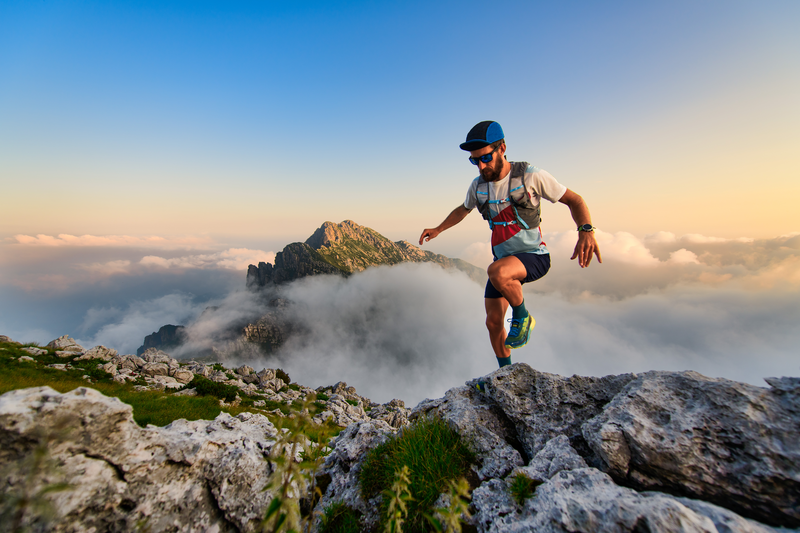
94% of researchers rate our articles as excellent or good
Learn more about the work of our research integrity team to safeguard the quality of each article we publish.
Find out more
ORIGINAL RESEARCH article
Front. Energy Res. , 07 March 2019
Sec. Indoor Environment
Volume 7 - 2019 | https://doi.org/10.3389/fenrg.2019.00009
This article is part of the Research Topic New Materials and Design of the Building Enclosure View all 7 articles
This study considers the applications of natural fiber composites in affordable housing projects located in the two districts of Gujarat, India, and Gorkha, Nepal, with the goal of addressing issues of thermal comfort and lateral loading from seismic activity. To help alleviate some of these issues, the team worked with the Hunnarshala Foundation, in Bhuj, India, to develop a fiber cement composite that could be applied to the interior or exterior of a home and provide both insulative and structural improvements. Thermal conductivity of the composites comprised of various mixes were measured using a heat flux machine. Experimental results of 0.16–0.19 W/m·K showed an improvement in thermal conductivity compared to current wall cladding materials ranging from 1.60 to 1.88 W/m·K. Modeling of the building envelope was completed using the EnergyPlus™ tool and showed that homes using the fiber cement composites would have an overall reduction in heating and cooling requirements. The study used coconut husk fibers (referred to as coconut coir), rattan, and pine wood fibers in addition to Portland cement, fly ash, and hydrated lime in the fabrication of fiber cement composite samples. Flexural strength of natural fiber samples was 70% stronger than comparison specimens, suggesting possible application for wall cladding to reduce out-of-plane failure during seismic events. Field tests conducted in Gujarat validated lab results as well as qualitative perceptions of the material. Continued work with local partners presents several possible applications of coconut coir fiber cement composites for retrofit and new construction housing.
Summer temperatures regularly reach 45°C in Gujarat, India, while in Gorkha, Nepal, the winter months of January and February experience a low of −2°C (Figure 1). Where India's per capita energy consumption is one-third of the global average, building-level energy consumption has been increasing at a rate of 4% per year, driven primarily by air conditioning and home appliances. With current building energy trends, these two extreme climates require better building envelopes with improved thermal performance thus reducing the need for fuel consuming heating and cooling systems. In 2001 and 2015, the two districts of Gujarat and Gorkha both experienced destructive earthquakes measuring 7.1 and 7.8 on the Richter scale, respectively. More than 20,000 were killed in the 2001 Gujarat earthquake (Phalkey et al., 2011), and more than 8,000 in the 2015 Nepal earthquake (World Health Organization, 2015).
Figure 1. Ahmedabad mortality rate during heat wave 2010 compared to previous year. Reproduced from Azhar et al. (2014).
