- 1Department of Orthopaedics, The First Affiliated Hospital of Dalian Medical University, Dalian, China
- 2Department of Pharmacy, The Third People’s Hospital of Dalian, Dalian, China
- 3Key Laboratory of Molecular Mechanism for Repair and Remodeling of Orthopaedic Diseases, Dalian, Liaoning, China
Serum urate (SU) levels are significantly elevated in conditions such as gout, type 2 diabetes (T2D), obesity, and other metabolic syndromes. Recently, due to the high prevalence of hyperuricemia (HUA), numerous clinical connections between SU and musculoskeletal disorders like sarcopenia, osteoarthritis (OA), rheumatoid arthritis (RA), intervertebral disc degeneration (IDD), and osteoporosis (OP) have been identified. This review discusses the mechanisms linking SU to musculoskeletal disorders, as well as the clinical associations of SU with conditions such as sarcopenia, T2D with sarcopenia, McArdle disease, heart failure, gout, OA, IDD, OP and exercise-induced acute kidney injury (EIAKI), offering valuable insights for improved prevention and treatment strategies. Mechanisms linking SU to musculoskeletal disorders include oxidative stress, MSU (monosodium urate) crystal deposition, inflammation, and other factors. In adults, both age and SU levels should be considered for preventing sarcopenia, while gender and SU may directly impact muscle mass in children and adolescents. HUA and gout may be risk factors for OA progression, although some reports suggest otherwise. A U-shaped relationship between SU and IDD has been reported, particularly in Chinese men, indicating lower or higher SU level may be risk factors for IDD. Maintaining SU levels within a certain range may help prevent OP and fractures. Future research, including epidemiological studies and new pathogenesis findings, will further clarify the relationship between musculoskeletal diseases and SU.
1 Introduction
Serum urate (SU) levels are known to be significantly elevated in conditions such as gout, type 2 diabetes (T2D), obesity, and other metabolic syndromes (1). It is widely recognized that elevated SU, which is the end product of purine metabolism in humans and other higher primates (2), can pose health risks and lead to adverse outcomes, potentially even death (3). Factors contributing to high SU levels include excessive intake of purine-rich foods, alcohol, and fructose, as well as abnormal purine metabolism and/or reduced renal and intestinal SU excretion (4, 5). When SU concentration exceeds a certain threshold, it is diagnosed as hyperuricemia (HUA) (>7.0 mg/dL in men and >6.0 mg/dL in women). According to a 2015-2016 American epidemiological survey, the prevalence of HUA was 20.2% in men and 20.0% in women, totaling 22.8 million and 24.4 million individuals, respectively (5, 6). In China, the largest developing country, the prevalence of HUA among adults was 8.4% from 2009 to 2010 (7), with a prevalence of 6.4% in middle-aged and older adults according to the CHARLS 2011 survey (8).
Unregulated HUA has been linked to various health conditions such as renal disease (1, 9–13), cardiovascular disease (CVD) (1, 11, 14–16), hypertension (10, 17), T2D (1, 18–20), diabetic kidney disease (21), and others, all of which are associated with increased morbidity and mortality (3). In the musculoskeletal system, on the one hand, persistent presence of supersaturated level of SU can lead to the formation of monosodium urate (MSU) crystals in and around synovial joints, causing severe sterile inflammation/pain known as gout (22). The prevalence of HUA has led to the clinical connections between SU and musculoskeletal disorders like sarcopenia, gout, osteoarthritis (OA), intervertebral disc degeneration (IDD), and more (23–26). OA, for instance, affects a significant portion of the population, with a lifetime risk of 50% and a current prevalence of 10-15% in adults (27). In Chinese older adults aged 65 and above, the prevalence of OA is 8.1% (28), while it is projected to affect 78 million American adults by 2040 (29). Sarcopenia, characterized by muscle mass loss, affects an estimated 9.9%-40.4% of older individuals (30). IDD primarily affects middle-aged and older individuals but is becoming more prevalent at a younger age due to modern lifestyle factors, leading to substantial medical costs and economic burdens globally (31). Exercise-induced acute kidney injury (EIAKI), a hereditary pathological condition frequently developed in patients with renal hypouricemia (RHUC), due to increased renal urate clearance as a result of genetic mutations in the urate transporter URAT1 (RHUC1) or GLUT9 (RHUC2) (32) that reabsorbs urate in the renal proximal tubule. After several hours of exhaustive (anaerobic) exercise, the symptoms of EIAKI include loin pain, nausea and vomiting. It was suggested that after exhaustive exercise, an increase in intraluminal urate in the proximal straight tubule and/or thick ascending limb of Henle’s loop might activate nucleotide-binding oligomerization domain-like receptor family pyrin domain-containing 3 (NLRP3) inflammasome via the luminal Toll-like receptor 4-myeloid differentiation factor 88-phosphoinositide 3-kinase-mammalian target of rapamycin (TLR4-MyD88-PI3K-mTOR) and release interleukin-1β (IL-1β), which might cause the symptoms of EIAKI (32). This review aims to compile recent literature on the involvement of uric acid (UA) in these musculoskeletal conditions and their underlying mechanisms to offer insights for improved prevention and treatment.
2 UA: a potential risk factor for musculoskeletal diseases
Approximately 80% of total UA in the human body is endogenously produced directly from xanthine the metabolic products of adenine nucleotides (ATP, ADP, and AMP), primarily in the liver, while the remaining 20% is derived from dietary purine metabolism. The typical UA pool in the body stores around 1200 mg of UA (33). Daily UA production is approximately 700 mg, with two-thirds excreted by the kidneys, one-third by the intestines, and the remainder by sweat glands. A dynamic equilibrium between UA production and excretion involving urate reabsorption (primarily via urate reabsorption transporters URAT1, GLUT9a/b, OAT10 and OAT4) and secretion transporters (primarily OAT1/OAT3, ABCG2, NPT1/4 and ABCC4) (34, 35) is maintained under normal physiological conditions (5, 36). Exogenous fructose-induced de novo increase of UA production in human body (37) as shown in the hepatocyte cell line (HepG2) in vitro, and mitochondrial superoxide generation (38), suggest that exogenous fructose-induced UA release from liver cells can lead to oxidative stress in various tissues, including skeletal muscle (39). The underlying mechanisms linking UA to musculoskeletal diseases are summarized in Figure 1. Besides, UA can stimulate vascular smooth muscle cell proliferation by entering into cells via a functional urate transporter (40). EIAKI was associated with RHUC due to genetic mutations in the urate transporter URAT1 or GLUT9 (32). In Urat1- urate oxidase (Uox) double knockout mice, the pathogenic mechanism of EIAKI was shown attributed to increased levels of IL-1β via NLRP3 inflammasome signaling and Na+-K+-ATPase dysfunction involved in excessive urinary urate excretion (41). Additionally, XOR inhibitors appeared to be a potential therapeutic drug for the treatment of EIAKI (41).
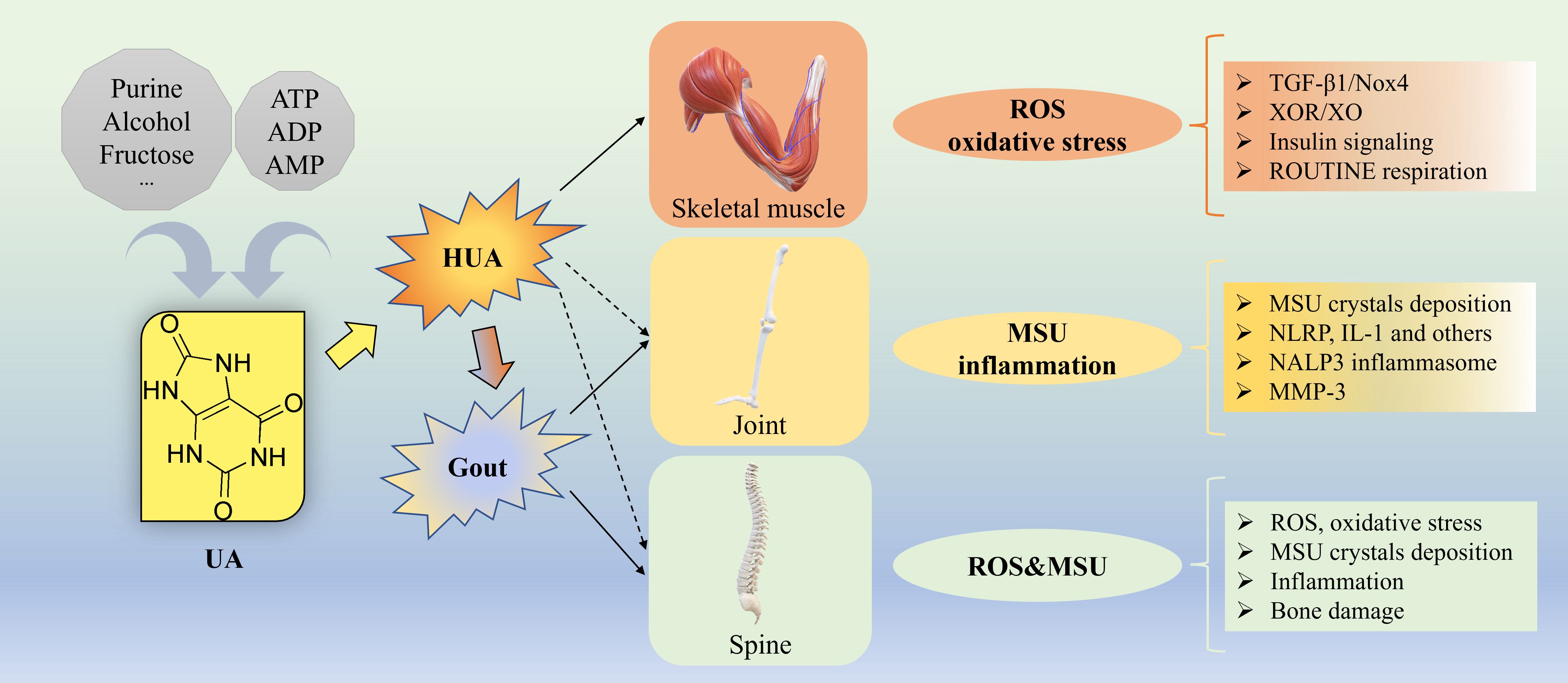
Figure 1. Underlying mechanisms for UA causing musculoskeletal diseases. Approximately 80% of total UA in the human body is endogenously produced from ATP, ADP, and AMP, while the remaining 20% is derived from dietary source such as purine, alcohol, fructose and others. Gout, a common metabolic disorder, is the MSU crystal-induced the most common inflammatory arthritis with episodic flares of intense inflammation related to long standing HUA. For skeletal muscle, the underlying mechanisms of how HUA causes oxidative stress by elevating ROS generation via activation of TGF-β1/Nox4 signaling, XOR/XO, insulin signaling, ROUTINE respiration and others. For joint, the underlying mechanisms of how HUA causes MSU crystal-induced inflammation via NLRP, IL-1, NALP3 inflammasome, MMP-3 and others. For spine, the underlying mechanisms of how HUA elevates the level of ROS and causes oxidative stress, MSU crystals deposition, inflammation, bone damage and others. ADP, adenosine diphosphate; AMP, adenosine 5’-monophosphate; ATP, adenosine triphosphate; HUA, hyperuricemia; IL, interleukin; MMP-3, Matrix Metalloproteinase-3; MSU, monosodium urate; NLRP, nucleotide binding domain and leucin-rich repeat containing protein; Nox4, NADPH oxidase-4; ROS, oxygen species; TGF, transforming growth factor; UA, uric acid, XOR, xanthine oxidoreductase; XO, xanthine oxidase.
2.1 Skeletal muscle activity enhances UA and reactive oxygen species production
Skeletal muscle presents a large surface area to plasma urate and plays a dominant role for the development of insulin resistance (IR)/T2D. In skeletal muscle, fructose-induced increase in purine nucleotide degradation and purine release from differentiated muscle cells (42), is a major source of purine metabolites (such as hypoxanthine and xanthine) that can lead to the increased production of UA in liver cells and endothelial cells that express xanthine oxidoreductase (XOR) (42). The skeletal muscle of male Sprague-Dawley (SD) rats was shown to have allopurinol-sensitive xanthine oxidase (XO) activity and synthesize UA (43). Exposure of rat skeletal muscle cells/myotubes (L6) in vitro to high fructose was shown to stimulate mitochondrial ROS and nitric oxide (NO) production, and the expression of inducible NO synthase (44). HUA-induced upregulation of transforming growth factor-β1 (TGF-β1), was shown to induce the NADPH oxidase isoform, NOX4 expression in freshly isolated human pulmonary artery smooth muscle cells (HPASMC) (45, 46). In HepG2 cells, UA produced by XO activity was shown to induce mitochondrial superoxide generation by translocating the NOX4, to the mitochondria (38). While UA is known to possess antioxidant properties, elevated UA production can paradoxically exert oxidative damage through generation of ROS (47, 48). HUA is strongly associated with IR, obesity and metabolic syndrome. Lowering UA levels in mice using allopurinol has been shown to improve insulin sensitivity in obese Pound mice with metabolic syndrome by improving proinflammatory endocrine imbalance in the adipose tissue (49). In potassium oxonate-induced acute HUA mouse model, very poor response to insulin activation of Akt in muscle, liver, and fat tissues, is indicative of HUA induced oxidative stress and IR (50). Exposure of differentiated mouse myoblasts (differentiated from mouse myoblast cell line, C2C12) to UA at a concentration of 15 mg/dL resulted in increased ROS production, inhibition of IRS1-AKT signaling, suppressed insulin-stimulated glucose uptake, and induction of IR (51). Similarly, exposure of differentiated C2C12 myotubes to UA at 12.6 mg/dL led to a 32% increase in oxidative stress, a 135% increase in antioxidant capacity, and a nearly 237% rise in triglyceride content. Additionally, UA reduced endogenous ROUTINE respiration, complex II-linked oxidative phosphorylation (OXPHOS), and electron transfer system (ETS) capacities in these myotubes, indicating detrimental effects of excessive UA on skeletal muscle (52) (Figure 1).
