- 1Institute of Health and Biological Science, Federal University of Mato Grosso, Barra do Garças, Brazil
- 2Araguaia Multi-User Research Center, Federal University of Mato Grosso, Barra do Garças, Brazil
- 3Academic Unit of Health Sciences, Federal University of Jataí, Jataí, Brazil
- 4Institute of Health Science, Federal University of Goiás, Goiania, Brazil
- 5Red Iberoamericana de Alteraciones Vasculares Asociadas a Transtornos del Embarazo (RIVATREM), Chillán, Chile
Introduction: Excess weight during pregnancy is a condition that can affect both mother and fetus, through the maternal-fetal interface, which is constituted by the placenta and umbilical cord. The umbilical vein is responsible for transporting oxygen and nutrients to the fetus, and its proper functioning depends on the integrity of its structure. The remodeling of the umbilical vein represents one of the causes of inadequate transport of nutrients to the fetus, being potentially harmful. This study aims to evaluate whether maternal overweight alters the structural characteristics of the umbilical vein.
Methods: Umbilical cords were collected from eutrophic and overweight pregnant women and were processed according to histological routine. We analyzed morphometry parameters, collagen and elastin fibers deposition, glycosaminoglycan level, and cell proliferation.
Results: Veins from overweight pregnant women were found to have greater total area, wall area, wall thickness, and diameter. There was higher collagen labeling in the perivascular region of the overweight group and a higher amount of type III collagen in the vascular smooth muscle. The proliferation of muscle and perivascular cells was higher in overweight pregnant women. A positive, although weak, correlation was observed between BMI and vessel thickness and with type III collagen deposition in vascular smooth muscle.
Discussion: With this study, we show that being overweight can structurally alter the umbilical vein, causing vascular remodeling of the vessel, through hypertrophy and hyperplasia.
1 Introduction
Pregestational maternal obesity is associated with unfavorable maternal and fetal outcomes, also favoring the developmental programming of obesity, diabetes, and cardiovascular diseases in early and later life (1, 2). Given the high incidence of obesity affecting pregnancies worldwide, it is imperative to understand the mechanisms involving the deleterious intrauterine environment upon obesity.
The umbilical cord (UC) connects the growing fetus to the placenta, supporting an environment required for a successful pregnancy (3), while it is an easily accessible structure reflecting changes in the intrauterine environment. In this regard, the UC has become an interesting target to investigate, considering its accessibility, almost exclusive fetal derivation, and the influence of maternal and fetal circulation on this component.
The UC circulation is composed of two arteries and a unique vein, all of them surrounded by a mucoid substance known as Wharton’s Jelly (WJ). The umbilical vein carries oxygenated blood and other maternal nutrients to the fetus, while the two umbilical arteries carry low-oxygen blood and fetal metabolites to the placenta (4).
Structurally, the UC vessels display peculiar characteristics, differentiating them from most vessels in the body, since in these vessels, the tunica adventitia is replaced by perivascular cells located around the vessel, known as the perivascular Wharton’s Jelly (PVWJ). This vascular layer has a high density of components including the mesenchymal cells and extracellular matrix components (ECM), standing out type I, III, and V collagen (5), proteoglycans, glycosaminoglycans (GAGs) and elastic fibers (6). This vascular arrangement builds up a strong structure, intending to protect these vessels from compression due to fetal movements and to guarantee the essential flow of substances (7).
Several physiological or pathological conditions may elicit alterations in the vascular architecture, leading to a process known as vascular remodeling. Interestingly, obesity favors vascular remodeling in several vessels (8). The overall mechanisms involved in vascular remodeling include proliferation and differentiation of vascular smooth muscle cells (VSMCs) degradation, disruption of elastic fibers, and ECM component deposition (9, 10).
The present work was designed to evaluate whether pregestational maternal overweight modifies the umbilical vein structure, eliciting its vascular remodeling.
2 Materials and methods
2.1 Participants
This is a case-control study. Pregnant women were recruited during prenatal visits at UNIMED Hospital from Jataí, Brazil. This study was approved by the Ethical Committee of the Federal University of Jataí and all participants provided written consent. Maternal body composition was assessed between 4–10 weeks of pregnancy. Eligibility criteria included age ≧̸18 years, live newborn delivery; newborn with no fetal malformations on ultrasound and/or postnatal exam, and gestational age ≧̸ 37 weeks. Exclusion criteria included smoking, pre-eclampsia, diabetes, hypertension, artificial reproductive techniques, HIV, syphilis, infections with possible vertical transmission, and deliveries occurring outside the study hospital. The participants were grouped by their pregestational body mass index (BMI) into two groups: control (n=19), where BMI was between 18.5 and 24.9 kg/m²; and overweight (n=16), where BMI was greater than 25.0 kg/m².
