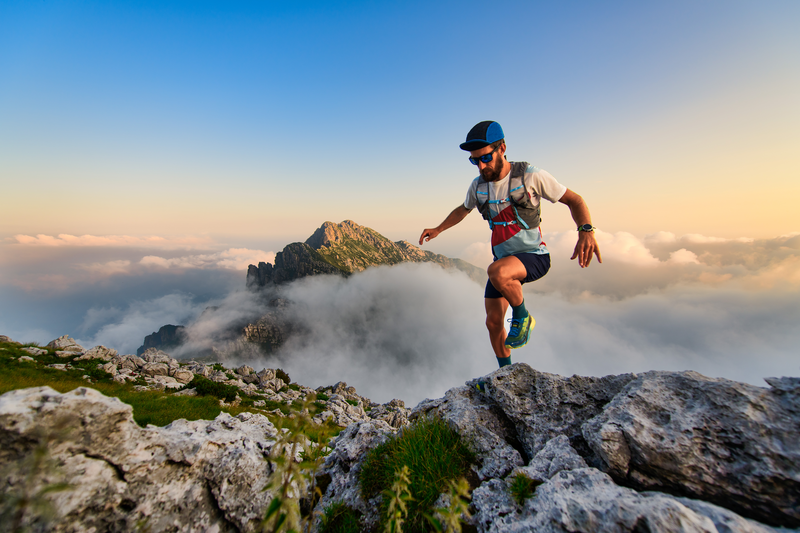
94% of researchers rate our articles as excellent or good
Learn more about the work of our research integrity team to safeguard the quality of each article we publish.
Find out more
ORIGINAL RESEARCH article
Front. Endocrinol. , 23 December 2024
Sec. Thyroid Endocrinology
Volume 15 - 2024 | https://doi.org/10.3389/fendo.2024.1489459
This article is part of the Research Topic Thyroid hormones and diet View all 9 articles
Thyroid function is closely linked to circadian rhythms, but the relationship between the frequency of night eating and thyroid function remains unclear. Our study aimed to investigate the association between night eating frequency and its impact on thyroid function and sensitivity. This study included 6093 participants from the U.S. National Health and Nutrition Examination Survey (2007–2012). Night eating behavior was assessed through 24-hour dietary recall, with night eating frequency calculated on the basis of food intake between 10:00 PM and 4:00 AM. The thyroid hormone indices examined included T3, T4, FT3, FT4, TSH, TGA, Tg, and TPOAb, whereas thyroid hormone sensitivity was assessed via indices such as the FT3/FT4, TSHI, TT4RI, and TFQI. The associations between night eating frequency and thyroid function were analyzed via weighted univariate and multivariate linear regression analyses. Subgroup analyses and interaction test analyses were also employed to test this correlation. Compared with individuals who did not eat at night, those who ate more frequently at night had higher levels of Tg (OR 1.223 [95% CI 1.048, 1.429], p trend=0.015) but lower levels of T3 (OR 0.728 [95% CI 0.611, 0.868], p trend=0.235) and TPOAb (OR 0.728 [95% CI 0.611, 0.868], p trend=0.235). Subgroup analysis indicated that this association between Tg and night eating was stronger in the DM group (Tg: OR 1.49 [95% CI 1.15, 1.93]), p interaction=0.022) and that the association between TPOAb and night eating was stronger in the group without DM (TPOAb: OR 0.9 [95% CI 0.82, 0.97]), p interaction=0.003). Our findings suggest a significant association between night eating frequency and thyroid function. However, no statistically significant differences were found in thyroid sensitivity based on night eating frequency. Despite these findings, the hormone fluctuations observed were within normal clinical ranges. Further rigorously designed studies are needed to establish a causal relationship between night eating frequency and thyroid function.
Thyroglobulin (Tg) is synthesized and stored within the thyroid follicular lumen (1). When the body requires thyroid hormone synthesis, thyroglobulin undergoes iodination and enzymatic digestion, leading to the production of thyroxine (T4) and triiodothyronine (T3). Thyroid peroxidase (TPO) is the key enzyme responsible for this biosynthesis (2). T3 and T4 are essential for human growth, development, and metabolism; they act on multiple target organs and play crucial roles in thermogenesis, protein synthesis, glucose metabolism, and nervous system development (3). The biologically active forms of these hormones are free T3 (FT3) and free T4 (FT4). The secretion of thyroid hormones, which includes thyroid-stimulating hormone (TSH) and thyrotropin-releasing hormone (TRH), is regulated by the hypothalamic–pituitary–thyroid axis. In addition to primary hormones, several calculated indices are commonly used to assess thyroid sensitivity: FT3/FT4 for evaluating peripheral thyroid hormone sensitivity and the thyroid feedback quantile-based index (TFQI), TSH index (TSHI), and thyrotroph T4 resistance index (TT4RI) for assessing central thyroid hormone sensitivity (4–6).
Research on circadian rhythms indicates that in metabolically healthy adults, various metabolic cycles, insulin secretion and sensitivity, and energy expenditure follow a rhythmic pattern (7). Maintaining alignment with circadian rhythms in sleep and diet can reduce the risk of diseases (8). On the other hand, night eating has been shown to impair glucose tolerance (9), trigger mental disorders (10), and accelerate weight gain (11) by disrupting these circadian rhythms. The consumption of foods, such as high-fat, high-sugar, high-salt snacks at night, can further increase total cholesterol and low-density lipoprotein (LDL) cholesterol levels while reducing fat oxidation (12, 13). Therefore, night eating may disrupt the balance of endocrine hormone secretion.
