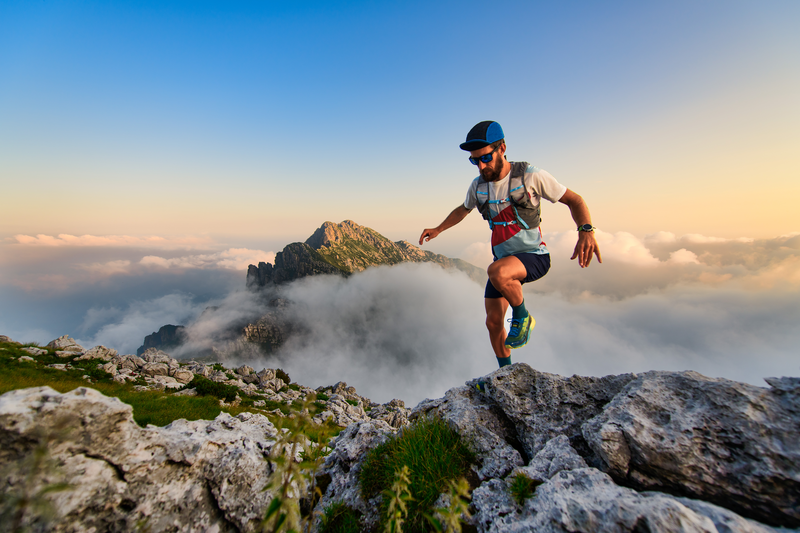
94% of researchers rate our articles as excellent or good
Learn more about the work of our research integrity team to safeguard the quality of each article we publish.
Find out more
ORIGINAL RESEARCH article
Front. Endocrinol. , 24 January 2025
Sec. Cardiovascular Endocrinology
Volume 16 - 2025 | https://doi.org/10.3389/fendo.2025.1467254
This article is part of the Research Topic Screening Remnant Lipid Markers in Cardiometabolic Diseases View all 9 articles
Objective: There is limited epidemiological data regarding the association of blood lipids with thyroid hormones. Thus, the present article aims to explore whether there is an association between non-high-density to high-density lipoprotein cholesterol ratio (NHHR) and thyroid hormones.
Methods: We analyzed samples from 3,881 adults aged 20 years and above who took part in the National Health and Nutrition Examination Survey (NHANES) spanning 2007 to 2012. The study tested for thyroid hormones, including total triiodothyronine (TT3), free triiodothyronine (FT3), total thyroxine (TT4), free thyroxine (FT4), as well as thyroid-stimulating hormone (TSH). Survey-weighted linear regression and restricted cubic spline (RCS) models were employed to investigate the relationship between NHHR and thyroid hormones. Subsequently, subgroup analyses were conducted. In Mendelian randomization (MR), the inverse variance weighting method (IVW) is used as the primary analytical approach.
Results: This study finally comprised 3,881 adults aged 20 years and older. After extensive adjustments for covariables, the regression analysis revealed significant negative associations between NHHR and FT4 (β: -0.11, 95% confidence interval [CI]: -0.18, -0.04), FT4/FT3 (β: -0.06, 95% CI: -0.08, -0.04), and TT4/TT3 (β: -0.001, 95% CI: -0.001, 0.000). Both observational and Mendelian randomization studies suggest that high-density lipoprotein cholesterol, low-density lipoprotein cholesterol, and total cholesterol may not significantly influence the risk of hyperthyroidism or hypothyroidism.
Conclusions: The study indicates negative associations between NHHR and FT4, as well as the ratios of FT4/FT3 and TT4/TT3. This suggests that NHHR may reflect changes in thyroid function, highlighting its potential clinical significance in assessing thyroid function and metabolic health.
Thyroid hormones consist of thyroxine (T4, approximately 80%) and triiodothyronine (T3, approximately 20%) (1). T4 is primarily released by the thyroid gland, whereas only about 20% of T3 is directly produced by the thyroid gland (2). The remaining 80% of T3 is converted from T4 in peripheral tissues by deiodinases (DIO1, DIO2) (2). Among these hormones, T3 is the active form, while T4 primarily serves as a precursor that can be converted into the active form (3). In the bloodstream, most T3 and T4 molecules are bound to serum binding proteins, with only a small fraction circulating freely as free thyroxine (FT4, approximately 0.03%) and free triiodothyronine (FT3, approximately 0.5%) (4). These free and bound portions together constitute total triiodothyronine (TT3) and total thyroxine (TT4) (5). The production of thyroid hormones is controlled by a complex negative feedback system involving thyroid-stimulating hormone (TSH) released by the pituitary gland (3). TSH regulates the synthesis and secretion of T3 and T4, while T3 and T4, in turn, regulate the production of TSH through negative feedback (3). T3 and T4 play crucial roles in many tissues, maintaining normal growth and metabolism, regulating energy expenditure and lipid balance, and playing key roles in the cardiovascular system (6). Studies have shown that abnormal levels of thyroid hormones, whether too high or too low, can have adverse effects on the body (7–9). Low levels of thyroid hormones can lead to coronary heart disease (7, 8), while high levels of thyroid hormones can lead to heart failure (9).
Some research has explored the association between thyroid hormones and lipid metabolism. For instance, research by Kim et al. on the Chinese population identified a significant positive association between FT4 and the levels of both high-density lipoprotein cholesterol (HDL-C) and low-density lipoprotein cholesterol (LDL-C) (10). Conversely, a study involving 2,183 adults found a negative association between FT4 and HDL-C (11). Similarly, Wang et al. reported a negative association between thyroid hormones and hyperlipidemia indicators, such as LDL-C (12). Non-high-density lipoprotein cholesterol (non-HDL-C) represents all cholesterol carried by lipoproteins other than HDL-C, including the cholesterol content in very low-density lipoprotein, low-density lipoproteins, and other lipoprotein particles (13). Furthermore, some research has shown that higher levels of non-HDL-C are linked to mild inflammation, which may facilitate the conversion of TT4 to TT3, thereby elevating TT3 levels (14, 15). However, one study suggested that activating inflammatory mediators and exposure to low temperatures could reduce FT3 levels (16).
