- Department of Clinical Nutrition, Peking Union Medical College Hospital, China Academic Medical Science and Peking Union Medical College, Beijing, China
Context: The beneficial effects of probiotic supplementation on bone health in postmenopausal women require further validation.
Objective: This study systematically reviewed and conducted a meta-analysis of randomized controlled trials (RCTs) to assess the relationship between probiotic supplementation and changes in bone mineral density (BMD) and bone turnover markers (BTMs) among postmenopausal women.
Methods: A systematic search was conducted across four databases to retrieve data on lumbar spine BMD, hip BMD, collagen type 1 cross-linked C-telopeptide (CTX), receptor activator of nuclear factor-κB ligand (RANKL), osteocalcin (OC), osteoprotegerin (OPG), N-terminal propeptide of type 1 procollagen (P1NP), and bone-specific alkaline phosphatase (BALP) in postmenopausal women. Eligible RCTs were quantitatively analyzed using random-effects meta-analyses. Additional analyses, including subgroup, sensitivity, and meta-regression analyses, were performed.
Results: Twelve RCTs involving 1183 postmenopausal women were included. Compared with the control group, postmenopausal women who received probiotic supplementation showed significantly greater BMD in both the lumbar spine (standardized mean difference [SMD] = 0.60, 95% confidence interval [CI] 0.14 to 1.05) and the hip (SMD = 0.74, 95%CI 0.15 to 1.33). Additionally, probiotic supplementation was associated with reduced levels of CTX (SMD = -1.51, 95%CI -1.88 to -0.41) and BALP (SMD = -1.80, 95%CI -2.78 to -0.81). No significant differences were found between the probiotic and control groups in terms of other BTMs. Subgroup analyses revealed that the increase in BMD due to probiotic supplementation was more significant in postmenopausal women with osteopenia than in those with osteoporosis. The meta-analysis results for both lumbar spine and hip BMD remained robust after conducting sensitivity analyses and meta-regressions.
Conclusion: Supplementation with probiotics may increase BMD among postmenopausal women, with stronger evidence in women with osteopenia than osteoporosis. Further RCTs are suggested to confirm and refine these findings.
Systematic review registration: https://www.crd.york.ac.uk/PROSPERO/, identifier CRD42024576764.
Introduction
Osteoporosis is a common bone disease characterized by reduced bone mass and density, leading to an increased risk of fractures (1). Globally, it affects a significant proportion of the population, particularly among older adults. The prevalence of osteoporosis is higher in women than in men, with approximately one in three women over the age of 50 being affected (2). Postmenopausal women are particularly at risk for osteoporosis and fractures due to the natural decline in endogenous estrogen production, which is known to have a protective effect on bone mineral density (BMD). This decline in estrogen leads to a substantial reduction of bone mineral density (generally ranging from 2% to 5% per year) during the late perimenopausal period as well as in the first postmenopausal years (3). Moreover, the reduction in estrogen adversely impacts the bone’s microarchitecture, making the bones more susceptible to fractures.
Despite pharmaceutical interventions being available, adherence rates remain strikingly low, with less than 50% of patients continuing treatment beyond the first year (4, 5). This has been attributed to various factors, including a preference for alternative treatments and concerns over medication side effects (6). There is a clear trend toward seeking low-risk strategies to counteract the effects of osteoporosis, with dietary supplements like calcium and vitamin D gaining popularity. However, their impact on osteoporosis management may be less significant than initially thought (7), prompting a search for additional interventions (8).
Existing evidence has shown that there are remarkable changes in gut microbiota or its metablolites in postmenopausal women, and such changes are notably correlated with postmenopausal osteoporosis (PMO) (9–12). These correlations offer novel insights into the underlying mechanism of PMO and new strategies for treatment that could improve bone health in postmenopausal women.
Probiotics, gaining popularity as dietary interventions, are beneficial live microorganisms that can provide health advantages when consumed in sufficient quantities. There is a growing body of research highlighting the gut microbiota’s substantial influence on bone health through various interconnected mechanisms. This influence may involve the regulation of pro-inflammatory cytokines, which can increase bone resorption, the stimulation of intestine-derived estrogen production, the preservation of intestinal barrier integrity to prevent endotoxin translocation, and the increased production of short-chain fatty acids to inhibit osteoclast differentiation and promote the formation of osteoblastic cells as well as nutrient absorption essential for bone formation and maintenance (13–17). In animal models that mimic postmenopausal osteoporosis, supplementation with probiotics, including both Lactobacillus and Bifidobacterium species, has been demonstrated to significantly enhance BMD and bone volume in ovariectomized subjects (18). However, in human studies, particularly those focused on postmenopausal women, there exists a scarcity of comprehensive meta-analyses. The existing meta-analysis (19) is limited by a small number of randomized controlled trials (RCTs) included—specifically, only five studies (20–24)—and a focus on English-language publications, which restricts the generalizability of the findings.
Recently, additional available trials focusing on postmenopausal women (25–28), plus the prior literature from China (29–31), has expanded the available data, effectively doubling the number of studies considered compared to previous meta-analysis (19). This has prompted a new systematic review and meta-analysis of a broader range of RCTs. Consequently, we embarked on a systematic review and meta-analysis that encompassed a range of RCTs, with the objective to assess the potential skeletal benefits of probiotic interventions specifically in postmenopausal women.
Materials and methods
This review was conducted in accordance with the PRISMA guidelines for reporting systematic reviews (32). The protocol has been registered in PROSPERO (https://www.crd.york.ac.uk/PROSPERO/, identifier: CRD42024576764).
Data sources and searches
We systematically searched four electronic databases—MEDLINE (PubMed), Embase, Web of Science, and China National Knowledge Infrastructure (CNKI)—from inception through 5 August 2024 for published RCTs evaluating the effects of probiotic supplementation (versus control or placebo) on bone mineral density (BMD) and bone turnover markers (BTMs) in postmenopausal women. We used the following search terms: ‘probiotics’, ‘probiotic*’, ‘lactobacillus’, ‘bifidobacterium’, ‘enterococcus’, ‘bone’, ‘bone mineral density’, ‘bone loss’, ‘bone turnover’, ‘osteoporosis’, ‘osteopenia’, ‘osteoporo*’, ‘osteopeni*’, ‘postmenopausal’, ‘post menopause’, and ‘postmenopause’ (see Supplementary Table 1 for details on the search strategy). Reference lists of original trials were manually examined to obtain additional relevant data. The language was restricted to English and Chinese.