2.2 Sample collection and processing
The UC samples were collected immediately at delivery, washed in phosphate buffered saline (PBS) and fixed in 4% paraformaldehyde for 24 hours. For histological analysis, the tissues were dehydrated, clarified and embedded in paraffin. Sections with a thickness of 5 μm were adhered to glass slides, deparaffinized, rehydrated and stained for morphometric measurements with hematoxylin-eosin; picrosirius for collagen deposition; resorcin-fuchsin for elastic fibers; alcian blue for glycosaminoglycans precipitation.
The collection of segments near the placental region was standardized due to the direction of fetal blood flow. The images were recorded using a light microscope with a digital camera (Nikon Eclipse E-200, Tokyo, Japan) and software (TCapture). The slides stained with picrosirius were also submitted to polarized light microscopy (Zeiss Axioskop 2). All the images were evaluated using the ImageJ software (National Institutes of Health, Bethesda, Maryland, USA).
2.3 Morphometric and histochemical analysis
Vascular measurements were defined as: total vein area (TVA), lumen area (LA), vein wall area (VWA), vein wall thickness (VWT), vein diameter (VD) and wall/lumen ratio. Three images of each vessel were obtained, and the average between them was used as the final value for that sample (40x magnification). For TVA and LA measurements, the contour of the inner and outer vessel wall was evaluated. The subtraction of these measurements (TVA - LA) resulted in the VWA. For VWT, the distance between the outer and inner border of the vessel’s muscular layer was measured in four different regions in the same vessel. The wall/lumen ratio was calculated by a simple division of wall area (mm2) and lumen area (mm2) for each sample. For the VD, two perpendicular measurements of each vein were used. Histochemical analysis of collagen, elastic fibers and glycosaminoglycans was performed by an average of ten images taken of perivascular region and smooth muscle layer (400x magnification). The tool “threshold” was used to set a limit of stain and standardize the protocol. The collagen birefringence analysis was conducted to evaluate type I and type III collagen fibers, according to the hue scale of the image, with values set from 0 to 40 for type I (yellowish red) and 40 to 120 for type III (greenish yellow) (11).
2.4 KI-67 immunohistochemistry
Deparaffinized slides were subjected to antigen retrieval with sodium citrate buffer pH 6.0 (#ab64236, Abcam, USA) at a high temperature (95°C) to expose the epitopes. Next, blocking steps were performed with human serum (1:50 in PBS/BSA 1%) and CAS-Block™ (#008120, ThermoFisher, USA). Incubation with a specific KI-67 primary antibody (#ab15580, Abcam, USA, 1:300) was performed at 4°C overnight. Anti-Rabbit IgG biotinylated secondary antibody (#ab6012, Abcam, USA, 1:250) was used. ImmunoCruz® ABC Kit (#sc-516216, Santa Cruz Biotechnology, USA) was used for signal amplification and background reduction, according to the manufacturer’s instructions. Slides were revealed using the ImmPACT™ NovaRED™ Peroxidase (HRP) Substrate kit (Vector Labs, USA). The ImageJ plugin “Cell Counter” was used to count the number of Ki67 positive cells in 10 image fields in the same region, for each sample. Images were deconvoluted using the tool “Color Deconvolution” with “Colour_1” and “Colour_2” used for cell counting and staining intensity, respectively. Then, it was measured the intensity of the staining using the tool “Threshold”, according to the instructions from the “Semi-quantitative determination of protein expression using immunohistochemistry staining and analysis (Crowe and Yue, 2019). The intensity value detected was divided by the number of cells (stained or not) from its respective field, the average was calculated for each sample and presented as percentage (12).
2.5 Data analysis
Statistical analysis was performed using GraphPad Prism 8 (Graph Pad Software Inc). Clinical characteristics and histological analysis results were described for the entire sample and represented by mean ± SEM. The distribution of the variables was tested using the Shapiro-Wilk test. Correlation analysis was performed between the variables using Pearson’s linear coefficient. Differences between groups were compared using the Student t-test (parametric data) or Mann-Whitney test (non-parametric data) and values were considered statistically significant when p < 0.05.