Currently, the methods for measuring TT3 and TT4 are primarily immunoassays, but these methods have significant errors and limitations (17). A study questioned the accuracy of immunoassay results for low, normal, and high concentrations of TT3 and validated the results using the gold standard liquid chromatography-tandem mass spectrometry (LC-MS/MS) (18). The results showed that the measurements of TT3 using low-concentration immunoassay methods were not ideal (18). Additionally, a recent study by the IFCC C-STFT used various immunoassay methods to measure TT4 and TT3 in the serum of healthy individuals and compared the results with those reported by isotope dilution tandem mass spectrometry (ID-LC-MS/MS) (19). The findings revealed that most methods did not meet the optimal 5% target set by C-STFT, with only a few TT4 measurements falling within 10% of the reference values (19, 20). Particularly, since the concentration of TT3 is ten times lower than that of TT4, its measurement requires higher sensitivity and precision (21). However, most TT3 measurements exhibited a positive bias, indicating that the detection of TT3 and TT4 needs to be re-standardized (19, 20). Although tandem mass spectrometry provides higher accuracy in measuring TT3 and TT4, its complex operational steps, which require the separation of analytes from binding proteins to measure free thyroid hormones, make it difficult to be widely applied in clinical settings in the short term (22). The assessment of thyroid function primarily relies on thyroid hormones and TSH levels (23). Therefore, it is particularly important to establish new blood lipid indicators that can more accurately reflect fluctuations in these levels for evaluating thyroid function.
The non-high-density lipoprotein cholesterol to high-density lipoprotein cholesterol ratio (NHHR) is increasingly regarded as a comprehensive and innovative parameter for assessing lipid components in atherosclerosis (24). NHHR is associated with atherosclerosis and provides significant predictive value for various diseases. For instance, a study on the Asian population demonstrated an association between NHHR and non-alcoholic fatty liver disease; a one-unit increase in NHHR could lead to a 64.5% increase in the ratio of alanine aminotransferase to aspartate aminotransferase (25, 26). Since the liver is the primary site for converting TT4 to TT3 (27), non-alcoholic fatty liver disease indirectly affects thyroid hormone levels by altering the metabolic rate of liver function (28). Another study indicated that NHHR offers more accurate predictions for metabolic syndrome than traditional lipid markers (29). Metabolic syndrome is characterized by elevated lipid levels, which can disrupt the iodination process of TT4, potentially resulting in increased levels of FT3 and TT3 and a decrease in FT4 (30, 31). Therefore, exploring the association between NHHR and thyroid hormones and functions is scientifically significant and positions NHHR as a novel biomarker, paving new pathways for monitoring and regulating thyroid hormone levels.
Mendelian randomization (MR) analysis uses genetic variation, such as single nucleotide polymorphisms (SNPs), as instrumental variables (32). By exploiting the random allocation of genetic variation, MR excludes the influence of confounding factors and helps identify causal relationships (32). Therefore, the MR method can effectively assess the causal association between NHHR and thyroid hormones.
Based on the background information and prior research, we hypothesize that NHHR, as an innovative biomarker, more accurately reflects fluctuations in thyroid hormone levels. Therefore, our study investigates the association between NHHR and thyroid hormones in American adults using data from the NHANES survey conducted between 2007 and 2012. Additionally, the use of two-sample MR deepens the understanding of the causal relationship between these variables.
This study utilizes data from the NHANES, a cross-sectional survey conducted every two years by the Centers for Disease Control and Prevention (CDC). This program seeks to evaluate the nutritional and health status of residents in the U.S. The survey was performed in accordance with the relevant guidelines and regulations. The survey includes face-to-face interviews and physical examinations, encompassing demographics, socioeconomic status, and health-related information. The research obtained official endorsement from the Research Ethics Review Board in the National Center for Health Statistics, and every individual has signed the corresponding written informed consent (33).
This study utilized data from three consecutive NHANES cycles (2007-2008, 2009-2010, and 2011-2012) because thyroid hormone data were only available during these specific periods. Initially, there were 30,442 participants. However, after excluding individuals under 20 years old (n = 12,729), those without thyroid hormone data (n = 8,974), those missing NHHR data (n = 1), and those with a weight of 0 or missing values (n = 4,857), the final sample size consisted of 3,881 participants. This final sample included individuals both with and without thyroid disorders such as thyroid cancer, hyperthyroidism, and hypothyroidism (Figure 1).
Figure 1. Flowchart of the sample selection from the 2007–2012 National Health and Nutrition Examination Survey (NHANES).
The NHHR was regarded as the exposure variable. The formula used was: NHHR = Non-HDL-C/HDL-C, where Non-HDL-C is calculated as total cholesterol (TC) minus HDL-C. To enhance the normality of the variables, we applied a log transformation on independent variables. The log2-transformed values closely approximate a normal distribution and exhibit homogeneity of variance (Levene’s test P > 0.05).
This study considered thyroid function parameters such as FT3, TT3, FT4, TT4, and TSH as outcome variables, with data sourced from the NHANES laboratory data. The survey utilized competitive binding immunoassay to measure TT3, FT3, and TT4 and employed a two-step enzyme immunoassay for assessing FT4. TSH levels were measured using the third-generation bi-site immunoassay for access to ultra-sensitive human Thyroid-Stimulating Hormone (TSH) (34, 35). Furthermore, our research determined the TT4/TT3 and FT4/FT3 ratios to examine peripheral TT4 metabolic activity and also assessed the FT4/TT4 ratio to investigate how thyroid hormones interact with their binding proteins.
Drawing on prior research (36, 37), this study included multiple factors that could affect the relationship between NHHR and thyroid hormones. These factors include age, gender, race, marital status, urine iodine concentration, moderate recreational activities, education level, poverty-to-income ratio (PIR), body mass index (BMI), smoking status, alcohol consumption, diabetes, hypertension, lipid-lowering medications and thyroid hormone medications. Detailed information on all covariables can be found in Supplementary Material 1.
