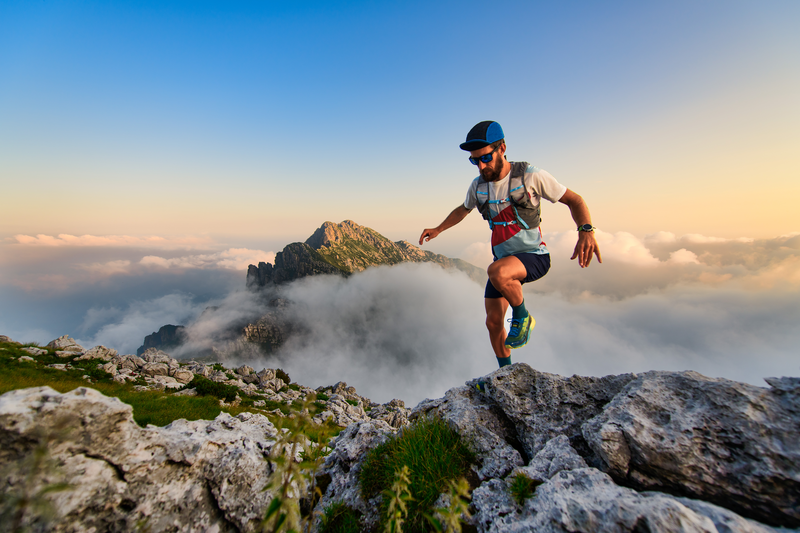
94% of researchers rate our articles as excellent or good
Learn more about the work of our research integrity team to safeguard the quality of each article we publish.
Find out more
EDITORIAL article
Front. Endocrinol. , 11 February 2025
Sec. Obesity
Volume 16 - 2025 | https://doi.org/10.3389/fendo.2025.1564003
This article is part of the Research Topic Lipid Metabolism Dysregulation in Obesity-Related Diseases and Neurodegeneration View all 7 articles
Editorial on the Research Topic
Lipid metabolism dysregulation in obesity-related diseases and neurodegeneration
Excessive lipid accumulation disrupts systemic homeostasis, contributing to conditions such as metabolic-associated steatotic liver disease (MASLD), diabetes, cardiovascular diseases, and neurodegenerative disorders (1–3). Lipid metabolism dysregulation, often associated with obesity, plays a pivotal role in metabolic disorders and their intricate interplay with neurological diseases. This body-brain interaction underscores the relationship between peripheral metabolic disturbances and central nervous system dysfunction (4, 5). As ectopic fat deposition, particularly in the liver, heightens systemic inflammation and metabolic stress, it also exacerbates neuroinflammatory pathways and intrinsically disordered protein aggregation (6–12), linking metabolic syndrome to conditions like Alzheimer’s disease (AD), Parkinson’s disease, and vascular dementia (Figure 1). Understanding these mechanisms provides a foundation for identifying potential prognostic markers or biomarkers and developing targeted therapeutic strategies that address the shared pathological processes of metabolic and neurological diseases, emphasizing the importance of holistic approaches to health management. This Research Topic highlights important insights into the heterogeneity of fat deposition in different organ tissues under obesity conditions, the association of high-density lipoprotein cholesterol (HDL-c) and non-HDL-c with risks of metabolic and neurological disorders, as well as providing translational insights into potential biomarkers and therapeutic targeting for obesity-related metabolic and neurological disorders that might be linked through body-brain interaction.
Figure 1. Peripheral metabolic dysfunction drives brain disorders through pathological body-brain interactions. Peripheral fat accumulation in organs such as the liver and pancreas leads to metabolic dysfunction, accompanied by the release of high-density lipoprotein (HDL) cholesterol particles and inflammatory cytokines. These changes not only affect other peripheral organs, such as the gut and cardiovascular system, but also propagate to the brain, contributing to neuroinflammation and neurodegeneration. (A) Differential contributions of peripheral fat deposition: Hepatic fat plays a more significant role than pancreatic fat in metabolic dysfunction. Hepatic fat accumulation is associated with increased fasting blood glucose, elevated insulin levels, and greater insulin resistance. (B) Metabolic dysfunction elevates the risk of brain disorders: An increased non high density lipoprotein cholesterol (non-HDL-c) to HDL-c ratio negatively impacts cerebral blood flow, exacerbates microvascular dysfunction, and increases stroke risk. (C) Biomarkers of metabolic dysfunction: Serum Aβ42 levels positively correlate with serum triglyceride levels and diastolic blood pressure, while negatively correlating with HDL-c levels. (D) Therapeutic strategies: Pharmacological interventions can be applied to target metabolic dysfunction and its associated impact on the brain through increasing lipid clearance, increasing metabolic activity, and reducing inflammation. Created in BioRender.