Heat waves are the deadliest weather phenomenon in the world, accounting for 128,885 deaths worldwide between 2000 and 2015. Coping with these heat waves is significantly harder for poorer economies, such as India and Nepal, that do not have the economic means to react to these climatic events (Vaidyanathan, 2015). The average temperature during winter in Kathmandu, Nepal, is 3°C, making it difficult for families living in earth and brick masonry homes to stay warm with limited supplies of fuel. The loss of life in these countries from both strong seismic activity and extreme temperatures serves as an indicator of a need for safer, healthier buildings.
Based on the 2011 Indian Census, 87% of rural households use solid fuels for cooking, heating, and lighting, causing respiratory illnesses from exposure to incinerated waste products. Additionally, the non-sustainable harvesting of biomass fuels has created enormous environmental problems. In the context of both India and Nepal, these materials are outside of the vernacular building supply system and a core component of this research is the reliance on existing materials that are present in the above mentioned regions.
The building enclosure serves a vital role in protecting occupants from these hazards, and from the associated mortality. However, in these areas, social aspirations outweigh considerations for thermal comfort and structural stability at the time of purchase. To improve the status-quo, fiber-reinforced panels may help to address both the structural and thermal issues within a single application. Fiber-reinforced panels are composite materials made of a binder and strand fiber. Specifically, this paper discusses the use of naturally-derived fibers within a cementitious binder. This combination has several advantages, especially when applied in the cost-constrained target areas.
Previous studies have largely considered applications in new constructions and Olorunnisola (2007); Amaindamhen (2013); Adefisan et al. (2016) and Torkaman et al. (2014) have all published studies outlining methods for fabrication and testing of mechanical and physical properties of fiber cement composites in Africa, South Asia, and Latin America (Table 1). The research and testing procedures presented in this paper builds on a number of these studies and compares results from research carried out in labs around the world. The above mentioned publications have shown that reinforcing cement composites with lingocellulosic materials improved stiffness, strength to weight ratio, and thermal and acoustic resistance. This paper proposes a solution for building envelopes not previously explored by these journals through the use of waste materials for affordable housing in regions of extreme climates and earthquake hazards.
Hunnarshala is a design and construction firm team made of architects, technicians, artisans and business leaders. Tejas Kotak and Mahavir Acharya founded the company in 2003 as a response to the growing demand for affordable, more environmentally friendly building strategies. Hunnarshala considers construction waste to be a valuable asset and often looks for new ways of reintegrating off cut or disposed materials back into the material stream, used for the building of a wide variety of projects including weekend cottages, government housing, and primary schools. The team practices a number of sustainable building practices including the use of rammed earth walls, compressed stabilized earth blocks, space frame roofs, and low volume vaulting, all under the premise of reducing material waste and promoting local innovation.
Hunnarshala is based in Bhuj, India, a town prone to temperatures exceeding 45°C (Nelson, 2017). The quarter million people town, built on a seismic fault line, suffered a nearly 8.0 Richter scale earthquake in late January 2001 leaving hundreds dead and thousands homeless. The firm has made it its mission to address issues of safety in the application of new models of housing for the region.
Fiber cement composite samples were tested for thermal conductivity, compressive strength, and flexural strength to best understand their use in wall applications. Tests were either completed in the lab at the Massachusetts Institute of Technology in Cambridge, MA or on the campus of Hunnarshala in Bhuj, India.
Fiber cement samples were tested with a Netzsch Heat Flux Meter (HFM) 436 Lambda heat flow meter machine, National Institute of Standards and Technology (NIST) certified and ASTM C518-171 compliant for testing thermal conductivity of materials. To account for the varying surface of the cast samples, compressible pads of rubber were placed between panels and the upper hot plate and lower cold plate. Ten incrementally increasing temperature set-points were preprogrammed and allowed to run before reaching ±1°C equilibrium to find the average conductivity.
Flexural and compressive strength were tested on an MTS universal testing machine with a 100 kN load cell. Field tests were also conducted using five sample casts in India, and were then installed at a test location and metered for the collection of temperature data over a period of 4 months. Sample casts at Hunnarshala were also tested for compression and bending using an Akash Industries machine mounted with a 1,000 kN load cell.
Compressive strength was measured according to standards of ASTM C1092 for crushing concrete material samples. Flexural strength was tested using three-point bending test as defined by ASTM standard D1037-00. Values for the Modulus of Rupture for control samples ranged from 2.3 to 2.8 N/mm2 and for fiber cement samples from 1.2 to 4.6 N/mm2.