Inclusion and exclusion criteria
The details regarding the PICOTS criteria are provided in Supplementary Table 2.
Inclusion criteria are as follows: 1) RCTs focusing on postmenopausal women; 2) the use of probiotic (multiple-strain or single-strain) supplementation as interventions and use of placebo (or control) as a comparison and consideration of the change of BMD and/or BTMs as outcomes, or trials with multiple interventions (e.g., coadministered probiotics and vitamin D or calcium) were eligible if the study groups differed only by the use of probiotics; 3) trials utilized dual-energy X-ray absorptiometry (DXA) for the measurement of BMD in the lumbar spine and hip at baseline and trial’s end. Concurrently, BTMs were identified via blood analysis at the same time points; 4) probiotic supplementation for at least 3 months and 5) original articles are written in English or Chinese. In addition, when results from a study population were reported more than once, the results with the longest follow-up time were utilized.
The following types of studies were excluded: 1) cross-sectional, cohort or case-control studies, reviews or meta-analyses, case reports, and animal or cell experiments; 2) articles only reporting protocols, editorials, comments, letters, conferences or abstracts of meeting presentations and 3) absence of expected data for meta-analysis.
Data extraction
Two reviewers (FW and PJL) extracted independently the following information from each trial: the first author, year of publication, country, main participants’ characteristics (sample size, age, and body mass index), type of probiotics, intervention duration, other treatments, adherence with intervention, adverse effects, main outcomes including BMD in the lumbar spine and hip, collagen type 1 cross-l inked C-telopeptide (CTX), receptor activator of nuclear factor-κ B ligand (RANKL), osteocalcin (OC), osteoprotegerin (OPG), N-terminal propeptide of type 1 procollagen (P1NP), and bone-specific alkaline phosphatase (BALP). Additionally, the descriptions of the evidence of gut coloization for included studies after probiotic supplementation were presented in Supplementary Table 3. In the current study, BMD in the lumbar spine and hip were defined as the primary outcomes, while BTMs were utilized as secondary outcomes.
Risk of bias assessment
Methodological quality was independently assessed by two investigators (W.W. and F.W.) by using the Cochrane Collaboration tool (33). All disagreements were resolved through consultation with a third investigator (P.J.L.). Bias in studies was appraised as low, high, or unclear, based on an evaluation of sequence generation, allocation concealment, participant and staff blinding, outcome assessor blinding, handling of incomplete data, selective outcome reporting, and other potential biases (Supplementary Table 4, Supplementary Figures 1A, B).
Statistical analysis
Statistical analyses for this review were performed using STATA 14.0, and Review Manager 5.3 software. The impact of probiotic supplementation on bone status and BTMs was evaluated by examining the mean relative change from baseline to the conclusion of the intervention, alongside its standard deviation (SD). Direct usage of means and SDs of changes from baseline was prioritized; where not available, data were transformed using established methods (34–37). In cases where trials had multiple intervention arms of the same nature, they were combined into a single arm as per previous methods (36).
The pooled effects of the studies were expressed as standardized mean differences (SMD) with 95% confidence intervals (CIs). Heterogeneity among the studies was assessed using Cochrane’s Q test and quantified with the I2 statistic, with thresholds for low, moderate, and high heterogeneity set at <25%, 25%-50%, and >50%, respectively (38). A random-effects model was employed for calculating pooled effect measures in the presence of any heterogeneity (I2 > 0%). Sensitivity analyses were conducted to test the robustness of the results, involving the sequential omission of individual studies and further removal of studies with a high risk of bias to observe changes in heterogeneity. For the primary outcomes of lumbar spine and hip BMD, subgroup analyses were conducted to explore potential interactions based on the types of probiotic supplements, dosage of probiotic supplementation, intervention duration, geographical region, and participants’ baseline BMD indicators, including the presence or absence of osteoporosis (defined by a T-score of ≤ -2.5). Additionally, meta-regression analysis was utilized to determine if heterogeneity could be attributed to specific baseline characteristics such as age and BMI. Publication bias was assessed in meta-analyses with at least 10 studies using funnel plots and the Egger test (39). The trim-and-fill method was applied to identify and adjust for potential publication bias in the effect estimates.
Results
Search results
Our initial search strategies across four databases yielded a total of 346 papers. After the removal of 112 duplicate records and screening the titles or abstracts of the remaining 234 records, we excluded 199 records that were obviously not relevant. The full text of the 35 eligible reports was read, which helped us to identify one additional article. Finally, we identified twelve RCTs that involved 1183 postmenopausal women (635 in the intervention group and 548 in the control group) as eligible for meta-analyses (20–31). A detailed overview of the selection process is provided in the PRISMA flow diagram in Figure 1.
Characteristics of the included studies
The general characteristics of the included trials published between 2017 and 2024 were presented in Table 1. These studies were parallel-design, single-center trials conducted in the China (25, 29–31), Denmark (20), Iran (21), Japan (22), Poland (26), Sweden (23, 24, 27), and Thailand (28). Of these studies, four trials (22, 24, 26, 27) employed a single-strain probiotic as their intervention approach, in contrast to the rest that used multiple-strain probiotic formulations (20, 21, 23, 25, 28–31). The duration (3~24 months) of intervention varied across the studies, with three studies implementing interventions for a period of three months (25, 26, 28), five studies with interventions spanning six months (21, 22, 29–31), and four studies conducting interventions for a timeframe exceeding one year (20, 23, 24, 27). Additionally, eleven trials reported the results of lumbar spine BMD (20–27, 29–31), and ten studies described the results of hip BMD (20–27, 29, 30). According to the dosage of probiotics, seven studies were defined as the high-dose group (≥ 1 x 10^9 CFU/d) (20–27) and three as the low-dose group (< 1 x10^8 CFU/d) (29–31).
