5zYWdoaXJAYmhhbS5hYy51aw==