3 Results
The current study comprises 35 pregnant women, who attended the inclusion and exclusion criteria, divided into control (n=19) and overweight group (n=16). The mean age among mothers and gestational age were similar between groups. BMI was reduced in control compared to the overweight group (p<0.0001). The newborn’s weight and the maternal weight gain during the pregnancy were similar between groups (Table 1).
3.1 Morphometric measurements of umbilical vein
Morphometric measurements are shown in Table 2 and the umbilical veins from groups are represented in Figure 1. Umbilical vein morphometric analysis revealed a significant increase in total area, vein wall area, diameter and thickness in the overweight group, compared to the control. The total lumen area, the wall/lumen ratio and diameter did not show significant differences when the two groups were compared between the groups.
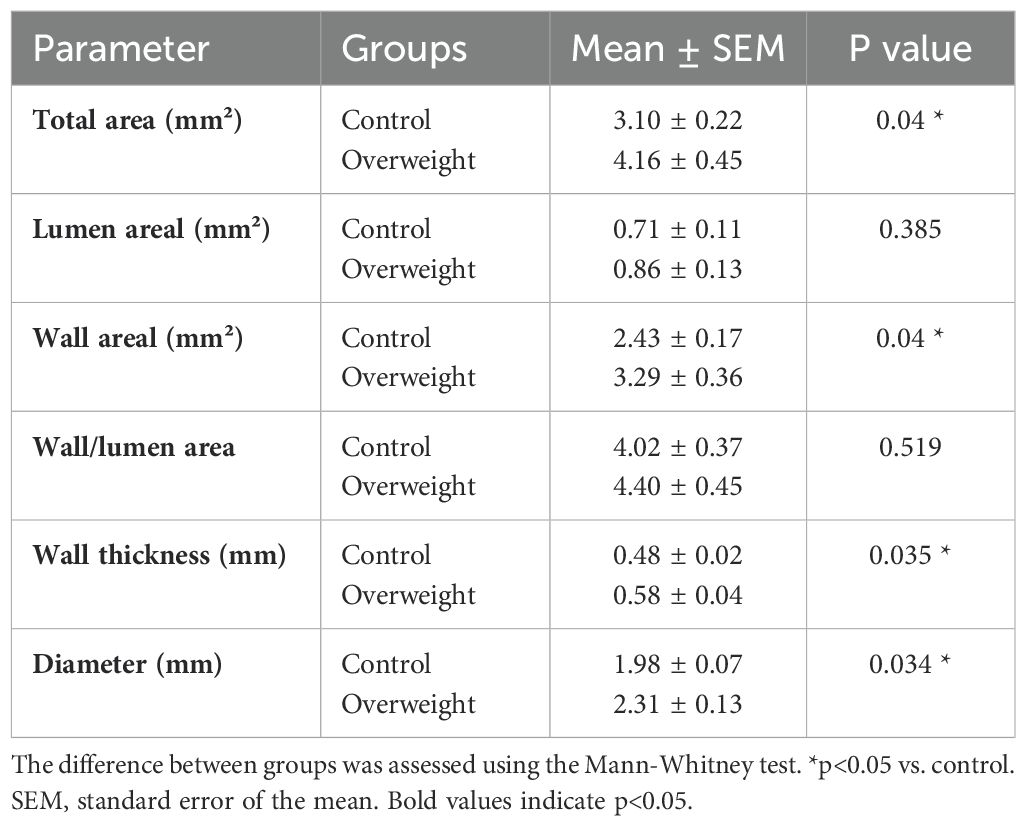
Table 2. Values of umbilical cord vein morphometry analysis of control and overweight pregnant women.