Main outcomes
Effects of probiotic supplementation on BMD
The meta-analysis investigating the effects of probiotics on BMD in the lumbar spine involved eleven trials (20–27, 29–31), while that concerning the hip BMD comprised ten trials (20–27, 29, 30). The pooled results using the random effects model showed that probiotic supplementation had a positive effect on both lumbar spine BMD (SMD=0.60, 95%CI [0.14, 1.05], P=0.01; I2 = 92.1%; Figure 2A) and hip BMD (SMD=0.74, 95%CI [0.15, 1.33], P=0.013; I2 = 94.5%; Figure 2B) when compared with control.
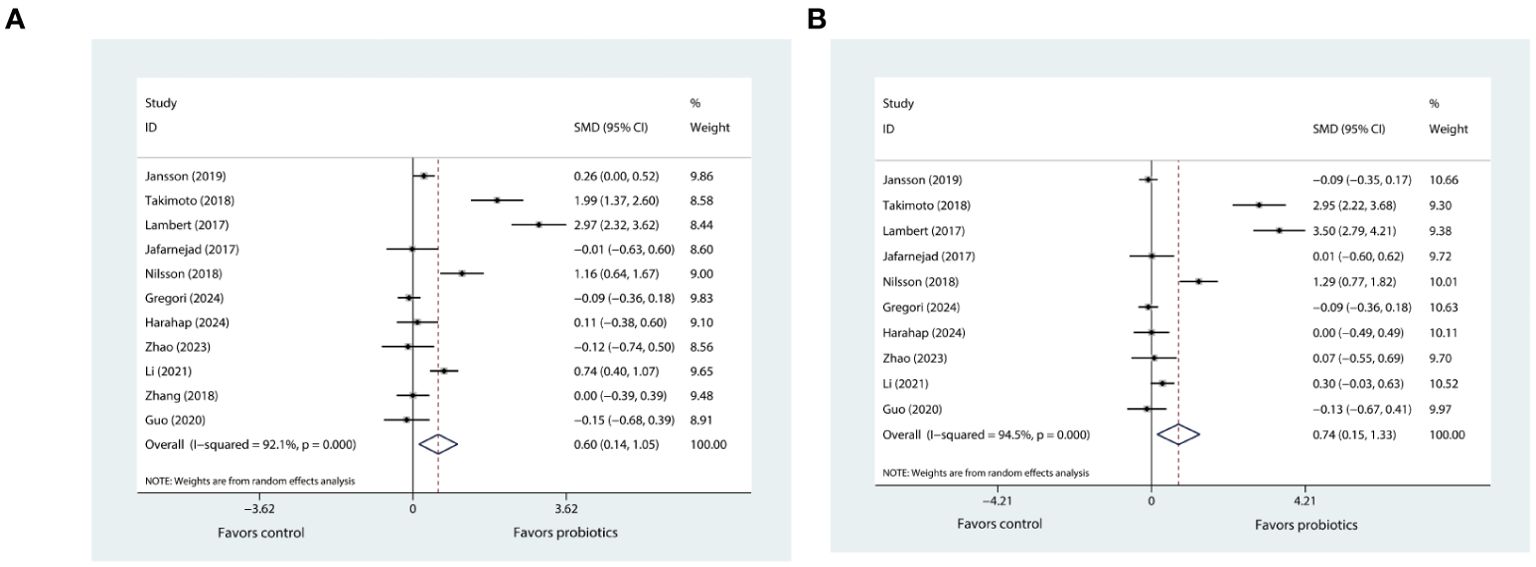
Figure 2. (A) Forest plot depicting the overall effect of probiotics on lumbar spine BMD. (B) Forest plot depicting the overall effect of probiotics on hip BMD.
Heterogeneity, subgroup, sensitivity analyses and meta-regressions
For the primary outcomes included in the meta-analysis, there was obvious heterogeneity (I2 > 50%). Subsequently, subgroup, sensitivity analyses and meta-regressions were performed to try to identify potential sources of heterogeneity.
The sensitivity analysis for lumbar spine BMD showed that the I² statistic did not fall below 50% after excluding any single trial from the eleven, and the hip BMD analysis also resulted in a similar finding. In addition, the I² statistic for both lumbar spine and hip BMD continued to exceed 50% even after the removal of trials that were reported in the Chinese language. Despite this, the meta-analysis results for both lumbar spine and hip BMD were robust.
Subgroup analyses revealed that probiotic supplementation, regardless of whether it involves single- or multiple-strain formulations, resulted in significant increases in both lumbar spine and hip BMD in trials that specifically included postmenopausal women with osteopenia. This enhancement in BMD was notably more pronounced in comparison to trials involving women who have osteoporosis (characterized by a T-score of more than or equal to -2.5) (Tables 2, 3). Furthermore, our results indicated that high-dose supplementation of probiotics could improve both lumbar spine and hip BMD more effectively than low-dose supplementation (Tables 2, 3). In addition, our findings showed that extended probiotic supplementation (at least one year) indicated greater benefits for enhancing lumbar spine BMD (SMD=1.03, 95%CI [0.06, 2.00], P=0.037; I2 = 96.4%) compared to supplementation regimens that last for six months or less (Table 2). Meta-regressions did not reveal any significant correlation between the effects of probiotics on BMD and the age and BMI of postmenopausal women (Supplementary Figures 2A, B).
Effects of probiotic supplementation on bone turnover markers and sensitivity analyses
CTX, BALP, and P1NP
According to data pooled from nine eligible trials (20, 21, 25–31), when compared with control, probiotic supplementation significantly reduced CTX levels (SMD= -1.51, 95%CI [-1.88, -0.41], P= 0.002; Figure 3A). The degree of heterogeneity was high (I2 = 95.2%).