Several studies have demonstrated that disruptions in circadian rhythms can interfere with TSH secretion, thereby affecting thyroid function (14). However, no studies have specifically examined the impact of night eating frequency on thyroid indicators. To address this gap, our study explored the relationships between night eating frequency and both thyroid function and thyroid sensitivity.
The data from 2007–2008, 2009–2010, and 2011–2012 were used for this study (n = 30442). We excluded 20039 individuals whose dietary recall data were unreliable and thyroid hormone indices were missing, 1366 participants younger than 18 years of age, 67 pregnant participants, 1043 participants with heart failure and cancer, 348 participants whose BMI and waist circumference data were missing, and 348 participants with thyroid disease. Overall, our sample consisted of 6093 participants (Figure 1).
The baseline dietary intake data from 2007-2012 were gathered from the initial 24-hour dietary recall interview. This first recall was conducted in person by trained personnel at the NHANES mobile examination centers. Standardized protocols and measurement tools were used to assess the volume and dimensions of the food. During the interviews, the participants were asked to provide details about the quantity and timing of each food and beverage they consumed. The nutrient values were calculated via the Food and Nutrient Database for Dietary Studies (FNDDS).
The main exposure was the frequency of night eating. Night eating was defined as food consumption between 10:00 PM and 4:00 AM, on the basis of the natural light cycle rhythm in this study. The frequency of night eating was categorized into three groups: “No night eating”, “One time”, and “Two times and over”.
In this study, the levels of TT3, FT3, and TT4 were measured via a competitive binding immunoenzymatic assay. Free T4 was determined through a two-step enzyme immunoassay. Sensitive human thyroid-stimulating hormone was detected via a two-site “sandwich” immunoenzyme test. TgAb (TGA) and TPOAb (IU/mL) levels were evaluated via a sequential two-step immunoenzymatic “sandwich” assay, whereas Tg levels (ng/ml) were measured via a simultaneous one-step “sandwich” assay. Detailed instructions for sample collection and processing are discussed in the NHANES Laboratory/Medical Technologists Procedures Manual (LPM). The following is the calculation method for sensitivity to thyroid hormone indices:
FT3/FT4 is achieved by FT3/FT4 ratio=FT3/FT4, TSHI is achieved by TSHI= lnTSH+0.1345*FT4, TT4RI is achieved by TT4RI=FT4*TSH, TFQI is achieved by TFQI = cumulative distribution function (cdfFT4) – (1 – cdfTSH).
The covariates included age (years), sex (male/female), race/ethnicity (Mexican American/non-Hispanic Black/non-Hispanic White/other), education (below high school/high school/above high school), income (poor/not poor), smoking status (never/former/now), drinking status (yes/no), body mass index (<25/25~29/≥30), physical activity (yes/no), hours of sleep (<6h/6~8 h/>8 h), hyperlipidemia (yes/no), hypertension (yes/no), diabetes (DM, yes/no), obesity (yes/no), abdominal obesity (Aobesity,yes/no), UIC (urinary iodine concentration, <100 (deficient), 100-299 (normal), ≥300 µg/L (excess)) (15), glycohemoglobin (%), TG (mmol/L), Ur (mmol/L), Cr (µmol/L), TC (mg/dL), LDL (mg/dl), HDL (mg/dl), neutrophil–lymphocyte ratio (NLR). Obesity participants were those whose BMI ≥ 30 (16). Poor participants were whose ratio of family income to poverty <1. For the definition of drinking status, all participants needed to answer this question,” Had at least 12 alcohol drinks/1 yr?”. Those who answered “No” were labeled as “No drinking,” while those who answered “Yes” were labeled “drinking”. Waist circumference (WC) < 102 cm was considered normal for men, and WC≥ 102 cm was considered Aobesity. WC < 88 cm was considered normal for women, and WC ≥ 88 cm was considered Aobesity (17).
Hypertension was defined as a diagnosis of hypertension, the use of antihypertensive drugs, a systolic blood pressure ≥140 mm Hg, or a diastolic blood pressure ≥90 mm Hg. DM was defined as self-reported, diagnosed diabetes, glycohemoglobin≥ 6.5%, or fasting plasma glucose ≥126 mg/dL. Hyperlipidemia was defined as taking antihyperlipidemic drugs, TG ≥200 mg/dL, TC ≥150 mg/dL, HDL <40 mg/dL for males and <50 mg/dL for females, and LDL ≥130 mg/dL. Heart failure participants were those who were informed that they had heart failure. The cancer participants were those who had been told that they had cancer. Thyroid disease participants were those who were told that they had cancer-related thyroid disease, or thyroid antibody abnormalities were defined as TPOAb≥5.61 IU/ml or TGA≥4.11 IU/ml.
The Dietary Inflammatory Index (DII) is a credible measure of the extent to which dietary factors contribute to an individual’s inflammatory response (18). The DII score of night eating was calculated from the 24-h dietary recall data according to the calculation method described in the R package dietaryindex (19). In this study, we used total alcohol, beta-carotene, caffeine, carbohydrate, cholesterol, energy, fat, fiber, folic acid, vitamins (A, C, E, B1, B12), iron, zinc, magnesium, monounsaturated, polyunsaturated and saturated fatty acids, niacin, ALA (octadecatrienoic acid), EPA (alpha-linolenic acid), protein, selenium and zinc to calculate DII scores.
