- Department of Ophthalmology, Tianjin Medical University Baodi Hospital, Tianjin, China
Objective: To compare the clinical efficacy of subthreshold micropulse laser (SML) combined with anti-VEGF drugs versus anti-VEGF drugs monotherapy for diabetic macular edema (DME) through a meta-analysis.
Methods: We systematically reviewed relevant literature from electronic databases and extracted key outcomes, including best corrected visual acuity (BCVA)—comprising ETDRS and LogMAR measures, central macular thickness (CMT), annual frequency of anti-VEGF injections, annual SML applications, and associated complications for both treatment groups at postoperative intervals of 3, 6, 9, and 12 months.
Results: A total of 13 relevant studies were included in this review, including 405 eyes in the experimental group (SML combined with anti-VEGF drugs intravitreal injections) and 400 eyes in the control group (anti-VEGF drugs monotherapy). The results showed no significant difference in ETDRS visual acuity between the two groups at any time point (P > 0.05). However, LogMAR visual acuity significantly improved in the experimental group compared to controls at both 6 and 12 months post-treatment (P < 0.05). Subgroup analysis based on baseline CMT values indicated that patients with baseline CMT < 400 µm had significantly more reduced CMT across all observation points in the experimental group (P < 0.05). Conversely, no significant differences in CMT were found among those with baseline CMT ≥ 400 µm (P > 0.05). Additionally, annual intravitreal injection frequency of anti-VEGF drugs was significantly reduced in the experimental group compared to the controls (P < 0.05). The average numbers of SML applications per year ranged from 1.41 ± 0.37 to 3.4 ± 1.4 times (range: 1–4 times). Common postoperative complications included mild subconjunctival hemorrhage, a light ocular inflammatory reaction, and/or ocular surface discomfort.
Conclusion: Compared to anti-VEGF drugs monotherapy, combining SML with anti-VEGF drugs can improve visual acuity and reduce macular edema among DME patients—especially those with central macular thickness < 400 µm. The combined approach also reduces anti-VEGF drugs intravitreal injection frequency, and repeated use of SML can alleviate economic burdens on patients.
Systematic review registration: https://inplasy.com, identifier INPLASY2024120068.
1 Introduction
Diabetic macular edema (DME) is one of the most prevalent microvascular complications of diabetes. The incidence rates of DME and clinically significant macular edema (CSME) among individuals with diabetes (1) are 5.2% (3.1-7.9%) and 3.5% (1.9-6%), respectively, with projections indicating that the number of affected individuals worldwide will rise to 28.61 million by 2045 (2). DME can occur at any stage of diabetic retinopathy, impairing central vision and serving as a major cause of severe vision loss in working-age populations (2). In recent years, intravitreal injection of anti-vascular endothelial growth factor (Anti-VEGF) drugs has become the first-line treatment for diabetic macular edema (3). However, this therapy has a short duration of effect, high recurrence rates, and requires repeated injections, leading to significant economic burdens and poor patient compliance. Traditional management for DME involves retinal laser photocoagulation; although effective, this method can cause retinal tissue burns and visible retinal scars (4). In contrast to traditional continuous-wave lasers, subthreshold micropulse laser (SML) selectively targets cells of the retinal pigment epithelium (RPE), causing only sublethal damage to RPE, preserving photoreceptor cells and surrounding tissues. Consequently, this approach allows for the treatment of macular edema without noticeable retinal scars, minimizing adverse effects, and enabling multiple repeat treatments (5, 6). While the efficacy of anti-VEGF intravitreal injections remains exact, the majority of studies (7–9) suggest that combining anti-VEGF with SML is more effective than anti-VEGF monotherapy in improving visual acuity and reducing the number of injections required. Nevertheless, there are still sceptical voices that DME patients can’t benefit from the combination strategy (10). Moreover, the majority of existing studies are small-sample independent studies, which limits their potential to offer evidence-based medicine. Therefore, this study conducted a comprehensive systematic review and meta-analysis to compare the clinical efficacy of SML combined with anti-VEGF drugs versus anti-VEGF monotherapy in the treatment of DME.
2 Materials and methods
This systematic review was performed in accordance with the PRISMA protocol and was previously registered in the INPLASY database (https://inplasy.com/inplasy-2024-12-0068/) under the registration number INPLASY2024120068.
2.1 Literature screening
We conducted a search throughout multiple databases, including Pubmed, Embase, Cochrane, Web of Science, China Journal Full Text Database (CNKI), China Wipo Full Text Database (VIP), Wanfang Database, and China Biomedical Literature Database (CBM), without any language restrictions since the inception of these databases. At the same time, the references involved in the included literature were tracked, and grey literature was identified. The search terms and detailed search strategies are presented in the Supplementary Data Sheet 1 search strategy.
2.2 Criteria for studies selection
Inclusion Criteria 1) Randomized controlled trial or retrospective study; 2) The study was conducted in patients >18 years of age with DME, defined as macular edema involving the macular central fovea, with no restriction on age, race, sex or duration of disease; 3) The intervention was anti-VEGF drugs intravitreal injection combined with subthreshold micropulsed laser (SML) treatment in the experimental group, and anti-VEGF drug monotherapy (or combined with sham laser treatment) in the control group; 4) Reported outcomes included at least one of the following: best corrected visual acuity (BCVA), central macular thickness (CMT), the number of anti-VEGF agents injections per year, the number of SML annual applications, and associated complications; 5) Papers reported at least one of the above outcomes at baseline (0) and at 3, 6, 9 and 12 months after treatment.
Exclusion Criteria 1) Patients who had received panretinal photocoagulation, SML, vitreous anti-VEGF injection, and any other intraocular surgeries other than cataract surgery in the previous 3 months were excluded; 2) The subjects were non-DME patients (e.g., macular edema due to other sources such as retinal vein occlusion) or fluorescein fundus angiography (FFA) examination suggestive of macular ischemia; 3) The interventions in the experimental group were either SML treatment alone or anti-VEGF drugs intravitreal injections combinated with non-SML treatment (e.g., combined with conventional retinal laser); 4) Animal experiments, case reports, conference papers, reviews, duplicate publications, literature from which full text was not available or from which original data could not be extracted.
2.3 Data extraction and quality assessment
The following data were extracted: 1) Author name, publication year; 2) General information about the subjects (age, sample size, glycated hemoglobin A1c (HbA1c), etc.); 3) Type of study, interventions, follow-up time, and outcome indicators. Continuous variables are presented as mean ± standard deviation (x ± s). All data collection and extraction in this paper were done independently by two researchers, and in a case of disagreement, the decision could be made through consultation or with the assistance of a third researcher.
A risk of bias assessment was undertaken for randomized controlled studies using the Cochrane Risk of Bias 2 (RoB 2) tool and for retrospective studies with the Cochrane Risk of Bias in Non-randomized Studies of Interventions (ROBINS-I) tool. Two researchers conducted the risk of bias evaluation separately, providing a rating to each item within each domain as either ‘low’, ‘some concerns/moderate’, or ‘high’ risk. The overall all of these factors resulted in the evaluation of overall bias. Any disagreement on ratings was discussed and resolved with a third reviewer.