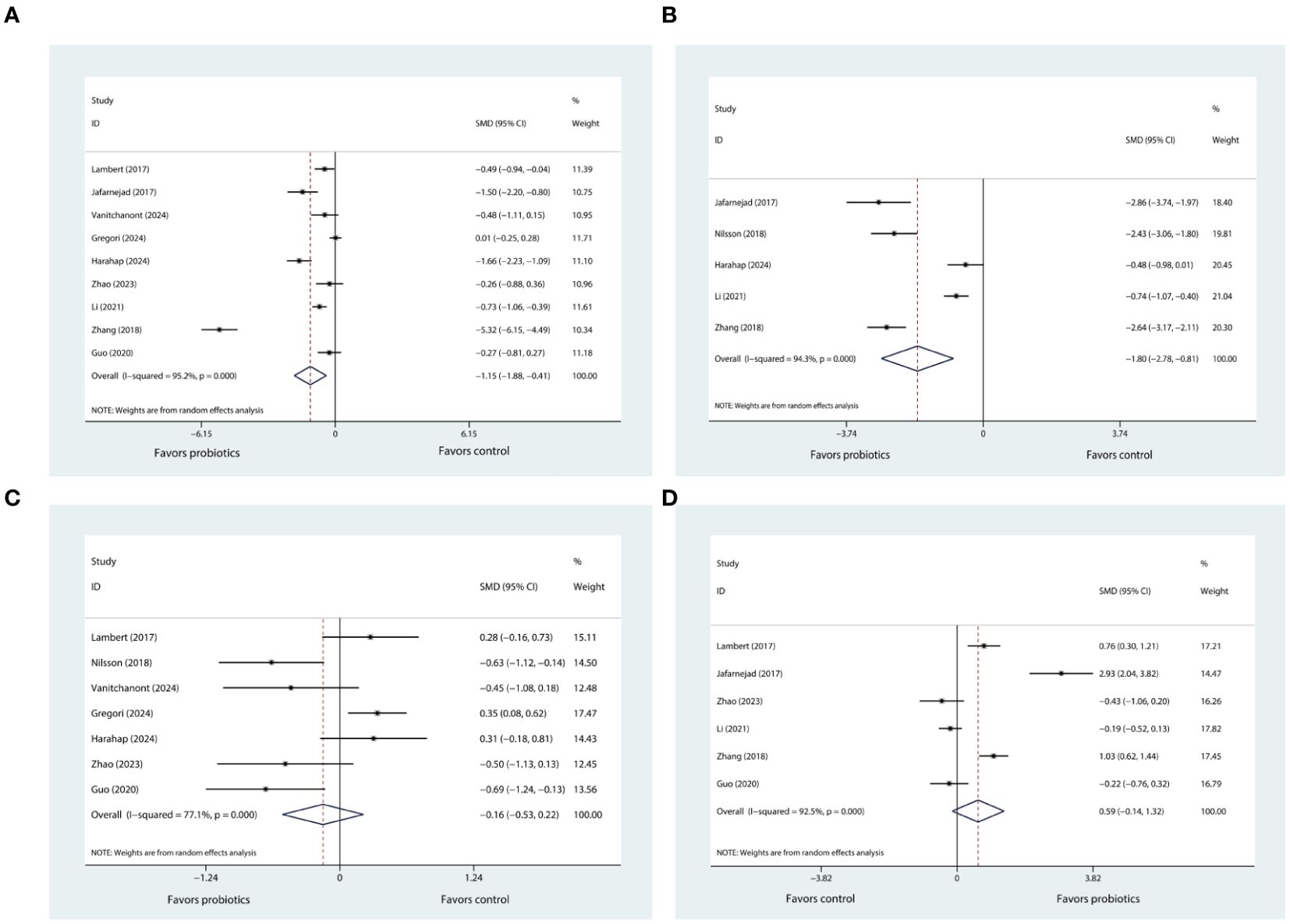
Figure 3. (A) Forest plot depicting the overall effect of probiotics on CTX. (B) Forest plot depicting the overall effect of probiotics on BALP. (C) Forest plot depicting the overall effect of probiotics on P1NP. (D) Forest plot depicting the overall effect of probiotics on OC.
There were five eligible trials reporting the results of BALP (21, 24, 26, 30, 31). Upon consolidating the data from these studies, it was determined that the supplementation with probiotics was associated with a significant reduction in BALP levels (SMD= -1.80, 95%CI [-2.78, -0.81], P < 0.001; I2 = 94.3%; Figure 3B) when compared to the control group.
The sensitivity analysis, in which we sequentially removed each of the nine trials from the meta-analysis, showed that the I² statistic did not decrease below 50%. Despite this persistent heterogeneity, the overall meta-analysis results for CTX-1maintained their robustness. The similar findings were observed in the sensitivity analysis of BALP.
Based on the pooled data from seven trials (20, 24–29), our results indicated that probiotic supplementation did not exert any significant influence on P1NP levels (SMD= 0.59, 95%CI [-0.14, 1.32], P= 0.112; I2 = 92.5%; Figure 3C) in comparison to control group. After conducting sensitivity analysis, it was observed that the results of meta-analysis for P1NP remained stable and did not show any significant alteration. Also, the heterogeneity across the studies, as indicated by the I² statistic, remained high, exceeding 50%.
OC, OPG and RANKL
Six trials (20, 21, 25, 29–31) reported changes in OC levels before and after the intervention. Pooled analysis showed that probiotic supplementation did not exert any significant influence on OC levels (SMD= -0.16, 95%CI [-0.53, 0.22], P= 0.416; I2 = 77.1%; Figure 3D) when compared to control. Through further sensitivity analysis, we did not observe any change in the meta-analysis results or heterogeneity. Only two trials reported the results pertaining to OPG (20, 21) (Supplementary Figure 3A) and RANKL (20, 21) (Supplementary Figure 3B), respectively. The pooled results indicated that use of probiotic supplementation had no significant effect on these two markers.
Publication bias
Potential publication bias was detected using funnel plots and the Egger test. The funnel plots of lumbar spine BMD (Supplementary Figure 4A) and hip BMD (Supplementary Figure 4B) were displayed in the Supplementary Materials. Funnel plots were not created for the other markers as there were fewer than ten included trials in their meta-analyses (39). The Egger test results revealed publication bias for the effects of probiotic supplementation on hip BMD (t=2.42, P=0.042; Supplementary Figure 5B), while the results regarding lumbar spine BMD did not show publication bias (t=1.52, p=0.162; Supplementary Figure 5A). We used the trim-and-fill method to detect and adjust for publication bias regarding the results of hip BMD, but the updated overall effect estimate did not show significant changes (z=2.945, P=0.003; Supplementary Figure 5C). Consequently, more studies with large number are further needed.