The complex survey design factors involved in the NHANES, including weights, clustering, and stratification, were all considered as recommended by the NCHS analytical guidelines. Data analysis was performed via R version 4.3.0. Weighted multiple linear regression model were applied to evaluate the associations of the frequency of night eating with thyroid function and sensitivity, including FT3, FT4, TT3, TT4, TSH, TG, TGA, TPOAb, FT3/FT4, TSHI, TT4RI and TFQI (no-night eating group as a reference), in three different model. The results are expressed as OR with 95% confidence intervals (CIs). We adjusted for no variable in Model 1. Model 1 is the unadjusted model. Model 2 was adjusted for baseline sex, age, BMI, race, marital status, family income and education. Model3 were further adjusted for baseline alcohol consumption, smoking status, physical activity, the DII of night eating, urinary iodine concentration, DM status, hypertension status, obesity status, hyperlipidemia status, abdominal obesity status and =UIC=. Owing to the presence of outliers in the data, some data were log-transformed, including T3, T4, TSH, Tg, TGA, TPOAb and TT4RI. We performed subgroup analyses with categorical variables, including sex, age, BMI, race, alcohol consumption, smoking status, physical activity, DII of night eating, UIC, DM, hypertension, obesity, hyperlipidemia, and Aobesity. We performed interaction term tests to check for heterogeneity between subgroups. Weighted multiple linear regression model were applied to investigate the correlations among the frequency of night eating and biochemical variables, including serum urea nitrogen (Ur), serum creatinine (Cr), HDL, LDL, TC, TG, and the neutrophil–lymphocyte ratio (NLR). The results are expressed as OR values with 95% CIs. Three model (model 1, 2, and 3) were adjusted as described above. A two-tailed p value of less than 0.05 was considered significant. The baseline characteristics are expressed as the means ± Standard error of mean (SME) or numbers (percentages).
This study included 6093 participants from NHANES 2007–2012. The baseline characteristics of the participants across night eating frequencies are shown in Table 1. In terms of the frequency of night eating, compared with participants who did not eat at night, those who had more frequent night eating behavior were more likely to be young, be male, Non-Hispanic Black, single, and poor, have higher levels of education, like smoking, sleep less than 6 hours, be less likely to have hyperlipidemia, have higher DII levels of night eating, have higher FT3, have lower TSH, have higher Tg, higher FT3/FT4, lower TSHI, lower TT4FRI, higher Cr, lower glycohemoglobin, lower TC, lower LDL and lower HDL at baseline.
The association of frequency of night eating with thyroid function and thyroid hormone sensitivity were evaluated (Figure 2, Supplementary Table S1). Compared with people who had no night eating, individuals who had frequent night eating were negatively associated with T3 levels in model 1, which was adjusted for nothing (OR 0.901 [95% CI 0.815, 0.995], p trend=0.104]). This association remained significant after further adjusting for sex, age, BMI, race, marital status, education, and family income in model 2 (OR 0.866 [95% CI 0.787, 0.953], p trend=0.064). Model 3, which was further adjusted for smoking behavior, physical activity, drinking behavior, sleep time, hypertension, hyperlipidemia, DM, UIC, and the DII of night eating, remained significant (OR 0.728 [95% CI 0.611, 0.868], p trend=0.235). For TPOAb, the results showed a similar trend to that at T3. In model 1, the OR was 0.901 (95% CI 0.815, 0.995), with a p trend of 0.104. In model 2, the OR was 0.866 (95% CI 0.787, 0.953), with a p trend of 0.064. In model 3, the OR was 0.728 (95% CI 0.611, 0.868), with a p trend of 0.235. Conversely, there was a positive association with Tg levels in model 1 (OR 1.36 [95% CI 1.026, 1.214], p trend=0.047), model 2 (OR 1.154 [95% CI 1.072, 1.242], p trend=0.088) and model3 (OR 1.223 [95% CI 1.048, 1.429], p trend=0.015).
Figure 2. Association between the frequency of night eating and thyroid function and sensitivity. OR, odds ratio, Cl, confidence interval. * p < 0.05, ** p < 0.01, *** p < 0.001; p < 0.05 was considered statistically significant. Model: 1 unadjusted. Model 2: further adjusted for sex, age, BMI, race, marital status, education, and family income. Model 3: further adjusted for smoking behavior, physical activity, drinking behavior, sleep time, hypertension, hyperlipidemia, DM, UIC, and the Dill of night eating.
Compared with people who had no night eating, individuals who had more frequent of night eating were positively associated with FT3 (OR 1.099 [95% CI 1.055, 1.145], p trend=0.011) and negatively associated with TSH (OR 0.935 [95% CI 0.889, 0.984], p trend=0.025) in model 1. Moreover, compared with people who had no night eating, individuals who consumed one time at night were negatively associated with T3 (OR 0.867 [95% CI 0.769, 0.979], p trend=0.235) in model 3 and T4 (OR 0.982 [95% CI 0.966, 0.999], p trend=0.266) in model 2.
Further analyses of thyroid sensitivity revealed that, compared with no night eating, more frequent night eating behavior were positively associated with FT3/FT4 levels in model 1 (OR 1.127 [95% CI 1.037, 1.225], p trend=0.05) and model3 (OR 1.168 [95% CI 1.021, 1.337], p trend=0.516), but this association was not statistically significant in model 2. Conversely, a significantly negative association with the TSHI (OR 0.934 [95% CI 0.881, 0.991], p trend=0.051) and TT4RI (OR 0.928 [95% CI 0.883, 0.976], p trend=0.015) was detected in model 1, but no statistically significant association was detected in the other model.