Deng et al. highlights the differential contributions of hepatic and pancreatic fat deposits to metabolic risks. This study underscores that liver fat plays a more significant role in influencing metabolic health compared to pancreatic fat. In obesity, ectopic fat accumulation disrupts normal organ functions, and the liver emerges as a dominant factor. Hepatic fat impacts glucose metabolism, lipid profiles, and systemic inflammation, contributing to conditions like insulin resistance and diabetes (Figure 1A). He et al. identifies the alanine aminotransferase (ALT) to HDL-c ratio as a potential indicator for diabetes risk in the Chinese population. A high ALT/HDL-c ratio correlates positively with the risk of diabetes development. This ratio reflects a significant association between liver function and metabolic health, as ALT levels are indicative of liver inflammation. ALT behaves similarly to non-HDL-c, which is a harmful lipid marker, highlighting the liver’s role as a major contributor to metabolic dysfunction. These studies emphasize the necessity of tailored strategies to mitigate liver-related metabolic risks, particularly in obesity management, where targeting liver fat reduction and addressing hepatic inflammation might yield more significant health benefits in metabolic disease interventions.
Hernandez Torres et al. examines the link between hyperlipidemia and increased microvascular dysfunction in mice, leading to reduced cerebral blood flow and impaired remote memory, which are key indicators of vascular dementia (Figure 1B). A Western diet high in cholesterol exacerbates heart-related issues, which cascade into brain complications. This research emphasizes the detrimental effects of non-HDL-c, a marker of cardiovascular risk, on brain health. Wang et al. explores the complex relationship between the non-HDL-c to HDL-c ratio and stroke risk in middle-aged and older adults (Figure 1B). This prospective cohort study demonstrates that a high non-HDL-c/HDL-c ratio is associated with increased stroke risk, particularly in the context of obesity, diabetes, and other co-variates. Interestingly, the study also identifies a non-linear relationship, indicating that stroke risk plateaus once the non-HDL-c/HDL-c ratio surpasses a certain threshold. These studies suggest that maintaining a balanced cholesterol profile is essential for vascular function, underscoring the need for early cholesterol management to preserve cardiovascular and brain health.
Li et al. investigates the correlation between serum amyloid beta 42 (Aβ42) levels with metabolic syndrome components, including obesity (Figure 1C). Their findings indicate that serum Aβ42 levels were positively associated with metabolic syndromes and related clinical parameters, such as body mass index and diastolic blood pressure, while showing a negative association with HDL-c levels in the Chinese population. While Aβ42 is traditionally linked to AD, this study shows that higher serum triglyceride levels contribute to elevated serum Aβ42, which could serve as a biomarker for metabolic syndromes rather than AD. Zeng et al. focuses on therapeutics that address peripheral inflammation and metabolic dysfunction as well as neuroinflammation and neurodegeneration driven by obesity and metabolic disorders (Figure 1D). This study presents a comprehensive approach to addressing the complex body-brain interaction by employing diverse therapeutic strategies. These include interventions to modify gut dysbiosis, lifestyle adjustments, dietary supplementation, and the use of pharmacological agents derived from natural sources, all aimed at mitigating obesity-induced neuroinflammation and neurodegeneration. These strategies prioritize restoring systemic metabolic balance to prevent inflammation that could propagate to neuroinflammation and drive neurodegeneration, highlighting the interplay between metabolic health and cognitive function.
The interplay between metabolic health and brain function is a recurring theme across these studies. Obesity, MASLD, diabetes, and lipid imbalances are central drivers of systemic inflammation, liver dysfunction, and vascular abnormalities, all of which have cascading effects on brain health. From the role of heterogenous peripheral fat deposition in metabolic risks to targeting the metabolic-inflammatory axis to mediate cognitive disorders, these findings illuminate the multifaceted connections between the body and the brain. Therapeutic interventions could adopt an integrated approach to address these interconnected systems. Managing liver health, controlling lipid profiles, and targeting inflammation are key steps in breaking the vicious cycle of metabolic and neurological decline (Figure 1D). Strategies involving the use of small molecules, nanoparticles, and advanced therapeutic modalities that target either the body, brain or body-brain interactions (13–16) hold significant potential to achieve these goals effectively. Integrative bioinformatics approaches could be applied to gain deeper insights into how different organs or tissues contribute to the metabolic-inflammatory axis (17–19). Understanding these changes may also facilitate the identification of biomarkers for metabolic syndromes and neurodegenerative diseases (20–22). This body-brain perspective not only deepens our understanding of disease mechanisms but also opens new avenues for preventative and therapeutic strategies, paving the way for comprehensive solutions to modern health challenges.