Ordinary Portland Cement (OPC) and hydrated lime used in the fabrication of panels were purchased in Cambridge, MA, or Bhuj, India. Prior to panel fabrication, a number of small sample batches were cast using varying ratios of water to cement and ultimately finished with an optimal ratio of 1 water to 2 binder by weight. Samples containing Coconut Husk (coir of Cocos nucifera) with a dry density of 209 kg/m3 used fiber purchased online and produced in the southern United States, while samples produced in Bhuj, India, were produced using fibers purchased from local suppliers.
For every fiber sample produced, a comparative non-fiber control was mixed and cast the same day using additional fine sand aggregate. The quantity of fiber for the samples was calculated at 5% of the total binder weight. Several mixes were tested using a range of binders including OPC, hydrated lime, fly ash, silica fume, and surkhi (Table 2). Surkhi is produced from the dust of fired, clay bricks, readily available in India. It provides additional strength to cement plasters and can be used in concrete mix as a replacement for sand.
Composite mixes, fiber volume, and fiber lengths were determined based on papers produced at the University of Ibadan (Olorunnisola, 2007; Adefisan et al., 2016). Coconut coir fiber was found to be one of the most effective fibers, and widely available in the studies regions and thus only results for samples containing coconut coir fiber were considered for this paper.
Composites produced at MIT (Massachusetts Institute of Technology) were cast in two wood molds lined with plastic and sprayed with corn vegetable oil to ensure delamination. The dry panels measured 300 × 300 × 3 mm (±5 mm) and the plastic cylinder molds measured 100 mm (±3 mm) in height and 50 mm (±2 mm) in diameter. Samples were covered with plastic and cured under a vent hood for 48 h, after which they were removed and left to cure for 26 days at a temperature of 23°C (±2°C).
Six of the panels fabricated with coconut husk fibers were used in the construction of two test walls built at Paraspar Nagar in Bhuj, India, a site owned by Hunnarshala and used as a location for testing other building materials and thermal insulation solutions (Figure 4). Panels were installed on an existing sand stone, perimeter fence wall, on a section rotated 16 degrees off an East-West axis (Figures 2, 3). The single wythe wall was built with sawn rectangular sandstone blocks, measuring 230 × 230 × 400 mm set into a 25 mm sand and clay mortar. The north side of the wall was chosen for the installation of the panels, and with this placement, the panels were in the shade at all times. To ensure heat flow from South to North, a 6.3 m3 area of the south face of the wall was painted with two coats of black oil paint.
Each test section, measuring 500 × 1,600 mm, was built with three fiber cement panels fixed to the existing sandstone wall on either a wood frame or a mortar bed. These two test sections, as well as a third control section, were connected to a series of thermocouples wired to HOBO data loggers. In addition to the collection of temperature data, the process of constructing the wall samples was useful in verifying the fabrication challenges and cost of material, giving a holistic understanding of the constraints and opportunities of this wall system.
All samples containing coconut coir fiber resulted in a thermal conductivity value below 0.20 W/m·K (Table 3). The coconut coir fiber panel, at 0.168 W/m·K, has also a lower conductivity when compared to the values from other comparable studies (Shawia et al., 2014; Wang et al., 2016). In addition, produced fiber cement composite samples were 30–40% less conductive than industry supplied fiber cement samples meeting EN 12467 standards (Fiber Cement Cladding Sheets | Cement Board Fabricators, 2012).
The fiber cement panel sample contained 4% coconut coir by weight (30% by volume) and is 7% less conductive than the control. While this is not a significant change in material conductivity from the control, the coconut coir in combination with hydrated lime and fly ash has more than half the thermal conductivity of standard OPC composites (0.37 W/m·K). Used in certain wall applications, these panels can provide a beneficial improvement for homes located in extreme climates and results from test assemblies are discussed further in the following section. Furthermore, the addition of coconut coir in the fiber cement panel had a positive impact on the bending strength of the panel and the results of structural tests (Brose, 2018).