Sampling weights, stratification, and clustering factors from the NHANES study were integrated into each statistical analysis to mirror the complex multi-stage sampling methodology precisely. This methodology guaranteed accurate estimation results and mitigated the risk of overstating statistical significance. When selecting weights, the NHANES official guidelines recommend first determining the smallest population group. In this study, we chose the specific sub-weight WTSAF2YR. For the combined survey cycles, according to the NHANES analysis guidelines, a new sampling weight is created by dividing the two-year weight of each cycle by three, resulting in a new weight. Continuous variables are reported as means ± standard errors and tested by one-way analysis of variance (ANOVA), while categorical variables are expressed as frequencies with the corresponding survey-weight percentages and assessed by weighted chi-square tests. Restricted cubic spline (RCS) analysis with three knots was employed to explore potential nonlinear relationships of NHHR with thyroid hormones. The knots were set at the 10th, 50th, and 90th percentiles of the NHHR. If the results demonstrated linear relationships, further linear regression analysis and subgroup analysis were conducted. The linear regression models were utilized to investigate the relationships between NHHR and various thyroid hormones. The coefficient (β), along with its 95% confidence intervals (CIs), were estimated. Meanwhile, logistic regression models were used to investigate the associations between HDL-C, LDL-C, TC, and hyperthyroidism and hypothyroidism. No covariables was adjusted in Model 1, and Model 2 incorporated adjustments for age, gender, and race. Model 3 extended these adjustments to encompass an array of variables, including age, gender, race, marital status, urine iodine concentration, moderate recreational activities, education level, PIR, BMI, smoking status, alcohol consumption, diabetes, hypertension, lipid-lowering medications and thyroid hormone medications. Additionally, a series of subgroup analyses were followed to investigate differences within specific populations. The subgroup factors considered include age (<60, ≥60), gender (male, female), smoking status (never, former, now), moderate recreational activities (inactive, active), stroke (no, yes), diabetes (no, yes), and hypertension (no, yes). Furthermore, we conducted sensitivity analyses to validate the stability of the results. We excluded participants with thyroid cancer, hyperthyroidism, and hypothyroidism to ensure the validity of the relationship between NHHR and thyroid hormones. Finally, the two-sample MR analysis was performed to evaluate the causal links between lipoprotein cholesterol and thyroid function. For this, we utilized GWAS data from the Global Lipids Genetics Consortium for HDL, LDL, and TC as exposures (38), and the UKB-based GWAS for hyperthyroidism and hypothyroidism as binary outcomes [from the Ben Elsworth study]. Genetic data for hyperthyroidism and hypothyroidism can be accessed via the following links: https://gwas.mrcieu.ac.uk/datasets/ukb-b-20289/ and https://gwas.mrcieu.ac.uk/datasets/ukb-b-19732/. We employed the multiplicative random-effects inverse-variance weighted (IVW) method, weighted median method, and MR-Egger regression for analysis. The Cochran’s Q test was used to test the heterogeneity among SNPs and the intercept from MR-Egger was used to assess the potential pleiotropy.
We used multiple imputation based on chained equations (MICE), implemented in the R MI package, to impute missing covariates with five iterations. Statistical significance was determined by a two-tailed P below 0.05. These analyses were conducted using the “TwoSampleMR” package (version 0.5.6) in R (version 4.1.2).
Table 1 shows that all included participants had a mean age of 46.93 ± 0.43 years, with males comprising 48.50% and females 51.50%. Notably, the thyroid hormone levels included an average TT3 of 115.37 ± 0.70 ng/dL, TT4 of 7.91 ± 0.05 µg/dL, FT3 of 3.23 ± 0.01 pg/mL, FT4 of 10.35 ± 0.07 pmol/L, and TSH of 2.14 ± 0.09 mIU/L. Additionally, significant differences were observed in BMI, TT3, and FT3 among participants in the fourth quartile group compared to those in lower NHHR quartiles (P < 0.05). Across different strata of NHHR, no significant differences were observed in age, TT4, TSH, urinary iodine concentration, PIR, marital status, hypertension, or CVD (all P > 0.05). The detailed information on the concentration of both independent and dependent variables can be found in Supplementary Material 2.
Additionally, RCS models were applied to evaluate the nonlinear relationships between NHHR and thyroid hormones, as illustrated in Figure 2. In these RCS models, linear associations were noted between NHHR and FT4 (P for non-linearity trend: 0.569), TSH (P for non-linearity trend: 0.539), FT4/FT3 (P for non-linearity trend: 0.147), as well as TT4/TT3 (P for non-linearity trend: 0.124). Conversely, non-linear associations were distinctly observed with NHHR and FT3 (P for non-linearity trend: 0.012), TT3 (P for non-linearity trend < 0.001), TT4 (P for non-linearity trend = 0.001), and the FT4/TT4 ratio (P- value for non-linearity trend < 0.001).
Figure 2. The nonlinear relationships between NHHR and thyroid hormones was investigated in the National Health and Nutrition Examination Survey (NHANES) conducted between 2007 and 2012. (A) FT3; (B) FT4; (C) TSH; (D) TT3; (E) TT4; (F) FT4/FT3; (G) FT4/FT3 (H) TT4/TT3; and (I) FT4/TT4.
Table 2 displays the comprehensive results of linear regression using three different models evaluating the relationships of NHHR with thyroid function. The fully adjusted model revealed a negative association of NHHR with FT4 (β: -0.18, 95% CI: -0.32, -0.04), FT4/FT3 (β: -0.12, 95% CI: -0.16, -0.07), TT4/TT3 (β: -0.001, 95% CI: -0.001, 0.000), and FT4/TT4 (β: -0.12, 95% CI: -0.16, -0.07).
Supplementary Material 3 presents the results of logistic regression analyses examining the associations of HDL-C, LDL-C, and TC with hyperthyroidism and hypothyroidism across three models. After adjusting for covariates, HDL-C (OR: 0.86, 95% CI: 0.38–1.93), LDL-C (OR: 0.81, 95% CI: 0.64–1.03), and TC (OR: 0.80, 95% CI: 0.65–1.00) showed no statistically significant associations with hyperthyroidism. Similarly, no significant associations were found between HDL-C (OR: 0.96, 95% CI: 0.84–1.10), LDL-C (OR: 0.98, 95% CI: 0.94–1.03), or TC (OR: 0.98, 95% CI: 0.93–1.03) and hypothyroidism.
Consistent with the cross-sectional findings from NHANES data, the MR analysis also revealed no significant associations between HDL-C, LDL-C, TC, and hyperthyroidism or hypothyroidism (P > 0.05). The detailed results are presented in Table 3. Furthermore, no evidence of horizontal pleiotropy was detected.