Discussion
Main findings
This meta-analysis of twelve RCTs involving 1183 postmenopausal women sheds new light on the influence of probiotic supplementation on bone health in this demographic. The analysis revealed that supplementation with probiotics, especially at a dose of at least 1 x 10^9 CFU per day, positively impacted bone health, with stronger evidence in women with osteopenia than osteoporosis, as indicated by improvements in lumbar spine and hip BMD. Additionally, probiotic supplementation correlated with reduced levels of CTX and BALP, pointing to a potential anti-osteoporotic effect of probiotics. Despite significant heterogeneity across the included studies, the findings are supported by objective primary and secondary outcome measures and a robust random-effects analysis model. The credibility of the results is further supported by the stability of the meta-analysis outcomes following sensitivity analysis.
Postmenopausal women are disproportionately affected by osteoporosis due to estrogen deficiency (40, 41). The current understanding of osteoporosis is not yet sufficient to develop pharmaceuticals capable of completely preventing or stopping the disease’s progression (42). However, research has shown a link between the gut microbiota and bone mass reduction, as well as osteoporosis prevalence (13–16, 43, 44). The gut microbiota is believed to influence bone metabolism by affecting the balance between osteoclast and osteoblast activity, thereby impacting the host’s metabolism and immune system (9, 10, 45) (The diagram illustrating the potential impact of gut microbiota regulation on promoting bone health is presented in Figure 4). This has led to the exploration of modulating the intestinal microbiome, such as through probiotic supplementation, as a treatment for osteoporosis or osteopenia in postmenopausal women (20–31). However, the effects of probiotics on the bone health in postmenopausal women are still inconsistent.
To our knowledge, only one previous meta-analysis has specifically examined the effects of probiotic supplementation on bone health in postmenopausal women (19). That study found positive effects on BMD in the spine but no observed benefits in the hip region. Another meta-analysis (46) evaluated the impact of probiotics on bone health among postmenopausal women and other individuals suffering from senile or diabetic osteoporosis, and it reported similar findings regarding BMD in the lumbar spine and hip among postmenopausal women as Yu et al.’s study (19). The discrepancy in the effects of probiotics on BMD in different areas among postmenopausal women may be due to the small sample size of studies included in these two meta-analyses (19, 46). Our current meta-analysis reaffirms the previous findings on lumbar spine BMD and further expands on these results by demonstrating positive effects of probiotics on hip BMD as well.
Additionally, subgroup analyses were conducted and revealed that the improvement in BMD was more pronounced in postmenopausal women with mild bone loss (osteopenia) (20–24, 26–31), when treated with probiotics, as opposed to those with osteoporosis (25, 29, 30). This may be due to the limited inclusion of studies in the osteoporosis group. Therefore, further research is needed on probiotic intervention for postmenopausal osteoporosis. When the included trials were divided into subgroups according to intervention duration (≤6 months or > 12 months), we found extended durations of probiotic intervention (> 12 months) (20, 23, 24, 27), in contrast to brief periods of treatment, demonstrated superior enhancement in lumbar spine BMD (P=0.037), suggesting that longer-term probiotic supplementation may yield more benefits in bone health. Additionally, the improvement in hip BMD did not reach statistical significance (SMD= 1.10, 95%CI [-0.02, 2.22], P=0.054). We also conducted subgroup analysis based on study region (Europe and Asia), and found that probiotics appear to be more effective in enhancing lumbar spine BMD in postmenopausal women from Europe compared to those from Asian backgrounds (21, 22, 25, 28–31). This observation could be attributed to potential differences in gut microbiome composition, genetic factors, lifestyle, or dietary habits between these populations, although further research is needed to clarify these regional disparities in response to probiotic treatment. Of note, our subgroup analysis also suggested that there was no significant difference in the impact on BMD between single- and multiple-strain probiotics.
CTX and P1NP are both widely recognized bone turnover markers (BTMs) in clinical use, with CTX indicating bone resorption and P1NP indicating bone formation (47). Our study’s findings that probiotic supplementation can lead to a reduction in CTX levels are in line with two previous meta-analyses (19, 46), neither of which reported the effects of probiotics on P1NP due to the limited data. By synthesizing our findings on both P1NP and CTX, it is clear that the mechanism through which probiotic supplementation may help in preventing bone loss is likely due to its effect on inhibiting bone resorption by suppressing osteoclast activity (22). BALP, a marker traditionally associated with osteoblast proliferation, is recognized as a bone formation indicator (45). However, there is a growing body of evidence suggesting that BALP should be reclassified as a marker of bone turnover rather than solely bone formation (48, 49). In our study, it was found that levels of BALP could be decreased by supplementation with probiotics. This is consistent with the findings of other studies, which have reported a decrease in BALP following the consumption of symbiotic products (50) or specific probiotic strains such as Lactobacillus reuteri (51). These collective findings suggest that the classification of BALP as a bone formation marker may need to be reconsidered in light of its association with the broader process of bone turnover. However, the meta-analysis by Yu et al. showed no significant changes in BALP (19). This is most likely due to the limited number of studies included.
In addition our study also examined other bone turnover markers, including OC, OPG, and RANKL. Our findings are in line with previous meta-analyses (19, 46), which did not find any significant differences in the levels of these markers between postmenopausal women who received probiotics and those in the control group. However, it is important to highlight a preclinical study that demonstrated the supplementation of heat-killed Lacticaseibacillus paracasei GMNL-653 could lead to a specific reduction in the mRNA level of RANKL in ovariectomized mice; Whole-genome sequencing and comparative genomics analysis indicated that genes associated with the transport and metabolism of carbohydrates, as well as the biogenesis of cell walls, membranes, and envelopes, might play a role in the anti-osteoporotic effects of GMNL-653 (52). This observation prompts the hypothesis that the impact of different probiotic strains on bone turnover markers might vary. To substantiate these preliminary findings, further research is essential to explore the potential variability in effects among different strains of probiotics on BTMs.