2.2 HUA affects joints and soft tissues
The proposed four distinct stages of pathological basis of gout involves four distinct stages: asymptomatic HUA, acute attacks, intercritical phase, and chronic tophaceous gout (53). Initially, asymptomatic HUA and acute attacks occur interchangeably, leading to polyarticular attacks in the chronic phase where symptoms include alternating attacks and MSU crystal deposition (tophi, typically white sand-like crystals) within joints typically the first metatarsophalangeal joint, ankle, knee, and elbow, periarticular and extra-articular sites of soft tissues (e.g., tendons, ligaments, muscles, cartilage) that are located outside of joints, ultimately resulting in OA and joint damage (54). MSU crystallizes when its plasma concentration exceeds its solubility (around 7 mg/dl, or 420 μmol/l). The acute gouty attack is called an acute sterile inflammation. MSU is widely accepted as a classical activator of the nucleotide-binding domain and leucine-rich repeat-containing protein 3 (NLRP3) linked to the development of gout (55, 56). Phagocytosis of MSU crystals particularly by neutrophils and macrophages, leads to the generation of ROS through activation of NADPH oxidases (52). This event activates the NLRP3 inflammasome which is a cytoplasmic multiprotein complex comprising a sensor protein, an adaptor protein ASC (apoptosis-associated speck-like protein containing a caspase-recruitment domain), and pro-caspase-1. When the inflammasome complex is assembled through a homotypic interaction between PYD and CARD domains of sensor proteins and ASC (apoptosis-associated speck-like protein containing a caspase-recruitment domain), inactive pro-caspase-1 is auto-processed to active caspase-1, leading to maturation of pro-interleukin-1β (pro-IL-1β) or pro-IL-18 to their active forms and released into the extracellular environment (55). Colchicine, that has been the drug of choice for gout attack for more than 200 years, that blocks MSU crystal-induced IL-1β generation upstream of NLRP3 inflammasome activation possibly by suppressing the microtubule-mediated NLRP3 inflammasome assembly (55, 56).
Several studies have investigated the signaling pathways in macrophages and neutrophils activated by MSU crystals, using animal experiments to identify potential therapy targets in the MSU-induced signaling pathway. IL-1β, a key inflammatory factor in gout, has been targeted for therapy and can be secreted by various immune cells such as macrophages, neutrophils, and mast cells. The processing of pro-IL-1β by caspase-1 in the NLRP3 inflammasome leads to the formation of mature IL-1β (57). SLC3037, an NLRP3 inhibitor that blocks NEK7 [never in mitosis A (NIMA)-related kinase 7 (NEK7) critical in NLRP3 oligomerization] binding to NLRP3, shows promise as a novel agent for diseases associated with NLRP3 inflammasome activation like gout (58). Experimental systems involving NLRP3 inflammasome activation in mouse bone marrow macrophages, along with in vivo mouse models, have been utilized to study the effects of Euodiae fructus (ER), a traditional Chinese medicine, on UA and gout. Results suggest that ER is a potent NLRP3 inhibitor, exerting anti-HUA and anti-gout effects by reducing UA production, enhancing UA excretion, and alleviating inflammation, partly through NLRP3 inflammasome inhibition (59). Furthermore, the role of T cells in gout is gaining recognition, with evidence indicating their involvement in both adaptive and innate immune responses. While T cells are not directly implicated in the inflammation caused by MSU crystals, they may have proinflammatory or anti-inflammatory regulatory functions in gouty inflammation (57).
Matrix Metalloproteinase-3 (MMP-3) has been studied in relation to the development of gouty arthritis from HUA. Research has shown that high levels of UA can lead to an increase in MMP-3, which in turn promotes the degradation of proteoglycan and induces the crystallization of MSU crystals (60). Genetic variants in UA transporters (such as GLUT9, URAT1, ABCG2, OAT1 and OAT3) have been linked to HUA (34, 61–65), increasing the risk of MSU crystal precipitation. The inflammatory response triggered by these crystals can cause cartilage damage, potentially leading to OA. A case-control study involving 243 unrelated Mexican-mestizo individuals revealed significant associations for 8 specific single nucleotide polymorphisms (SNPs) related to various genes. Gene-gene interactions were also observed, highlighting synergistic or antagonistic effects on OA development. It was found that common gene variants associated with UA transport were linked to OA in the Mexican population (66). Since MSU crystals are more likely to form at lower temperatures, recently by leveraging mild hyperthermia to synergistically enhancing catalytic decomposition of UA and endogenous hydrogen peroxide (H2O2) with polydopamine-platinum nanoparticle was shown to be a potential therapeutic strategy for the management of acute gout (67) (Figure 1).
2.3 HUA: a risk factor for spinal inflammation
The incidence of spinal gout (with symptoms of fever, back pain and spine compression that occur within a short time frame and weakness) is more common than previously thought, in patients with extensive prior history of gout or HUA. In spinal gout, the lumbar spine is the more commonly affected (68) than cervical vertebrae or thoracic vertebrae. Spinal gout can be identified by magnetic resonance imaging (MRI with gadolinium contrast), computed tomography (CT) and positron emission tomography (PET) (68). Patients with HUA or gout can have the presence of tophi in the intervertebral disc (IVD) or endplate, and these patients also exhibited severe IDD (4). Therefore, it was speculated that MSU crystals accumulation in the endplate and IVD may lead to mechanical damages disrupting the supply of nutrients and oxygen to the IVD and trigger inflammatory reactions through oxidative stress in mitochondria exacerbating IDD (69). The extracellular matrix (ECM) of the IVD contains a variety of proteoglycans that possess multiple negative charges. The anion gap caused by negatively charged proteoglycans can significantly increase the solubility of MSU (69) and thus prevent its crystallization. Therefore, it was also speculated that destruction of the proteoglycans by MSU-induced MMPs can further decrease the solubility of MSU and help grow tophi into larger structures (69) (Figure 1).
2.4 HUA: an important player in osteoporosis
OP is the most common metabolic musculoskeletal disorders primarily in postmenopausal women with estrogen deficiency, diabetic patients, and obese patients, affecting about 200 million people worldwide. OP is characterized by reduced bone mineral density (BMD), reduced bone mass and destruction of bone microarchitecture, leading to increased bone fragility and increased risk of fracture. The clinical manifestation of OP includes joint inflammation/pain, stiffness, and reduced mobility. Common risk factors of OP are aging, sex hormone deficiency, calcium and vitamin D deficiency, elevated parathyroid hormone (PTH) levels, genetics, and unhealthy lifestyle (70). Moreover, oxidative stress caused by excessive accumulation ROS produced in mitochondria also plays a pivotal role in the pathogenesis of OP (70). Excessive accumulation of ROS can damage cell membranes, cytoplasmic structures, and even DNA. Excessive ROS can inhibit the differentiation and proliferation of osteoblasts (OB) (70). Multiple studies also show that disorders in purine metabolism may cause various type of osteoporosis. Abnormal purine metabolism causes HUA and the accumulation of ROS. HUA and gout also increase the risk of bone fragility/fracture because MSU-induced oxidative stress and proinflammatory cytokines (e.g., IL-1β, TNFα, IL-6, IL-8) released by MSU-phagocytosed monocytes, increase bone resorption and decrease bone formation (71). For bone remodeling, osteoclasts (OC), OB, and osteocytes play important roles. OB regulate OC differentiation and activation through receptor activator of nuclear factor kappa-β ligand (RANKL)-mediated signaling pathways. The RANKL receptor (called RANK) is expressed in OC progenitors to promote OC differentiation and maturation. 1,25 vitamin D, parathyroid hormone (PTH), and IL-6 activate RANKL-signaling in OB. Both proinflammatory cytokines and oxidative stress, induced by MSU, stimulate OC activity and inhibit OB function, which result in bone loss (71). MSU was shown to directly inhibit 1α-hydroxylase expression in renal proximal tubules and thereby reduce the active form of vitamin D, 1,25-dihydroxyvitamin D3 1,25(OH) concentrations in hyperuricemic SD rats (72). In postmenopausal women, HUA is associated with vitamin D insufficiency (73). The impact of SU on bone health also depends on the hormonal status in postmenopausal women (74). The SU level was shown positively correlated with elevated parathyroid hormone (PTH) levels in SD rats (71, 72). Therefore, UA has the ability to inhibit 1,25-dihydroxyvitamin D3 1,25(OH) production, leading to secondary hyperparathyroidism and further worsening UA-related bone loss (70). Additionally, using simple hyperuricemic group and HUA castration group of male Wistar rats, it has been shown that the castration group of hyperuricemic rats had significantly decreased level of SU and serum phosphorus suggesting androgen might have positive impact on UA levels and bone metabolism/growth in presence high SU level (75). In US adult females aged 40-60 years, testosterone level was shown positively associated with lumbar BMD (76).
2.5 HUA: a potential risk factor in rheumatoid arthritis
In the context of RA, a polyarticular systemic autoimmune disease characterized by the presence of autoantibodies, the role of UA remains unclear. RA is a musculoskeletal disease characterized by progressive joint cartilage and bone destruction, inflammation affecting muscle, bone, lungs, exocrine glands, liver and brain, chronic synovial inflammation and hyperplasia, production of autoantibodies including rheumatoid factor (RF) and anti-citrullinated protein antibody (ACPA), physical impairment, work disability and early morbidity and mortality (77). RA is different from OA because RA is an autoimmune disease in which the body’s own immune system attacks the body’s joints and OA is caused by mechanical wear and tear on joints. Sarcopenia, a progressive and generalized skeletal muscle disorder involving accelerated loss of muscle strength and mass, affects approximately one in four people with RA (78). Hypertension is highly prevalent among RA patients. Rats treated with 2% oxonic acid was shown to develop mild HUA and hypertension indicating increased SU levels can promote the development of hypertension and systolic blood pressure (79) most likely by causing vascular smooth muscle cell proliferation as shown in an in vitro experiment (79). The HUA and hypertension were partially inhibited by losartan (an angiotensin II receptor blocker) suggesting involvement of activation of the renin-angiotensin system in developing hypertension. Allopurinol (a xanthine oxidase inhibitors) treatment also prevented the hyperuricemia and hypertension (79) suggesting role of HUA in developing hypertension. Allopurinol treatment in adults with hypertension and HUA also effectively lowered SU levels and hypertension (80). The prevalence of metabolic syndrome, caused by hyper-insulinemia/IR that stimulates UA reabsorption in the proximal tubule (81), is as high as 40% in RA patients (82) suggesting potential role of HUA in developing RA. HUA causes hypertension and CVD, and CVD is associated with renal dysfunction in patients with RA (83) again indicating potential role of HUA in developing RA. Endothelial dysfunction is also common in RA (84) and UA was shown to promote endothelial dysfunction and atherogenesis (85). Thus, it appears that UA is not just an innocent bystander, it could be an active player in CVD-associated mortality in patients with RA. UA was shown as a strong correlate of renal dysfunction in RA patients (83). Deposition of MSU crystals can promote cartilage degradation in the joints (especially metatarsophalangeal joint, mid-foot, knee and finger distal interphalangeal joints) (86, 87). Levels of serum proinflammatory cytokines IL-1β, IL-6, IL-17 and TNFα were shown significantly higher in patients with RA (88, 89). Peripheral blood mononuclear cells, pre-treated with soluble UA and stimulated by MSU crystals, were also shown to enhance IL-1β, IL-6 production (90).
3 Underlying clinical correlations for SU and musculoskeletal diseases
3.1 SU and skeletal muscle diseases
Abnormal concentration of SU is related to some skeletal muscle and metabolic diseases, such as sarcopenia, T2D, McArdle disease (MCD), heart failure and peritoneal dialysis and others (91–94).
3.1.1 SU levels and sarcopenia
Sarcopenia is defined as a gradual reduction in skeletal muscle mass and function (95). Elevated levels of SU may act as a protective mechanism against free radical damage, potentially preventing muscle protein damage and loss of muscle mass and strength in the elderly population (96). A community-based cross-sectional study using 3079 Chinese adults (963 men and 2116 women) aged 40-75 years revealed that higher SU levels were linked to increased BMD and muscle mass, with the relationship between SU and BMD being influenced by muscle mass (97). Additionally, research on individuals aged 92.8 ± 3.1 years indicated that higher SU levels were associated with improved muscle function, suggesting a potential role of UA in slowing the progression of sarcopenia (98).
A recent cross-sectional study conducted in western China with 4236 adults aged 50 years and older found a negative correlation between SU levels and sarcopenia in both men and women (23). Additionally, the study revealed an inverted J-shaped correlation between SU levels and handgrip strength in women, as well as a positive correlation between SU quartiles and skeletal muscle index regardless of gender. The study recommended that Chinese adults aged 50 years and older with higher SU levels (men: <5.36, 5.36–6.25, 6.25-7.12, ≥7.12 mg/dL; women: <4.26, 4.26–4.94, 4.94-5.72, ≥5.72 mg/dL) may experience increased muscle mass and grip strength, potentially slowing down the progression of sarcopenia (23), which is consistent with previous research findings (97, 98). Furthermore, gender differences in SU levels during adolescence in children and adolescents were noted, likely influenced by sex hormones and pubertal development, with muscle mass playing a role in this variation (99). In this cross-sectional study involving 823 children and adolescents aged 6-18 years of both sexes found that muscle mass contributed to 7.7% and 43.0% of SU variability in girls and boys, respectively (99) (Table 1).