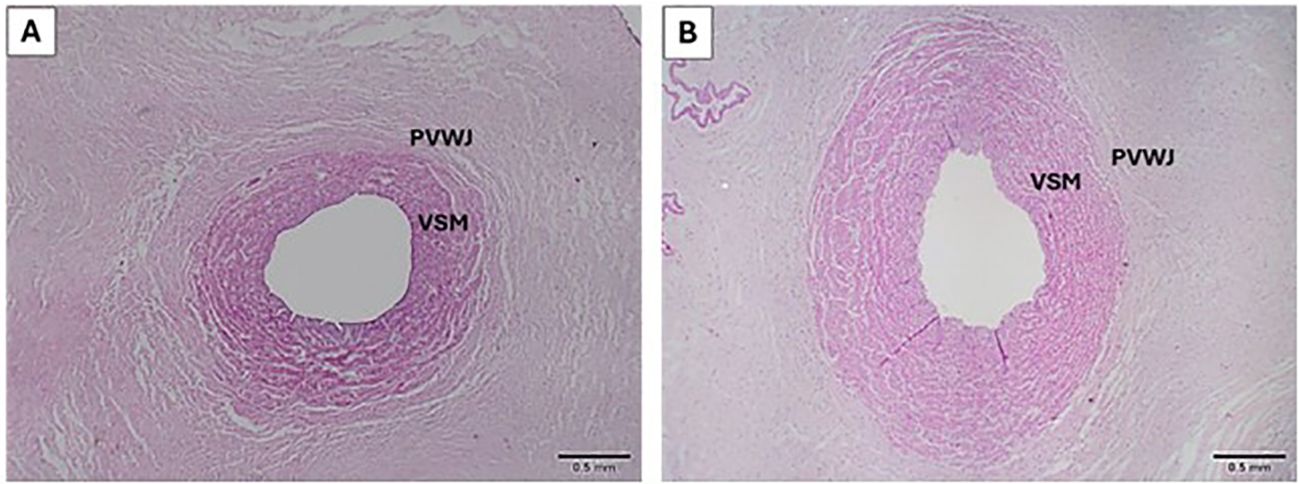
Figure 1. Representatives images of umbilical veins from control (A) and overweight (B) groups. PVWJ, Perivascular Wharton's Jelly; VSM, Vascular Smooth Muscle. H&E 40x.
3.2 Collagen and elastin deposition
Total collagen and elastin deposition was evaluated around the PVWJ and between the vascular smooth muscle (VSM) cells from the umbilical vein. Greater deposition of total collagen in the PVWJ from umbilical veins was observed in the overweight group, compared to the control. However, total collagen deposition between the VSM was similar between groups (Figure 2). Polarized microscopy was performed to access type I and III collagen quantification. In the PVWJ region, type I and III collagens were similar between