Limitations and strengths
Our study has several limitations. Firstly, there is high heterogeneity between the included studies, although random-effects model was used to calculate the results, complemented by suitable subgroup analyses and meta-regressions. Secondly, we had to calculate SMD rather than the weighted mean difference due to the inconsistent units describing BMD change among the included studies. Third, in several trials (20–22, 25, 27, 28, 30, 31), co-interventions were used as treatment methods, rather than probiotics alone. This might lead to potential bias; however, after we conducted sensitivity analysis, the robustness of the meta-analysis findings was maintained. Lastly, based on the current trials included, it was not possible for us to discern which probiotic strains specifically improve BMD or BTMs.
On the other hand, this study also has several strengths. Firstly, most of the included trials were of high quality. Secondly, we performed adequate subgroup analyses; sensitivity analysis and meta-regressions were also conducted to minimize heterogeneity between the included studies. Thirdly, compared with previous meta-analyses and reviews, this meta-analysis includes a larger number of trials and a wider population.
Conclusion
Our systematic review and meta-analysis found that probiotic supplementation in postmenopausal women was associated with improved BMD in the lumbar spine and hip, with stronger evidence in women with osteopenia than osteoporosis. This suggests that probiotic supplementation may serve as an alternative approach to decelerate bone mass deterioration in postmenopausal women with osteopenia. In addition, administration of probiotics could decrease levels of CTX and BALP. In the future, more research is needed to validate these findings, and specific strains beneficial for bone health in postmenopausal women need to be further explored.
Data availability statement
The original contributions presented in the study are included in the article/Supplementary Material, further inquiries can be directed to the corresponding author.
Author contributions
FW: Conceptualization, Funding acquisition, Writing – review & editing, Methodology. WW: Formal analysis, Investigation, Methodology, Software, Validation, Writing – review & editing. PL: Conceptualization, Funding acquisition, Writing – original draft, Writing – review & editing.
Funding
The author(s) declare financial support was received for the research, authorship, and/or publication of this article. This research was supported by National High Level Hospital Clinical Research Funding (no. 2022-PUMCH-B-055). The funding source had no role in the study design or in the execution, data collection or analysis, or manuscript writing.
Acknowledgments
The authors thank Guannan Luan for assisting with the literature search process.
Conflict of interest
The authors declare that the research was conducted in the absence of any commercial or financial relationships that could be construed as a potential conflict of interest.
Publisher’s note
All claims expressed in this article are solely those of the authors and do not necessarily represent those of their affiliated organizations, or those of the publisher, the editors and the reviewers. Any product that may be evaluated in this article, or claim that may be made by its manufacturer, is not guaranteed or endorsed by the publisher.
Supplementary material
The Supplementary Material for this article can be found online at: https://www.frontiersin.org/articles/10.3389/fendo.2024.1487998/full#supplementary-material
Abbreviations
BALP, bone-specific alkaline phosphatase; BMD, bone mineral density; BTM, bone turnover marker; CTX, collagen type 1 cross-l inked C-telopeptide; OC, osteocalcin; OPG, osteoprotegerin; P1NP, N-terminal propeptide of type 1 procollagen; PMO, postmenopausal osteoporosis; RANKL, receptor activator of nuclear factor-κB ligand.
References
1. Cummings SR, Melton LJ. Epidemiology and outcomes of osteoporotic fractures. Lancet. (2002) 359:1761–7. doi: 10.1016/S0140-6736(02)08657-9
2. Kanis JA, Burlet N, Cooper C, Delmas PD, Reginster JY, Borgstrom F, et al. European guidance for the diagnosis and management of osteoporosis in postmenopausal women. Osteoporos Int. (2008) 19:399–428. doi: 10.1007/s00198-008-0560-z
3. Finkelstein JS, Brockwell SE, Mehta V, Greendale GA, Sowers MR, Ettinger B, et al. Bone mineral density changes during the menopause transition in a multiethnic cohort of women. J Clin Endocrinol Metab. (2008) 93:861–8. doi: 10.1210/jc.2007-1876
4. Modi A, Siris ES, Tang J, Sen S. Cost and consequences of noncompliance with osteoporosis treatment among women initiating therapy. Curr Med Res Opin. (2015) 31:757–65. doi: 10.1185/03007995.2015.1016605
5. Fatoye F, Smith P, Gebrye T, Yeowell G. Real-world persistence and adherence with oral bisphosphonates for osteoporosis: a systematic review. BMJ Open. (2019) 9:e027049. doi: 10.1136/bmjopen-2018-027049
6. Hopkins RB, Burke N, Von Keyserlingk C, Leslie WD, Morin SN, Adachi JD, et al. The current economic burden of illness of osteoporosis in Canada. Osteoporos Int. (2016) 27:3023–32. doi: 10.1007/s00198-016-3631-6
7. Bolland MJ, Grey A, Reid IR. Should we prescribe calcium or vitamin D supplements to treat or prevent osteoporosis? Climacteric. (2015) 18 Suppl 2:22–31. doi: 10.3109/13697137.2015.1098266
8. Stoecker WV, Carson A, Nguyen VH, Willis AB, Cole JG, Rader RK. Addressing the crisis in the treatment of osteoporosis: better paths forward. J Bone Miner Res. (2017) 32:1386–7. doi: 10.1002/jbmr.v32.6
9. Yang X, Chang T, Yuan Q, Wei W, Wang P, Song X, et al. Changes in the composition of gut and vaginal microbiota in patients with postmenopausal osteoporosis. Front Immunol. (2022) 13:930244. doi: 10.3389/fimmu.2022.930244