2.4 Statistical analysis
Meta-analysis was completed using Revman 5.3 and Stata SE15 software provided by the Cochrane Collaboration. Since the included studies had inconsistent indicator measures or units, standardized mean difference (SMD) was served as the effect size, and each effect size was given its 95% confidence interval (95% Cl). Heterogeneity between studies was assessed using Cochran’s Q test and I2 statistic. When P > 0.1 and I2 < 50%, it was considered that there was no statistically significant heterogeneity between studies, and statistical analysis was performed using a fixed-effect model. When P < 0.1, I2 ≥ 50%, then it was considered that there was a statistical heterogeneity. A meta-regression analysis was taken to attempt to figure out the clinical reasons for the heterogeneity, and a subgroup analysis was performed when necessary. If no obvious reasons for clinical heterogeneity were found, Galbraith star plots were created, and a fixed-effect model was employed after eliminating the literature with large heterogeneity, or a random-effect model was adopted. Sensitivity analyses were performed using a one-by-one exclusion method. Finally, the Begg’s test for publication bias was performed.
3 Results
3.1 Literature search
A total of 1046 documents were identified by searching the database using the appropriate search strategy. 13 documents were finally included after screening, including 11 randomized clinical trials and 2 retrospective studies. There were 405 eyes in the experimental group (anti-VEGF drugs combined with SML) and 400 eyes in the control group (anti-VEGF drugs alone). There was no statistically significant difference in the baseline BCVA, baseline CMT, and baseline HbA1c levels between the two groups (all P > 0.05). However, the experimental group was older than the controls (SMD = 0.23, fixed-effect model, P = 0.002 < 0.05), which was statistically significant. The search process and results are displayed in Figure 1, and the basic characteristics of the included studies are provided in Tables 1, 2.
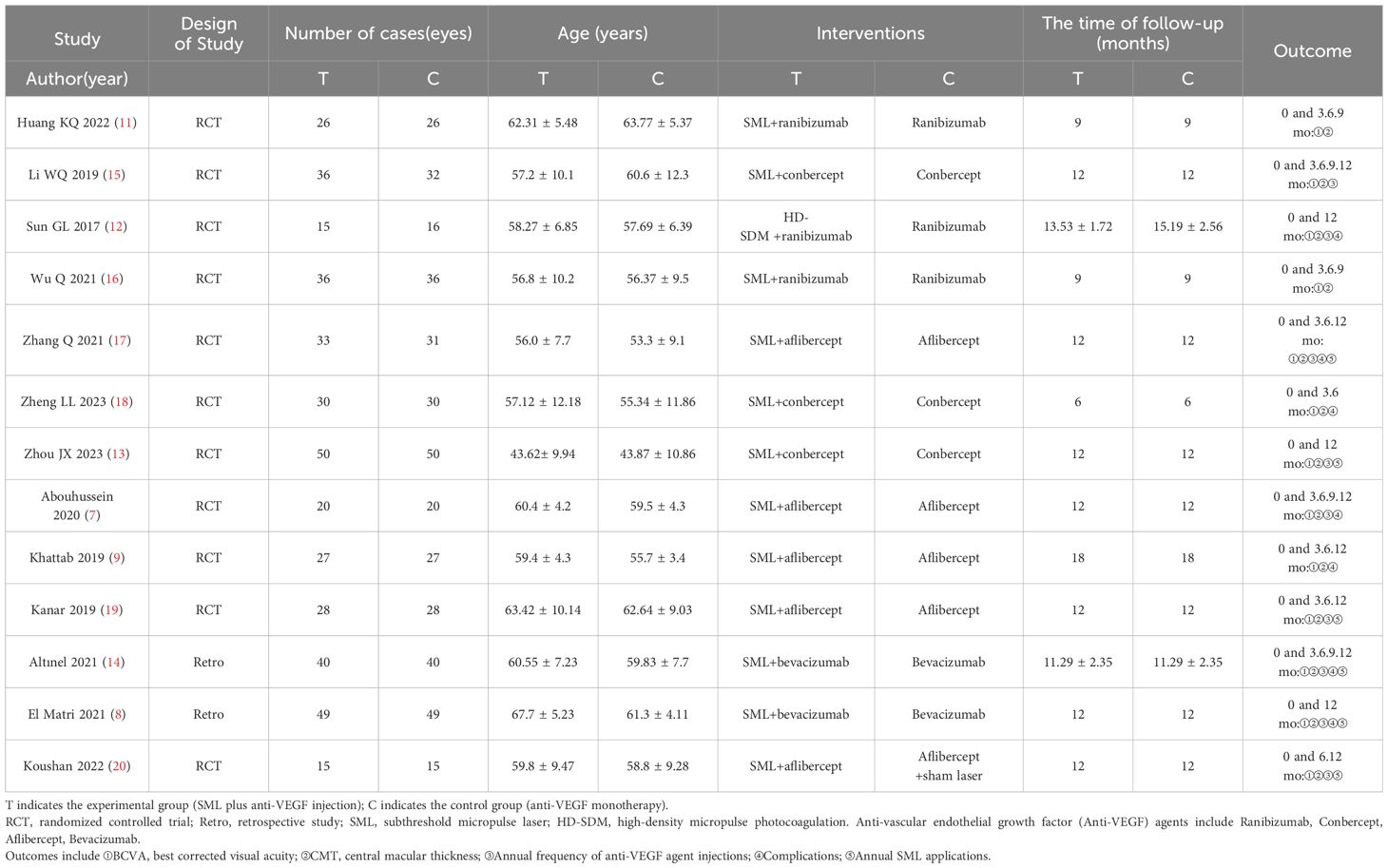
Table 1. Basic characteristics of included literature (1).