groups. Interestingly, collagen III was more abundant in VSM in the umbilical veins from the overweight group, compared to the control, and no differences were observed related to type I collagen in VSM between groups (Table 3, Figure 2).
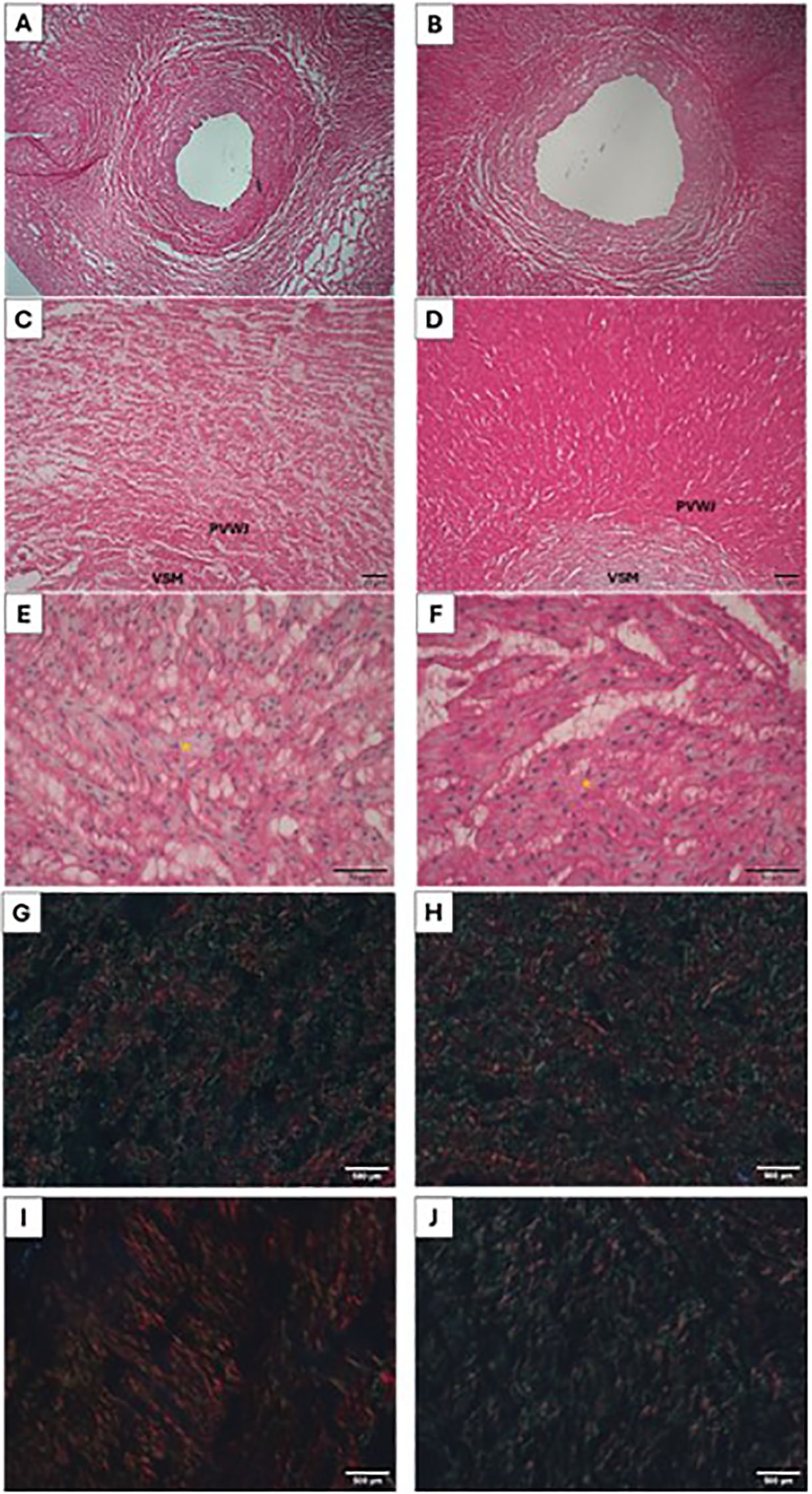
Figure 2. Micrograph of the umbilical vein for collagen analysis in the control (A, C, E, G, I) and overweight (B, D, F, H, J) groups. In (A, C), it is possible to observe less collagen deposition (red) in the perivascular region when compared to (B, D) (Picrosirius 40x and 100x). Collagen deposition between smooth muscle cells (red), nuclei stained with hematoxylin (yellow asterisks) [(E, F), (Picrosirius 400x)]. Type I (red) and type III (green) collagen deposition in the perivascular (G, H) and vascular smooth cells (I, J) regions, under polarized light (Picrosirius 200x).