However, abnormally high SU levels (>8 mg/dL) are linked to increased production of ROS and inflammation, both of which contribute to sarcopenia (91). SU at higher concentrations exhibits oxidant properties that are associated with systemic inflammation, leading to a higher risk of muscle strength loss. A cross-sectional study involving 630 Japanese men (aged ≥ 30 years) examined the relationship between SU concentration, grip strength, and leg extension power. The results indicated that HUA (>7.0 mg/dL) was related to poor muscle strength, demonstrating an inverted J-shaped correlation between SU quartiles and muscle strength (100). As age plays a significant role in skeletal muscle composition and function, the adverse effects of UA are more pronounced in younger individuals compared to older individuals. The association between SU levels (men: <5.5, 5.6-6.5, ≥6.6 mg/dL; women: <4.2, 4.3-5.2, ≥5.3 mg/dL) and muscle strength varied across age groups. While a negative correlation was observed in adults aged 20 to 40 years, this relationship reversed in individuals above 60 years old. It is essential to consider both age and muscle strength when setting SU goals for individuals with HUA (101). Both age and SU levels should be considered when evaluating skeletal muscle function and preventing sarcopenia, aligning with the notion that sarcopenia involves age-related muscle mass loss influenced by various factors. For middle-aged and older individuals (≥40 years), higher SU levels (≥5.5 mg/dL) may help safeguard muscle mass and function against sarcopenia. Conversely, younger individuals (aged 20 to 40 years) exhibit a negative correlation between SU levels and muscle strength. In children and adolescents (aged 6 to 18 years), gender and SU levels could directly impact muscle mass. Moreover, excessively high SU levels (>7.0 mg/dL) could contribute to sarcopenia by promoting increased ROS and inflammation (Table 1, Figure 2).
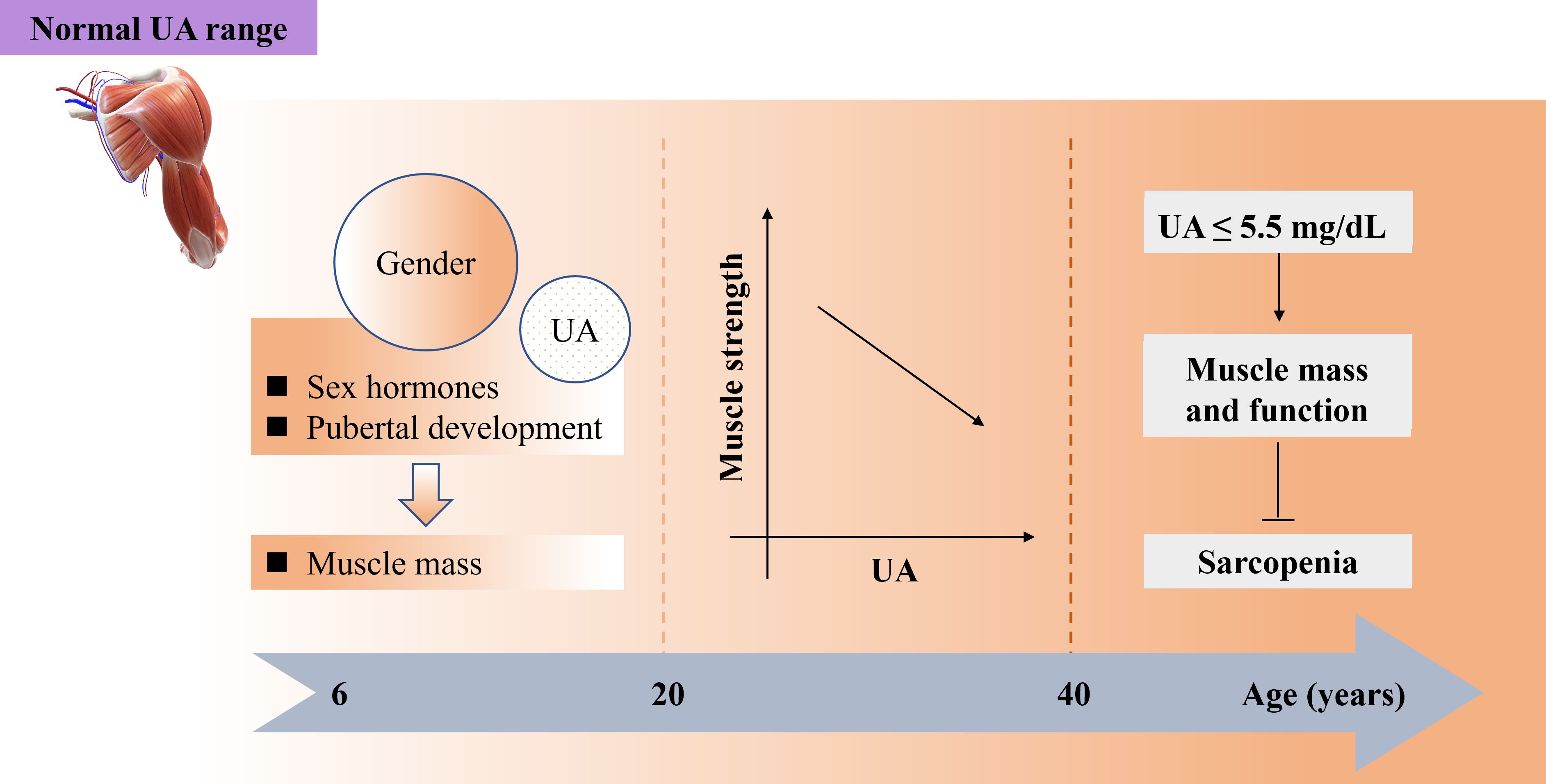
Figure 2. Both age and SU play important role for maintaining normal function of skeletal muscles preventing sarcopenia while SU stays within normal range. SU, serum urate.
3.1.2 HUA: a risk factor for T2D and sarcopenia
UA is primarily produced in the liver and to a lesser extent in small intestine (102), adipose tissue (103), and endothelial cells (42), and is predominantly excreted through the urinary tract and sightly through intestine and sweat glands. Several factors can contribute to HUA, including a diet rich in fructose (104), the consumption of exotic organic products (47), and alcohol intake (105, 106). HUA is often associated with metabolic syndrome, which is characterized by abdominal obesity, glucose intolerance, IR, dyslipidemia, and hypertension (47). Elevated SU levels play a role in regulating oxidative stress, inflammation, and enzymes involved in glucose and lipid metabolism, contributing to the deterioration of metabolic homeostasis (47). In fact, HUA and abdominal obesity was shown to synergistically increase the risk of hypertriglyceridemia and low HDL-C in both sexes (107). It is still debatable whether high SU levels are a strong independent predictor of diabetes and precede the development of IR and diabetes (108), because results from some studies indicate IR/hyperinsulinemia might precede HUA (81, 109, 110). Obese individuals with elevated SU levels tend to have lower insulin sensitivity and higher levels of oxidative stress compared to those with normal SU levels (111). A cohort study involving 440 non-diabetic Caucasian subjects with higher SU levels (6.4 ± 0.9) showed a weaker metabolic phenotype and lipid profile, increased 2-hour post-load glucose levels, elevated fasting and 2-hour post-load insulin levels, reduced skeletal muscle insulin sensitivity, and impaired hepatic insulin sensitivity (112) (Table 2).
Skeletal muscle plays a critical role in the effects of insulin and the development of T2D, making sarcopenia a common complication in individuals with diabetes. HUA is frequently seen in conjunction with T2D. A cross-sectional study involving 401 subjects with T2D (209 men and 192 postmenopausal women) was conducted to explore the relationship between SU levels and muscle mass. The study found that higher SU levels were an independent risk factor for men with T2D and sarcopenia (93). Furthermore, a strong positive association was observed between obesity, visceral fat accumulation, and HUA. Another cross-sectional study involving 3393 Chinese participants (43.3% men) investigated the ratio of visceral fat area to leg muscle mass and SU levels. The results indicated a positive correlation between the visceral fat area to leg muscle mass ratio and the risk of HUA in women, even after adjusting for confounding variables. Notably, pre-menopausal women exhibited a higher risk of HUA compared to postmenopausal women as the visceral fat area to leg muscle mass ratio increased. The study also revealed that the highest risk of HUA was observed in individuals with both high visceral fat area and leg muscle mass, placing them in the highest quartile of risk (113) (Table 2).
3.1.3 HUA and McArdle disease
MCD, also called Type V glycogen storage disease, is an autosomal recessive hereditary condition that results from the lack of glycogen phosphorylase enzyme (the enzyme required for efficient glycogen breakdown) activity in skeletal muscle. Patients with MCD are exercise intolerant because of muscle weakness, fatigue and cramp/pain during moderate- to high-intensity exercise (114). MCD patients often develop HUA because muscular exercise accelerates the degradation of muscle purine nucleotides into inosine and hypoxanthine in the blood that serve as substrates for the synthesis of uric acid in cells of tissues/organ (e.g., liver cells and endothelial cells) expressing xanthine oxidase enzyme, leading to HUA (115). Increased synthesis of UA produces H2O2 causing increased oxidative stress. Patients with MCD have elevated level of markers of oxidative stress (8-isoprostanes and protein carbonyls) with insufficient levels of compensatory antioxidant enzyme (mitochondrial superoxide dismutase) activity to fully protect skeletal muscle from oxidative damage. Oxidative stress in McArdle patients can potentially lead to rhabdomyolysis and the subsequent development of fixed myopathy (92) (Table 2).
3.1.4 XO: a potential link between skeletal muscle abnormality and heart failure
Patients with HF are intolerant to exercise partly due to skeletal muscle abnormalities caused by ROS. In ischemic tissue, higher activity of XO, that catalyzes UA production from xanthine and hypoxanthine, overproduces ROS (H2O2) (36). Skeletal muscle atrophy is a condition of loss of muscle mass caused by various diseases such as diabetes, heart failure, and sarcopenia, resulting in the reduction of total adenine nucleotides, including ATP, ADP, and AMP involved in cellular signaling (36). The elevated level of XO and degradation products such as hypoxanthine, xanthine, in the atrophic skeletal muscle leads to the over production of UA in XO-expressing tissues (36, 116). Higher SU level in patients with heart failure is considered as an independent marker of prognosis and poor exercise tolerance, partly attributed to abnormal skeletal muscle function. In mouse models of HF, myocardial infarction (MI) induced by ligating the left coronary artery, led to skeletal muscle abnormalities, exercise intolerance and eventually to HF (94) and inhibition of XO by febuxostat/allopurinol was shown to prevent XO-derived ROS (H2O2) overproduction in XO-expressing tissues and skeletal muscle abnormalities and exercise intolerance (94, 117) suggesting inhibition of XO-derived ROS production can prevent skeletal muscle abnormalities.
3.1.5 Association of SU levels with skeletal muscle strength
In hemodialysis patients, SU level was suggested as a good marker positively correlated with body composition parameters, muscle function, inflammation, and quality of life body composition (118). In a single-center retrospective cohort study on 802 peritoneal dialysis patients (with 464 men and an average age of 46.2 ± 14.2 years), the SU levels in middle-aged and elderly male patients were shown positively associated with appendicular skeletal muscle mass, suggesting a significant impact of appendicular skeletal muscle mass on the relationship between SU and peritoneal dialysis-related mortality (119) (Table 2). Additionally, in a cross-sectional study on 113 Brazilian kidney transplant patients (75 men and 38 women), SU level was shown positively correlated with muscle mass (fat-free mass, appendicular muscle mass, muscle mass index, and appendicular muscle mass index) and strength, but not to functional capacity (120) (Table 2). A study conducted on a Tasmanian cohort of older adults, consisting of 409 individuals aged 50-79 years (205 men) over the 10-year period, showed association of elevated level of asymmetric dimethylarginine (ADMA) in the blood with the decrease in hand grip and knee extension strength (121). Furthermore, increase in SU level was shown associated with the decline in leg muscle strength over the same 10-year period (121). In a genome-wide association study (GWAS), a single nucleotide polymorphism (SNP) rs1125718 near the WISP1 gene was shown associated with ADMA levels (121). These findings suggest that higher levels of ADMA and SU at baseline may contribute to age-related muscle strength decline, highlighting potential new targets for preventing muscle strength loss over time (121) (Table 2).
3.1.6 RHUC involved in EIAKI
Renal hypouricemia (RHUC) was shown as an important risk factor for EIAKI and urinary stones (32). There are two types of RHUC described, RHUC1 and RHUC2. RHUC1 is caused by mutations in the SLC22A12 gene (encoding the urate reabsorption transporter URAT1), while RHUC2 is caused by mutations in the SLC2A9 gene (encoding the urate reabsorption transporter GLUT9 isoforms, GLUT9a and GLUT9b). A single−center study from China reported that a proportion of 10 out of 19 patients suffered from EIAKI, the prognosis of which was favorable (122). A large-scale cross-sectional study from Japan found that genetic hypouricemia in men is more strongly involved in EIAKI than transient hypouricemia which is mostly influenced by female hormones (123). Another large-scale cross-sectional study of Japanese schoolchildren discovered that hypouricemia in children including RHUC, as a main cause of EIAKI, was a crucial clinical problem, over and above HUC. The school-age children were investigated, who were younger than the overwhelming majority of people with EIAKI, for reducing the risk of subsequent EIAKI via offering more care and guidance (124). It was suggested that after exhaustive exercise, RHUC patients develop higher concentration of urate in the lumen of proximal straight tubule and/or thick ascending limb of Henle’s loop that could stimulate the luminal TLR4-MyD88-PI3K-mTOR to activate the NLRP3 inflammasome and release IL-1β, which might cause the symptoms of EIAKI (32).