Subgroup analyses were conducted to explore the impact of specific population characteristics on the relationship between NHHR and thyroid hormones, as detailed in Supplementary Materials 4–9. Analyses by gender indicated negative associations between NHHR and FT4 and FT4/FT3 in both males and females. Notably, in females, a similar negative relationship was observed with the TT4/TT3 ratio. When analyzed by age, NHHR showed a consistent negative association with FT4/FT3 in both subgroups (≥60 and <60 years), with a positive relationship with TSH in the older subgroup and FT4 in the younger subgroup. Analyses by hypertension status revealed a negative association between NHHR and FT4-related ratios in both hypertensive and non-hypertensive groups. Subgroup analyses by diabetes, CVD, and BMI categories consistently showed that NHHR was negatively associated with FT4 and FT4/FT3 ratios. These findings suggest that NHHR may influence thyroid function differently across gender, age, and health conditions, with a general trend of negative associations with FT4 and FT4/FT3 across various subgroups.
To ensure the robustness of our findings, we conducted sensitivity analyses. First, we excluded individuals diagnosed with hyperthyroidism, hypothyroidism, or thyroid cancer. We then applied weighted linear regression to evaluate the association between NHHR and thyroid hormone levels, and the results remained consistent and stable (Supplementary Material 10).
The results found that NHHR is negatively association with FT4, the FT4/FT3, and TT4/FT3. The distinct non-linear relationships between NHHR and FT3, TT3, TT4, and the FT4/TT4 ratio suggest that changes in NHHR may have a more complex impact on the regulation of these thyroid hormones. In particular, the presence of non-linear associations may indicate that at certain levels of NHHR, thyroid hormone regulation could exhibit a threshold effect. That is, once NHHR reaches a critical point, the secretion or conversion rate of thyroid hormones may undergo a dramatic shift, increasing the sensitivity of thyroid function or making it more prone to disruption. This phenomenon could be due to the differential effects of NHHR on various metabolic pathways, further revealing the complexity of the interactions between metabolism and hormone balance. NHHR is an extremely valuable tool in clinical practice, crucial for predicting thyroid hormone levels and function (29, 39). For instance, patients with metabolic syndrome and dyslipidemia are at high risk for thyroid disorders (39). As an effective indicator for assessing metabolic syndrome, NHHR may also play a significant role in the early detection of thyroid diseases (29). For patients who exhibit thyroid dysfunction, regular monitoring of NHHR values can help doctors adjust treatment plans and effectively control hormone level fluctuations (29, 40). This is particularly critical for promoting healthy growth in children (41). Additionally, research indicates that NHHR is an effective tool for assessing the risk of CVD (42). Both hypothyroid and hyperthyroid patients are at increased risk for CVD (7, 9), and patients undergoing cardiac surgery may also develop acquired hypothyroidism (43). Thus, utilizing NHHR can more effectively manage the progression and prognosis of thyroid-related diseases in clinical practice.
This study discovered a positive association between NHHR and both TT3 and FT3 while noting a negative association with FT4. Although no studies directly report these relationships, previous investigations into the interaction between thyroid hormones and lipid abnormalities lend support to our findings. For example, a study in Malaysia demonstrated a notable negative association between FT3 levels and HDL-C (44). Roos and colleagues observed that FT4 showed a negative association with LDL-C and TG yet exhibited a positive association with HDL-C (45). Likewise, studies involving the Spanish population revealed a positive association between FT4 levels and HDL-C, alongside a marked negative association with LDL-C (46). A different research study showed that TT3 has a negative association with LDL-C by increasing the gene expression of LDL-C receptors and enhancing the clearance rate of LDL-C (47). Additionally, elevated levels of HDL-C and reduced levels of LDL-C are associated with a heightened risk of CVD (48). Many researchers have noted that the ratio of FT3/FT4, utilized to evaluate the conversion rate from thyroxine TT4 to triiodothyronine TT3, associates with heightened mortality rates from CVD (49). Consequently, there exists a remarkable link between high HDL-C, low LDL-C, and heightened TT3 levels (48, 49). Moreover, research indicates that heightened levels of NHHR are connected to an amplified risk of type 2 diabetes in patients with hyperthyroidism (42). Leptin, present in the adipose tissue of type 2 diabetes patients (50), can elevate TSH levels, thereby boosting deiodinase activity, facilitating the conversion of FT4 to FT3, and augmenting basal metabolic rate (51). Thus, the escalation in NHHR closely correlates with the decrease in FT4 and the increase in FT3. An animal experiment on obese dogs revealed that adipose tissue within obese animals stimulates the conversion of TT4 to TT3 in order to increase energy expenditure; 42% of obese dogs showed a decrease in FT4 concentration (52, 53). During this process, the transcriptional regulation of genes in adipose tissue contributes to elevated TG levels and decreased HDL-C levels (54). These findings reflect the correlation between NHHR and thyroid hormone levels, aligning with our conclusions. This association emphasizes the importance of NHHR in detecting thyroid hormones.
The underlying mechanisms of this relationship may involve several aspects: First, thyroid hormones increase the flow of bile acids, which promotes the liver’s consumption and synthesis of cholesterol, as well as its uptake from the blood (55, 56). This helps maintain a cholesterol balance in the liver. However, elevated NHHR levels disrupt this balance, leading to increased conversion of stored TT4 into the active form TT3 to restore equilibrium (57). Second, leptin, an essential neuroendocrine regulator, modulates the hypothalamic-pituitary-thyroid axis and governs the thyrotropin-releasing hormone production in the paraventricular and arcuate nuclei (58). An increase in lipid content raises leptin levels, which may lower FT4 levels by influencing serum TSH levels (59). Studies indicate that while FT4 is negatively associated with BMI, TSH is positively associated (60, 61). Moreover, the higher TSH and TT3 levels seen in individuals with obesity might reflect a physiological adaptation aimed at enhancing energy expenditure and managing body weight (57). Third, cholesterol is vital for the production of thyroid hormones (62). High lipid levels are associated with thyroid dysfunction and metabolic disturbances, which can affect the synthesis of thyroid hormones, potentially promoting the synthesis of TT3, although the impact on TT4 synthesis and release may vary (63). Lastly, elevated non-HDL-C levels are often associated with a low-grade inflammatory state, which could affect hormone metabolism (64). Research shows that inflammatory factors can enhance the expression of deiodinases, enzymes that specifically remove iodine atoms from TT4, producing active TT3 and inactive diiodothyronine (65, 66). Thus, inflammation may promote the conversion of TT4 to TT3 while reducing the overall level of TT4.
This research possesses multiple advantages. Firstly, it represents the most extensive population-based analysis conducted thus far to examine the associations of NHHR with various thyroid hormones. Secondly, our analysis utilized a sample that represents the entire U.S. adult demographic to depict the general population accurately. Additionally, we carried out a comprehensive analysis of different variables and made adjustments for covariates to confirm the reliability of our results.