Furthermore, weighted linear regression analysis was applied to investigate the relationships between the frequency of night eating and other biochemical variables (Figure 3). Statistical differences were found in laboratory tests for blood lipids between those who had higher eating frequency at night and those who did not. Our results indicate that, compared with people who did not eat at night, individuals who consumed at least one night were negatively associated with HDL levels in model 1 (OR 0.812 [95% CI 0.670, 0.985]) and model 2 (OR 0.862 [95% CI 0.749, 0.993]). Moreover, it was also negatively associated with LDL levels in model 1 (OR 0.799 [95% CI 0.650, 0.982]), model 2 (OR 0.806 [95% CI 0.665, 0.977]) and model 3 (OR 0.575 [0.339, 0.977]) and with TC levels in model 1 (OR 0.782 [95% CI 0.649, 0.941]) and model 2 (OR 0.821 [95% CI 0.693, 0.972]) and with Ur levels in model 1(OR 0.874 [95% CI 0.780, 0.978]). The laboratory parameters Cr, glycohemoglobin, NLR, and TG were not significantly different among the model.
Figure 3. The association of frequency of night eating and other laboratory biochemical variables. OR, odds ratio. Cl, confidence interval. * p < 0.05, ** p < 0.01, *** p < 0.001; p < 0.05 was considered statistically significant. Model 1: unadjusted. Model 2. further adjusted for sex, age, BMI, race, marital status, education, and family income. Model 3: further adjusted for smoking behavior, physical activity, drinking behavior, sleep time, hypertension, hyperlipidemia, DM, UIC, and the Dll of night eating.
Subgroup analyses were performed to examine the associations between eating frequency and T3, Tg, TGA, and TPOAb levels in various populations that were categorized according to age, BMI, sex, race, smoking status, alcohol consumption, physical activity, sleep time, hypertension, hyperlipidimia, obesity, Aobesity, DM, and UIC (Figures 4–7). The results revealed that none of the subgroups mentioned above affected the association between the frequency of night eating and T3 (each p interaction > 0.05). For Tg levels, it is noteworthy that a significant positive association between the frequency of night eating and the number of participants with (OR 1.49 [95% CI 1.15, 1.93]), p interaction=0.022) or without (OR 1.13 [95% CI 1.04, 1.22]) DM was found. Moreover, an interaction effect between smoking and one time night eating on Tg levels was also found (OR 0.86 [95% CI 0.79, 0.98]), p interaction=0.025). For TGA levels, a significant positive association with higher eating frequency at night was found in participants aged 65 years and older (OR 1.15 [95% CI 1.03, 1.27]), p interaction=0.03). Conversely, a significant negative association with higher eating frequency at night eating was found in the participants with UIC (OR 0.94 [95% CI 0.9, 0.98]), p interaction=0.008). Furthermore, TPOAb was first found to be negatively associated with one-time (OR 0.88 [95% CI 0.8, 0.96]), p interaction=0.014) or more-time (OR 0.9 [95% CI 0.82, 0.97]), p interaction=0.003) night eating in participants without DM. Conversely, a significant positive association with more night eating was found in the participants with DM (OR 1.34 [95% CI 1.03, 1.72]), p interaction=0.003).
Figure 4. Subgroup analysis of association between night eating frequency and T3 levels. The "No night eating" group was used as a reference.
Figure 5. Subgroup analysis of association between night eating frequency and Tg levels. The "No night eating" group was used as a reference.
Figure 6. Subgroup analysis of association between night eating frequency and TGA levels. The "No night eating" group was used as a reference.
Figure 7. Subgroup analysis of association between night eating frequency and TPOAb levels. The "No night eating" group was used as a reference.
This study expands on existing research regarding night eating frequency and is the first, to our knowledge, to examine its relationship with thyroid function and sensitivity. To investigate this association, we analyzed data from a nationally representative cohort of U.S. adults. To reduce potential confounding factors, we excluded participants who were under 18 years of age, had cancer, had heart failure, were pregnant, or had thyroid disease. Our findings revealed a strong correlation between thyroid function indices and night eating frequency. Specifically, more frequent night eating was associated with higher levels of Tg and lower levels of T3 and TPOAb. Additionally, our study revealed that, compared with not eating at night, more frequent night eating was linked to lower LDL levels. Subgroup analysis, stratified by DM status, indicated that among participants with DM, increased night eating frequency might lead to more pronounced increases in Tg and TPOAb levels. Conversely, in healthy participants, frequent night eating was associated with a decrease in TPOAb levels. Notably, we observed that among participants aged 65 years and older, frequent night eating led to a relative increase in TGA levels, whereas the opposite effect was observed in those with UIC deficiency. Importantly, our results did not reveal a statistically significant difference between thyroid sensitivity and night eating frequency.
Regulating the timing of food intake throughout the day can influence the rhythmicity and regularity of certain aspects of the circadian system and related behaviors (20). Organisms encounter different rhythmic occurrences, such as daily and seasonal cycles. To effectively cope with these cyclic changes in their environment, organisms have developed biological clocks. Almost every cell in the body possesses an intrinsic circadian clock lasting approximately 24 hours, which meticulously governs physiological processes and hormonal rhythms (21). Studies on rodents have shown that consuming a high-fat diet during the rest phase (the light phase for nocturnal rodents) leads to more significant weight gain than does consuming the same diet during the active phase (22). Furthermore, nocturnal light exposure increases body weight by altering the timing of food intake (23). In addition, many previous studies have suggested that disruptions in circadian rhythms may affect the endocrine system, potentially leading to obesity, DM and cardiovascular disease (24–26). The hypothalamus-pituitary-thyroid axis is regulated by the circadian rhythm through the central circadian pacemaker located in the suprachiasmatic nuclei of the anterior hypothalamus (27). Moreover, the literature has reported that the expression profiles of circadian clock genes are abnormal in well-differentiated thyroid cancer but not in benign nodules or healthy thyroids (14). Disruptions in circadian rhythms have also been associated with elevated TSH levels, increasing the risk of thyroid nodules and autoimmune thyroiditis (AIT) (14, 26, 28). AIT, also known as Hashimoto’s thyroiditis (HT) or chronic lymphocytic thyroiditis, is characterized by excessive production of TGA and TPO and infiltration of lymphocytes into thyroid tissue. Notably, a previous study demonstrated a broad disruption of clock gene profiles within thyroid samples from patients with autoimmune thyroiditis (28). Interestingly, numerous studies have reported the effects of Ramadan fasting on thyroid function in Muslim populations. During Ramadan, Muslims are required to abstain from food and drink between dawn and sunset as part of their religious obligations. This practice not only disrupts eating patterns and sleep schedules but also disturbs circadian rhythms. Current research indicates that in healthy adults, TSH levels increase significantly throughout the fasting month, while fT4 and fT3 levels remain unchanged (29). However, in individuals with hypothyroidism, Ramadan fasting worsens thyroid function (30, 31). Shift workers, whose circadian rhythms are significantly disrupted, also exhibit variations in thyroid hormone levels under different dietary influences (32). This suggests a dual impact of nighttime eating and circadian rhythm disturbances on thyroid function. Our study revealed that more frequent night eating resulted in a decrease in T3 and TPOAb levels and an increase in Tg levels, but TSH concentrations were not significantly different from no night eating participants, which suggested a complex interaction between circadian rhythms and thyroid dysfunction.