3.2 UA, gout and OA
3.2.1 UA: a potential risk factor for OA
Osteoarthritis (OA), one of the top two leading causes of disability worldwide affecting about 7% of the global population, is a chronic, heterogeneous and degenerative joint disease characterized by degeneration of articular cartilage, sclerosis of subchondral bone (just below the cartilage), and osteophyte (growth of bone at the edges of joints/spine) formation, which is considered a leading cause of activity limitation and disability among the elderly population. OA has multiple clinical phenotypes such as abnormal joint tissue metabolism followed by cartilage degradation, bone remodeling, osteophyte formation, joint inflammation and loss of normal joint function (125). The diverse overlapping components of molecular endotypes of OA include bone and cartilage, inflammatory, metabolic and low repair (126). The etiology of OA is multifaceted, involving a complex interplay of metabolic, genetic, mechanical, and inflammatory factors (127). The inflammation of OA could be independent of NLRP3 activation because stress-induced cartilage degradation, in OA cartilage explants from OA patients, was reported not linked to increased IL-1β production (128). NLRP3-deficient mice also showed similar cartilage degradation upon stimulation by inflammatory agents like LPS, IL-1α, and tumor necrosis factor (TNF)α-induced activity of MMP-3, MMP-9, and MMP-13 in cultured chondrocytes and in NLRP3(-/-) chondrocytes (128). Positive associations between SU levels and OA were reported by several studies (129–131) especially in patients of hip or knee OA with elevated SU levels (132, 133). Additionally, it was reported that SU levels may serve as a biomarker for OA progression (134) as SU levels can differ between patients with non-progressive and progressive OA over time, as demonstrated by changes in X-ray joint space narrowing (134). Recently, in a prospective cohort study with a total of 4671 American participants (age range: 20 to 85 years old), including 2988 females and 1683 males, a nonlinear J-shaped relationship between SU levels (with the cut-off values of 5.6 mg/dl for females and 6.2 mg/dl for males) and all-cause mortality in females and males with OA has been reported (135). However, recent data from the Seventh Korea National Health and Nutrition Examination Survey in 2016 suggested no significant association of SU levels with OA in the Korean population, as some patients with OA actually had lower SU levels compared to those without OA (136) (Table 3).
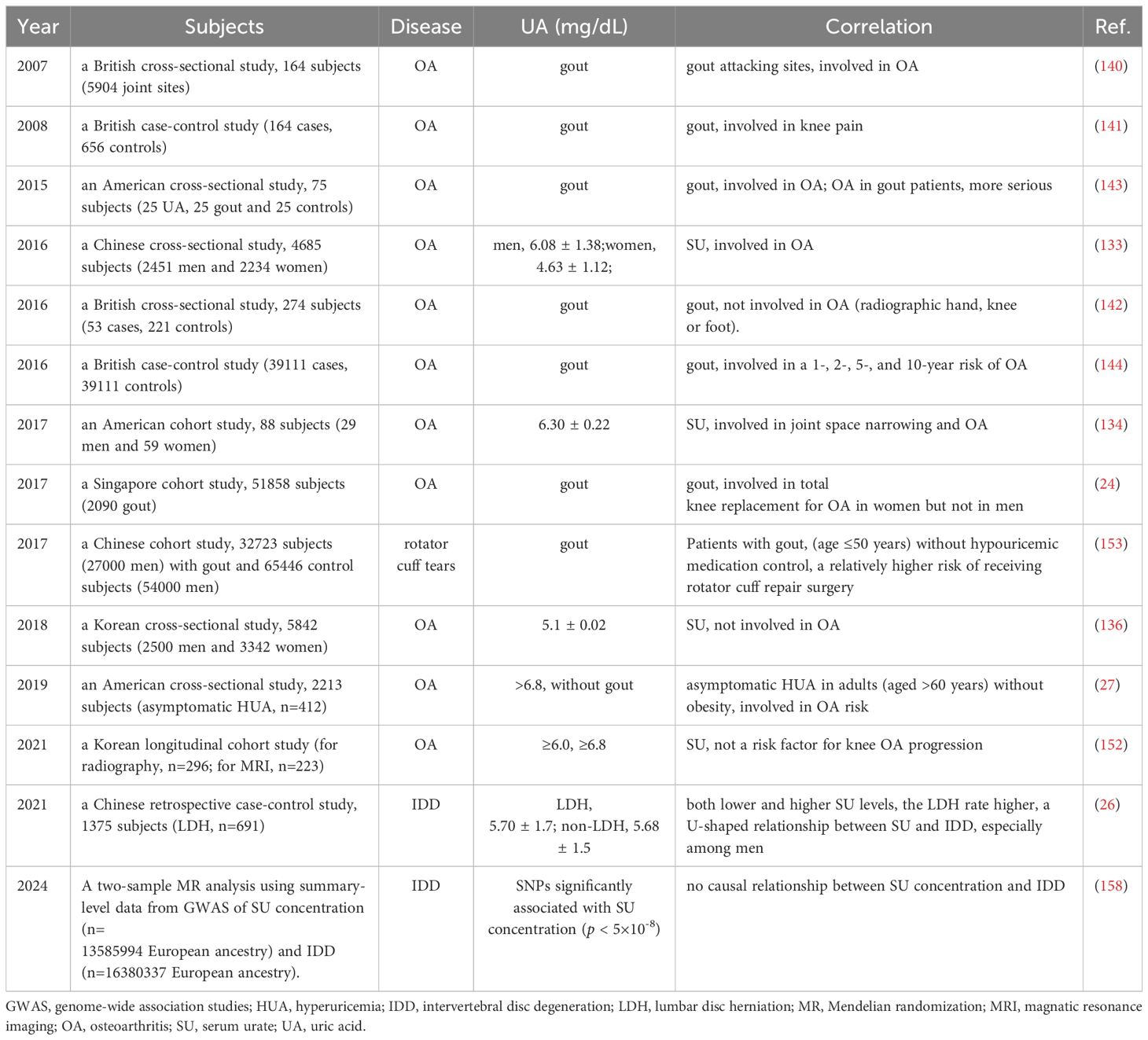
Table 3. Update of clinical studies on correlation between UA or gout and OA or other bone diseases.
3.2.2 The relationship between gout and OA
OA affects about 30 million individuals and gout affects about 8.3 million individuals though about 43 million individuals in the U.S. are diagnosed with HUA (27). OA is a low-grade inflammatory condition primarily caused by wear and tear on joints that causes cartilage erosion symmetrically on both sides of the body leading to morning stiffness for short period of time with little or no swelling. Gout, a common metabolic disorder, is the MSU crystal-induced the most common inflammatory arthritis with episodic flares of intense inflammation related to long standing HUA (137). Tophaceous gout occurs when MSU crystals form white deposits called tophi around joints and soft tissues causing restricted joint function. Deposition of MSU crystals were detected in various bodily structures such as joints, cartilage, synovial bursa, tendons, or soft tissues (138). Gout and OA frequently coexist clinically in the same patient (139). However, the pathophysiologic relationship between the two remains elusive. A nested case-control study revealed that joints with a history of gout attacks were more likely to be affected by OA (140). Specifically, the distal interphalangeal joint of the finger, knee, mid-foot, and the first metatarsophalangeal joint showed a 12.7-fold, 3.1-fold, 2.9-fold, and 2.1-fold increased risk of clinically defined OA when attacked by gout, accompanied by chronic pain symptoms (141). A cross-sectional study, which included three cohorts of subjects aged 50 years or older with joint pain, found that individuals with gout had an increased risk of hand and foot OA but a decreased risk of knee OA (142). Another small cohort study focusing on middle-aged to elderly men with HUA, gout, or neither, revealed an elevated risk of knee OA in patients with gout, with no significant differences observed between patients with HUA and the other groups (143). Additionally, a cohort study involving 51,858 subjects and 1,435 cases of incident knee arthroplasty showed a significantly higher prevalence of knee arthroplasty among women with gout (24). Furthermore, a case-control study with 39,111 cases and 39,111 controls indicated that gout was associated with a 1-, 2-, 5-, and 10-year risk of OA (144).
Gout also promotes cartilage degradation due to the direct effects of MSU crystals. Studies have shown that joints (especially metatarsophalangeal joint, mid-foot, knee and finger distal interphalangeal joints) affected by acute attacks of gout also display clinical or radiographic features of OA (86, 87) suggesting that OA may predispose to the localized deposition of MSU crystals. It remains elusive whether gout promotes the development or progression of OA but aging and obesity remain as significant risk factors for both OA and gout. In a cross-sectional study, higher fat mass, increased muscle mass, and the presence of osteophytes were shown associated with the higher risk of tophaceous gout (145). Evidence from epidemiological studies supports an association between gout and OA (127). Compared to the painful flares of gout, the pain in OA is less intense and more variable. OA but not gout commonly affects hip, and wrist however OA and gout share some joints, like the distal interphalangeal joints, the first metatarsal phalangeal joints and knees (27). Poor renal excretion of urate is an important risk factor for gout but not for OA. OA genetics relates most commonly to cartilage biology and body mass index (BMI), whereas gout genetics relates largely to renal handling of urate. Men are more likely to develop gout, whereas women are more likely to develop OA indicating gender difference might play an important role in both gout and OA. UA has been hypothesized as a pathological link between gout and OA but current therapies for OA include only analgesia and joint replacement. The role of MSU crystal-induced inflammation in both OA and gout could be a possible shared pathway for pathogenesis. MSU and calcium pyrophosphate dihydrate (CPPD) crystal deposits in the talus were reported with cartilage degradation (146) suggesting these lesions are biomechanically induced. Soluble form of UA/urate in synovial fluid of knee OA was suggested to be strongly and positively associated with OA severity with pro-inflammatory cytokines like IL-1β and IL-18 (produced by uric acid-activated inflammasomes) in the synovial fluid (147). Thus, it was hypothesized that urate from systemic circulation or released from dying chondrocytes, might get into the joint and form micro-particles with proteoglycan released from dying cells. These micro-particles may then trigger the innate immunity and NALP3 inflammasome pathway (147). MSU crystals may trigger toll-like receptor 4 (TLR-4) primed by the reservoir of lipopolysaccharide (LPS) from gastrointestinal microbiome of obese people leading to phagocytosis, inflammasome activation, and subsequent inflammation and joint damage (148). Peripheral blood mononuclear cells (PBMCs), pre-treated with soluble UA and stimulated by MSU crystals, were shown to enhance IL-1β, IL-6 production (90) suggesting the condition of HUA may influence inflammatory responses by facilitating IL-1β production in peripheral blood leukocytes. IL-1β via activation of the MAPK (mitogen-activated protein kinase) can elevate the levels of matrix metalloproteinases that can cause cartilage matrix protein degradation (149). MSU is also linked to complement activation, direct T cell activation, and mast cell degranulation, all of which may be involved in the pathogenesis of OA (150, 151). The overlap between OA and gout/HUA suggests the efficacy of urate-lowering therapies for OA could be an interesting hypothetical strategy.
Previous research has established a reciprocal relationship between UA and OA (27). In a Korean longitudinal cohort study aimed at validating the potential link between gout and OA, it was found that over a 3-year period, SU levels in subjects without gout were inversely related to patellofemoral cartilage loss. However, SU was not identified as a risk factor for OA progression in the study, which was consistent with previous findings (136). Nonetheless, large-scale longitudinal studies involving diverse populations are needed to further elucidate this association (152). Lastly, an American cross-sectional study involving 2,213 individuals, including 412 with asymptomatic HUA, revealed that asymptomatic HUA was associated with an increased risk of OA, particularly in adults over the age of 60 without obesity (25) (Table 3). Clinical epidemiological studies on gout involving tendons are rare. Rotator cuff tears are a common cause of shoulder disability, and surgery is often chosen to restore function. In a 7-year Chinese longitudinal cohort study, 32,723 patients with gout and 65,446 control individuals participated at a 1:2 ratio. Patients with gout, especially those under 50 years old and not on hypouricemic medication, had a higher risk of needing rotator cuff repair surgery. Strict control of UA levels with hypouricemic medication could reduce this risk (153). Generally, SU levels should be kept below 6.0 mg/dL. For patients with tophi, initial SU levels should be below 5.0 mg/dL to dissolve tophi (138) (Table 3).
3.3 UA, spinal gout and IDD
3.3.1 Spinal gout: a rare but serious disease involving MSU crystal deposit
Spinal gout, a rare disease, can often be mistaken for infections or neoplastic lesions, posing a challenge in diagnosis. In a case study, a patient with a long history of HUA and gout with progressive impairment of walking, MRI features revealed vascularized gouty tophi in the cervical spine in areas of low signal intensity both on T1 and T2 images and pathologic examination of the specimen demonstrated presence of fibrous tissue and amorphous MSU deposits and bone erosions by the MSU crystal deposits (154). In another case study, a 71-year-old man with a long history of HUA and gout (multiple tophi in elbows, hands, knees, and toes) with progressive quadriparesis (muscle weakness in both legs and both arms), severe cervical spondylosis and a herniated cervical disc at the C3-C4 level on MRI, was found to have intradiscal chalky white granular material called gouty tophus (155). Though spinal involvement in gout and cervical spinal cord compression caused by gout are uncommon, it should be considered in all patients presenting myelopathy and history of gout and HUA (156).