Several limitations should be considered when interpreting the findings of this study. First, the cross-sectional design limits our ability to establish a causal relationship between NHHR and thyroid hormones. Although a potential association between these two factors was suggested in the present study, the simultaneous measurement of lipid biomarkers and thyroid hormone levels prevents us from determining the directionality or temporality of this relationship. Specifically, dyslipidemia, as indicated by an elevated NHHR, may impact thyroid function through mechanisms such as altered deiodination or disruption of thyroid hormone transport. Conversely, thyroid hormones have also been reported to play a crucial role in regulating lipid metabolism. Changes in thyroid function can lead to alterations in lipid profiles, including HDL-C and non-HDL-C cholesterol levels. The possibility of bidirectional interactions introduces the potential for reverse causality, where changes in thyroid hormones could influence NHHR rather than the other way around.
Secondly, despite adjusting for various confounding factors, residual confounding remains an issue that warrants attention. For example, during the NHANES 2007-2012 period, data on inflammatory markers were lacking. However, changes in inflammatory markers may concurrently affect both NHHR and thyroid hormone levels, thereby leading to biased results. Our analysis may not have sufficiently accounted for this potential factor.
Additionally, we conducted MR analysis to further investigate the relationship. Unfortunately, there is no available GWAS specifically focused on NHHR, and the GWAS summary data for TT3, FT3, TT4 and FT4 are also restricted (67). Therefore, we utilized GWAS data from the Global Lipids Genetics Consortium for HDL, LDL, and TC as exposures (32), and the UKB-based GWAS for hyperthyroidism and hypothyroidism as binary outcomes. However, no significant results were observed. In addition to the racial differences (mixed American vs. European descent), the discrepancy between the cross-sectional findings and MR results, we think, may be due to the use of related, but not fully equivalent, phenotypes. To further investigate, we analyzed the associations between HDL-C, LDL-C, TC, and these two binary phenotypes using NHANES data, and again, no significant results were found. As highlighted in our Introduction section, NHHR may provide greater value for disease prediction than traditional lipid markers, emphasizing the importance of further research into NHHR, including dedicated GWAS.
Additionally, thyroid hormone levels were measured using immunoassay methods, which are known to have lower accuracy, especially at low concentrations. Although NHANES employed high-quality laboratory techniques, the lack of LC-MS/MS validation might introduce measurement bias. Future studies incorporating LC-MS/MS validation could provide more accurate and reliable results.
Finally, the NHANES population primarily represents the U.S. population, and the findings may not be fully generalizable to other populations. Cultural, dietary, and genetic differences may influence the association between NHHR and thyroid hormones in other regions, necessitating validation in diverse cohorts.
In summary, this study revealed that among U.S. adults, an increase in NHHR was significantly negatively associated with FT4, FT4/FT3, and TT4/TT3. However, no significant association was found between HDL-C, LDL-C, TC, and the risk of hyperthyroidism or hypothyroidism, highlighting the importance of monitoring NHHR and thyroid hormone levels in clinical practice.
Publicly available datasets were analyzed in this study. This data can be found here: The data in the current study can be found on the website: https://www.cdc.gov/nchs/nhanes/.
Ethics approval and consent to participate The NHANES protocols received approval from the National Center for Health Statistics Ethics Review Board of the U.S. CDC, and all participants provided written informed consent during the survey. The studies were conducted in accordance with the local legislation and institutional requirements. The participants provided their written informed consent to participate in this study.
MT: Conceptualization, Data curation, Formal analysis, Funding acquisition, Investigation, Methodology, Project administration, Resources, Software, Supervision, Validation, Visualization, Writing – original draft, Writing – review & editing. PZ: Conceptualization, Data curation, Formal analysis, Funding acquisition, Investigation, Methodology, Project administration, Resources, Software, Supervision, Validation, Visualization, Writing – original draft, Writing – review & editing. SW: Conceptualization, Data curation, Formal analysis, Funding acquisition, Investigation, Methodology, Project administration, Resources, Software, Supervision, Validation, Visualization, Writing – original draft, Writing – review & editing. SZ: Conceptualization, Data curation, Formal analysis, Funding acquisition, Investigation, Methodology, Project administration, Resources, Software, Supervision, Validation, Visualization, Writing – original draft, Writing – review & editing. MG: Conceptualization, Data curation, Formal analysis, Funding acquisition, Investigation, Methodology, Project administration, Resources, Software, Supervision, Validation, Visualization, Writing – original draft, Writing – review & editing.
The author(s) declare that no financial support was received for the research, authorship, and/or publication of this article.
The authors declare that the research was conducted in the absence of any commercial or financial relationships that could be construed as a potential conflict of interest.
All claims expressed in this article are solely those of the authors and do not necessarily represent those of their affiliated organizations, or those of the publisher, the editors and the reviewers. Any product that may be evaluated in this article, or claim that may be made by its manufacturer, is not guaranteed or endorsed by the publisher.
The Supplementary Material for this article can be found online at: https://www.frontiersin.org/articles/10.3389/fendo.2025.1467254/full#supplementary-material
NHHR, non-high-density lipoprotein cholesterol to high-density lipoprotein cholesterol ratio; TG, Triglyceride; TC, Total cholesterol; HDL-C, high-density lipoprotein cholesterol; LDL-C, low-density lipoprotein cholesterol; NHANES, National Health and Nutrition Examination Survey; NCHS, National Center for Health Statistics; BMI, body mass index; PIR, poverty income ratio; OR, Odds ratio; CI, Confidence interval; TT3, total triiodothyronine; TT4, total thyroxine; FT3, free triiodothyronine; FT4, free thyroxine; TSH, thyroid-stimulating hormone; RCS, restricted cubic spline; ANOVA, one-way analysis of variance; CVD, cardiovascular disease.
1. Pirahanchi Y, Tariq MA, Jialal I. Physiology, Thyroid. St Petersburg: StatPearls Publishing. (2023).
2. Bianco AC, Dumitrescu A, Gereben B, Ribeiro MO, Fonseca TL, Fernandes GW, et al. Paradigms of dynamic control of thyroid hormone signaling. Endocr Rev. (2019) 40:1000–47. doi: 10.1210/er.2018-00275
3. Brent G. Thyroid Hormones (T4, T3). In: Conn PM, Melmed S. eds. Endocrinology: Basic and Clinical Principles. Totowa, NJ: Humana Press (1997) p. 291–306.