JZ: Conceptualization, Funding acquisition, Writing – original draft, Writing – review & editing. CL: Conceptualization, Funding acquisition, Writing – original draft, Writing – review & editing.
The author(s) declare financial support was received for the research, authorship, and/or publication of this article. CL is supported by a start-up grant from the Department of Biology at Syracuse University and JZ is supported by a start-up grant from the Department of Biomedical and Chemical Engineering at Syracuse University.
The authors declare that the research was conducted in the absence of any commercial or financial relationships that could be construed as a potential conflict of interest.
All claims expressed in this article are solely those of the authors and do not necessarily represent those of their affiliated organizations, or those of the publisher, the editors and the reviewers. Any product that may be evaluated in this article, or claim that may be made by its manufacturer, is not guaranteed or endorsed by the publisher.
1. Deprince A, Haas JT, Staels B. Dysregulated lipid metabolism links NAFLD to cardiovascular disease. Mol Metab. (2020) 42:101092. doi: 10.1016/j.molmet.2020.101092
2. Sourianarayanane A, Brydges CR, McCullough AJ. Liver tissue lipids in metabolic dysfunction-associated steatotic liver disease with diabetes and obesity. Clin Res Hepatol Gastroenterol. (2024) 48:102402. doi: 10.1016/j.clinre.2024.102402
3. Tong B, Ba Y, Li Z, Yang C, Su K, Qi H, et al. Targeting dysregulated lipid metabolism for the treatment of Alzheimer’s disease and Parkinson’s disease: Current advancements and future prospects. Neurobiol Dis. (2024) 196:106505. doi: 10.1016/j.nbd.2024.106505
4. Mikkelsen ACD, Kjærgaard K, Schapira AHV, Mookerjee RP, Thomsen KL. The liver-brain axis in metabolic dysfunction-associated steatotic liver disease. Lancet Gastroenterol Hepatol. (2024). doi: 10.1016/S2468-1253(24)00320-0
5. De Cól JP, de Lima EP, Pompeu FM, Cressoni Araújo A, de Alvares Goulart R, Bechara MD, et al. Underlying mechanisms behind the brain–gut–liver axis and metabolic-associated fatty liver disease (MAFLD): an update. Int J Mol Sci. (2024) 25:3694. doi: 10.3390/ijms25073694
6. Le Thuc O, García-Cáceres C. Obesity-induced inflammation: connecting the periphery to the brain. Nat Metab. (2024) 6:1237–52. doi: 10.1038/s42255-024-01079-8
7. Asimakidou E, Saipuljumri EN, Lo CH, Zeng J. Role of metabolic dysfunction and inflammation along the liver–brain axis in animal models with obesity-induced neurodegeneration. Neural Regener Res. (2025) 20:1069–76. doi: 10.4103/NRR.NRR-D-23-01770
8. Asimakidou E, Reynolds R, Barron AM, Lo CH. Autolysosomal acidification impairment as a mediator for TNFR1 induced neuronal necroptosis in Alzheimer’s disease. Neural Regener Res. (2024) 19:1869–70. doi: 10.4103/1673-5374.390979
9. Lo CH. Recent advances in cellular biosensor technology to investigate tau oligomerization. Bioeng Transl Med. (2021) 6:e10231. doi: 10.1002/btm2.10231
10. Lo CH. Heterogeneous tau oligomers as molecular targets for for Alzheimer’s disease and related tauopathies. Biophysica. (2022) p:440–51. doi: 10.3390/biophysica2040039
11. Liu S, Gao J, Zhu M, Liu K, Zhang H-L. Gut microbiota and dysbiosis in Alzheimer’s disease: implications for pathogenesis and treatment. Mol Neurobiol. (2020) 57:5026–43. doi: 10.1007/s12035-020-02073-3
12. Neto A, Fernandes A, Barateiro A. The complex relationship between obesity and neurodegenerative diseases: an updated review. Front Cell Neurosci. (2023) 17. doi: 10.3389/fncel.2023.1294420