Cylinders of the control sample, fabricated with OPC, fly ash, and hydrated lime, yielded under 89 kN, averaging 44 N/mm2 for all samples. Measured compressive strength of cylinders of the coconut coir Fiber mix were markedly lower with an average of 33 N/mm2 (Table 3). Panels containing coconut coir fiber at 30% by volume were found to be 74% stronger in bending than the control: 4.55 N/mm2 for samples containing coconut coir compared to 2.62 N/mm2 control samples.
Findings mirrored results presented in comparable publications in that fiber cement samples generally had a lower compressive strength than the control samples not containing any fiber (Adefisan et al., 2016). Other research studies using different agricultural fiber products have listed an average flexural strength of fiber cement composites of 3.4 N/mm2 (Adefisan et al., 2016), 5.6 N/mm2 (Shawia et al., 2014), and 5.9 N/mm2 (Olorunnisola, 2007). Results from compressive strength tests fall in the middle of the values presented in surveyed literature.
As discussed earlier, an additional advantage of the coconut fiber cement panels comes from the thermal benefits that they provide as building cladding. When utilized as a non-perforated panel, as in this research, the thermal benefits arise from two main avenues.
The first contribution of adding a non-perforated panel to the wall is the reduction of the air infiltration rate from the previously-unsealed facade. While this main avenue may be scoffed at with higher quality construction, this issue is non-negligible due to the large vacancies and cavities within the wall structure observed in finished homes in Nepal (Kongoletos, 2018). As existing assumptions based on ASHRAE publications are not applicable to Nepal, ongoing work from Kathmandu University is currently aiming to address this lack of data (Kuklov and Kongoletos, personal communication). To simplify the system amid this uncertainty, the team chose to neglect the infiltration contribution in the energy modeling. Setting aside the infiltration criteria allowed the team to consider the air gap created by the installation of the fiber panels on wood battens to be modeled as a closed volume and thus, as a single surface instead of as a dynamic layer. The team made this approximation using a table lookup for the thermal resistance of a three-centimeters thick plane air space (ASHRAE, 2017).
The second contribution of adding the fiber panel to the wall is the introduction of a low thermal conductivity surface which is akin to a low grade insulation. The team used the tested results from a Netzsch HFM 436 Lambda to determine the thermal conductivity near room temperature and then apply the as-tested conductivity to the energy model (Table 4).
For the geometry of the modeled structure, the team used the 2015 Nepal Housing Reconstruction Programme Design Catalog for Reconstruction of Earthquake Resilient Houses, Model SMC-2.3. This government publication was made available to increase the quality of homes in the wake of the 2015 Gorkha earthquake. The model that the team chose is a two-story home with 65.2 m2 of total floor area and an expected occupancy level of eight to ten people. It is built using locally available construction materials and the walls are composed of 45 cm thick stone masonry in cement mortar, and a roof constructed of coated, corrugated metal sheet. The team performed the energy simulation over a year period through EnergyPlus v8.4 using the ArchSim interface, which provided an aggregate of heating and cooling values on an annual basis.
As part of the EnergyPlus setup, the team assumed a family size of eight people living in the home, 10% of which were present from 8 a.m. to 8 p.m. and 100% of which were present from 8 p.m. to 8 a.m. The cooking stove was modeled as a uniform heat generation within the living space equivalent to a 1,500 W heater operated from 7:30 to 8:30 a.m. and 6:30 to 8 p.m. There were five LED bulbs modeled at 9 W each that operated from 6 to 10 p.m. The heat was set at 15°C and the cooling at 30°C, numbers which were chosen from local experiences in both Bhuj, India, and Kathmandu, Nepal. The team also used the associated weather files from these two cities to be indicators of hot climates (Bhuj, India) and cooler climates (Kathmandu, Nepal).
Between different runs of the simulation, the team applied the fiber panels to only the walls, leaving the rest of the structure and set-points unchanged between different model runs. Beginning with a baseline of solid sandstone block, the team first added the coconut coir fiber panel directly to the interior wall, and then also to the exterior wall. The fourth simulation was configured such that an air gap was present between the wall surface and the fiber panel. The representative equivalent conductivity of this configuration was taken from experimental tests presented in the 2017 ASHRAE Fundamentals. Simulations did not take into account any ventilation opportunities (including night-ventilation) and future studies would benefit from exploring these opportunities for further thermal comfort improvements.
The four types of wall structures were configured as follows, listed from the outside to the inside:
• 45 cm thick sandstone block.