4. Tejomani M. A Study of Creatine Kinase Activity, Serum Creatinine and Creatinine Clearance in Hypothyroidism. [Doctoral dissertation]. India: Rajiv Gandhi University of Health Sciences (2013).
5. Bartalena L, Bogazzi F, Brogioni S, Burelli A, Scarcello G, Martino E. Measurement of serum free thyroid hormone concentrations: an essential tool for the diagnosis of thyroid dysfunction. Horm Res. (1996) 45:142–7. doi: 10.1159/000184777
6. Mullur R, Liu YY, Brent GA. Thyroid hormone regulation of metabolism. Physiol Rev. (2014) 94:355–82. doi: 10.1152/physrev.00030.2013
7. Rodondi N, den Elzen WP, Bauer DC, Cappola AR, Razvi S, Walsh JP, et al. Subclinical hypothyroidism and the risk of coronary heart disease and mortality. Jama. (2010) 304:1365–74. doi: 10.1001/jama.2010.1361
8. Pearce EN. Update in lipid alterations in subclinical hypothyroidism. J Clin Endocrinol Metab. (2012) 97:326–33. doi: 10.1210/jc.2011-2532
9. Biondi B. Mechanisms in endocrinology: Heart failure and thyroid dysfunction. Eur J Endocrinol. (2012) 167:609–18. doi: 10.1530/eje-12-0627
10. Kim BJ, Kim TY, Koh JM, Kim HK, Park JY, Lee KU, et al. Relationship between serum free T4 (FT4) levels and metabolic syndrome (MS) and its components in healthy euthyroid subjects. Clin Endocrinol (Oxf). (2009) 70:152–60. doi: 10.1111/j.1365-2265.2008.03304.x
11. Punda A, Škrabić V, Torlak V, Gunjača I, Boraska Perica V, Kolčić I, et al. Thyroid hormone levels are associated with metabolic components: a cross-sectional study. Croat Med J. (2020) 61:230–8. doi: 10.3325/cmj.2020.61.230
12. Wang F, Tan Y, Wang C, Zhang X, Zhao Y, Song X, et al. Thyroid-stimulating hormone levels within the reference range are associated with serum lipid profiles independent of thyroid hormones. J Clin Endocrinol Metab. (2012) 97:2724–31. doi: 10.1210/jc.2012-1133
13. Chowdhury S, Chowdhury JR, Goswami S. The importance of non high density lipoprotein cholesterol in Dyslipidaemia management. J Diabetes Metab. (2015) 6:1–5. doi: 10.4172/2155-6156.1000623
14. Majnarić LT, Bosnić Z, Štefanić M, Wittlinger T. Cross-talk between the cytokine IL-37 and thyroid hormones in modulating chronic inflammation associated with target organ damage in age-related metabolic and vascular conditions. Int J Mol Sci. (2022) 23:6456. doi: 10.3390/ijms23126456
15. Hsu SH, Jang MH, Torng PL, Su TC. Positive association between small dense low-density lipoprotein cholesterol concentration and biomarkers of inflammation, thrombosis, and prediabetes in non-diabetic adults. J Atheroscler Thromb. (2019) 26:624–35. doi: 10.5551/jat.43968
16. Mancini A, Di Segni C, Raimondo S, Olivieri G, Silvestrini A, Meucci E, et al. Thyroid hormones, oxidative stress, and inflammation. Mediators Inflammation. (2016) 2016:6757154. doi: 10.1155/2016/6757154
17. Welsh KJ, Soldin S J. How reliable are free thyroid and total T3 hormone assays? Eur J Endocrinol. (2016) 175:R255–63. doi: 10.1530/EJE-16-0193
18. Masika LS, Zhao Z, Soldin S J. Is measurement of TT3 by immunoassay reliable at low concentrations? A comparison of the Roche Cobas 6000 vs. LC–MSMS Clin Biochem. (2016) 49:846–9. doi: 10.1016/j.clinbiochem.2016.02.004
19. Thienpont LM, Van Uytfanghe K, Beastall G, Faix JD, Ieiri T, Miller WG, et al. Report of the IFCC working group for standardization of thyroid function tests; part 3: total thyroxine and total triiodothyronine. Clin Chem. (2010) 56:921–9. doi: 10.1373/clinchem.2009.140228
20. Thienpont LM, Van Uytfanghe K, Marriott J, Stokes P, Siekmann L, Kessler A, et al. Feasibility study of the use of frozen human sera in split-sample comparison of immunoassays with candidate reference measurement procedures for total thyroxine and total triiodothyronine measurements. Clin Chem. (2005) 51:2303–11. doi: 10.1373/clinchem.2005.058180
21. Thienpont LM, Van Uytfanghe K, Poppe K, Velkeniers B. Determination of free thyroid hormones. Best Pract Res Clin Endocrinol Metab. (2013) 27:689–700. doi: 10.1016/j.beem.2013.05.012
22. Sun Q, Avallone L, Stolze B, Araque KA, Özarda Y, Jonklaas J, et al. Demonstration of reciprocal diurnal variation in human serum T3 and rT3 concentration demonstrated by mass spectrometric analysis and establishment of thyroid hormone reference intervals. Ther Adv Endocrinol Metab. (2020) 11:204201882092268. doi: 10.1177/2042018820922688
23. Babić Leko M, Gunjača I, Pleić N, Zemunik T. Environmental factors affecting thyroid-stimulating hormone and thyroid hormone levels. Int J Mol Sci. (2021) 22:6521. doi: 10.3390/ijms22126521
24. Qing G, Deng W, Zhou Y, Zheng L, Wang Y, Wei B. The association between non-high-density lipoprotein cholesterol to high-density lipoprotein cholesterol ratio (NHHR) and suicidal ideation in adults: a population-based study in the United States. Lipids Health Dis. (2024) 23:17. doi: 10.1186/s12944-024-02012-4
25. Lu S, Kuang M, Yue J, Hu C, Sheng G, Zou Y. Utility of traditional and non-traditional lipid indicators in the diagnosis of nonalcoholic fatty liver disease in a Japanese population. Lipids Health Dis. (2022) 21:95. doi: 10.1186/s12944-022-01712-z
26. Wang M, Wang M, Zhang R, Zhang L, Ding Y, Tang Z, et al. A combined association of serum uric acid, alanine aminotransferase and waist circumference with non-alcoholic fatty liver disease: A community-based study. PeerJ. (2022) 10:e13022. doi: 10.7717/peerj.13022
27. Peeters RP, Visser TJ. Metabolism of Thyroid Hormone. In: Feingold KR, Anawalt B, Blackman MR, Boyce A, Chrousos G, Corpas E, de Herder WW, Dhatariya K, Dungan K, Hofland J, et al. editors. Endotext. South Dartmouth (MA): MDText.com, Inc. (2017).