3.3.2 UA: a potential risk factor for IDD
Intervertebral disc degeneration (IDD) is a common musculoskeletal disorder. Several case reports have described the presence of tophi in the endplate (a bilayer of cartilage and porous bone) or intervertebral disc (IVD) of patients with HUA or gout, and severe IDD. UA with its potent antioxidant activity helps maintain the stability of the IVD, but higher levels of UA production associated with generation of higher level of ROS (H2O2) production can lead to accelerate IDD (5). Moreover, MSU crystals accumulated in the endplate and IVD, can cause mechanical damage and inflammatory reactions that worsen IDD. In a recent study involving 1375 Chinese patients, it was found that there was a U-shaped relationship between SU levels and IDD, especially among men (26). The study also revealed a positive correlation between SU and lumbar spine BMD in American men within a certain range (SU < 5 mg/dL), although this correlation was no longer significant when SU levels exceeded 5 mg/dL. Factors such as race and age were found to influence this relationship (157). However, in a recent Mendelian randomization study, no causal relationship between SU and IDD was reported (158) (Table 3).
3.4 UA and bone remodeling
Bone remodeling takes place throughout life and is required not only for skeletal growth but also to maintain normal bone structure. Bone remodeling requires a fine balance between bone resorption by OC (osteoclast, differentiated from hematopoietic mononuclear cells) and bone formation by OB (osteoblast, differentiated from mesenchymal stem cells). Disruption of this balance leads to disease conditions like skeletal abnormalities, OP (osteophyte, bony lumps that grow on the bones in the spine or around joints) and rheumatoid arthritis (RA). Disorders in purine metabolism can lead to HUA with accumulation of ROS (H2O2) during UA biosynthesis. The pathological states induced by MSU and ROS, characterized by oxidative stress and inflammation, disrupt the balance of bone resorption and bone formation by inhibiting the expression of osteogenic factors, promoting OC differentiation, inducing bone erosion resulting to the loss of bone mass and destruction of bone microarchitecture (159). Previous research has shown a positive independent association between SU levels and BMD (bone mineral density), with a non-linear relationship observed in individuals with normal or low body weight. SU levels below 296 μmol/l may have a protective effect on BMD in normal- and low-weight OP patients, while levels above this threshold show no significant relationship with BMD (160). One study discovered a negative correlation between SU levels and total trabecular bone score (TBS) in adults (161). Maintaining SU at an optimal level can potentially help prevent OP and fractures. Interestingly, the association between SU levels and BMD at various skeletal sites differed in middle-aged and elderly men, with a positive relationship observed only in the normal weight group (162).
In Chinese patients with Parkinson’s disease (PD), higher SU levels within the normal range may serve as a biomarker for higher BMD and are associated with a lower prevalence of OP (163). Among hypertensive males, the relationship between SU and total femur BMD followed an inverted U-shaped curve, while in females, the association was predominantly negative. In the non-hypertensive group, the relationship between SU and total femur BMD also exhibited an inverted U-shaped curve across genders (164). Lumbar BMD showed a negative correlation with HUA in obese individuals, but this trend was observed only in men, not women. Furthermore, there was no significant link between hip BMD and HUA in obesity (165). SU levels were positively correlated with BMD at three different sites and were found to have a protective effect against abnormal BMD after adjusting for various confounders, including fat-free mass (FFM) and skeletal muscle mass index (SMI), in men aged 50 years and above (166). Among Mexican females, higher SU levels were associated with lower BMD at various skeletal sites (167). Additionally, individuals with HUA, gout, or varying levels of SU may have a higher risk of poor outcomes following hip fractures (168). In patients with T2D, higher SU levels were suggested to be a protective factor for bone health. However, the osteoprotective effects of SU were influenced by age and gender, being significant only in non-elderly men and elderly women (169). The SU-to-creatinine (UA/Cr) ratio was identified as an independent factor influencing bone turnover markers in patients with T2D (170).
4 Limitations and future perspectives
All the above studies unequivocally indicated that UA was clinically involved in musculoskeletal diseases, such as sarcopenia, gout, OA, RA, IDD, OP, EIAKI and others. The relationship between UA and many of musculoskeletal diseases still remained as part of a clinical discovery, analysis and summary. In this study, only clinical associations and some underlying mechanisms were mainly focused on and reviewed for indicating the potential links between UA and many musculoskeletal diseases. Moving forward, emerging evidences from epidemiological studies and newly discovered pathogenic pathways will contribute to a deeper understanding of the relationship between UA and musculoskeletal diseases. More and more animal studies and molecular biology experiments were expected to explain action mechanisms of UA influencing musculoskeletal functions. And more drugs and biotechnologies were urged to be developed for treating such musculoskeletal diseases associated with UA. Recently, an intestine-targeted explosive hydrogel microsphere has been developed to promotes UA excretion for gout treatment via an in situ small intestine mucosal layer regulation by oral microspheres (171). It may provide a brand-new pathway for the prevention and treatment of gout and other musculoskeletal diseases.
5 Conclusion
Nowadays, UA has been recognized as a significant risk factor for metabolic syndromes. Moreover, an increasing number of studies have highlighted the connection between UA and musculoskeletal diseases such as sarcopenia, OA, IDD, OP and EIAKI due to their prevalence (Table 4). This review presents the most recent and valuable insights into the mechanisms and associations between UA and musculoskeletal diseases, aiming to improve prevention and treatment strategies. In the context of preventing sarcopenia, both age and UA levels should be considered in adults, while gender and SU levels may directly impact muscle mass in children and adolescents. In the case of OA, higher SU levels and gout may serve as risk factors for OA progression, although there are conflicting reports on their relevance. As for IDD, there appears to be a U-shaped relationship between UA and IDD, particularly among Chinese men. And maintaining SU levels within a certain range may help prevent OP and fractures (Table 4). Various mechanisms, such as oxidative stress, deposition of MSU crystals, and inflammation, have been proposed to explain the effects of HUA in these conditions. Emerging evidences from epidemiological studies, together with recently discovered pathogenic pathways, drugs and biotechnologies will help to solve the therapeutic puzzle troubling UA related musculoskeletal diseases.
Author contributions
JZ: Investigation, Software, Writing – original draft. NS: Funding acquisition, Investigation, Writing – original draft. WZ: Investigation, Writing – original draft. WY: Investigation, Writing – original draft. XQ: Writing – review & editing. ZL: Writing – review & editing. GX: Funding acquisition, Investigation, Writing – original draft, Writing – review & editing.
Funding
The author(s) declare financial support was received for the research, authorship, and/or publication of this article. This study was supported by National Natural Science Foundation of China (82204820), and Dalian Medical Science Research Plan (21Z11010). The funders had no role in the study design, decision to publish, or preparation of the manuscript.
Acknowledgments
We would like to thank all the authors in the study.
Conflict of interest
The authors declare that the research was conducted in the absence of any commercial or financial relationships that could be construed as a potential conflict of interest.
Generative AI statement
The author(s) declare that no Generative AI was used in the creation of this manuscript.
Publisher’s note
All claims expressed in this article are solely those of the authors and do not necessarily represent those of their affiliated organizations, or those of the publisher, the editors and the reviewers. Any product that may be evaluated in this article, or claim that may be made by its manufacturer, is not guaranteed or endorsed by the publisher.
Abbreviations
ADMA, asymmetric dimethylarginine; ADP, adenosine diphosphate; AMP, adenosine 5’-monophosphate; Akt, protein kinase B; ATP, adenosine triphosphate; BMD, bone mineral density; EIAKI, exercise-induced acute kidney injury; HUA, hyperuricemia; IDD, intervertebral disc degeneration; IL, interleukin; IR, insulin resistance; IVD, intervertebral disc; LDH, lumbar disc herniation; MCD, McArdle disease; MMP-3, Matrix Metalloproteinase-3; MRI, magnatic resonance imaging; MSU, monosodium urate; NLRP, nucleotide binding domain and leucin-rich repeat containing protein; Nox4, NADPH oxidase-4; OA, osteoarthritis; OP, osteoporosis; RA, rheumatoid arthritis; ROS, reactive oxygen species; RHUC, renal hypouricemia; SU, serum urate; TGF, transforming growth factor; T2D, type 2 diabetes; UA, uric acid.
References
1. Gherghina M-E, Peride I, Tiglis M, Neagu TP, Niculae A, Checherita IA. Uric acid and oxidative stress—Relationship with cardiovascular, metabolic, and renal impairment. Int J Mol Sci. (2022) 23:3188. doi: 10.3390/ijms23063188
2. Mori G, Liuzzi A, Ronda L, Di Palma M, Chegkazi MS, Bui S, et al. Cysteine enrichment mediates co-option of uricase in reptilian skin and transition to uricotelism. Mol Biol Evol. (2023) 40:msad200. doi: 10.1093/molbev/msad200
3. Browne LD, Jaouimaa FZ, Walsh C, Perez-Ruiz F, Richette P, Burke K, et al. Serum uric acid and mortality thresholds among men and women in the Irish health system: A cohort study. Eur J Intern Med. (2021) 84:46–55. doi: 10.1016/j.ejim.2020.10.001
4. Nakagawa T, Tuttle KR, Short RA, Johnson RJ. Hypothesis: fructose-induced hyperuricemia as a causal mechanism for the epidemic of the metabolic syndrome. Nat Clin Pract Nephrol. (2005) 1:80–6. doi: 10.1038/ncpneph0019
5. Chang Y, Yang M, Zhang Y, Xu G, Li Z. Does hyperuricemia correlate with intervertebral disc degeneration? Med Hypotheses. (2020) 140:109673. doi: 10.1016/j.mehy.2020.109673
6. Chen-Xu M, Yokose C, Rai SK, Pillinger MH, Choi HK. Contemporary prevalence of gout and hyperuricemia in the United States and decadal trends: the national health and nutrition examination survey, 2007-2016. Arthritis Rheumatol. (2019) 71:991–9. doi: 10.1002/art.40807
7. Li L ZY, Zeng C. Update on the epidemiology, genetics, and therapeutic options of hyperuricemia. Am J Transl Res. (2020) 17:3167–81.