10. He J, Xu S, Zhang B, Xiao C, Chen Z, Si F, et al. Gut microbiota and metabolite alterations associated with reduced bone mineral density or bone metabolic indexes in postmenopausal osteoporosis. Aging (Albany NY). (2020) 12:8583–604. doi: 10.18632/aging.103168
11. Wang H, Liu J, Wu Z, Zhao Y, Cao M, Shi B, et al. Gut microbiota signatures and fecal metabolites in postmenopausal women with osteoporosis. Gut Pathog. (2023) 15:33. doi: 10.1186/s13099-023-00553-0
12. Wang Z, Chen K, Wu C, Chen J, Pan H, Liu Y, et al. An emerging role of Prevotella histicola on estrogen deficiency-induced bone loss through the gut microbiota-bone axis in postmenopausal women and in ovariectomized mice. Am J Clin Nutr. (2021) 114:1304–13. doi: 10.1093/ajcn/nqab194
13. Tu Y, Yang R, Xu X, Zhou X. The microbiota-gut-bone axis and bone health. J Leukoc Biol. (2021) 110:525–37. doi: 10.1002/JLB.3MR0321-755R
14. Zaiss MM, Jones RM, Schett G, Pacifici R. The gut-bone axis: how bacterial metabolites bridge the distance. J Clin Invest. (2019) 129:3018–28. doi: 10.1172/JCI128521
15. He Y, Chen Y. The potential mechanism of the microbiota-gut-bone axis in osteoporosis: a review. Osteoporos Int. (2022) 33:2495–506. doi: 10.1007/s00198-022-06557-x
16. Chen YC, Greenbaum J, Shen H, Deng HW. Association between gut microbiota and bone health: potential mechanisms and prospective. J Clin Endocrinol Metab. (2017) 102:3635–46. doi: 10.1210/jc.2017-00513
17. Billington EO, Mahajan A, Benham JL, Raman M. Effects of probiotics on bone mineral density and bone turnover: A systematic review. Crit Rev Food Sci Nutr. (2023) 63:4141–52. doi: 10.1080/10408398.2021.1998760
18. Bose S, Sharan K. Effect of probiotics on postmenopausal bone health: a preclinical meta-analysis. Br J Nutr. (2024) 131:567–80. doi: 10.1017/S0007114523002362
19. Yu J, Cao G, Yuan S, Luo C, Yu J, Cai M. Probiotic supplements and bone health in postmenopausal women: a meta-analysis of randomised controlled trials. BMJ Open. (2021) 11:e041393. doi: 10.1136/bmjopen-2020-041393
20. Lambert MNT, Thybo CB, Lykkeboe S, Rasmussen LM, Frette X, Christensen LP, et al. Combined bioavailable isoflavones and probiotics improve bone status and estrogen metabolism in postmenopausal osteopenic women: a randomized controlled trial. Am J Clin Nutr. (2017) 106:909–20. doi: 10.3945/ajcn.117.153353
21. Jafarnejad S, Djafarian K, Fazeli MR, Yekaninejad MS, Rostamian A, Keshavarz SA. Effects of a multispecies probiotic supplement on bone health in osteopenic postmenopausal women: A randomized, double-blind, controlled trial. J Am Coll Nutr. (2017) 36:497–506. doi: 10.1080/07315724.2017.1318724
22. Takimoto T, Hatanaka M, Hoshino T, Takara T, Tanaka K, Shimizu A, et al. Effect of Bacillus subtilis C-3102 on bone mineral density in healthy postmenopausal Japanese women: a randomized, placebo-controlled, double-blind clinical trial. Biosci Microbiota Food Health. (2018) 37:87–96. doi: 10.12938/bmfh.18-006
23. Jansson PA, Curiac D, Lazou Ahrén I, Hansson F, Martinsson Niskanen T, Sjögren K, et al. Probiotic treatment using a mix of three Lactobacillus strains for lumbar spine bone loss in postmenopausal women: a randomised, double-blind, placebo-controlled, multicentre trial. Lancet Rheumatol. (2019) 1:e154–62. doi: 10.1016/S2665-9913(19)30068-2
24. Nilsson AG, Sundh D, Bäckhed F, Lorentzon M. Lactobacillus reuteri reduces bone loss in older women with low bone mineral density: a randomized, placebo-controlled, double-blind, clinical trial. J Intern Med. (2018) 284:307–17. doi: 10.1111/joim.2018.284.issue-3
25. Zhao F, Guo Z, Kwok LY, Zhao Z, Wang K, Li Y, et al. Bifidobacterium lactis Probio-M8 improves bone metabolism in patients with postmenopausal osteoporosis, possibly by modulating the gut microbiota. Eur J Nutr. (2023) 62:965–76. doi: 10.1007/s00394-022-03042-3
26. Harahap IA, Moszak M, Czlapka-Matyasik M, Skrypnik K, Bogdański P, Suliburska J. Effects of daily probiotic supplementation with Lactobacillus acidophilus on calcium status, bone metabolism biomarkers, and bone mineral density in postmenopausal women: a controlled and randomized clinical study. Front Nutr. (2024) 11:1401920. doi: 10.3389/fnut.2024.1401920
27. Gregori G, Pivodic A, Magnusson P, Johansson L, Hjertonsson U, Brättemark E, et al. Limosilactobacillus reuteri 6475 and prevention of early postmenopausal bone loss: A randomized clinical trial. JAMA Netw Open. (2024) 7:e2415455. doi: 10.1001/jamanetworkopen.2024.15455
28. Vanitchanont M, Vallibhakara SA, Sophonsritsuk A, Vallibhakara O. Effects of multispecies probiotic supplementation on serum bone turnover markers in postmenopausal women with osteopenia: A randomized, double-Blind, placebo-controlled Trial. Nutrients. (2024) 16:461. doi: 10.3390/nu16030461
29. Guo ZG. Clinical study on the effect of probiotic lactic acid bacteria on osteoporosis in postmenopausal women. Hohhot, China: Inner Mongolia Medical University (2020).
30. Li F, Gong JC, Wang QL, Chen Q, Lu Y. Effects of probiotics on bone metabolism in postmenopausal patients with osteoporosis. Chin J Gerontol. (2021) 41:2356–9. doi: 10.3969/j. issn.1005-9202.2021.11.036
31. Zhang JF, Zhong JP, Nie DR, Lin ZP, Gao S. Effect of probiotics on the bone status of postmenopausal women with osteopenia. Chin J Microecol. (2018) 30:4. doi: 10.13381/j.cnki.cjm.201812017
32. Liberati A, Altman DG, Tetzlaff J, Mulrow C, Gøtzsche PC, Ioannidis JP, et al. The PRISMA statement for reporting systematic reviews and meta-analyses of studies that evaluate healthcare interventions: explanation and elaboration. BMJ. (2009) 339:b2700. doi: 10.1136/bmj.b2700
33. Higgins JPT, Thomas J, Chandler J, Cumpston M, Li T, Page MJ, et al. Cochrane handbook for systematic reviews of interventions, 6th Edition. Chichester (UK): John Wiley & Sons, 2019. (2019). Available at: http://www.training.cochrane.org/handbook.