Importantly, we evaluated the night eating DII. The DII, proposed by J.R. Hébert et al. (33), is a literature-derived dietary tool for measuring individual dietary inflammation. Many studies have shown that higher increased levels of IL-6 and CRP (34–36), which results in increased FT3 and TT4 levels (37, 38) and increased risk of thyroid carcinoma (39). Our results revealed that those who ate two or more times at night had higher DII scores. This may be because multiple eating episodes resulted in a higher inflammation score. Therefore, we speculate that in addition to rhythm disruption, dietary inflammation may also be a contributing factor. Specifically, we hypothesize that when diurnal eating rhythms are disrupted and night eating promotes inflammation, thyroid function may be more severely impaired.
Thyroid hormones are reportedly related to pancreatic β-cell development and influence glucose metabolism through several organs (38). Therefore, thyroid disease and DM are two closely associated disorders. Compared with the general population, type 1 diabetes(T1DM) patients develop thyroid disease at an early age (40). The prevalence of thyroid disease is significantly greater among patients with type 2 diabetes (T2DM) than in the general population (41). In individuals with DM and normal thyroid function, the nocturnal TSH peak has been found to be absent or weak, and the TSH response to TRH is also impaired (42). Although the prevalence of thyroid dysfunction was greater in the T2DM group, TPOAb and TGA were more common in the healthy group (43). In addition, a study reported a greater prevalence of thyroid disease in subjects in the United States with DM than in those without DM, especially in patients who were TPOAb positive. Surprisingly, our results revealed that frequent night eating may be one of the reasons for the increased production of TPOAb in diabetic patients, even if the effects of glucose metabolism disorders are not as significant as those caused by circadian rhythm disruptions.
A higher prevalence of thyroid disorders has been documented in people with DM than in normoglycemic individuals, whereas patients with both endocrinopathies have poorer glycemic control and are more vulnerable to the development of complications. We speculate that the reason for the elevated Tg levels may be that night eating caused an increase in blood glucose levels, prompting the secretion of thyroid hormones to participate in glucose homeostasis, which also positively affected the Tg level. Therefore, owing to the interaction effects, the Tg levels in DM participants increased more than those in participants who did not eat at night.
As we all know, iodine intake significantly affects thyroid function. Chronic exposure to excess iodine intake induces autoimmune thyroiditis, partly because highly iodinated Tg is more immunogenic (44). Conversely, iodine deficiency may decrease the level of iodinated Tg and lead to a decrease in TGA levels. This situation is more pronounced in participants with iodine deficiency, who eat more times at night. Furthermore, our results indicate that the elevation in Tg levels is more pronounced in the elderly population, particularly those who eat more times at night. One study reported that the level of TGA gradually increased with increasing age in females, whereas TGA levels peaked in males aged 50–59 years (42), which is consistent with our results.
Additionally, we focused on laboratory indicators such as renal function, glycated hemoglobin, blood lipids, and the NLR, in addition to thyroid function, with the intention of identifying any associations between these indicators and impaired thyroid function. Thyroid hormones are known to affect energy metabolism. Many patients with metabolic syndrome have subclinical or clinical hypothyroidism and vice versa (45). A study of railway workers revealed that irregular work schedules, which affect their circadian rhythm of sleep, may lead to dyslipidemia (46). Another study indicated that men who slept less than 7 h had a greater probability of dyslipidemia than day workers did (47). One study reported that subjects consumed a specified snack (192.4 ± 18.3 kcal) either during the10:00AMor 23:00PM for 13 days. Compared with the daytime group, the night eating group presented increased LDL levels (12). In addition, the results of a meta-analysis indicated that increased TSH levels were significantly associated with increased TC and LDL levels (48). The metabolically unhealthy no-obese (MUNO) phenotype is significantly associated with hypothyroidism in individuals with thyroid autoimmunity, with a pronounced prevalence in women (49). The above studies revealed the intricate relationships among lipid metabolism, thyroid hormones, and the circadian rhythm. Notably, our results revealed that more frequent night eating was associated with lower levels of LDL, and these findings are almost consistent with those reported in another study (50). However, in the subgroup analyses of obesity, Aobesity, BMI, and hyperlipidemia, no interactions were found between these indicators and T3, Tg, TPOAb, or TGA. The common perception is that circadian rhythm disruptions lead to an increase in LDL, typically regarded as a “bad” lipid parameter, which in turn increases the risk of developing cardiovascular diseases and disrupts thyroid hormone secretion (51). However, our research revealed the opposite findings. These results, not only stem from variations in study methods, subjects, and populations, but also suggest the presence of potential underlying mechanisms that warrant further investigation.