13. Lo CH, O’Connor LM, Loi GWZ, Saipuljumri EN, Indajang J, Lopes KM, et al. Acidic nanoparticles restore lysosomal acidification and rescue metabolic dysfunction in pancreatic β-cells under lipotoxic conditions. ACS Nano. (2024) 18:15452–67. doi: 10.1021/acsnano.3c09206
14. Lo CH. TNF receptors: Structure-function relationships and therapeutic targeting strategies. Biochim Biophys Acta - Biomembr. (2025) 1867:184394. doi: 10.1016/j.bbamem.2024.184394
15. Zeng J, Loi GWZ, Saipuljumri EN, Romero Durán MA, Silva-García O, Perez-Aguilar JM, et al. Peptide-based allosteric inhibitor targets TNFR1 conformationally active region and disables receptor–ligand signaling complex. Proc Natl Acad Sci. (2024) 121:e2308132121. doi: 10.1073/pnas.2308132121
16. Asimakidou E, Tan JKS, Zeng J, Lo CH. Blood-brain barrier-targeting nanoparticles: biomaterial properties and biomedical applications in translational neuroscience. Pharm (Basel). (2024) 17:612. doi: 10.3390/ph17050612
17. Rodriguez-Muñoz A, Motahari-Rad H, Martin-Chaves L, Benitez-Porres J, Rodriguez-Capitan J, Gonzalez-Jimenez A, et al. A systematic review of proteomics in obesity: unpacking the molecular puzzle. Curr Obes Rep. (2024) 13:403–38. doi: 10.1007/s13679-024-00561-4
18. O’Connor LM, O’Connor BA, Bin LS, Zeng J, Lo CH. Integrative multi-omics and systems bioinformatics in translational neuroscience: A data mining perspective. J Pharm Anal. (2023) 13:836–50. doi: 10.1016/j.jpha.2023.06.011
19. O’Connor LM, O’Connor BA, Zeng J, Lo CH. Data mining of microarray datasets in translational neuroscience. Brain Sci. (2023) 13:1318. doi: 10.3390/brainsci13091318
20. Lo CH. Towards clinical translation of biomarkers and therapies targeting autolysosomal acidification dysfunction in neuroinflammation and neurodegeneration—an interview with Prof. David Rubinsztein Aging Pathobiol Ther. (2024) 6:138–40. doi: 10.31491/APT.2024.09.153
21. Pillai JA, Bena J, Bekris L, Kodur N, Kasumov T, Leverenz JB, et al. Metabolic syndrome biomarkers relate to rate of cognitive decline in MCI and dementia stages of Alzheimer’s disease. Alzheimers Res Ther. (2023) 15:54. doi: 10.1186/s13195-023-01203-y
Keywords: obesity, neurodegenerative diseases, body-brain interactions, metabolic dysfunction, neuroinflammation, therapeutic targets, biomarker discovery
Citation: Zeng J and Lo CH (2025) Editorial: Lipid metabolism dysregulation in obesity-related diseases and neurodegeneration. Front. Endocrinol. 16:1564003. doi: 10.3389/fendo.2025.1564003
Received: 20 January 2025; Accepted: 28 January 2025;
Published: 11 February 2025.
Edited and Reviewed by:
Katherine Samaras, St Vincent’s Hospital Sydney, AustraliaCopyright © 2025 Zeng and Lo. This is an open-access article distributed under the terms of the Creative Commons Attribution License (CC BY). The use, distribution or reproduction in other forums is permitted, provided the original author(s) and the copyright owner(s) are credited and that the original publication in this journal is cited, in accordance with accepted academic practice. No use, distribution or reproduction is permitted which does not comply with these terms.
*Correspondence: Jialiu Zeng, anplbmcyMkBzeXIuZWR1; Chih Hung Lo, Y2xvMTAxQHN5ci5lZHU=
†These authors have contributed equally to this work
Disclaimer: All claims expressed in this article are solely those of the authors and do not necessarily represent those of their affiliated organizations, or those of the publisher, the editors and the reviewers. Any product that may be evaluated in this article or claim that may be made by its manufacturer is not guaranteed or endorsed by the publisher.
Research integrity at Frontiers
Learn more about the work of our research integrity team to safeguard the quality of each article we publish.