Elastin deposition was smaller between VSM from the overweight group, compared to the control. No differences were observed in elastin deposition around PVWJ from the umbilical vein (Table 4, Figure 3).
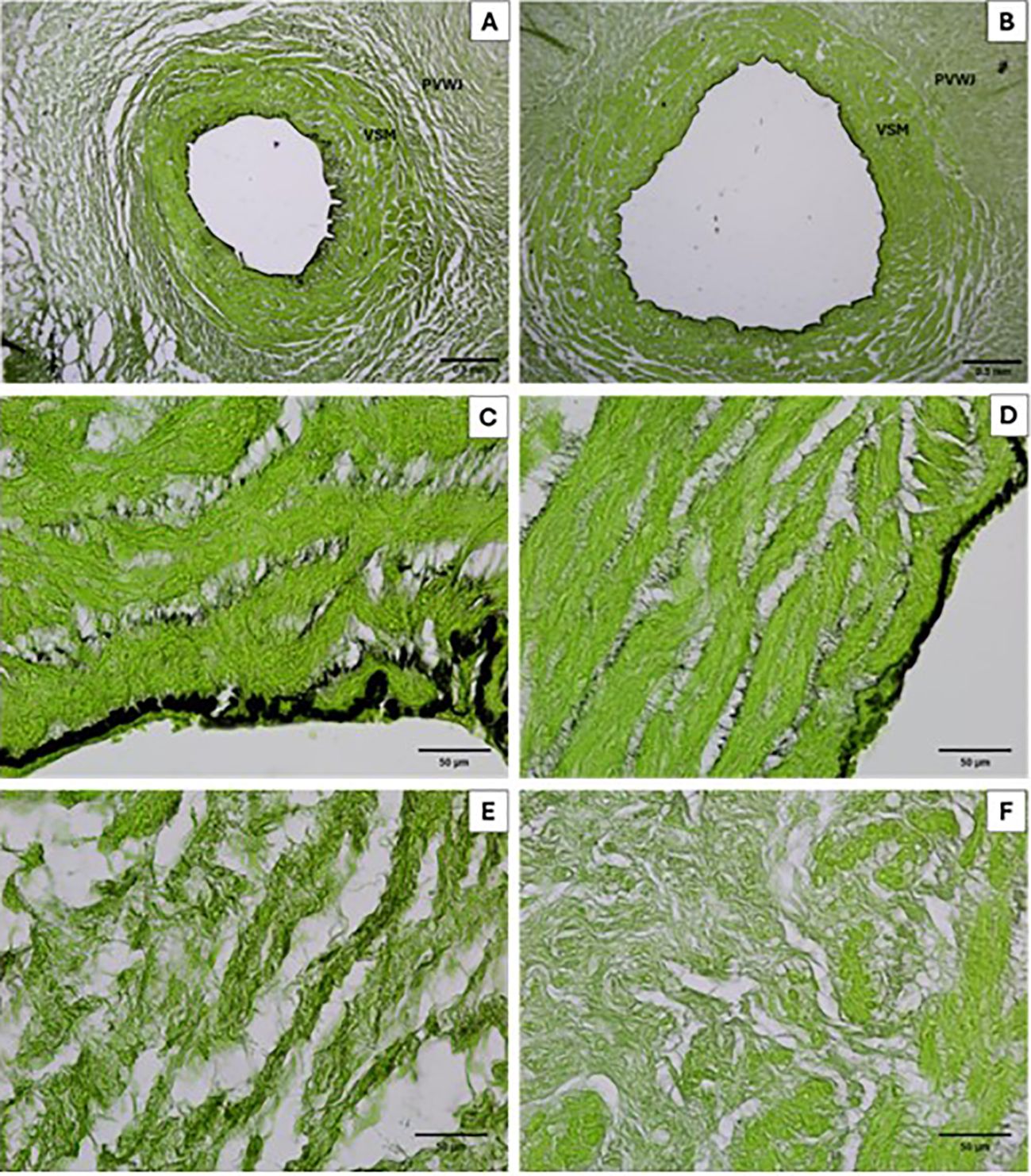
Figure 3. Micrograph of the umbilical vein for elastin analysis in the control (A, C, E) and overweight (B, D, F) groups. In (A, C), it is possible to observe more elastin deposition (black) in the vascular region when compared to (B, D) (Resorcin-fuchsin 40x and 400x). No difference were observed in the perivascular region (E, F).
3.3 Glycosaminoglycans deposition