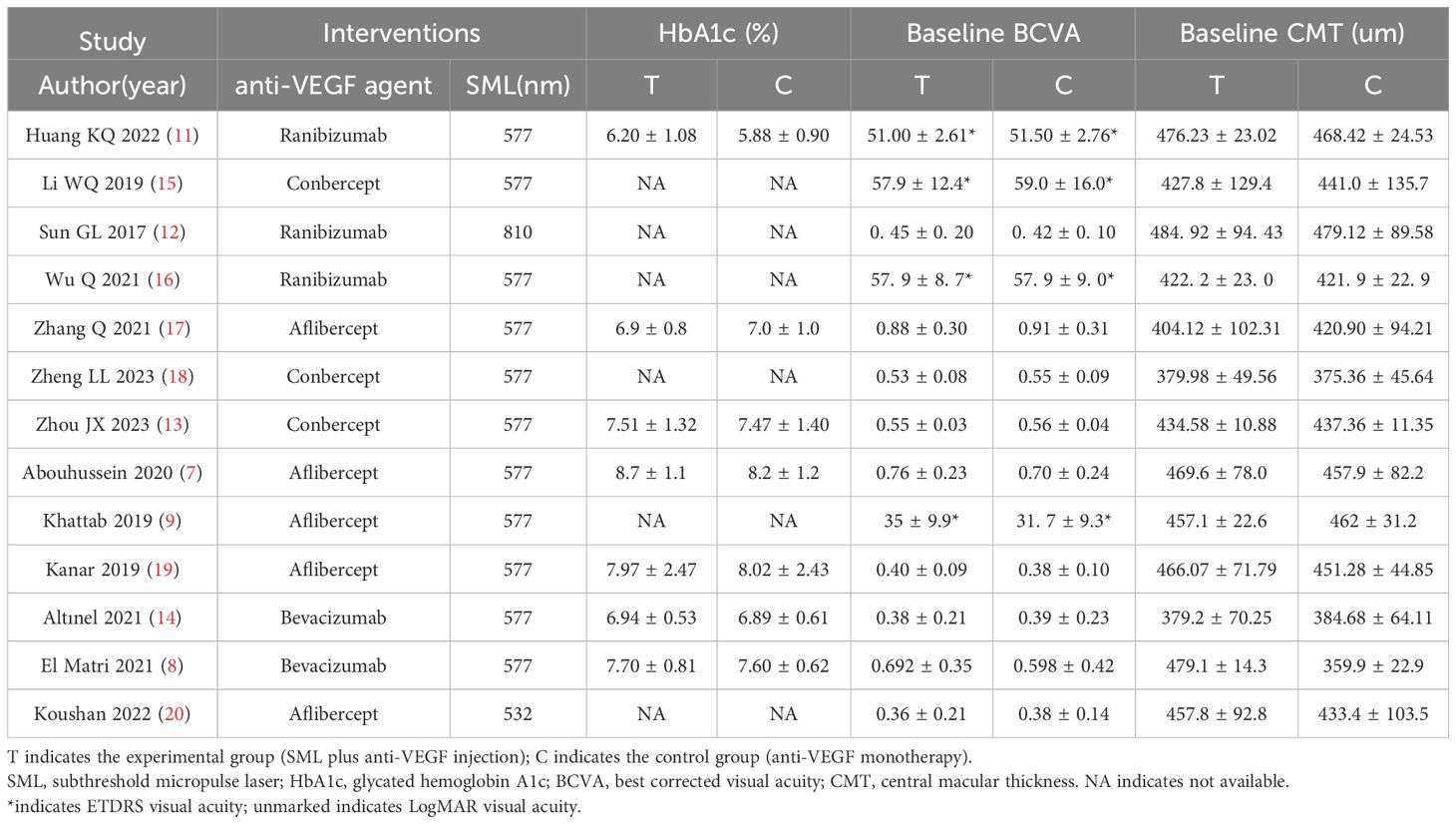
Table 2. Basic characteristics of included literature (2).
3.2 Quality evaluation
Of these 11 randomized controlled trials, RoB2 assessments yield four studies (7, 11–13) recognized as having a lack of allocation concealment during randomization, while one research (9) indicated probable discrepancies in baseline data between the two groups post-randomization. Two retrospective studies (8, 14) were assessed by the ROBINS-I tool. Both studies were classified as ‘moderate risk’, mostly due to the absence of significant confounding information in the original papers, such as systemic comorbidities and the severity of diabetic retinopathy. This may give rise to worries about the possibility of confounding bias. A summary of the risk of bias assessments for the randomized trials (Figure 2) and the retrospective investigations (Figure 3) is presented, respectively.
3.3 Meta-analysis
3.3.1 Best corrected visual acuity (BCVA)
The BCVA of the 13 papers included in the current study comprised 4 studies for ETDRS visual acuity (9, 11, 15, 16), and 9 articles for LogMAR visual acuity (7, 8, 12–14, 17–20).
3.3.1.1 ETDRS visual acuity
The heterogeneity test showed I2 = 0.0% (< 50%) and P = 0.733 (> 0.1) for Cochran’s Q test, indicating that there was no heterogeneity between the included literature. A fixed-effect model was selected for the meta-analysis. Overall, the results indicated that the visual acuity of the experimental group was superior to that of the control group, but with no statistically significant difference (SMD = 0.1; 0.95% Cl: -0.04, 0.24; P = 0.154 > 0.05). Subgroup analyses were conducted at various observation times, revealing that ETDRS visual acuity improved in the experimental group compared to the controls at different time points; however, the difference was not statistically significant (P = 0.669, 0.378, 0.498, and 0.338 for the 3, 6, 9, and 12 months, respectively) (Table 3).
3.3.1.2 LogMAR visual acuity
The heterogeneity test indicated I2 = 55.0% (> 50%) and P = 0.001 (< 0.1) for Cochran’s Q test, suggesting the presence of heterogeneity among the literature selected for this study. Consequently, we performed a meta-regression analysis based on sample size (n < 30 vs. n ≥ 30), baseline central macular thickness (CMT ≥ 400 µm vs. CMT < 400 µm), and SML laser type (577 nm laser vs. others), revealing that those variables did not account for the observed heterogeneity (all P > 0.05). Furthermore, a Galbraith star plot was created (Figure 4). This figure illustrates that the heterogeneity of two studies, Zheng LL (2023) and Abouhussein (2020), is significant. Following the exclusion of these publications, the results of the meta-analysis covering a total of seven studies (8, 12–14, 17, 19, 20) revealed I2 = 0.00% (< 50%) and P = 0.784 (> 0.1) for Cochran’s Q test; effect sizes were subsequently combined using a fixed-effect model. Overall, our findings demonstrated that LogMAR visual acuity in the experimental group was superior to that in the controls, with a statistically significant difference (SMD = -0.19; 95% CI: -0.32, -0.07; P = 0.003 < 0.05). Subgroup analysis conducted at different time intervals showed that the experimental group achieved improved LogMAR visual acuity compared to the control group at both 6 and 12 months, with statistically significant differences (P = 0.026 and 0.041 for 6 and 12 months, respectively) (Table 4).
3.3.2 Central macular thickness
The heterogeneity test displayed I2 = 42.1% (< 50%) but P = 0.0061 (< 0.1) for Cochran’s Q test, indicating significant heterogeneity among the included studies. We then conducted a meta-regression analysis, accounting for the sample size, baseline CMT, and the laser type of SML. The results demonstrated that neither sample size nor SML laser type (both P > 0.05) contributed to the observed heterogeneity. However, baseline CMT emerged as a significant factor (P = 0.000 < 0.05) influencing this variability.
Participants were classified into two subgroups based on the baseline CMT (CMT ≥ 400 µm and CMT < 400 µm). In the subgroup with baseline CMT < 400µm, the CMT value was consistently and significantly reduced in the experimental group compared to the control group at each observation time point (P = 0.024, 0.003, 0.031, and 0.011 for the 3, 6, 9, and 12 months, respectively). In contrast, no significant differences in the CMT levels were observed between the two groups at any time point for the subgroup with baseline CMT ≥ 400 µm (P = 0.406, 0.673, 0.468, and 0.056 for the 3, 6, 9, and 12 months, respectively). The results are summarized in Figures 5A–D.