Our study also had some limitations. First, the participants included in the study did not represent the entire population. Second, the frequency of night eating was obtained through dietary recall, the accuracy of which cannot be reliably estimated. Additionally, night eating information is only a single dietary recall and cannot represent long-term habits. Third, unknown and unmeasured confounding factors are likely present, so we cannot make strong causal inferences. Fourth, we could not determine whether participants were taking any medications or supplementation. Given the limitations mentioned above, the present results still need further confirmation by longitudinal prospective large cohort studies with accurate information.
Publicly available datasets were analyzed in this study. This data can be found here: https://wwwn.cdc.gov/nchs/nhanes/Default.aspx.
The studies involving humans were approved by National Center for Health Statistics. The studies were conducted in accordance with the local legislation and institutional requirements. The participants provided their written informed consent to participate in this study. Written informed consent was obtained from the individual(s) for the publication of any potentially identifiable images or data included in this article.
YZ: Conceptualization, Methodology, Software, Writing – original draft. SZ: Investigation, Software, Writing – review & editing. SL: Validation, Visualization, Writing – review & editing. YW: Investigation, Writing – review & editing. HZ: Software, Writing – review & editing. JW: Validation, Writing – review & editing. LW: Writing – review & editing. XW: Project administration, Supervision, Writing – review & editing.
The author(s) declare that no financial support was received for the research, authorship, and/or publication of this article.
The authors declare that the research was conducted in the absence of any commercial or financial relationships that could be construed as a potential conflict of interest.
All claims expressed in this article are solely those of the authors and do not necessarily represent those of their affiliated organizations, or those of the publisher, the editors and the reviewers. Any product that may be evaluated in this article, or claim that may be made by its manufacturer, is not guaranteed or endorsed by the publisher.
The Supplementary Material for this article can be found online at: https://www.frontiersin.org/articles/10.3389/fendo.2024.1489459/full#supplementary-material
1. Di Jeso B, Arvan P. Thyroglobulin from molecular and cellular biology to clinical endocrinology. Endocr Rev. (2016) 37:2–36. doi: 10.1210/er.2015-1090
2. Williams D, Le S, Godlewska M, Hoke D, Buckle A. Thyroid peroxidase as an autoantigen in hashimoto’s disease: structure, function, and antigenicity. Horm Metab Res. (2018) 50:908–21. doi: 10.1055/a-0717-5514
3. Kim B. Thyroid hormone as a determinant of energy expenditure and the basal metabolic rate. Thyroid. (2008) 18:141–4. doi: 10.1089/thy.2007.0266
4. Hoermann R, Midgley JEM, Larisch R, Dietrich JW. The role of functional thyroid capacity in pituitary thyroid feedback regulation. Eur J Clin Invest. (2018) 48:e13003. doi: 10.1111/eci.13003
5. Chen S, Sun X, Zhou G, Jin J, Li Z. Association between sensitivity to thyroid hormone indices and the risk of osteoarthritis: an NHANES study. Eur J Med Res. (2022) 27:114. doi: 10.1186/s40001-022-00749-1
6. Susiarjo M, Xin F, Bansal A, Stefaniak M, Li C, Simmons RA, et al. Bisphenol a exposure disrupts metabolic health across multiple generations in the mouse. Endocrinology. (2015) 156:2049–58. doi: 10.1210/en.2014-2027
7. Andriessen C, Schrauwen P, Hoeks J. The importance of 24-h metabolism in obesity-related metabolic disorders: opportunities for timed interventions. Int J Obes. (2021) 45:479–90. doi: 10.1038/s41366-020-00719-9
8. Lane JM, Qian J, Mignot E, Redline S, Scheer FAJL, Saxena R. Genetics of circadian rhythms and sleep in human health and disease. Nat Rev Genet. (2023) 24:4–20. doi: 10.1038/s41576-022-00519-z
9. Allison KC, Hopkins CM, Ruggieri M, Spaeth AM, Ahima RS, Zhang Z, et al. Prolonged, controlled daytime versus delayed eating impacts weight and metabolism. Curr Biol. (2021) 31:650–657.e3. doi: 10.1016/j.cub.2020.10.092
10. Meyer N, Lok R, Schmidt C, Kyle SD, McClung CA, Cajochen C, et al. The sleep-circadian interface: A window into mental disorders. Proc Natl Acad Sci U.S.A. (2024) 121:e2214756121. doi: 10.1073/pnas.2214756121
11. Wang JB, Patterson RE, Ang A, Emond JA, Shetty N, Arab L. Timing of energy intake during the day is associated with the risk of obesity in adults. J Hum Nutr Diet. (2014) 27:255–62. doi: 10.1111/jhn.12141
12. Hibi M, Masumoto A, Naito Y, Kiuchi K, Yoshimoto Y, Matsumoto M, et al. Nighttime snacking reduces whole body fat oxidation and increases LDL cholesterol in healthy young women. Am J Physiol Regul Integr Comp Physiol. (2013) 304:R94–R101. doi: 10.1152/ajpregu.00115.2012
13. Barrington WE, Beresford SAA. Eating occasions, obesity and related behaviors in working adults: does it matter when you snack? Nutrients. (2019) 11:2320. doi: 10.3390/nu11102320
14. Ikegami K, Refetoff S, Cauter EV, Yoshimura T. Interconnection between circadian clocks and thyroid function. Nat Rev Endocrinol. (2019) 15:590–600. doi: 10.1038/s41574-019-0237-z
15. World Health Organization. Assessment of iodine deficiency disorders and monitoring their elimination: A guide for programme managers, Vol. 1. WHO. (2007).