8. Song P, Wang H, Xia W, Chang X, Wang M, An L. Prevalence and correlates of hyperuricemia in the middle-aged and older adults in China. Sci Rep. (2018) 8:4314. doi: 10.1038/s41598-018-22570-9
9. Park JH, Jo YI, Lee JH. Renal effects of uric acid: hyperuricemia and hypouricemia. Korean J Intern Med. (2020) 35:1291–304. doi: 10.3904/kjim.2020.410
10. Ponticelli C, Podesta MA, Moroni G. Hyperuricemia as a trigger of immune response in hypertension and chronic kidney disease. Kidney Int. (2020) 98:1149–59. doi: 10.1016/j.kint.2020.05.056
11. Petreski T, Ekart R, Hojs R, Bevc S. Hyperuricemia, the heart, and the kidneys - to treat or not to treat? Ren Fail. (2020) 42:978–86. doi: 10.1080/0886022X.2020.1822185
12. Eleftheriadis T, Golphinopoulos S, Pissas G, Stefanidis I. Asymptomatic hyperuricemia and chronic kidney disease: Narrative review of a treatment controversial. J Adv Res. (2017) 8:555–60. doi: 10.1016/j.jare.2017.05.001
13. Li L, Yang C, Zhao Y, Zeng X, Liu F, Fu P. Is hyperuricemia an independent risk factor for new-onset chronic kidney disease? A systematic Rev meta-analysis based observational cohort Stud BMC Nephrol. (2014) 15:122. doi: 10.1186/1471-2369-15-122
14. Feig DI, Kang DH, Johnson RJ. Uric acid and cardiovascular risk. N Engl J Med. (2008) 359:1811–21. doi: 10.1056/NEJMra0800885
15. Moulin SR, Baldo MP, Souza JB, Luchi WM, Capingana DP, Magalhaes P, et al. Distribution of serum uric acid in black africans and its association with cardiovascular risk factors. J Clin Hypertens (Greenwich). (2017) 19:45–50. doi: 10.1111/jch.2017.19.issue-1
16. Kleber ME, Delgado G, Grammer TB, Silbernagel G, Huang J, Kramer BK, et al. Uric acid and cardiovascular events: A mendelian randomization study. J Am Soc Nephrol. (2015) 26:2831–8. doi: 10.1681/ASN.2014070660
17. Aktas G, Khalid A, Kurtkulagi O, Duman TT, Bilgin S, Kahveci G, et al. Poorly controlled hypertension is associated with elevated serum Uric Acid to HDL-cholesterol ratio: A cross-sectional cohort study. Postgrad Med. (2022) 134(3):297–302. doi: 10.1080/00325481.2022.2039007
18. Kodama S, Saito K, Yachi Y, Asumi M, Sugawara A, Totsuka K, et al. Association between serum uric acid and development of type 2 diabetes. Diabetes Care. (2009) 32:1737–42. doi: 10.2337/dc09-0288
19. Bhole V, Choi JW, Kim SW, de Vera M, Choi H. Serum uric acid levels and the risk of type 2 diabetes: a prospective study. Am J Med. (2010) 123:957–61. doi: 10.1016/j.amjmed.2010.03.027
20. van der Schaft N, Brahimaj A, Wen KX, Franco OH, Dehghan A. The association between serum uric acid and the incidence of prediabetes and type 2 diabetes mellitus: The Rotterdam Study. PloS One. (2017) 12:e0179482. doi: 10.1371/journal.pone.0179482
21. Mauer M, Doria A. Uric acid and risk of diabetic kidney disease. J Nephrol. (2020) 33:995–9. doi: 10.1007/s40620-020-00796-z
22. Scirocco C, Rutigliano IM, Finucci A, Iagnocco A. Musculoskeletal ultrasonography in gout. Med Ultrason. (2015) 17:535–40. doi: 10.11152/mu.2013.2066.174.msk
23. Liu X, Chen X, Hu F, Xia X, Hou L, Zhang G, et al. Higher uric acid serum levels are associated with sarcopenia in west China: a cross-sectional study. BMC Geriatr. (2022) 22:121. doi: 10.1186/s12877-022-02817-x
24. Teng GG, Leung YY, Ang LW, Yuan JM, Koh WP. Gout and risk of knee replacement for severe knee osteoarthritis in the Singapore Chinese Health Study. Osteoarthritis Cartilage. (2017) 25:1962–8. doi: 10.1016/j.joca.2017.07.017
25. Wang S, Pillinger MH, Krasnokutsky S, Barbour KE. The association between asymptomatic hyperuricemia and knee osteoarthritis: data from the third National Health and Nutrition Examination Survey. Osteoarthritis Cartilage. (2019) 27:1301–8. doi: 10.1016/j.joca.2019.05.013
26. Yang M, Wang N, Zhang W, Sun T, Zhang D, Chang Y, et al. The dual effect of abnormal serum uric acid on intervertebral disc degeneration. Oxid Med Cell Longev. (2021) 2021:2362799. doi: 10.1155/2021/2362799
27. Neogi T, Krasnokutsky S, Pillinger MH. Urate and osteoarthritis: Evidence for a reciprocal relationship. Joint Bone Spine. (2019) 86:576–82. doi: 10.1016/j.jbspin.2018.11.002
28. Wei J. High prevalence and burden of osteoarthritis in China. Lancet Rheumatol. (2020) 2:e127–8. doi: 10.1016/S2665-9913(20)30034-5
29. Hootman JM, Helmick CG, Barbour KE, Theis KA, Boring MA. Updated projected prevalence of self-reported doctor-diagnosed arthritis and arthritis-attributable activity limitation among US adults, 2015-2040. Arthritis Rheumatol. (2016) 68:1582–7. doi: 10.1002/art.39692
30. Mayhew AJ, Amog K, Phillips S, Parise G, McNicholas PD, de Souza RJ, et al. The prevalence of sarcopenia in community-dwelling older adults, an exploration of differences between studies and within definitions: a systematic review and meta-analyses. Age Ageing. (2019) 48:48–56. doi: 10.1093/ageing/afy106
31. Zhu L, Yu C, Zhang X, Yu Z, Zhan F, Yu X, et al. The treatment of intervertebral disc degeneration using Traditional Chinese Medicine. J Ethnopharmacol. (2020) 263:113117. doi: 10.1016/j.jep.2020.113117
32. Hosoyamada M. Hypothetical mechanism of exercise-induced acute kidney injury associated with renal hypouricemia. Biomedicines. (2021) 9:1847. doi: 10.3390/biomedicines9121847
33. Song Y, March J. Hyperuricemia and the small intestine: Transport mechanisms and co-morbidities. Biotechnol Notes. (2022) 3:32–7. doi: 10.1016/j.biotno.2022.05.001
34. Mandal AK, Mount DB. The molecular physiology of uric acid homeostasis. Annu Rev Physiol. (2015) 77:323–45. doi: 10.1146/annurev-physiol-021113-170343
35. Mandal AK, Mercado A, Foster A, Zandi-Nejad K, Mount DB. Uricosuric targets of tranilast. Pharmacol Res Perspect. (2017) 5:e00291. doi: 10.1002/prp2.291
36. Miller SG, Hafen PS, Brault JJ. Increased adenine nucleotide degradation in skeletal muscle atrophy. Int J Mol Sci. (2019) 21:88. doi: 10.3390/ijms21010088
37. Raivio KO, Becker A, Meyer LJ, Greene ML, Nuki G, Seegmiller JE. Stimulation of human purine synthesis de novo by fructose infusion. Metabolism: Clin Exp. (1975) 24:861–9. doi: 10.1016/0026-0495(75)90133-X
38. Lanaspa MA, Sanchez-Lozada LG, Choi Y-J, Cicerchi C, Kanbay M, Roncal-Jimenez CA, et al. Uric acid induces hepatic steatosis by generation of mitochondrial oxidative stress: potential role in fructose-dependent and -independent fatty liver. J Biol Chem. (2012) 287:40732–44. doi: 10.1074/jbc.M112.399899
39. Madlala HP, Maarman GJ, Ojuka E. Uric acid and transforming growth factor in fructose-induced production of reactive oxygen species in skeletal muscle. Nutr Rev. (2016) 74:259–66. doi: 10.1093/nutrit/nuv111
40. Kang D-H, Han L, Ouyang X, Kahn AM, Kanellis J, Li P, et al. Uric acid causes vascular smooth muscle cell proliferation by entering cells via a functional urate transporter. Am J Nephrol. (2005) 25:425–33. doi: 10.1159/000087713
41. Hosoya T, Uchida S, Shibata S, Tomioka NH, Matsumoto K, Hosoyamada M. Xanthine oxidoreductase inhibitors suppress the onset of exercise-induced AKI in high HPRT activity urat1-uox double knockout mice. J Am Soc Nephrology: JASN. (2022) 33:326–41. doi: 10.1681/ASN.2021050616
42. Miller SG, Matias C, Hafen PS, Law AS, Witczak CA, Brault JJ. Uric acid formation is driven by crosstalk between skeletal muscle and other cell types. JCI Insight. (2024) 9:e171815. doi: 10.1172/jci.insight.171815
43. Wadley GD, Nicolas MA, Hiam DS, McConell GK. Xanthine oxidase inhibition attenuates skeletal muscle signaling following acute exercise but does not impair mitochondrial adaptations to endurance training. Am J Physiol Endocrinol Metab. (2013) 304:E853–62. doi: 10.1152/ajpendo.00568.2012
44. Jaiswal N, Maurya CK, Arha D, Avisetti DR, Prathapan A, Raj PS, et al. Fructose induces mitochondrial dysfunction and triggers apoptosis in skeletal muscle cells by provoking oxidative stress. Apoptosis. (2015) 20:930–47. doi: 10.1007/s10495-015-1128-y
45. Klück V, Cabău G, Mies L, Bukkems F, van Emst L, Bakker R, et al. TGF-β is elevated in hyperuricemic individuals and mediates urate-induced hyperinflammatory phenotype in human mononuclear cells. Arthritis Res Ther. (2023) 25:30. doi: 10.1186/s13075-023-03001-1
46. Sturrock A, Cahill B, Norman K, Huecksteadt TP, Hill K, Sanders K, et al. Transforming growth factor-beta1 induces Nox4 NAD(P)H oxidase and reactive oxygen species-dependent proliferation in human pulmonary artery smooth muscle cells. Am J Physiol Lung Cell Mol Physiol 2. (2006) 90:L661–73. doi: 10.1152/ajplung.00269.2005
47. Lima WG, Martins-Santos ME, Chaves VE. Uric acid as a modulator of glucose and lipid metabolism. Biochimie. (2015) 116:17–23. doi: 10.1016/j.biochi.2015.06.025
48. So A, Thorens B. Uric acid transport and disease. J Clin Invest. (2010) 120:1791–9. doi: 10.1172/JCI42344
49. Baldwin W, McRae S, Marek G, Wymer D, Pannu V, Baylis C, et al. Hyperuricemia as a mediator of the proinflammatory endocrine imbalance in the adipose tissue in a murine model of the metabolic syndrome. Diabetes. (2011) 60:1258–69. doi: 10.2337/db10-0916
50. Zhu Y, Hu Y, Huang T, Zhang Y, Li Z, Luo C, et al. High uric acid directly inhibits insulin signalling and induces insulin resistance. Biochem Biophys Res Commun. (2014) 447:707–14. doi: 10.1016/j.bbrc.2014.04.080
51. Yuan H, Hu Y, Zhu Y, Zhang Y, Luo C, Li Z, et al. Metformin ameliorates high uric acid-induced insulin resistance in skeletal muscle cells. Mol Cell Endocrinol. (2017) 443:138–45. doi: 10.1016/j.mce.2016.12.025
52. Maarman GJ, Andrew BM, Blackhurst DM, Ojuka EO. Melatonin protects against uric acid-induced mitochondrial dysfunction, oxidative stress, and triglyceride accumulation in C2C12 myotubes. J Appl Physiol. (1985) 122:1003–10. doi: 10.1152/japplphysiol.00873.2016
53. Dalbeth N, Stamp L. Hyperuricaemia and gout: time for a new staging system? Ann Rheum Dis. (2014) 73:1598–600. doi: 10.1136/annrheumdis-2014-205304
55. Martinon F, Pétrilli V, Mayor A, Tardivel A, Tschopp J. Gout-associated uric acid crystals activate the NALP3 inflammasome. Nature. (2006) 440:237–41. doi: 10.1038/nature04516
56. Misawa T, Takahama M, Kozaki T, Lee H, Zou J, Saitoh T, et al. Microtubule-driven spatial arrangement of mitochondria promotes activation of the NLRP3 inflammasome. Nat Immunol. (2013) 14:454–60. doi: 10.1038/ni.2550
57. Liu W, Peng J, Wu Y, Ye Z, Zong Z, Wu R, et al. Immune and inflammatory mechanisms and therapeutic targets of gout: An update. Int Immunopharmacol. (2023) 121:110466. doi: 10.1016/j.intimp.2023.110466
58. Park K, Shin I, Kim Y, Kang H, Oh SJ, Jang E, et al. A novel NLRP3 inhibitor as a therapeutic agent against monosodium urate-induced gout. Front Immunol. (2023) 14:1307739. doi: 10.3389/fimmu.2023.1307739
59. Wang Z, Liu J, Mou Y, Liao W, Li Y, Liu J, et al. Anti-inflammatory and uric acid lowering effects of Euodiae fructus on hyperuricemia and gout mice. Front Pharmacol. (2024) 15:1296075. doi: 10.3389/fphar.2024.1296075
60. Shi L, Liang T, Yang F, Zhu FF, Liu J, Jiang JQ, et al. Matrix Metalloproteinase-3 induces proteoglycan degradation in gouty arthritis model. Gene. (2021) 765:145120. doi: 10.1016/j.gene.2020.145120
61. VanItallie TB. Gout: epitome of painful arthritis. Metabolism: Clin Exp. (2010) 59 Suppl 1:S32–6. doi: 10.1016/j.metabol.2010.07.009
62. Choi HK, Zhu Y, Mount DB. Genetics of gout. Curr Opin In Rheumatol. (2010) 22:144–51. doi: 10.1097/BOR.0b013e32833645e8
63. Merriman TR. An update on the genetic architecture of hyperuricemia and gout. Arthritis Res Ther. (2015) 17:98. doi: 10.1186/s13075-015-0609-2
64. Vávra J, Mančíková A, Pavelcová K, Hasíková L, Bohatá J, Stibůrková B. Functional characterization of rare variants in OAT1/SLC22A6 and OAT3/SLC22A8 urate transporters identified in a gout and hyperuricemia cohort. Cells. (2022) 11:1063. doi: 10.3390/cells11071063
65. Sun M, Sun W, Zhao X, Li Z, Dalbeth N, Ji A, et al. A machine learning-assisted model for renal urate underexcretion with genetic and clinical variables among Chinese men with gout. Arthritis Res Ther. (2022) 24:67. doi: 10.1186/s13075-022-02755-4
66. Fernandez-Torres J, Martinez-Nava GA, Oliviero F, Lopez-Reyes AG, Martinez-Flores K, Garrido-Rodriguez D, et al. Common gene variants interactions related to uric acid transport are associated with knee osteoarthritis susceptibility. Connect Tissue Res. (2019) 60:219–29. doi: 10.1080/03008207.2018.1483359
67. Zhao P, Hu HZ, Chen XT, Jiang QY, Yu XZ, Cen XL, et al. Mild hyperthermia enhanced synergistic uric acid degradation and multiple ROS elimination for an effective acute gout therapy. J Nanobiotechnology. (2024) 22:275. doi: 10.1186/s12951-024-02539-9
68. Toprover M, Krasnokutsky S, Pillinger MH. Gout in the spine: imaging, diagnosis, and outcomes. Curr Rheumatol Rep. (2015) 17:70. doi: 10.1007/s11926-015-0547-7
69. Chang Y, Yang M, Zhang Y, Xu G, Li Z. Does hyperuricemia correlate with intervertebral disc degeneration? Med Hypotheses. (2020) 140:109673. doi: 10.1016/j.mehy.2020.109673
70. Xu R, Lian D, Xie Y, Mu L, Wu Y, Chen Z, et al. Relationship between serum uric acid levels and osteoporosis. Endocr Connect. (2023) 12:e230040. doi: 10.1530/EC-23-0040
71. Lin K-M, Lu C-L, Hung K-C, Wu P-C, Pan C-F, Wu C-J, et al. The paradoxical role of uric acid in osteoporosis. Nutrients. (2019) 11:2111. doi: 10.3390/nu11092111
72. Chen W, Roncal-Jimenez C, Lanaspa M, Gerard S, Chonchol M, Johnson RJ, et al. Uric acid suppresses 1 alpha hydroxylase in vitro and in vivo. Metabolism: Clin Exp. (2014) 63:150–60. doi: 10.1016/j.metabol.2013.09.018
73. Peng H, Li H, Li C, Chao X, Zhang Q, Zhang Y. Association between vitamin D insufficiency and elevated serum uric acid among middle-aged and elderly Chinese Han women. PloS One. (2013) 8:e61159. doi: 10.1371/journal.pone.0061159
74. Hermann S, Palmowski A, Hermann KGA, Muche B, Léprêtre N, Alexander T, et al. Elevated uric acid is associated with a low bone mineral density in pre- but not post-menopausal women with rheumatoid arthritis: a pilot study. Rheumatol Int. (2024) 44:2497–504. doi: 10.1007/s00296-024-05655-9
75. Ling L, Wei YX, Sun YF, Zhang MJ, Chen J, Luo SY, et al. Effect of androgen on bone metabolism in hyperuricemic rats. Arch Med Sci. (2022) 18:1351–6. doi: 10.5114/aoms.2020.96456
76. Zhang H, Ma K, Li R-M, Li J-N, Gao S-F, Ma L-N. Association between testosterone levels and bone mineral density in females aged 40-60 years from NHANES 2011-2016. Sci Rep. (2022) 12:16426. doi: 10.1038/s41598-022-21008-7
77. McInnes IB, Schett G. The pathogenesis of rheumatoid arthritis. New Engl J Med. (2011) 365:2205–19. doi: 10.1056/NEJMra1004965
78. Bennett JL, Pratt AG, Dodds R, Sayer AA, Isaacs JD. Rheumatoid sarcopenia: loss of skeletal muscle strength and mass in rheumatoid arthritis. Nat Rev Rheumatol. (2023) 19:239–51. doi: 10.1038/s41584-023-00921-9
79. Mazzali M, Kanellis J, Han L, Feng L, Xia Y-Y, Chen Q, et al. Hyperuricemia induces a primary renal arteriolopathy in rats by a blood pressure-independent mechanism. Am J Physiol Renal Physiol. (2002) 282:F991–7. doi: 10.1152/ajprenal.00283.2001
80. Feig DI, Soletsky B, Johnson RJ. Effect of allopurinol on blood pressure of adolescents with newly diagnosed essential hypertension: a randomized trial. JAMA. (2008) 300:924–32. doi: 10.1001/jama.300.8.924
81. Mandal AK, Leask MP, Estiverne C, Choi HK, Merriman TR, Mount DB. Genetic and physiological effects of insulin on human urate homeostasis. Front Physiol. (2021) 12:713710. doi: 10.3389/fphys.2021.713710
82. Toms TE, Panoulas VF, John H, Douglas KMJ, Kitas GD. Methotrexate therapy associates with reduced prevalence of the metabolic syndrome in rheumatoid arthritis patients over the age of 60- more than just an anti-inflammatory effect? A cross sectional study. Arthritis Res Ther. (2009) 11:R110. doi: 10.1186/ar2765
83. Daoussis D, Panoulas V, Toms T, John H, Antonopoulos I, Nightingale P, et al. Uric acid is a strong independent predictor of renal dysfunction in patients with rheumatoid arthritis. Arthritis Res Ther. (2009) 11:R116. doi: 10.1186/ar2775
84. Bacon PA, Raza K, Banks MJ, Townend J, Kitas GD. The role of endothelial cell dysfunction in the cardiovascular mortality of RA. Int Rev Immunol. (2002) 21:1–17. doi: 10.1080/08830180210413
85. Kuo C-F, Yu K-H, Luo S-F, Ko Y-S, Wen M-S, Lin Y-S, et al. Role of uric acid in the link between arterial stiffness and cardiac hypertrophy: a cross-sectional study. Rheumatol (Oxford England). (2010) 49:1189–96. doi: 10.1093/rheumatology/keq095
86. Roddy E, Zhang W, Doherty M. Are joints affected by gout also affected by osteoarthritis? Ann Rheumatic Dis. (2007) 66:1374–7. doi: 10.1136/ard.2006.063768
87. Kawenoki-Minc E, Eyman E, Leo W, Weryńska-Przybylska J. Osteoarthrosis and spondylosis in gouty patients. Analysis of 262 cases of gout. Reumatologia. (1974) 12:267–7.