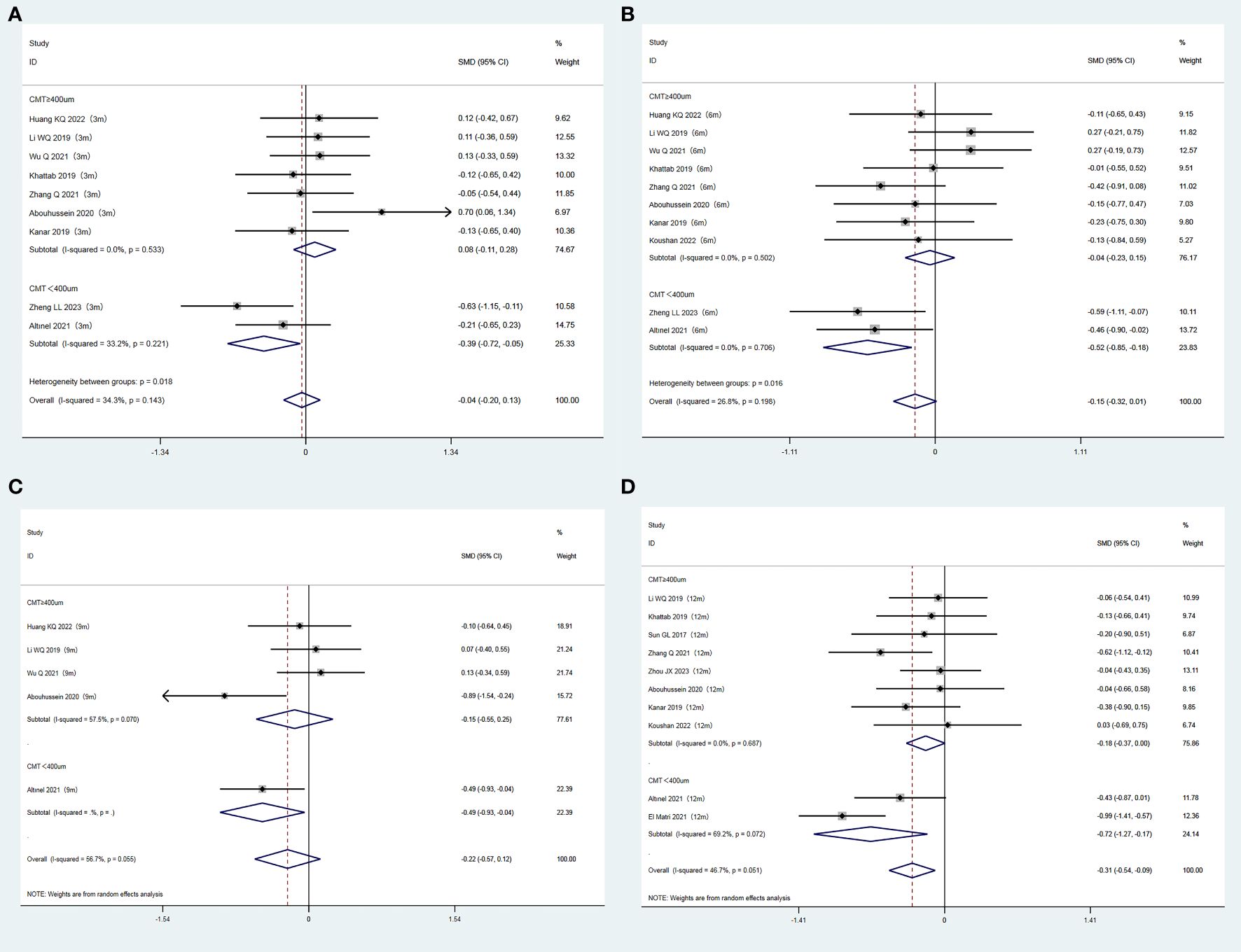
Figure 5. (A) Forest plots comparing subgroups (CMT ≥ 400 um/CMT < 400 um) for CMT at 3 months after treatment in the experimental and control groups (Fixed-effect model). (B) Forest plots comparing subgroups (CMT ≥ 400 um/CMT < 400 um) for CMT at 6 months after treatment in the experimental and control groups (Fixed-effect model). (C) Forest plots comparing subgroups (CMT ≥ 400 um/CMT < 400 um) for CMT at 9 months after treatment in the experimental and control groups (Random-effect model). (D) Forest plots comparing subgroups (CMT ≥ 400 um/CMT < 400 um) for CMT at 12 months after treatment in the experimental and control groups (Random-effect model).
3.3.3 The annual frequency of anti-VEGF drugs injections
In this investigation, a total of 9 publications (7, 8, 12–15, 17, 19, 20) reported the annual frequency of vitreous anti-VEGF drugs injections. Following the heterogeneity test, I² was judged to be 80.3%, showing high heterogeneity among the included studies (P = 0.000 < 0.1 from Cochran’s Q test). We took a meta-regression analysis considering sample size, CMT, and SML laser types and indicated that none of these parameters significantly contributed to the heterogeneity (all P > 0.05). A Galbraith star diagram was later constructed, and the findings are given in Figure 6. Particularly, investigations by Sun GL (2017), Abouhussein (2020), El Matri (2021), and Koushan (2022) demonstrated high heterogeneity. After eliminating these articles, a meta-analysis was undertaken on 5 remaining studies (13–15, 17, 19). The heterogeneity test gave I² = 37.8% (< 50%) with P = 0.169 (> 0.1), thus we proceeded with a combined effect size estimate using a fixed-effect model. Overall, the frequency of annual vitreous anti-VEGF drugs injections in the experimental group was considerably lower than that in the control group (SMD = -1.32; 95% Cl: -1.54, -1.09; P = 0.000 < 0.05) (Figure 7).