• 45 cm thick sandstone block, 2 cm thick coconut coir fiber panel.
• 2 cm thick coconut coir fiber panel, 45 cm thick sandstone block, 2 cm thick coconut coir fiber panel.
• 45 cm thick sandstone block, 2 cm thick Air Gap, 2 cm thick coconut coir fiber panel.
Using this approach, the results from the EnergyPlus simulation are for representation purposes only and seek to identify the changes in heating and cooling energy resulting from a single change while keeping the remaining variables constant. Within an occupied home, this assumption would fall apart due to the natural differences in utilization of housing between different groups of people.
The results of the eight simulation runs are presented in the Table 5 and show that the simulation for Bhuj, India, predicted no heating requirement even for the base case. Although the outdoor ambient temperature did go below 15°C several times throughout the year, the thermal mass of the structure and internal heat gains from occupant and related heat loads removed the necessity for additional heating. However, in the cooling dominated climate of Bhuj, the additional insulation provided by the fiber panel helped save between 6 and 11% of annual cooling energy. Savings would be much higher if the wall insulation was a part of an enclosure that also included an improved roof system with an added air gap and thermal break.
In actual terms, the energy savings provided by applying a single layer of fiber panels on a wood battens setup (creating an air gap) saved the equivalent of 964 kWh over the duration of the year. For reference, the climate of Bhuj exceeds 40°C multiple times per year, meaning that the representative cooling to 30°C is not indicative of how a system would be run. It has been kept constant between the two locations for comparison purposes.
For Kathmandu, Nepal, the addition of insulation in the form of coconut coir fiber panels decreased energy usage for heating (Table 6), but increased the cooling energy loads as well. The same addition of coconut coir fiber panels on wood battens (creating an air gap) resulted in a 14% decrease in the heating energy loads, or the equivalent of 9 kWh. By adding insulation, however, the structure is less able to absorb heat loads within the thermal mass and release heat into the environment, increasing the cooling load significantly. While this model was constructed with pine shutters in place of windows, the pine shutters remained closed throughout the model and infiltration was neglected, meaning that the conclusion of heightened cooling usage may be inappropriate. The activation of thermal massing, inclusion of infiltration, and inclusions of appropriately modulated windows would help reduce this figure.
The addition of the coconut coir fiber panel decreases the energy consumption for the elements that are indicative of both climates; cooling in the hot Indian climate and heating in the cooler Nepali climate. These energy savings are of particular importance to carbon emissions as savings will directly translate into carbon emissions that would have been released. For the Nepali climate, the carbon is likely to be released from wood burned to heat the home, providing secondary benefits in reduced smog-forming emissions, and lower demand of deforestation. For the Indian climate, the carbon is likely to be released from local electrical fuel sources, which range widely from diesel to nuclear.
As further validation, temperature points were collected over 4 months from data loggers connected to the constructed wall samples in Bhuj, India. The south side of the wall receives solar energy and the temperature of the wall's north side gives an indication of overall heat flow through the wall system. The temperature data was confined to hourly averages for each thermocouple and collected at regular intervals to map heat flow through the sample wall section.
Results for the fiber cement panels installed on the mortar (Figure 5 “MP”) show a minor reduction in temperature difference between the face of the control panels and the face of the fiber cement panel. For the fiber cement panels installed on wood battens with an air gap between the panel face and the wall, the temperature difference between the face of the fiber cement panel (Figure 5 “AG—North Panel Exposed”) and the shaded face of the control (Figure 5 “Control—North Wall”) ranged from 0 to 7.3°C. Throughout the day, there was an average 3.5°C temperature difference between the air-gap panel face and the north face of the control wall. The temperature difference between panels mounted on the mortar bed (Figure 5 “MP—North Wall”) and the control (Figure 5 “Control—North Wall”) ranged from 0.1 to 4°C.
The results from the heat flow tests conducted in Bhuj, assuming steady state heat flow and ignoring convective cooling, show a reduction in internal heat gain in comparison to the control. While the addition of wood fibers to the composite does not reduce the conductivity by a large degree (0.01 W/m-K), mounted to a wood batten frame to provide an air gap, the wall assembly using fiber cement panels is 13% more effective than the sandstone wall. The fiber cement cladding mounted in such a wall envelope system reduces heat gain and reduces overheating hours by 3, as prescribed by the Indian Adaptive Comfort Model (Manu et al., 2016).