88. Li Y-C, Chou Y-C, Chen H-C, Lu C-C, Chang D-M. Interleukin-6 and interleukin-17 are related to depression in patients with rheumatoid arthritis. Int J Rheumatic Dis. (2019) 22:980–5. doi: 10.1111/apl.2019.22.issue-6
89. Lozada-Mellado M, Llorente L, Hinojosa-Azaola A, Ogata-Medel M, Valdez-Echeverría RD, Lira-Reyes AR, et al. Inflammatory profile in patients with rheumatoid arthritis and sarcopenia. Clin Rheumatol. (2024) 43:1865–70. doi: 10.1007/s10067-024-06974-9
90. Crisan TO, Cleophas MCP, Oosting M, Lemmers H, Toenhake-Dijkstra H, Netea MG, et al. Soluble uric acid primes TLR-induced proinflammatory cytokine production by human primary cells via inhibition of IL-1Ra. Ann Rheumatic Dis. (2016) 75:755–62. doi: 10.1136/annrheumdis-2014-206564
91. Beavers KM, Beavers DP, Serra MC, Bowden RG, Wilson RL. Low relative skeletal muscle mass indicative of sarcopenia is associated with elevations in serum uric acid levels: findings from NHANES III. J Nutr Health Aging. (2009) 13:177–82. doi: 10.1007/s12603-009-0054-5
92. Kaczor JJ, Robertshaw HA, Tarnopolsky MA. Higher oxidative stress in skeletal muscle of McArdle disease patients. Mol Genet Metab Rep. (2017) 12:69–75. doi: 10.1016/j.ymgmr.2017.05.009
93. Tanaka KI, Kanazawa I, Notsu M, Sugimoto T. Higher serum uric acid is a risk factor of reduced muscle mass in men with type 2 diabetes mellitus. Exp Clin Endocrinol Diabetes. (2021) 129:50–5. doi: 10.1055/a-0805-2197
94. Nambu H, Takada S, Maekawa S, Matsumoto J, Kakutani N, Furihata T, et al. Inhibition of xanthine oxidase in the acute phase of myocardial infarction prevents skeletal muscle abnormalities and exercise intolerance. Cardiovasc Res. (2021) 117:805–19. doi: 10.1093/cvr/cvaa127
95. Cruz-Jentoft AJ, Sayer AA. Sarcopenia. Lancet. (2019) 393:2636–46. doi: 10.1016/S0140-6736(19)31138-9
96. Macchi C, Molino-Lova R, Polcaro P, Guarducci L, Lauretani F, Cecchi F, et al. Higher circulating levels of uric acid are prospectively associated with better muscle function in older persons. Mech Ageing Dev. (2008) 129:522–7. doi: 10.1016/j.mad.2008.04.008
97. Dong XW, Tian HY, He J, Wang C, Qiu R, Chen YM. Elevated serum uric acid is associated with greater bone mineral density and skeletal muscle mass in middle-aged and older adults. PloS One. (2016) 11:e0154692. doi: 10.1371/journal.pone.0154692
98. Molino-Lova R, Sofi F, Pasquini G, Vannetti F, Del Ry S, Vassalle C, et al. Higher uric acid serum levels are associated with better muscle function in the oldest old: Results from the Mugello Study. Eur J Intern Med. (2017) 41:39–43. doi: 10.1016/j.ejim.2017.03.014
99. Alvim RO, Siqueira JH, Zaniqueli D, Dutra DM, Oliosa PR, Mill JG. Influence of muscle mass on the serum uric acid levels in children and adolescents. Nutr Metab Cardiovasc Dis. (2020) 30:300–5. doi: 10.1016/j.numecd.2019.08.019
100. Huang C, Niu K, Kobayashi Y, Guan L, Momma H, Cui Y, et al. An inverted J-shaped association of serum uric acid with muscle strength among Japanese adult men: a cross-sectional study. BMC Musculoskelet Disord. (2013) 14:258. doi: 10.1186/1471-2474-14-258
101. Garcia-Esquinas E, Rodriguez-Artalejo F. Association between serum uric acid concentrations and grip strength: Is there effect modification by age? Clin Nutr. (2018) 37:566–72. doi: 10.1016/j.clnu.2017.01.008
102. Yin H, Liu N, Chen J. The role of the intestine in the development of hyperuricemia. Front In Immunol. (2022) 13:845684. doi: 10.3389/fimmu.2022.845684
103. Tsushima Y, Nishizawa H, Tochino Y, Nakatsuji H, Sekimoto R, Nagao H, et al. Uric acid secretion from adipose tissue and its increase in obesity. J Biol Chem. (2013) 288:27138–49. doi: 10.1074/jbc.M113.485094
104. Johnson RJ, Nakagawa T, Sanchez-Lozada LG, Shafiu M, Sundaram S, Le M, et al. Sugar, uric acid, and the etiology of diabetes and obesity. Diabetes. (2013) 62:3307–15. doi: 10.2337/db12-1814
106. Yamamoto T, Moriwaki Y, Takahashi S. Effect of ethanol on metabolism of purine bases (hypoxanthine, xanthine, and uric acid). Clinica Chimica Acta; Int J Clin Chem. (2005) 356:35–57. doi: 10.1016/j.cccn.2005.01.024
107. Lee MJ, Khang AR, Kang YH, Yun MS, Yi D. Synergistic interaction between hyperuricemia and abdominal obesity as a risk factor for metabolic syndrome components in korean population. Diabetes Metab J. (2022) 46:756–66. doi: 10.4093/dmj.2021.0166
108. Lv Q, Meng XF, He FF, Chen S, Su H, Xiong J, et al. High serum uric acid and increased risk of type 2 diabetes: a systemic review and meta-analysis of prospective cohort studies. PloS One. (2013) 8:e56864. doi: 10.1371/journal.pone.0056864
109. Quiñones Galvan A, Natali A, Baldi S, Frascerra S, Sanna G, Ciociaro D, et al. Effect of insulin on uric acid excretion in humans. Am J Physiol. (1995) 268:E1–5. doi: 10.1152/ajpendo.1995.268.1.E1
110. Muscelli E, Natali A, Bianchi S, Bigazzi R, Galvan AQ, Sironi AM, et al. Effect of insulin on renal sodium and uric acid handling in essential hypertension. Am J Hypertens. (1996) 9:746–52. doi: 10.1016/0895-7061(96)00098-2
111. Fabbrini E, Serafini M, Colic Baric I, Hazen SL, Klein S. Effect of plasma uric acid on antioxidant capacity, oxidative stress, and insulin sensitivity in obese subjects. Diabetes. (2014) 63:976–81. doi: 10.2337/db13-1396
112. Fiorentino TV, Sesti F, Succurro E, Pedace E, Andreozzi F, Sciacqua A, et al. Higher serum levels of uric acid are associated with a reduced insulin clearance in non-diabetic individuals. Acta Diabetol. (2018) 55:835–42. doi: 10.1007/s00592-018-1153-8
113. Wang XH, Jiang WR, Zhang MY, Shi YX, Ji YP, Li CJ, et al. The visceral fat area to leg muscle mass ratio is significantly associated with the risk of hyperuricemia among women: a cross-sectional study. Biol Sex Differ. (2021) 12:17. doi: 10.1186/s13293-021-00360-9
114. DiMauro S, Bruno C. Glycogen storage diseases of muscle. Curr Opin Neurol. (1998) 11:477–84. doi: 10.1097/00019052-199810000-00010
115. Mineo I, Kono N, Hara N, Shimizu T, Yamada Y, Kawachi M, et al. Myogenic hyperuricemia. A common pathophysiologic feature of glycogenosis types III, V, and VII. New Engl J Med. (1987) 317:75–80. doi: 10.1056/NEJM198707093170203
116. Linder N, Rapola J, Raivio KO. Cellular expression of xanthine oxidoreductase protein in normal human tissues. Lab Invest. (1999) 79:967–74.
117. Derbre F, Ferrando B, Gomez-Cabrera MC, Sanchis-Gomar F, Martinez-Bello VE, Olaso-Gonzalez G, et al. Inhibition of xanthine oxidase by allopurinol prevents skeletal muscle atrophy: role of p38 MAPKinase and E3 ubiquitin ligases. PloS One. (2012) 7:e46668. doi: 10.1371/journal.pone.0046668
118. Beberashvili I, Sinuani I, Azar A, Shapiro G, Feldman L, Stav K, et al. Serum uric acid as a clinically useful nutritional marker and predictor of outcome in maintenance hemodialysis patients. Nutrition. (2015) 31:138–47. doi: 10.1016/j.nut.2014.06.012
119. Xiao X, Yi C, Peng Y, Ye H, Wu H, Wu M, et al. The association between serum uric acid and appendicular skeletal muscle mass and the effect of their interaction on mortality in patients on peritoneal dialysis. Kidney Blood Press Res. (2020) 45:969–81. doi: 10.1159/000510746
120. Floriano JP, Nahas PC, de Branco FMS, Dos Reis AS, Rossato LT, Santos HO, et al. Serum uric acid is positively associated with muscle mass and strength, but not with functional capacity, in kidney transplant patients. Nutrients. (2020) 12:2390. doi: 10.3390/nu12082390
121. Werdyani S, Aitken D, Gao Z, Liu M, Randell EW, Rahman P, et al. Metabolomic signatures for the longitudinal reduction of muscle strength over 10 years. Skelet Muscle. (2022) 12:4. doi: 10.1186/s13395-022-00286-9
122. Mou L, Zhu L, Chen X, Hu Y, Zhu H, Xu Y. Genotype and phenotype of renal hypouricemia: A single-center study from China. Mol Diagnosis Ther. (2023) 28:87–99. doi: 10.1007/s40291-023-00683-w
123. Kuwabara M, Niwa K, Ohtahara A, Hamada T, Miyazaki S, Mizuta E, et al. Prevalence and complications of hypouricemia in a general population: A large-scale cross-sectional study in Japan. PloS One. (2017) 12:e0176055. doi: 10.1371/journal.pone.0176055
124. Aoki Y, Sofue T, Kawakami R, Ozaki T, Manabe M, Kanda K, et al. Prevalence and factors related to hypouricemia and hyperuricemia in schoolchildren: results of a large-scale cross-sectional population-based study conducted in Japan. Sci Rep. (2022) 12:17848. doi: 10.1038/s41598-022-19724-1
125. Kraus VB, Blanco FJ, Englund M, Karsdal MA, Lohmander LS. Call for standardized definitions of osteoarthritis and risk stratification for clinical trials and clinical use. Osteoarthritis Cartilage. (2015) 23:1233–41. doi: 10.1016/j.joca.2015.03.036
126. Mobasheri A, Saarakkala S, Finnilä M, Karsdal MA, Bay-Jensen A-C, van Spil WE. Recent advances in understanding the phenotypes of osteoarthritis. F1000Res. (2019) 8:F1000 Faculty Rev-2091. doi: 10.12688/f1000research
127. Ma CA, Leung YY. Exploring the link between uric acid and osteoarthritis. Front In Med. (2017) 4:225. doi: 10.3389/fmed.2017.00225
128. Bougault C, Gosset M, Houard X, Salvat C, Godmann L, Pap T, et al. Stress-induced cartilage degradation does not depend on the NLRP3 inflammasome in human osteoarthritis and mouse models. Arthritis Rheumatism. (2012) 64:3972–81. doi: 10.1002/art.v64.12
129. Acheson RM, Collart AB. New Haven survey of joint diseases. XVII. Relationship between some systemic characteristics and osteoarthrosis in a general population. Ann Rheum Dis. (1975) 34:379–87. doi: 10.1136/ard.34.5.379
130. Anderson JJ, Felson DT. Factors associated with osteoarthritis of the knee in the first national Health and Nutrition Examination Survey (HANES I). Evidence for an association with overweight, race, and physical demands of work. Am J Epidemiol. (1988) 128:179–89. doi: 10.1093/oxfordjournals.aje.a114939
131. Hart DJ DD, Spector TD. Association between metabolic factors and knee osteoarthritis in women: the Chingford Study. J Rheumatol. (1995) 22:1118–23.