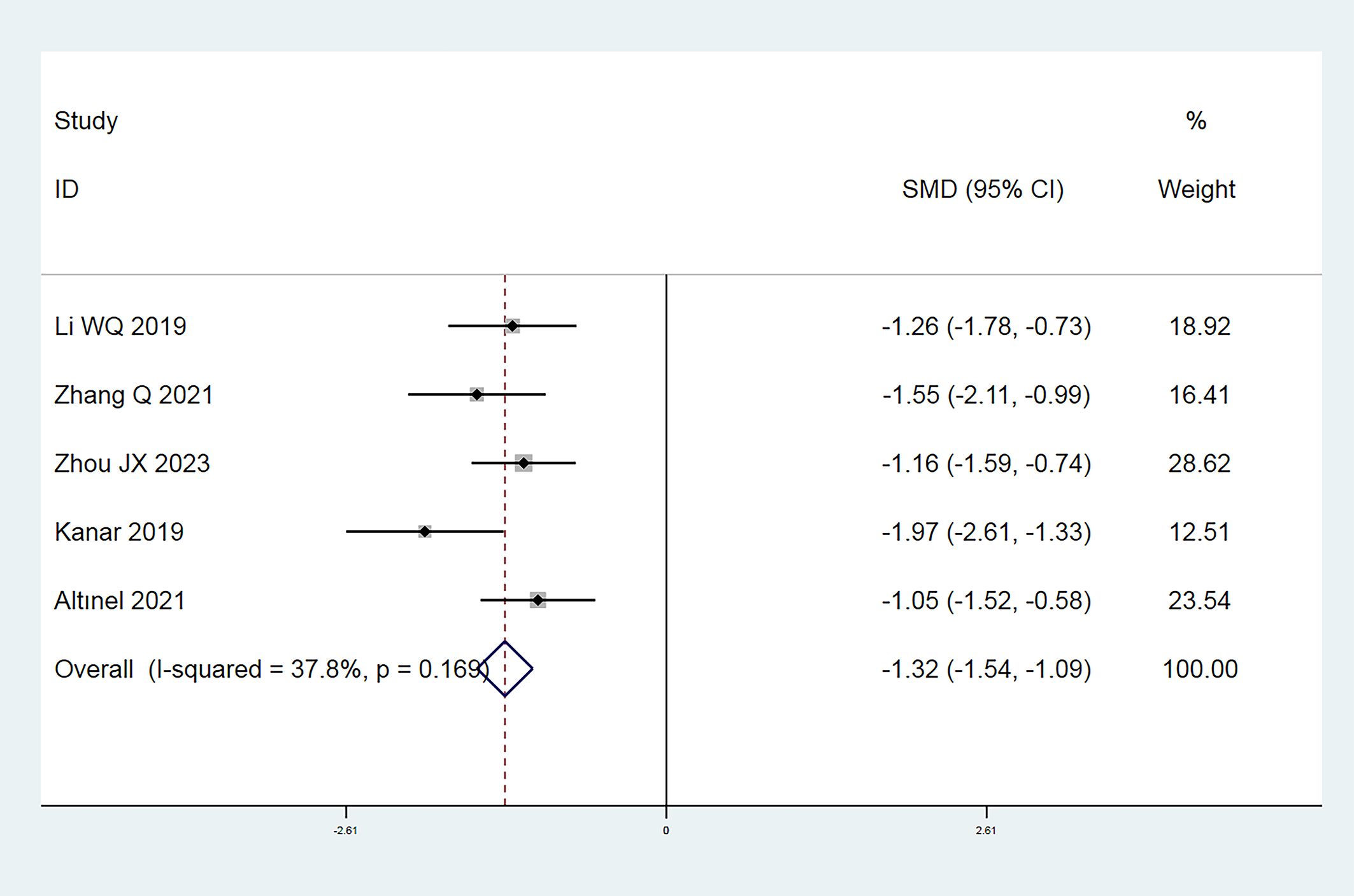
Figure 7. Forest plot comparing the number of annual anti-VEGF drugs injections in the experimental and control groups (Fixed-effect model).
3.3.4 The annual frequency of SML applications
Six research papers (8, 13, 14, 17, 19, 20) tracked the annual frequency of SML applications within the combined group. The experimental designs vary across investigations (refer to Supplementary Data Sheet 2 for detailed methodologies). Most studies adopted a threshold-based strategy in which the experimental group was monitored monthly following the initial SML treatment combined with vitreous injection of anti-VEGF agents (once a month for a total of 3 doses as a loading dose). SML should be re-administered if the loss of vision and/or the change of CMT rose above the defined threshold value, but this intervention could not occur earlier than 2 or 3 months after the prior SML treatment. The results showed that an average annual SML application frequency ranged from 1.41 ± 0.37 to 3.4 ± 1.4 times (range: 1-4 times).
3.3.5 Complications
A total of seven articles (7–9, 12, 14, 17, 18) reported complications in our research. Three papers (12, 14, 17) observed no ocular or systemic complications during the follow-up. Two papers (7, 8) reported that there were no injection-related complications apart from mild subconjunctival hemorrhage at the injection site. No significant SML laser scars were observed in the combined treatment group. One study (9) described a mild inflammatory reaction in the eye postoperatively, which could subside within a week with topical steroid drops. Only one paper (18) provided data on the incidence of complications, which were eye pain, dry eye, elevated intraocular pressure, and foreign body sensation. However, the total incidence was not statistically different between the two groups (X2 = 0.185, P = 0.667).
3.4 Sensitivity analysis
Sensitivity evaluations were conducted for three outcome indicators including BCVA (ETDRS visual acuity and LogMAR visual acuity), CMT, and the frequency of annual vitreous anti-VEGF drugs injections using the one-by-one exclusion method. The results showed that the exclusion of any one piece of literature would not cause significant interference to this meta-analysis, indicating that our study has good stability (Supplementary Data Sheet 3).
3.5 Publication bias test
A Begg’s test was conducted with Stata SE15 software. The results revealed that there was no publication bias for the outcome measures of BCVA (ETDRS visual acuity and LogMAR visual acuity), CMT, and the annual frequency of anti-VEGF drugs injections (Table 5).
4 Discussion
Diabetic macular edema (DME) is the primary cause of visual impairment for diabetic patients. Vitreous injection of anti-VEGF drugs is currently the first-line treatment to control DME. However, this drug has a short half-life, requires multiple injections to maintain efficacy, and is expensive, thereby increasing the financial burden on patients. In addition, recurrent vitreous injections may carry certain risks, including cardiovascular and cerebrovascular accidents, as well as ocular complications such as glaucoma, cataracts, retinal detachment, endophthalmitis, and others (21). Therefore, reducing the frequency of injection and minimizing the complications on the basis of ensuring the efficacy is an urgent problem to be solved at present. A number of scholars (22–24) have advised the use of anti-VEGF drugs intravitreal injection combined with retinal laser photocoagulation as a treatment for DME. This strategy has been demonstrated to considerably reduce macular edema and improve postoperative visual acuity, while also reducing the amount of vitreous injections necessary (25). It is important to note that traditional photocoagulation treatment still involves a destructive process on the retina. This process can result in a number of adverse effects, including permanent loss of photoreceptors, choroidal neovascularization, and subretinal fibrosis (26). Different from the traditional retinal laser photocoagulation, in which the laser action time is equal to the whole exposure time, the subthreshold micropulse laser (SML) consists of a series of complete and repeatable high-frequency pulses, and due to the small duty cycle and long intervals between the pulses, the laser energy cannot be accumulated continuously, so the damage to the retina caused by subthreshold micropulse lasers is limited. What’s more, the heat effect it created is only limited to the retinal pigment epithelial (RPE) layer with no visible retinal laser spot (6), thus reducing the burn injury on the retinal neurosensory layer and deep choroidal capillaries (12). In addition, the SML can produce biomodulatory effects on the RPE cells. The SML promotes the expression of heat shock protein 70 (HSP70) in the RPE cells (27), which in turn folds the damaged RPE cells, prevents apoptosis, and blocks inflammatory pathways. This contributes to the remodeling of the RPE cells and accelerates the absorption of macular edema. Furthermore, micropulse laser treatment has been demonstrated to reduce the expression of various growth factors, including vascular endothelial growth factor (VEGF), transforming growth factor-β (TGF-β), pigment epithelium-derived factor (PEDF), and basic fibroblast growth factor (bFGF). As well as, the SML contributes to the upregulation of angiogenesis inhibitors, which in turn inhibit retinal neovascularization (28).