34. Luo D, Wan X, Liu J, Tong T. Optimally estimating the sample mean from the sample size, median, mid-range, and/or mid-quartile range. Stat Methods Med Res. (2018) 27:1785–805. doi: 10.1177/0962280216669183
35. Hozo SP, Djulbegovic B, Hozo I. Estimating the mean and variance from the median, range, and the size of a sample. BMC Med Res Methodol. (2005) 5:13. doi: 10.1186/1471-2288-5-13
36. Liu PJ, Guo J, Zhang Y, Wang F, Yu K. Effects of oral nutritional supplements on the nutritional status and inflammatory markers in patients on maintenance dialysis: a systematic review and meta-analysis of randomized clinical trials. Clin Kidney J. (2023) 16:2271–88. doi: 10.1093/ckj/sfad130
37. Wan X, Wang W, Liu J, Tong T. Estimating the sample mean and standard deviation from the sample size, median, range and/or interquartile range. BMC Med Res Methodol. (2014) 14:135. doi: 10.1186/1471-2288-14-135
38. Higgins JP, Thompson SG, Deeks JJ, Altman DG. Measuring inconsistency in meta-analyses. BMJ. (2003) 327:557–60. doi: 10.1136/bmj.327.7414.557
39. Sterne JA, Sutton AJ, Ioannidis JP, Terrin N, Jones DR, Lau J, et al. Recommendations for examining and interpreting funnel plot asymmetry in meta-analyses of randomised controlled trials. BMJ. (2011) 343:d4002. doi: 10.1136/bmj.d4002
40. Eastell R, O’Neill TW, Hofbauer LC, Langdahl B, Reid IR, Gold DT, et al. Postmenopausal osteoporosis. Nat Rev Dis Primers. (2016) 2:16069. doi: 10.1038/nrdp.2016.69
41. Yu F, Xia W. The epidemiology of osteoporosis, associated fragility fractures, and management gap in China. Arch Osteoporos. (2019) 14:32. doi: 10.1007/s11657-018-0549-y
42. Reid IR, Billington EO. Drug therapy for osteoporosis in older adults. Lancet. (2022) 399:1080–92. doi: 10.1016/S0140-6736(21)02646-5
43. D’Amelio P, Sassi F. Gut microbiota, immune system, and bone. Calcif Tissue Int. (2018) 102:415–25. doi: 10.1007/s00223-017-0331-y
44. Xu Q, Li D, Chen J, Yang J, Yan J, Xia Y, et al. Crosstalk between the gut microbiota and postmenopausal osteoporosis: Mechanisms and applications. Int Immunopharmacol. (2022) 110:108998. doi: 10.1016/j.intimp.2022.108998
45. Yan L, Wang X, Yu T, Qi Z, Li H, Nan H, et al. Characteristics of the gut microbiota and serum metabolites in postmenopausal women with reduced bone mineral density. Front Cell Infect Microbiol. (2024) 14:1367325. doi: 10.3389/fcimb.2024.1367325
46. Zeng L, Yu G, Yang K, Hao W, Chen H. The improving effect and safety of probiotic supplements on patients with osteoporosis and osteopenia: A systematic review and meta-analysis of 10 randomized controlled trials. Evid Based Complement Alternat Med. (2021) 2021:9924410. doi: 10.1155/2021/9924410
47. Wu CH, Chang YF, Chen CH, Lewiecki EM, Wüster C, Reid I, et al. Consensus statement on the use of bone turnover markers for short-term monitoring of osteoporosis treatment in the asia-pacific region. J Clin Densitom. (2021) 24:3–13. doi: 10.1016/j.jocd.2019.03.004
48. Azinge EC, Bolarin DM. Osteocalcin and bone-specific alkaline phosphatase in sickle cell haemoglobinopathies. Niger J Physiol Sci. (2006) 21:21–5. doi: 10.4314/njps.v21i1-2.53934
49. Hooshmand S, Chai SC, Saadat RL, Payton ME, Brummel-Smith K, Arjmandi BH. Comparative effects of dried plum and dried apple on bone in postmenopausal women. Br J Nutr. (2011) 106:923–30. doi: 10.1017/S000711451100119X
50. Scholz-Ahrens KE, Ade P, Marten B, Weber P, Timm W, Açil Y, et al. Prebiotics, probiotics, and synbiotics affect mineral absorption, bone mineral content, and bone structure. J Nutr. (2007) 137:838S–46S. doi: 10.1093/jn/137.3.838S
51. Britton RA, Irwin R, Quach D, Schaefer L, Zhang J, Lee T, et al. Probiotic L. reuteri treatment prevents bone loss in a menopausal ovariectomized mouse model. J Cell Physiol. (2014) 229:1822–30. doi: 10.1002/jcp.24636
Keywords: probiotic, postmenopausal women, osteoporosis, osteopenia, bone, bone turnover marker
Citation: Wang F, Wei W and Liu PJ (2024) Effects of probiotic supplementation on bone health in postmenopausal women: a systematic review and meta-analysis. Front. Endocrinol. 15:1487998. doi: 10.3389/fendo.2024.1487998
Received: 29 August 2024; Accepted: 17 October 2024;
Published: 01 November 2024.
Edited by:
Mor-Li Hartman, The Forsyth Institute, United StatesReviewed by:
Poonam Chanderlal Sharma, University of Mississippi Medical Center, United StatesKatherine Brooke-Wavell, Loughborough University, United Kingdom
Copyright © 2024 Wang, Wei and Liu. This is an open-access article distributed under the terms of the Creative Commons Attribution License (CC BY). The use, distribution or reproduction in other forums is permitted, provided the original author(s) and the copyright owner(s) are credited and that the original publication in this journal is cited, in accordance with accepted academic practice. No use, distribution or reproduction is permitted which does not comply with these terms.
*Correspondence: Peng Ju Liu, bHBqamlhQDEyNi5jb20=
†ORCID: Peng Ju Liu, orcid.org/0000-0002-4769-4252