132. Sun Y, Brenner H, Sauerland S, Gunther KP, Puhl W, Sturmer T. Serum uric acid and patterns of radiographic osteoarthritis–the Ulm Osteoarthritis Study. Scand J Rheumatol. (2000) 29:380–6. doi: 10.1080/030097400447589
133. Ding X, Zeng C, Wei J, Li H, Yang T, Zhang Y, et al. The associations of serum uric acid level and hyperuricemia with knee osteoarthritis. Rheumatol Int. (2016) 36:567–73. doi: 10.1007/s00296-015-3418-7
134. Krasnokutsky S, Oshinsky C, Attur M, Ma S, Zhou H, Zheng F, et al. Serum urate levels predict joint space narrowing in non-gout patients with medial knee osteoarthritis. Arthritis Rheumatol. (2017) 69:1213–20. doi: 10.1002/art.40069
135. Zhao J, Sha B, Zeng L, Dou Y, Huang H, Liang G, et al. J-shaped association of serum uric acid concentrations with all-cause mortality in individuals with osteoarthritis: A prospective cohort study. Joint Bone Spine. (2024) 91:105679. doi: 10.1016/j.jbspin.2023.105679
136. Kim SK, Kwak SG, Choe JY. Serum uric acid level is not associated with osteoarthritis in Korean population: data from the Seventh Korea National Health and Nutrition Examination Survey 2016. Rheumatol Int. (2018) 38:2077–85. doi: 10.1007/s00296-018-4141-y
137. Zhu J, Wang Y, Chen Y, Li X, Yang Z, Li H. Association between hyperuricemia, gout, urate lowering therapy, and osteoarthritis: A protocol for a systematic review and meta-analysis. Med (Baltimore). (2020) 99:e21610. doi: 10.1097/MD.0000000000021610
138. Yu KH, Chen DY, Chen JH, Chen SY, Chen SM, Cheng TT, et al. Management of gout and hyperuricemia: Multidisciplinary consensus in Taiwan. Int J Rheum Dis. (2018) 21:772–87. doi: 10.1111/apl.2018.21.issue-4
139. Lally EV, Zimmermann B, Ho G Jr., Kaplan SR. Urate-mediated inflammation in nodal osteoarthritis: clinical and roentgenographic correlations. Arthritis Rheum. (1989) 32:86–90. doi: 10.1002/anr.1780320115
140. Roddy E, Zhang W, Doherty M. Are joints affected by gout also affected by osteoarthritis? Ann Rheum Dis. (2007) 66:1374–7. doi: 10.1136/ard.2006.063768
141. Roddy E, Zhang W, Doherty M. Gout and nodal osteoarthritis: a case-control study. Rheumatol (Oxford). (2008) 47:732–3. doi: 10.1093/rheumatology/ken087
142. Bevis M, Marshall M, Rathod T, Roddy E. The association between gout and radiographic hand, knee and foot osteoarthritis: a cross-sectional study. BMC Musculoskelet Disord. (2016) 17:169. doi: 10.1186/s12891-016-1032-9
143. Howard RG, Samuels J, Gyftopoulos S, Krasnokutsky S, Leung J, Swearingen CJ, et al. Presence of gout is associated with increased prevalence and severity of knee osteoarthritis among older men: results of a pilot study. J Clin Rheumatol. (2015) 21:63–71. doi: 10.1097/RHU.0000000000000217
144. Kuo CF, Grainge MJ, Mallen C, Zhang W, Doherty M. Comorbidities in patients with gout prior to and following diagnosis: case-control study. Ann Rheum Dis. (2016) 75:210–7. doi: 10.1136/annrheumdis-2014-206410
145. Hsu CM, Hsu CC, Wu RW, Huang CC, Chen YC. Interplay between fat, muscle, bone mass, and oteophytes and risk for tophaceous gout. J Investig Med. (2023) 71:58–61. doi: 10.1136/jim-2022-002407
146. Muehleman C, Li J, Aigner T, Rappoport L, Mattson E, Hirschmugl C, et al. Association between crystals and cartilage degeneration in the ankle. J Rheumatol. (2008) 35:1108–17.
147. Denoble AE, Huffman KM, Stabler TV, Kelly SJ, Hershfield MS, McDaniel GE, et al. Uric acid is a danger signal of increasing risk for osteoarthritis through inflammasome activation. Proc Natl Acad Sci U.S.A. (2011) 108:2088–93. doi: 10.1073/pnas.1012743108
148. Huang Z, Kraus VB. Does lipopolysaccharide-mediated inflammation have a role in OA? Nat Rev Rheumatol. (2016) 12:123–9. doi: 10.1038/nrrheum.2015.158
149. Goldring SR, Goldring MB. The role of cytokines in cartilage matrix degeneration in osteoarthritis. Clin Orthop Relat Res. (2004) 427 Suppl:S27–36. doi: 10.1097/01.blo.0000144854.66565.8f
150. Giclas PC, Ginsberg MH, Cooper NR. Immunoglobulin G independent activation of the classical complement pathway by monosodium urate crystals. J Clin Invest. (1979) 63:759–64. doi: 10.1172/JCI109360
151. Fields TR, Abramson SB, Weissmann G, Kaplan AP, Ghebrehiwet B. Activation of the alternative pathway of complement by monosodium urate crystals. Clin Immunol Immunopathol. (1983) 26:249–57. doi: 10.1016/0090-1229(83)90143-5
152. Go DJ, Kim DH, Kim JY, Guermazi A, Crema MD, Hunter DJ, et al. Serum uric acid and knee osteoarthritis in community residents without gout: a longitudinal study. Rheumatol (Oxford). (2021) 60:4581–90. doi: 10.1093/rheumatology/keab048
153. Huang SW, Wu CW, Lin LF, Liou TH, Lin HW. Gout can increase the risk of receiving rotator cuff tear repair surgery. Am J Sports Med. (2017) 45:2355–63. doi: 10.1177/0363546517704843
154. Duprez TP MJ, Vande Berg BC, Noel HM, Munting EA, Maldague BE. Gout in the cervical spine: MR pattern mimicking diskovertebral infection. AJNR Am J Neuroradiol. (1996) 17:151–3.
155. Sanmillan Blasco JL, Vidal Sarro N, Marnov A, Acebes Martin JJ. Cervical cord compression due to intradiscal gouty tophus: brief report. Spine (Phila Pa. (2012) 1976) 37:E1534–6. doi: 10.1097/BRS.0b013e31826f2886
156. Zhou S, Xiao Y, Liu X, Zhong Y, Yang H. Gout involved the cervical disc and adjacent vertebral endplates misdiagnosed infectious spondylodiscitis on imaging: case report and literature review. BMC Musculoskelet Disord. (2019) 20:425. doi: 10.1186/s12891-019-2813-8
157. Wang R, Gao Y, Wang P, He C, Lu H. Association between serum uric acid and bone mineral density in males from NHANES 2011-2020. Sci Rep. (2024) 14:4292. doi: 10.1038/s41598-024-52147-8
158. Cai Y-T, Li Y-X, Wang L-R, Mo L, Li Y, Zhang S-C. The non-causative role of abnormal serum uric acid in intervertebral disc degeneration: A Mendelian randomization study. JOR Spine. (2024) 7:e1283. doi: 10.1002/jsp2.1283
159. Yang K, Li J, Tao L. Purine metabolism in the development of osteoporosis. BioMed Pharmacother. (2022) 155:113784. doi: 10.1016/j.biopha.2022.113784
160. Xu MZ, Lu K, Yang XF, Ye YW, Xu SM, Shi Q, et al. Association between serum uric acid levels and bone mineral density in patients with osteoporosis: a cross-sectional study. BMC Musculoskelet Disord. (2023) 24:306. doi: 10.1186/s12891-023-06414-w
161. Li Y, Tan J, Tian J, Xu J, Shao H, Zhang J, et al. Cross-sectional analysis of the correlation between serum uric acid and trabecular bone score: NHANES 2005-2008. Sci Rep. (2023) 13:21546. doi: 10.1038/s41598-023-48739-5
162. Lin ZC, Wu JF, Chang CY, Lai KM, Yang HY. Association between serum uric acid level and bone mineral density at multiple skeletal sites in middle-aged and elderly men: a cross-sectional study of a healthy population in Taiwan. Arch Osteoporos. (2022) 17:142. doi: 10.1007/s11657-022-01186-7
163. Ma C, Yu R, Li J, Wang X, Guo J, Xiao E, et al. Association of serum uric acid levels with bone mineral density and the presence of osteoporosis in Chinese patients with Parkinson’s disease: a cross-sectional study. J Bone Miner Metab. (2023) 41:714–26. doi: 10.1007/s00774-023-01446-7
164. Su Y, Ding N, Zhou Y, Yang G, Chai X. The relationship between uric acid and total femur bone mineral density in hypertensive and non-hypertensive populations. Front Endocrinol (Lausanne). (2022) 13:1022031. doi: 10.3389/fendo.2022.1022031
165. Zhang Y, Tan M, Liu B, Zeng M, Zhou Y, Zhang M, et al. Relationship between bone mineral density and hyperuricemia in obesity: A cross-sectional study. Front Endocrinol (Lausanne). (2023) 14:1108475. doi: 10.3389/fendo.2023.1108475
166. Kim S, Lee S, Kwon H. Association between serum uric acid level and bone mineral density in men more than 50 years of age. Front Endocrinol (Lausanne). (2023) 14:1259077. doi: 10.3389/fendo.2023.1259077
167. Robles-Rivera K, Argoty-Pantoja AD, Hidalgo-Bravo A, Quezada-Sanchez AD, Leon-Reyes G, Flores YN, et al. Uric acid levels are associated with bone mineral density in mexican populations: A longitudinal study. Nutrients. (2022) 14:4245. doi: 10.3390/nu14204245
168. Liu M, Chu Q, Yang C, Wang J, Fu M, Zhang Z, et al. The paradoxical relation between serum uric acid and outcomes of hip fracture in older patients after surgery: A 1-year follow-up study. Surgery. (2022) 172:1576–83. doi: 10.1016/j.surg.2022.07.008
169. Xu N, Wang Y, Chen Y, Guo YN, Li RX, Zhou YM, et al. The associations between uric acid with BMDs and risk of the 10-year probability of fractures in Chinese patients with T2DM stratified by age and gender. Endocrine. (2023) 80:520–8. doi: 10.1007/s12020-023-03353-3
170. Xing Y, Liu J, Liu H, Ma H. Relationship of bone turnover markers with serum uric acid-to-creatinine ratio in men and postmenopausal women with type 2 diabetes. Diabetes Metab Syndr Obes. (2022) 15:3205–17. doi: 10.2147/DMSO.S384694
Keywords: uric acid, musculoskeletal diseases, sarcopenia, osteoarthritis, intervertebral disc degeneration, gout, osteoporosis
Citation: Zhang J, Sun N, Zhang W, Yue W, Qu X, Li Z and Xu G (2025) The impact of uric acid on musculoskeletal diseases: clinical associations and underlying mechanisms. Front. Endocrinol. 16:1515176. doi: 10.3389/fendo.2025.1515176
Received: 22 October 2024; Accepted: 15 January 2025;
Published: 04 February 2025.
Edited by:
Maria Felicia Faienza, University of Bari Aldo Moro, ItalyReviewed by:
Nan Jiang, Southern Medical University, ChinaAsim Kumar Mandal, Brigham and Women’s Hospital and Harvard Medical School, United States
Xiyue Chen, Purdue University, United States
Copyright © 2025 Zhang, Sun, Zhang, Yue, Qu, Li and Xu. This is an open-access article distributed under the terms of the Creative Commons Attribution License (CC BY). The use, distribution or reproduction in other forums is permitted, provided the original author(s) and the copyright owner(s) are credited and that the original publication in this journal is cited, in accordance with accepted academic practice. No use, distribution or reproduction is permitted which does not comply with these terms.
*Correspondence: Zhonghai Li, bGl6aG9uZ2hhaXNwaW5lQDEyNi5jb20=; Gang Xu, eHVnYW5nQGRtdS5lZHUuY24=
†These authors have contributed equally to this work