Our research indicates that in the LogMAR visual acuity group, postoperative visual acuity improved in the combination therapy group compared to the monotherapy group at 6 and 12 months and the annual frequency of vitreous injections was significantly decreased in the combination group. As anti-VEGF drugs have the capacity to rapidly reduce macular edema, the implementation of micropulse laser treatment in most trials occurred within 1-2 weeks after vitreous injection of anti-VEGF drugs. At the moment, the reduction of central macular thickness avoids the formation of a barrier owing to fluid accumulation in the macula, which was more favorable to the micropulse laser’s direct action on the RPE cells (15). In addition, vitreous injection of anti-VEGF drugs can reduce vascularization, leakage, edema, and inflammation after photocoagulation, minimizing retinal damage from the SML laser (23, 29). Attention is drawn to the fact that anti-VEGF drugs have the disadvantage of a short duration of action, thus requiring repeated injections to maintain efficacy, whereas micropulse lasers may take several months to show therapeutic effects (30), thereby combining the two methods to achieve a complementary advantage. What’s more, the cost of one SML session is roughly $60, but the cost of one vitreous anti-VEGF drug injection is approximately $430, and the combination of them considerably decreases the financial burden of patients.
Unfortunately, in the ETDRS visual acuity group, there was no statistically significant difference for BCVA between the two groups. The ETDRS visual acuity chart is a standardized version based on the design of the LogMAR principles, with each line of letters corresponding to a change in visual acuity of 0.1 log units. ETDRS visual acuity measurement is standardized by controlling the test distance (31, 32), density of letter arrangement (33), standardization of illumination, etc. Compared with conventional LogMAR visual acuity, ETDRS visual acuity can control the measurement error within ± 0.02 logMAR (two lines of letters) (34) and is more sensitive. What’s more, although the difference was not statistically significant, we also observed an improvement of ETDRS visual acuity in the combination therapy group compared to the monotherapy group at all observation time points.
It is documented that the impact of micropulse laser is closely related to central macular thickness (CMT) (11, 15), and the efficacy of micropulse laser monotherapy in eyes with CMT > 400 μm is inferior. Therefore, combining micropulse laser with anti-VEGF drugs injections is required (35), which is consistent with the findings of our study. Based on clinical observation, when macular oedema is more severe, the distribution of micropulsed laser energy is more diffused in the targeted tissues, which makes it difficult to penetrate the edema to operate on the RPE cells, and thus the efficacy of the treatment is not satisfactory (13).
At present, primarily two kinds of lasers are employed for clinical treatment: the 577 nm yellow laser and the 810 nm near-infrared laser. Both lasers are suitable for diabetic macular edema. The 810-nm SML has an effect on melanocytes in both the RPE layer and choroid. In contrast, the 577-nm SML has a more targeted effect, especially affecting the melanocytes in the RPE layer. The 577-nm laser has the advantage of scattering slightly less than the 810-nm laser, allowing for more focused energy in the RPE layer, which lessens the laser strength and shortens pulse duration (36). Furthermore, since the absorption spectrum of lutein does not contain 577 nm yellow light, 577 nm SML can be employed in close proximity to the central concave of the macula, making 577 nm laser the favored option (37). Although there is a bit of research on the 810 nm near-infrared laser, recent findings indicate that at a duty cycle of 5%, the two lasers have comparable therapeutic efficacy (36, 38). The results of our study reveal that the type of laser employed does not alter the change of central macular thickness. However, the lack of non-577 nm laser literature may introduce a publication bias. Therefore, further investigation is required to confirm whether laser type could affect therapeutic efficacy.
The adverse effects of combination therapy appear to be more mild in the current research, with the most common postoperative complications including mild subconjunctival hemorrhage, a light ocular inflammatory reaction, and/or ocular surface discomfort.
5 Limitations
Some important limitations should be considered. First, the baseline data revealed a discrepancy in age between the experimental and control groups. Although age is a relevant factor in the development of DME (39, 40), it has been demonstrated in the study by Zhu TT et al. (41) that age is not an independent risk factor for the prognosis of DME treated with conbercept by a multifactorial logistic regression analysis. Ayumi et al. (42) investigated the factors associated with the efficacy of aflibercept or ranibizumab in treating DME. They also failed to find a statistically significant difference between the age of the good response group (> 20% decrease in CMT) and the poor response group (≤ 20% decrease in CMT) (P = 0.061). In addition, the study by Alshalan et al. (43) showed that although there were differences between the mean improvement in BCVA and the mean change in the central subfield thickness (CST) decreased with DME patients of different ages (age ≤ 60 years vs. age > 60 years) treated with anti-VEGF agents, the differences were not statistically significant (P = 0.5429, 0.08, respectively). Therefore, we believe that the difference in baseline age may not have a major impact on outcome measures such as CMT and visual acuity between the two groups. Second, due to missing or incomplete information in the literature, the clinical heterogeneity sources were not detected for certain outcome indicators. Factors such as the severity of diabetic retinopathy (44), lens status (45), and other factors may contribute to the clinical heterogeneity. In the future, we need to include as much literature as possible and find out the sources of clinical heterogeneity.
6 Conclusion
In conclusion, we suggest that subthreshold micropulse laser (SML) combined with vitreous anti-VEGF drugs injections can significantly reduce macular edema and improve visual acuity in patients of DME, especially in those with CMT < 400 um. In addition, combined therapy can significantly reduce the frequency of anti-VEGF drugs injections with fewer postoperative complications, and SML can be repeated, which significantly reduces the economic burden on patients.
Author contributions
YJ: Conceptualization, Data curation, Formal Analysis, Investigation, Methodology, Software, Writing – original draft. WH: Data curation, Methodology, Supervision, Validation, Writing – review & editing. SQ: Data curation, Methodology, Project administration, Writing – review & editing.
Funding
The author(s) declare that no financial support was received for the research and/or publication of this article.
Conflict of interest
The authors declare that the research was conducted in the absence of any commercial or financial relationships that could be construed as a potential conflict of interest.
Generative AI statement
The author(s) declare that no Generative AI was used in the creation of this manuscript.
Publisher’s note
All claims expressed in this article are solely those of the authors and do not necessarily represent those of their affiliated organizations, or those of the publisher, the editors and the reviewers. Any product that may be evaluated in this article, or claim that may be made by its manufacturer, is not guaranteed or endorsed by the publisher.