The median temperature of the control south wall hovered just below 34°C and changed by 3.3°C as it passed through 23 cm of sandstone (Figure 6 “Control Sample”). For the panels mounted on mortar, the temperature difference in total was 5.8°C and decreased at the greatest rate in the 2 cm thick earth mortar (Figure 6 “Mortar Sample”). A temperature difference of 7.4°C was measured in the air gap wall sample and on average the temperature dropped by 3.5°C through the air gap (Figure 6 “Air Gap Sample”).
There is a marked difference between the hours in the day when the air gap wall panel is more than 1°C cooler than the control (20 h) in comparison to the mortar gap panel (14 h). These results bring to light insights that, while not revolutionary, show that the inclusion of an air space in the wall envelope can have significant impact on the overall conductivity. The average temperature difference between the air gap panel face and the mortar face was almost doubled, indicating that the fiber cement panel installed over the air gap performed optimally out of the three conditions.
The results of the Bhuj wall assembly study show that there are benefits for the application of fiber cement panels in the wall envelop, either applied on a mortar bed or with a batten, air gap system.
The process of fabricating fiber cement panels revealed several opportunities for the use of reclaimed materials, helping to reduce the costs of building construction in low-income settings. The cost of materials and labor for samples made at the Hunnarshala campus was calculated to total between 60 INR and 70 INR (0.90–1.05 USD) per panel. The cost per m3 of 8,500 INR (128 USD) is roughly one-quarter the price of imported fiber cement panels manufactured and shipped from China. This price may be reduced even further through the replacement of Portland cement with other pozolonic binders.
The fabrication of samples and the assembly of wall prototypes with the Hunnarshala team provided a number of additional insights into methods of production not originally conceived in the project. A number of iterations required new ways of cutting and separating coconut husk fiber and the planning and cutting of waste wood. The addition of mechanical systems and thoughtful processes would help increase the speed and reduce the cost for fiber extraction. Compressing the fiber cement composites is an additional technique that would have added strength to the panels without requiring a great deal of upfront investment.
A 0.06 W/m2·K difference between the control samples and fiber samples is a small improvement. This wall envelope system allows for an air gap while providing a solid interior surface and can be adapted to a number of existing wall types. When used in a wood batten cladding system, results showed a reduction in indoor overheating by 30% and a decrease in max indoor temperature by 5°C. While it is still far from insulation values of most closed cell foams and soft woods cladding materials, combined with the increased flexural strength, it is a viable product solution for housing in regions of extreme climates with few options for insulative building materials.
It is important to consider the aesthetic and cultural appeal of these products in a local market. For this research, qualitative surveys were conducted in India and Nepal through a series of informal interviews with homeowners. Respondents were generally excited about the idea of affordable cladding materials for the exterior walls of their home. The primary desire was to have the ability to apply certain motifs and bright colors to the panels in order for the walls of their homes to both adhere to a common style and also represent a level of prestige through its customization.
The nature of organic fibers and the other elements used in the composites prohibits certain modifications or additions. In his own survey in Nigeria, Olorunnisola noticed that coloration difference with Calcium Chloride samples also decreased the attractiveness of panels made from fiber waste (Olorunnisola, 2007). Even with these challenges, making certain changes to the style and geometry of the panel to appeal to local design aesthetic is an attractive option and should be explored in greater detail.
The study used coconut coir in addition to OPC, fly ash, and hydrated lime in the fabrication of fiber cement composite samples. Flexural strength of natural fiber samples was 70% stronger than comparison specimens, suggesting possible applications for wall cladding for reduced out-of-plane failure during seismic events. Field tests conducted in Gujarat validated lab results as well as qualitative perceptions of the material. Continued work with local partners presents several possible applications of the fiber cement composites for the retrofitting of old building stock and construction of new affordable homes.
During earthquakes, walls overturning can cause significant injuries and death to building occupants. Often the lack of a tie structure, holding walls in plane, can lead to overturning of large wall sections, potentially landing on a person. Delamination of the building envelope from the primary structure can also be a hazard during significant earth movement. The use of materials with more ductile properties allows the wall to deform without failing. This gives occupants the opportunity to escape before a sudden failure and can potentially save many lives.