28. Sinha RA, Bruinstroop E, Singh BK, Yen PM. Nonalcoholic fatty liver disease and hypercholesterolemia: roles of thyroid hormones, metabolites, and agonists. Thyroid. (2019) 29:1173–91. doi: 10.1089/thy.2018.0664
29. Kim SW, Jee JH, Kim HJ, Jin SM, Suh S, Bae JC, et al. Non-HDL-cholesterol/HDL-cholesterol is a better predictor of metabolic syndrome and insulin resistance than apolipoprotein B/apolipoprotein A1. Int J Cardiol. (2013) 168:2678–83. doi: 10.1016/j.ijcard.2013.03.027
30. Brinton EA. Lipid abnormalities in the metabolic syndrome. Curr Diabetes Rep. (2003) 3:65–72. doi: 10.1007/s11892-003-0056-3
31. Ren R, Jiang X, Zhang X, Guan Q, Yu C, Li Y, et al. Association between thyroid hormones and body fat in euthyroid subjects. Clin Endocrinol (Oxf). (2014) 80:585–90. doi: 10.1111/cen.12311
32. Sanderson E, Glymour MM, Holmes MV, Kang H, Morrison J, Munafò MR, et al. Mendelian randomization. Nat Rev Methods Primers. (2022) 2:6. doi: 10.1038/s43586-021-00092-5
33. Centers for Disease Control and Prevention. Ethics Review Board (ERB) Approval. (2024). Available online at: https://www.cdc.gov/nchs/nhanes/irba98.htm (Accessed March 15, 2024).
34. Kim K, Argos M, Persky VW, Freels S, Sargis RM, Turyk ME. Associations of exposure to metal and metal mixtures with thyroid hormones: Results from the NHANES 2007-2012. Environ Res. (2022) 212:113413. doi: 10.1016/j.envres.2022.113413
35. Hollowell JG, Staehling NW, Flanders WD, Hannon WH, Gunter EW, Spencer CA, et al. Serum TSH, T(4), and thyroid antibodies in the United States population (1988 to 1994): National Health and Nutrition Examination Survey (NHANES III). J Clin Endocrinol Metab. (2002) 87:489–99. doi: 10.1210/jcem.87.2.8182
36. Cheng H, Hu Y, Zhao H, Zhou G, Wang G, Ma C, et al. Exploring the association between triglyceride-glucose index and thyroid function. Eur J Med Res. (2023) 28:508. doi: 10.1186/s40001-023-01501-z
37. Liu F, Wang K, Nie J, Feng Q, Li X, Yang Y, et al. Relationship between dietary selenium intake and serum thyroid function measures in U.S. adults: Data from NHANES 2007-2012. Front Nutr. (2022) 9:1002489. doi: 10.3389/fnut.2022.1002489
38. Willer CJ, Schmidt EM, Sengupta S, Peloso GM, Gustafsson S, Kanoni S, et al. Discovery and refinement of loci associated with lipid levels. Nat Genet. (2013) 45:1274. doi: 10.1038/ng.2797
39. Park JH, Choi M, Kim JH, Kim J, Han K, Kim B, et al. Metabolic syndrome and the risk of thyroid cancer: A nationwide population-based cohort study. Thyroid. (2020) 30:1496–504. doi: 10.1089/thy.2019.0699
40. Ogbera AO, Kuku S, Dada O. The metabolic syndrome in thyroid disease: A report from Nigeria. Indian J Endocrinol Metab. (2012) 16:417–22. doi: 10.4103/2230-8210.95688
41. Lazarus J, Brown RS, Daumerie C, Hubalewska-Dydejczyk A, Negro R, Vaidya B. 2014 European thyroid association guidelines for the management of subclinical hypothyroidism in pregnancy and in children. Eur Thyroid J. (2014) 3:76–94. doi: 10.1159/000362597
42. Sheng G, Liu D, Kuang M, Zhong Y, Zhang S, Zou Y. Utility of non-high-density lipoprotein cholesterol to high-density lipoprotein cholesterol ratio in evaluating incident diabetes risk. Diabetes Metab Syndr Obes. (2022) 15:1677–86. doi: 10.2147/dmso.S355980
43. Kong SH, Yoon JW, Kim SY, Oh TJ, Park KH, Choh JH, et al. Subclinical hypothyroidism and coronary revascularization after coronary artery bypass grafting. Am J Cardiol. (2018) 122:1862–70. doi: 10.1016/j.amjcard.2018.08.029
44. Chin KY, Ima-Nirwana S, Mohamed IN, Aminuddin A, Johari MH, Ngah WZ. The relationships between thyroid hormones and thyroid-stimulating hormone with lipid profile in euthyroid men. Int J Med Sci. (2014) 11:349–55. doi: 10.7150/ijms.7104
45. Roos A, Bakker SJL, Links TP, Gans ROB, Wolffenbuttel BHR. Thyroid function is associated with components of the metabolic syndrome in euthyroid subjects. J Clin Endocrinol Metab. (2007) 92:491–6. doi: 10.1210/jc.2006-1718
46. Garduño-Garcia Jde J, Alvirde-Garcia U, López-Carrasco G, Padilla Mendoza ME, Mehta R, Arellano-Campos O, et al. TSH and free thyroxine concentrations are associated with differing metabolic markers in euthyroid subjects. Eur J Endocrinol. (2010) 163:273–8. doi: 10.1530/eje-10-0312
47. Natah TM, Wtwt MAA, Hadi MA, Farhood HF, Zaidan HK, Al-Saadi A. Thyroid metabolic hormones and its correlation with BMI and lipid profile in healthy people. Food Sci Qual Manage. (2013) 18:18–24. doi: 10.1210/jc.2006-1718
48. Mahdy Ali K, Wonnerth A, Huber K, Wojta J. Cardiovascular disease risk reduction by raising HDL cholesterol–current therapies and future opportunities. Br J Pharmacol. (2012) 167:1177–94. doi: 10.1111/j.1476-5381.2012.02081.x
49. Lang X, Li Y, Zhang D, Zhang Y, Wu N, Zhang Y. FT3/FT4 ratio is correlated with all-cause mortality, cardiovascular mortality, and cardiovascular disease risk: NHANES 2007-2012. Front Endocrinol (Lausanne). (2022) 13:964822. doi: 10.3389/fendo.2022.964822
50. Katsiki N, Mikhailidis DP, Banach M. Leptin, cardiovascular diseases and type 2 diabetes mellitus. Acta Pharmacol Sin. (2018) 39:1176–88. doi: 10.1038/aps.2018.40