16. World Health Organization. Obesity - preventing and managing the global epidemic: Report on a WHO consultation. Geneva: World Health Organization (2000). p. 266.
17. World Health Organization. Waist circumference and waist-hip ratio: Report of a WHO expert consultation (2011). Geneva. Available online at: https://iris.who.int/handle/10665/44583 (Accessed December 3, 2024). 8-11 december 2008.
18. Shivappa N, Steck SE, Hurley TG, Hussey JR, Hébert JR. Designing and developing a literature-derived, population-based dietary inflammatory index. Public Health Nutr. (2014) 17:1689–96. doi: 10.1017/S1368980013002115
19. Zhan JJ, Hodge RA, Dunlop AL, Lee MM, Bui L, Liang D, et al. Dietaryindex: A user-friendly and versatile R package for standardizing dietary pattern analysis in epidemiological and clinical studies. Am J Clin Nutr. (2024) 120:1165–74. doi: 10.1016/j.ajcnut.2024.08.021
20. Dashti HS, Scheer FAJL, Saxena R, Garaulet M. Timing of food intake: identifying contributing factors to design effective interventions. Adv Nutr. (2019) 10:606–20. doi: 10.1093/advances/nmy131
21. Mohawk JA, Green CB, Takahashi JS. Central and peripheral circadian clocks in mammals. Annu Rev Neurosci. (2012) 35:445–62. doi: 10.1146/annurev-neuro-060909-153128
22. Arble DM, Bass J, Laposky AD, Vitaterna MH, Turek FW. Circadian timing of food intake contributes to weight gain. Obes (Silver Spring). (2009) 17:2100–2. doi: 10.1038/oby.2009.264
23. Fonken LK, Workman JL, Walton JC, Weil ZM, Morris JS, Haim A, et al. Light at night increases body mass by shifting the time of food intake. Proc Natl Acad Sci U.S.A. (2010) 107:18664–9. doi: 10.1073/pnas.1008734107
24. Wehrens SMT, Christou S, Isherwood C, Middleton B, Gibbs MA, Archer SN, et al. Meal timing regulates the human circadian system. Curr Biol. (2017) 27:1768–1775.e3. doi: 10.1016/j.cub.2017.04.059
25. Morris CJ, Yang JN, Garcia JI, Myers S, Bozzi I, Wang W, et al. Endogenous circadian system and circadian misalignment impact glucose tolerance via separate mechanisms in humans. Proc Natl Acad Sci U.S.A. (2015) 112:E2225–2234. doi: 10.1073/pnas.1418955112
26. Rizza S, Neri A, Capanna A, Grecuccio C, Pietroiusti A, Magrini A, et al. Night shift working is associated with an increased risk of thyroid nodules. J Occup Environ Med. (2020) 62:1–3. doi: 10.1097/JOM.0000000000001711
27. Kalsbeek A, Fliers E. Daily regulation of hormone profiles. In: Kramer A, Merrow M, editors. Circadian Clocks. Springer, Berlin, Heidelberg (2013). p. 185–226. doi: 10.1007/978-3-642-25950-0_8
28. Fu J, Fan Z, He L, Liu Q, Liu H, Li Y, et al. Circadian clock disruption in autoimmune thyroiditis. Eur Thyroid J. (2023) 12:e230035. doi: 10.1530/ETJ-23-0035
29. Sajid KM, Akhtar M, Malik GQ. Ramadan fasting and thyroid hormone profile. J Pak Med Assoc. (1991) 41:213–6.
30. Alkaf B, Siddiqui M, Ali T, Bakir A, Murphy K, Meeran K, et al. Ramadan fasting and changes in thyroid function in hypothyroidism: Identifying patients at risk. Thyroid. (2022) 32:368–75. doi: 10.1089/thy.2021.0512
31. Sheikh A, Mawani M, Mahar SA. Impact of ramadan fasting on thyroid status and quality of life in patients with primary hypothyroidism: A prospective cohort study from karachi, Pakistan. Endocr Pract. (2018) 24:882–8. doi: 10.4158/EP-2018-0038
32. Holmbäck U, Forslund A, Lowden A, Forslund J, Åkerstedt T, Lennernäs M, et al. Endocrine responses to nocturnal eating - possible implications for night work. Eur J Nutr. (2003) 42:75–83. doi: 10.1007/s00394-003-0386-6
33. Cavicchia PP, Steck SE, Hurley TG, Hussey JR, Ma Y, Ockene IS, et al. A new dietary inflammatory index predicts interval changes in serum high-sensitivity C-reactive protein. J Nutr. (2009) 139:2365–72. doi: 10.3945/jn.109.114025
34. Shivappa N, Hébert JR, Rietzschel ER, De Buyzere ML, Langlois M, Debruyne E, et al. Associations between dietary inflammatory index and inflammatory markers in the asklepios study. Br J Nutr. (2015) 113:665–71. doi: 10.1017/S000711451400395X
35. Shivappa N, Wirth MD, Hurley TG, Hébert JR. Association between the dietary inflammatory index (DII) and telomere length and C-reactive protein from the National Health and Nutrition Examination Survey-1999-2002. Mol Nutr Food Res. (2017) 61(4):10. doi: 10.1002/mnfr.201600630
36. Shin D, Lee KW, Brann L, Shivappa N, Hébert JR. Dietary inflammatory index is positively associated with serum high-sensitivity C-reactive protein in a Korean adult population. Nutrition. (2019) 63–64:155–61. doi: 10.1016/j.nut.2018.11.016
37. Wang M, Lu X, Zheng X, Liu J. The relationship between dietary inflammatory index values and thyroid function in the US adult population: An analysis of the NHANES 2007-2012 cohort. Immun Inflammation Dis. (2023) 11:e1016. doi: 10.1002/iid3.1016