This paper proposed tests of composites made with organic fibers to increase both compressive and bending strength in order for the wall to perform more elastically. Results of these tests revealed higher flexural strength than their corresponding control sample and showed that the addition of wood waste fiber can improve the strength performance of cement composites. The studies performed assumed an idealized scenario in which the connection between masonry walls and the composite cladding sufficiently transfers load. Further testing is needed to identify the optimal mounting system for attaching the panels to the wall and to understand the flow of forces through the connections between cladding and wall.
Additional studies are necessary to ensure that panels made from Coconut Coir Fibers are waterproof and durable. It is also important to understand the hydrothermal effects within the wall assembly as it is probable that in colder climates, adding significant insulation to the walls will create an internal dew-point which may cause damage to the materials. New mix compositions and fabrication methods would yield further data to help develop even further improvements in the application of waste fiber materials for insulation and structural integrity to wall systems in material constrained settings.
The authors believe that both the thermal and strength characteristics of fiber cement panels produced with coconut coir fiber make it a relevant cladding application in Bhuj, India, and Gorkha District, Nepal. This paper proposed methods for increasing strength and reducing heat flow through wall cladding made with inexpensive, low carbon binders and waste wood fibers. Both lab and field testing show that the use of coconut coir in the production of fiber cement composites can improve indoor comfort as well as increase the strength of wall systems for buildings constructed in the extreme climates of Bhuj, India and Gorkha District, Nepal.
All authors listed have made a substantial, direct and intellectual contribution to the work, and approved it for publication.
We expect that all fees will be waved for this article as written to us by Dr. Biswas on September 8: We have received interests from some authors for our special issue of Frontiers in Energy Research. We have also been authorized by the publisher to offer full fee waivers to a limited number of authors.
The authors declare that the research was conducted in the absence of any commercial or financial relationships that could be construed as a potential conflict of interest.
John Ochsendorf at MIT served as advisor to the authors and provided support for the topic development and materials analysis. The Tata Center at MIT contributed funding for travel and research support for the duration of the project. Tejas Kotak, co-founder of Hunnarshala, was instrumental in the design and implementation of this research in Bhuj. Tejas is an advocate for the use of discarded materials and provided input in the sourcing and production of all materials used for the testing in India. Pradip Rangani is an engineer at Hunnarshala working on projects around India, for the 2018 Spring Pradip was tasked with the installation, metering, and testing of fiber cement panels.
1. ^ASTM C518-17. (2017). Standard Test Method for Steady-State Thermal Transmission Properties by Means of the Heat Flow Meter Apparatus. (West Conshohocken, PA: ASTM International) Available online at: www.astm.org
2. ^ASTM C109/C109M-16a. (2016). Standard Test Method for Compressive Strength of Hydraulic Cement Mortars (Using 2-in. or [50-mm] Cube Specimens). (West Conshohocken, PA: ASTM International) Available online at: www.astm.org
Adefisan, O. O., Fabiyi, J. S., and McDonald, A. G. (2016). Effects of rattan particle treatments on the strength and sorption properties of cement bonded composites. J. Bamboo Rattan 15, 1–12.
Amaindamhen, S. (2013). Technology and Market Opportunities in Fiber Cement Composites for Small Scale Enterprises in Nigeria. Glob. J. Agric. Sci. 12:1. doi: 10.4314/gjass.v12i1.2
American Society of Heating, Refrigerating, and Air-Conditioning Engineers. (2017). Pocket Guide for Air-Conditioning, Heating, Ventilation, and Refrigeration, 9th edn—SI (Atlanta).
Azhar, G. S., Mavalankar, D., Nori-Sarma, A., Rajiva, A., Dutta, P., Jaiswal, A., et al. (2014). Heat-related mortality in india: excess all-cause mortality associated with the 2010 ahmedabad heat wave. PLoS ONE 9:e91831. doi: 10.1371/journal.pone.0091831
Brose, A. (2018). Peripheral Timber: Applications for Waste Wood Material in Extreme Climates and Earthquake Risk Regions. Cambridge, MA: Massachusetts Institute of Technology.
Fiber Cement Cladding Sheets | Cement Board Fabricators, Inc. (2012). Cement Board Fabricators (blog). Available online at: https://cementboardfabricators.com/fiber-cement-cladding-sheets/ (Accessed November 6, 2012).