Supplementary material
The Supplementary Material for this article can be found online at: https://www.frontiersin.org/articles/10.3389/fendo.2025.1553311/full#supplementary-material
References
1. Fundus Disease Group of Ophthalmological Society of Chinese Medical Association. Guidelines for clinical diagnosis and treatment of diabetic retinopathy in China. Chin J Ophthalmol. (2014) 50:851–65. doi: 10.3760/cma.j.issn.0412-4081.2014.11.014
2. Teo ZL, Tham Y-C, Yu M, Chee ML, Rim TH, Cheung N, et al. Global prevalence of diabetic retinopathy and projection of burden through 2045. Ophthalmology. (2021) 128:1580–91. doi: 10.1016/j.ophtha.2021.04.027
3. Wong TY, Sun J, Kawasaki R, Ruamviboonsuk P, Gupta N, Lansingh VC, et al. Guidelines on diabetic eye care. Ophthalmology. (2018) 125:1608–22. doi: 10.1016/j.ophtha.2018.04.007
4. Brader HS, Young LHY. Subthreshold diode micropulse laser: A review. Semin Ophthalmol. (2016) 31:30–9. doi: 10.3109/08820538.2015.1114837
5. Shi YY, Wu JH, Li ZH. Advanced in the treatment of diabetic macular edema. Med J Wuhan University. (2018) 39:323–32. doi: 10.14188/j.1671-8852.2017.0601
6. Tao Y, Jiang HT, Liu JY. Effect of micro-pulse laser at 577nm threshold in patients with diabetic macular edema. Int Eye Sci. (2019) 19:821–5. doi: 10.3980/j.issn.1672-5123.2019.5.26
7. Abouhussein MA, Gomaa AR. Aflibercept plus micropulse laser versus aflibercept monotherapy for diabetic macular edema: 1-year results of a randomized clinical trial. Int Ophthalmol. (2020) 40:1147–54. doi: 10.1007/s10792-019-01280-9
8. El Matri L, Chebil A, El Matri K, Falfoul Y, Chebbi Z. Subthreshold micropulse laser adjuvant to therapeutic advances in ophthalmologybevacizumab versus bevacizumab monotherapy in treating diabetic macular edema: One- year- follow-up. Ther Adv Ophthalmol. (2021) 13:1–12. doi: 10.1177/25158414211040887
9. Khattab AM, Hagras SM, AbdElhamid A, Torky MA, Awad EA, Abdelhameed AG. Aflibercept with adjuvant micropulsed yellow laser versus aflibercept monotherapy in diabetic macular edema. Graefe’s Arch Clin Exp Ophthalmol. (2019) 257:1373–80. doi: 10.1007/s00417-019-04355-6
10. Tatsumi T, Takatsuna Y, Oshitari T, Kaiho T, Kawasaki Y, Shiko Y, et al. Randomized clinical trial comparing intravitreal aflibercept combined with subthreshold laser to intravitreal aflibercept monotherapy for diabetic macular edema. Sci Rep. (2022) 12:10672. doi: 10.1038/s41598-022-14444-y
11. Huang KQ, Liu LH, Li M, Zeng SM, Wu XJ, Zhong HB, et al. Clinical effect of ranibizumab combined with 577nm micropulse laser in the treatment of severe diabetic macular edema. Int Eye Sci. (2022) 22:1377–80. doi: 10.3980/j.issn.1672-5123.2022.8.27
12. Sun GL, Jiang J, Wang CH, Zhang S, Yao J. High-density micropulse photocoagulation combined with intravitreal injection of ranibizumab for diabetic macular edema. Rec Adv Ophthalmol. (2017) 37:297–81. doi: 10.13389/j.cnki.rao.2017.0070
13. Zhou JX, Zhang JH. Observation on the efficacy of conbercept combined with subthreshold micropulsed laser photocoagulation in the treatment of diabetic macular edema. Chin J Ocul Fundus Dis. (2023) 39:132–6. doi: 10.3760/cma.j.cn511434-20221222-00667
14. Altınel MG, Acikalin B, Alis MG, Demir G, Mutibayraktaroglu KM, Totuk OMG, et al. Comparison of the efficacy and safety of anti-vegf monotherapy versus anti-vegf therapy combined with subthreshold micropulse laser therapy for diabetic macular edema. Lasers Med Sci. (2021) 36:1545–53. doi: 10.1007/s10103-021-03306-0
15. Li WQ, Song YP, Ding Q. The effect of conbercept combined with 577 nm subthreshold micropulse laser photocoagulation on diabetic macular edema. Chin J Ocul Fundus Dis. (2019) 35:129–34. doi: 10.3760/cma.j.issn.1005-1015.2019.02.005
16. Wu Q, Wang Y, Jiao J. Effect of ranibizumab injection combined and 577 nm micropulse laser photocoagulation on cmt and tmv of patients with diabetic macular edema. Chin J Laser Med Surg. (2021) 30:155–60. doi: 10.13480/j.issn1003-9430.2021.0155
17. Zhang Q, Duan YP, Jin ZQ. Effect of aflibercept combined with sub-threshold micropulse laser in the treatment of 30 cases with diabetic macular edema. Herald Med. (2021) 40:631–4. doi: 10.3870/j.issn.1004-0781.2021.05.010
18. Zheng LL, Mao YJ, Zheng CF, Chen DB. Observation on the efficacy of conbercept combined with subthreshold micropulsed laser photocoagulation in the treatment of diabetic macular edema. Contemp Med. (2023) 39:42–5. doi: 10.3969/j.issn.1009-4393.2023.31.011
19. Kanar H, Arsan A, Altun A, Akı S, Hacısalihoglu A. Can subthreshold micropulse yellow laser treatment change the anti-vascular endothelial growth factor algorithm in diabetic macular edema? A randomized clinical trial. Indian J Ophthalmol. (2019) 68:145–51. doi: 10.4103/ijo.IJO_350_19
20. Koushan K, Eshtiaghi A, Fung P, Berger AR, Chow DR. Treatment of diabetic macular edema with aflibercept and micropulse laser (dam study). Clin Ophthalmol. (2022) 16:1109–15. doi: 10.2147/opth.S360869
21. Chen HX, Cleck JN. Adverse effects of anticancer agents that target the vegf pathway. Nat Rev Clin Oncol. (2009) 6:465–77. doi: 10.1038/nrclinonc.2009.94
22. Lei JY, Zhao X, Yin SD, Li S, Liu YQ, Wang H. Effect of anti-vegf combined with laser photocoagulation on diabetic macular edema in elderly. Geriatrics Res. (2021) 2:1–4. doi: 10.3969/j.issn.2096-9058.2021.04.001
23. Chen J, Lai MY, Luo H, She JT. Clinical study on anti-vegf plus photocoagulation for diabetic macular edema. Chin J Pract Ophthalmol. (2014) 32:693–7. doi: 10.3760/cma.j.issn.1006-4443.2014.06.006
24. Liu LL, Liao HL, Wang LY, Zheng XJ. Effectiveness and safety of conbercept and macular local/grid laser photocoagulation for diabetic macular edema: A meta-analysis. J Guangdong Med University. (2023) 41:58–63. doi: 10.3969/j.issn.1005-4057.2023.01.012