38. Liu N, Ma F, Feng Y, Ma X. The association between the dietary inflammatory index and thyroid function in U.S. adult males. Nutrients. (2021) 13:3330. doi: 10.3390/nu13103330
39. Paquet M, Shivappa N, Hébert JR, Baron-Dubourdieu D, Boutron-Ruault M-C, Guénel P, et al. Dietary inflammatory index and differentiated thyroid carcinoma risk: A population-based case-control study in New Caledonia. Am J Epidemiol. (2020) 189:95–107. doi: 10.1093/aje/kwz192
40. Fatourechi A, Ardakani HM, Sayarifard F, Sheikh M. Hypothyroidism among pediatric patients with type 1 diabetes mellitus, from patients’ characteristics to disease severity. Clin Pediatr Endocrinol. (2017) 26:73–80. doi: 10.1297/cpe.26.73
41. Gu Y, Li H, Bao X, Zhang Q, Liu L, Meng G, et al. The relationship between thyroid function and the prevalence of type 2 diabetes mellitus in euthyroid subjects. J Clin Endocrinol Metab. (2016) 102(2):434–42. doi: 10.1210/jc.2016-2965
42. Grigoriadis G, Koufakis T, Kotsa K. Epidemiological, pathophysiological, and clinical considerations on the interplay between thyroid disorders and type 2 diabetes mellitus. Med (Kaunas). (2023) 59:2013. doi: 10.3390/medicina59112013
43. Radaideh A-RM, Nusier MK, Amari FL, Bateiha AE, El-Khateeb MS, Naser AS, et al. Thyroid dysfunction in patients with type 2 diabetes mellitus in Jordan. Saudi Med J. (2004) 25:1046–50. doi: 10.1016/j.revmed.2004.05.001
44. Rayman MP. Multiple nutritional factors and thyroid disease, with particular reference to autoimmune thyroid disease. Proc Nutr Soc. (2019) 78:34–44. doi: 10.1017/S0029665118001192
45. Gutch M, Rungta S, Kumar S, Agarwal A, Bhattacharya A, Razi SM. Thyroid functions and serum lipid profile in metabolic syndrome. BioMed J. (2017) 40:147–53. doi: 10.1016/j.bj.2016.12.006
46. Dong C, Liu H, Yang B, Pan J, Tang L, Zeng H, et al. Circadian rhythm sleep-wake disorders and the risk of dyslipidemia among railway workers in southwest China: A cross-sectional study. Chronobiol Int. (2023) 40:734–43. doi: 10.1080/07420528.2023.2205933
47. Joo JH, Lee DW, Choi D-W, Park E-C. Association between night work and dyslipidemia in South Korean men and women: a cross-sectional study. Lipids Health Dis. (2019) 18:75. doi: 10.1186/s12944-019-1020-9
48. Wang J-J, Zhuang Z-H, Shao C-L, Yu C-Q, Wang W-Y, Zhang K, et al. Assessment of causal association between thyroid function and lipid metabolism: a Mendelian randomization study. Chin Med J (Engl). (2021) 134:1064–9. doi: 10.1097/CM9.0000000000001505
49. Jing M, Shao S, Ma S, Gao L, Wang Q, Zhou M. Exploring the link between obesity and hypothyroidism in autoimmune thyroid diseases: a metabolic perspective. Front Mol Biosci. (2024) 11:1379124. doi: 10.3389/fmolb.2024.1379124
50. Wang P, Tan Q, Zhao Y, Zhao J, Zhang Y, Shi D. Night eating in timing, frequency, and food quality and risks of all-cause, cancer, and diabetes mortality: findings from national health and nutrition examination survey. Nutr Diabetes. (2024) 14:5. doi: 10.1038/s41387-024-00266-6
Keywords: thyroid function, thyroid sensitivity, circadian rhythms, night eating, cross-sectional study 31 32
Citation: Zhang Y, Zhou S, Liu S, Wang Y, Zhou H, Wang J, Wang L and Wang X (2024) Association between night eating frequency and thyroid function and sensitivity: a cross-sectional study from the NHANES database. Front. Endocrinol. 15:1489459. doi: 10.3389/fendo.2024.1489459
Received: 01 September 2024; Accepted: 10 December 2024;
Published: 23 December 2024.
Edited by:
Joao Dts Anselmo, Hospital do Divino Espírito Santo, PortugalReviewed by:
Inês Mendes, Hospital do Divino Espírito Santo, PortugalCopyright © 2024 Zhang, Zhou, Liu, Wang, Zhou, Wang, Wang and Wang. This is an open-access article distributed under the terms of the Creative Commons Attribution License (CC BY). The use, distribution or reproduction in other forums is permitted, provided the original author(s) and the copyright owner(s) are credited and that the original publication in this journal is cited, in accordance with accepted academic practice. No use, distribution or reproduction is permitted which does not comply with these terms.
*Correspondence: Xiaosong Wang, WHNfd2FuZzE2M0AxNjMuY29t
Disclaimer: All claims expressed in this article are solely those of the authors and do not necessarily represent those of their affiliated organizations, or those of the publisher, the editors and the reviewers. Any product that may be evaluated in this article or claim that may be made by its manufacturer is not guaranteed or endorsed by the publisher.
Research integrity at Frontiers
Learn more about the work of our research integrity team to safeguard the quality of each article we publish.