Kongoletos, J. (2018). Implementation and Evaluation of Thermal Avoidance Strategies in Arid, Cost-Constrained Climates Aimed at Improving Indoor Thermal Comfort: A Case Study in Bhuj, India. Cambridge, MA: Massachusetts Institute of Technology.
Manu, S., Shukla, Y., Rawal, R., Thomas, L. E., and de Dear, R. (2016). Field studies of thermal comfort across multiple climate zones for the subcontinent: India Model for Adaptive Comfort (IMAC). Build. Environ. 98, 55–70. doi: 10.1016/j.buildenv.2015.12.019
Nelson, E. (2017). Demonstration and Implementation of Thermally Passive Low-Income Housing : A Case Study in Bhuj. Thesis, Massachusetts Institute of Technology. Available online at: http://dspace.mit.edu/handle/1721.1/111707
Olorunnisola, A. O. (2007). Effects of particle geometry and chemical accelerator on strength properties of rattan–cement composites. Afr. J. Sci. Technol. 8, 22–27.
Phalkey, R., Reinhardt, J. D., and Marx, M. (2011). Injury epidemiology after the 2001 gujarat earthquake in India: a retrospective analysis of injuries treated at a rural hospital in the kutch district immediately after the disaster. Glob. Health Action 4:7196. doi: 10.3402/gha.v4i0.7196
Shawia, N. B., Jabber, M. A., and Mamouri, A. F. (2014). Mechanical and physical properties of natural fiber cement board for building partitions. Phys. Sci. Res. Int. 2, 49–53.
Torkaman, J., Ashori, A., and Momtazi, A. S. (2014). Using wood fiber waste, rice husk ash, and limestone powder waste as cement replacement materials for lightweight concrete blocks. Constr. Build. Mater. 50, 432–436. doi: 10.1016/j.conbuildmat.2013.09.044
Vaidyanathan, G. (2015). “Killer Heat Grows Hotter around the World.” Scientific American. Available online at: https://www.scientificamerican.com/article/killer-heat-grows-hotter-around-the-world/ (Accessed September 27, 2018).
Wang, L., Chen, S. S., Tsang, D. C.W., Poon, C. S., and Shih, K. (2016). Value-added recycling of construction waste wood into noise and thermal insulating cement-bonded particleboards. Constr. Build. Mater. 125, 316–325. doi: 10.1016/j.conbuildmat.2016.08.053
World Health Organization (2015). Humanitarian Crisis After the Nepal Earthquakes. Available online at: www.searo.who.int/entity/emergencies/phra_nepal_may2015.pdf?ua=1
Keywords: India, Nepal, earthquake (EQ), heating, cooling heating, building
Citation: Brose A, Kongoletos J and Glicksman L (2019) Coconut Fiber Cement Panels as Wall Insulation and Structural Diaphragm. Front. Energy Res. 7:9. doi: 10.3389/fenrg.2019.00009
Received: 01 October 2018; Accepted: 24 January 2019;
Published: 07 March 2019.
Edited by:
Kaushik Biswas, Oak Ridge National Laboratory (DOE), United StatesReviewed by:
Adrian Pitts, University of Huddersfield, United KingdomCopyright © 2019 Brose, Kongoletos and Glicksman. This is an open-access article distributed under the terms of the Creative Commons Attribution License (CC BY). The use, distribution or reproduction in other forums is permitted, provided the original author(s) and the copyright owner(s) are credited and that the original publication in this journal is cited, in accordance with accepted academic practice. No use, distribution or reproduction is permitted which does not comply with these terms.
*Correspondence: Andrew Brose, YWJyb3NlQG1pdC5lZHU=
Johnathan Kongoletos, amtvbmdvbGVAbWl0LmVkdQ==
Leon Glicksman, Z2xpY2tzQG1pdC5lZHU=
Disclaimer: All claims expressed in this article are solely those of the authors and do not necessarily represent those of their affiliated organizations, or those of the publisher, the editors and the reviewers. Any product that may be evaluated in this article or claim that may be made by its manufacturer is not guaranteed or endorsed by the publisher.
Research integrity at Frontiers
Learn more about the work of our research integrity team to safeguard the quality of each article we publish.