25. Dong M, Chen S. Research progress of ranibizumab in the treatment of diabetic macular edema. Chin J Pract Ophthalmol. (2014) 32:686–9. doi: 10.3760/cma.j.issn.1006-4443.2014.06.004
26. Schatz H, Madeira D, McDonald HR, Johnson RN. Progressive enlargement of laser scars following grid laser photocoagulation for diffuse diabetic macular edema. Arch Ophthalmol. (1991) 109:1549–51. doi: 10.1097/00006324-199112000-00014
27. Inagaki K, Shuo T, Katakura K, Ebihara N, Murakami A, Ohkoshi K. Sublethal photothermal stimulation with a micropulse laser induces heat shock protein expression in arpe-19 cells. J Ophthalmol. (2015) 2015:1–9. doi: 10.1155/2015/729792
28. Li ZY, Song YP, Chen X, Chen ZS, Ding Q. Biological modulation of mouse rpe cells in response to subthreshold diode micropulse laser treatment. Cell Biochem Biophys. (2015) 73:545–52. doi: 10.1007/s12013-015-0675-8
29. Ding GP, Ding GL, Lei S, Shan WQ, Xie GJ, Ding XJ. Clinical effect of conbercept intravitreal injection combined with 577nm micro-pulse laser on the treatment of diabetic macular edema. Int Eye Sci. (2015) 15:1942–4. doi: 10.3980/j.issn.1672-5123.2015
30. Chen GH, Radouil T, Li WS, Jiang FZ, Mao SH, Tong YH. Subthreshold micropulse diode laser versus conventional laser photocoagulation for diabetic macular edema: A meta-analysis of randomized controlled trial. Retina. (2016) 36:2059–65. doi: 10.1097/IAE.0000000000001053
31. Qi T, Liu LQ. The principles and utilization of etdrs chart and the comparison with snellen chart. Chin J Optom Ophthalmol Vis Sci. (2013) 15:633–6. doi: 10.3760/cma.j.issn.1674-845X.2013.10.014
32. Ferris FL, Kassoff A, Bresnick GH, Bailey I. New visual acuity charts for clinical research. Am J Ophthalmology. (1982) 94:91–6. doi: 10.1016/0002-9394(82)90197-0
33. li H, Xiao MY. Comparison of international standard and diabetic retinopathy visual acuity chart in amblyopic children. Chin J Pract Ophthalmol. (2014) 32:26–31. doi: 10.3760/cma.j.issn.1006-4443.2014.01.007
34. Rosser DA, Cousens SN, Murdoch IE, Fitzke FW, Laidlaw DAH. How sensitive to clinical change are etdrs logmar visual acuity measurements? Invest Opthalmology Visual Science. (2003) 44:3278–81. doi: 10.1167/iovs.02-1100
35. Mansouri A, Sampat KM, Malik KJ, Steiner JN, Glaser BM. Efficacy of subthreshold micropulse laser in the treatment of diabetic macular edema is influenced by pre-treatment central foveal thickness. Eye. (2014) 28:1418–24. doi: 10.1038/eye.2014.264
36. Vujosevic S, Martini F, Longhin E, Convento E, Cavarzeran F, Midena E. Subthreshold micropulse yellow laser versus subthreshold micropulse infrared laser in center-involving diabetic macular edema: Morphologic and functional safety. Retina. (2015) 35:1594–603. doi: 10.1097/IAE.0000000000000521
37. Zhao HK, Zou Y, Li YQ. Process in applicat ion of subthreshold micropulse laser. Int Eye Sci. (2020) 20:92–5. doi: 10.3980/j.issn.1672-5123.2020.1.21
38. Chang DB, Luttrull JK. Comparison of subthreshold 577 and 810 nm micropulse laser effects on heat-shock protein activation kinetics: Implications for treatment efficacy and safety. Trans Vis Sci Tech. (2020) 9:23. doi: 10.1167/tvst.9.5.23
39. Taouk Y, Fenwick EK. Relative importance and contribiton of risk factors for diabetic retinopathy and macular edema. J Diabetes Metab. (2014) 5. doi: 10.4172/2155-6156.1000337
40. Klein R, Klein BEK, Moss SE, Davis MD, DeMets DL. The wisconsin epidemiologic study of diabetic retinopathy. Ophthalmology. (1984) 91:1464–74. doi: 10.1016/s0161-6420(84)34102-1
41. Zhu TT, Wang Q, Zhao R, Liu Y, Chen W. Influencing factors on clinical response to conbercept for diabetic macular edema. Chin J Ocul Fundus Dis. (2018) 34:436–42. doi: 10.3760/cma.j.issn.1005-1015.2018.05.005
42. Usui-Ouchi A, Tamaki A, Sakanishi Y, Tamaki K, Mashimo K, Sakuma T, et al. Factors affecting a short-term response to anti-vegf therapy in diabetic macular edema. Life. (2021) 11:83. doi: 10.3390/life11020083
43. Alshalan HA, Arevalo JF, Alomary SI, Ardah HI, Hazzazi MA. Effect of age on response to anti-vegf agents in patients with center involving diabetic macular edema in a tertiary hospital. Int J Retina Vitreous. (2022) 8:84. doi: 10.1186/s40942-022-00434-9
44. Yang LX, DongZuo H, Xv X, Liu F. Prevalence of diabetic macular edema and its related factors. J Shanghai Jiaotong Univ Med Sci. (2012) 32:160–7. doi: 10.3969/j.issn.1674-8115.2012.02.008
Keywords: diabetic macular edema, subthreshold micropulse laser, anti-VEGF drugs, combination therapy, meta-analysis
Citation: Jiang Y, He W and Qi S (2025) Evaluating the efficacy of subthreshold micropulse laser combined with anti-VEGF drugs in the treatment of diabetic macular edema: a systematic review and meta-analysis. Front. Endocrinol. 16:1553311. doi: 10.3389/fendo.2025.1553311
Received: 30 December 2024; Accepted: 10 March 2025;
Published: 28 March 2025.
Edited by:
Martina Tomić, Merkur University Hospital, CroatiaReviewed by:
James David Adams, Independent Researcher, Benicia, CA, United StatesXiao Chen, General Hospital of Central Theater Command, China
Copyright © 2025 Jiang, He and Qi. This is an open-access article distributed under the terms of the Creative Commons Attribution License (CC BY). The use, distribution or reproduction in other forums is permitted, provided the original author(s) and the copyright owner(s) are credited and that the original publication in this journal is cited, in accordance with accepted academic practice. No use, distribution or reproduction is permitted which does not comply with these terms.
*Correspondence: Shixin Qi, cXNoeGluQDEyNi5jb20=
†These authors have contributed equally to this work and share first authorship