51. Walczak K, Sieminska L. Obesity and thyroid axis. Int J Environ Res Public Health. (2021) 18:9434. doi: 10.3390/ijerph18189434
52. Hoenig M, Caffall Z, Ferguson DC. Triiodothyronine differentially regulates key metabolic factors in lean and obese cats. Domest Anim Endocrinol. (2008) 34:229–37. doi: 10.1016/j.domaniend.2007.06.002
53. Osto M, Lutz TA. Translational value of animal models of obesity-Focus on dogs and cats. Eur J Pharmacol. (2015) 759:240–52. doi: 10.1016/j.ejphar.2015.03.036
54. Sajuthi SP, Sharma NK, Comeau ME, Chou JW, Bowden DW, Freedman BI, et al. Genetic regulation of adipose tissue transcript expression is involved in modulating serum triglyceride and HDL-cholesterol. Gene. (2017) 632:50–8. doi: 10.1016/j.gene.2017.08.019
55. Yan Y, Niu Z, Sun C, Li P, Shen S, Liu S, et al. Hepatic thyroid hormone signalling modulates glucose homeostasis through the regulation of GLP-1 production via bile acid-mediated FXR antagonism. Nat Commun. (2022) 13:6408. doi: 10.1038/s41467-022-34258-w
56. Ellis ECS. Suppression of bile acid synthesis by thyroid hormone in primary human hepatocytes. World J Gastroenterol. (2006) 12:4640. doi: 10.3748/wjg.v12.i29.4640
57. Biondi B. Subclinical hypothyroidism in patients with obesity and metabolic syndrome: A narrative review. Nutrients. (2023) 16:87. doi: 10.3390/nu16010087
58. Campos AMP, Teixeira PDS, Wasinski F, Klein MO, Bittencourt JC, Metzger M, et al. Differences between rats and mice in the leptin action on the paraventricular nucleus of the hypothalamus: Implications for the regulation of the hypothalamic-pituitary-thyroid axis. J Neuroendocrinol. (2020) 32. doi: 10.1111/jne.12895
59. Marzullo P, Minocci A, Tagliaferri MA, Guzzaloni G, Di Blasio A, De Medici C, et al. Investigations of thyroid hormones and antibodies in obesity: leptin levels are associated with thyroid autoimmunity independent of bioanthropometric, hormonal, and weight-related determinants. J Clin Endocrinol Metab. (2010) 95:3965–72. doi: 10.1210/jc.2009-2798
60. Shon HS, Jung ED, Kim SH, Lee JH. Free T4 is negatively correlated with body mass index in euthyroid women. Korean J Intern Med. (2008) 23:53–7. doi: 10.3904/kjim.2008.23.2.53
61. Zimmermann-Belsing T, Brabant G, Holst JJ, Feldt-Rasmussen U. Circulating leptin and thyroid dysfunction. Eur J Endocrinol. (2003) 149:257–71. doi: 10.1530/eje.0.1490257
62. Bonde Y, Breuer O, Lütjohann D, Sjöberg S, Angelin B, Rudling M. Thyroid hormone reduces PCSK9 and stimulates bile acid synthesis in humans. J Lipid Res. (2014) 55:2408–15. doi: 10.1194/jlr.M051664
63. Duntas LH, Brenta G. A renewed focus on the association between thyroid hormones and lipid metabolism. Front Endocrinol (Lausanne). (2018) 9:511. doi: 10.3389/fendo.2018.00511
64. Barbalho SM, Tofano RJ, de Oliveira MB, Quesada KR, Barion MR, Akuri MC, et al. HDL-C and non-HDL-C levels are associated with anthropometric and biochemical parameters. J Vasc Bras. (2019) 18:e20180109. doi: 10.1590/1677-5449.180109
65. van der Spek AH, Fliers E, Boelen A. Thyroid hormone and deiodination in innate immune cells. Endocrinology. (2021) 162. doi: 10.1210/endocr/bqaa200
66. Köhrle J, Frädrich C. Deiodinases control local cellular and systemic thyroid hormone availability. Free Radic Biol Med. (2022) 193:59–79. doi: 10.1016/j.freeradbiomed.2022.09.024
Keywords: NHHR, serum thyroid function, NHANES, mendelian randomization, clinical practice
Citation: Tan M-Y, Zhang P, Wu S, Zhu S-X and Gao M (2025) Association between non-high-density lipoprotein cholesterol to high-density lipoprotein cholesterol ratio and serum thyroid function measures: Recent Findings from NHANES 2007–2012 and Mendelian randomization. Front. Endocrinol. 16:1467254. doi: 10.3389/fendo.2025.1467254
Received: 19 July 2024; Accepted: 03 January 2025;
Published: 24 January 2025.
Edited by:
Zhiyuan Wu, Harvard University, United StatesReviewed by:
Azadeh Anna Nikouee, Loyola University Chicago, United StatesCopyright © 2025 Tan, Zhang, Wu, Zhu and Gao. This is an open-access article distributed under the terms of the Creative Commons Attribution License (CC BY). The use, distribution or reproduction in other forums is permitted, provided the original author(s) and the copyright owner(s) are credited and that the original publication in this journal is cited, in accordance with accepted academic practice. No use, distribution or reproduction is permitted which does not comply with these terms.
*Correspondence: Ming Gao, MTgyMjQ0MDkzNTVAMTYzLmNvbQ==
Disclaimer: All claims expressed in this article are solely those of the authors and do not necessarily represent those of their affiliated organizations, or those of the publisher, the editors and the reviewers. Any product that may be evaluated in this article or claim that may be made by its manufacturer is not guaranteed or endorsed by the publisher.
Research integrity at Frontiers
Learn more about the work of our research integrity team to safeguard the quality of each article we publish.