The distribution of GAGs was evaluated in the perivascular and smooth muscle regions of the umbilical vein (Figure 4). There was no difference in GAGs deposition between the groups, as shown in Table 5.
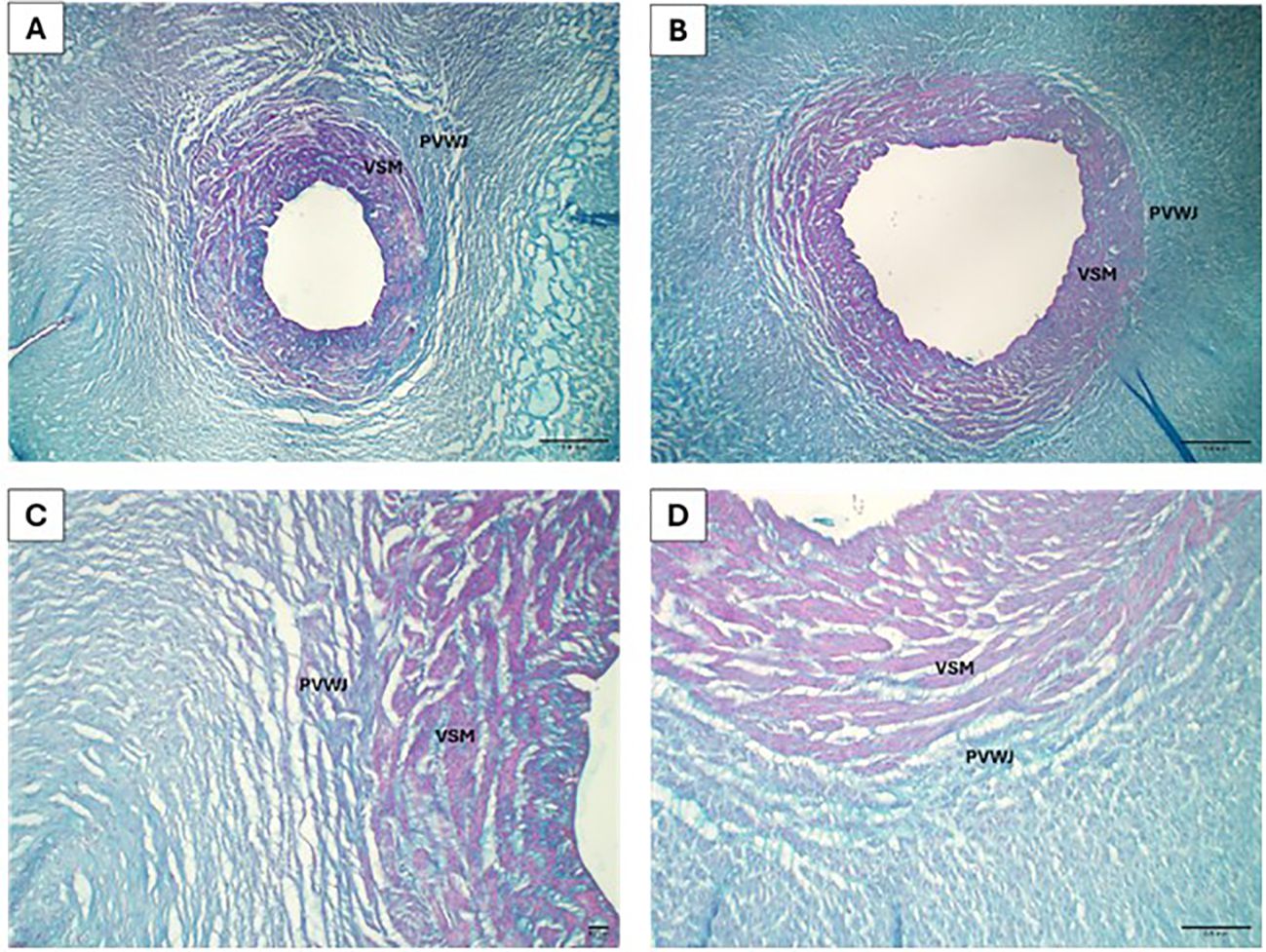
Figure 4. Micrograph of the umbilical vein for glycosaminoglycans analysis in the control (A, C) and overweight (B, D) groups. Veins from the control (A) and overweight (B) groups are observed, with the glycosaminoglycans stained in blue (Alcian blue, 40x). The delimitation between PVWJ and VSM is evident in the control (C) and overweight (D) groups (Alcian blue, 100x).
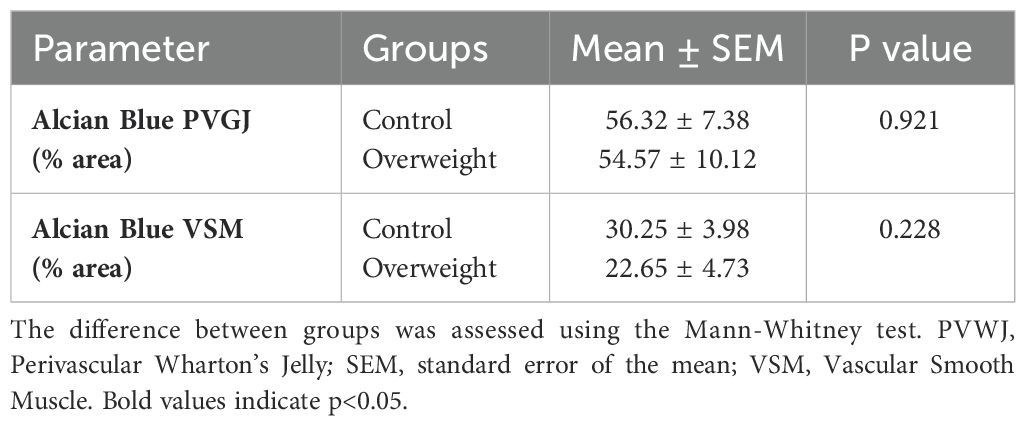
Table 5. Glycosaminoglycans deposition in the perivascular Wharton’s Jelly and around Smooth Muscle Cells.
3.4 Cell proliferation
Cell proliferation, accessed with positive labeling for the KI-67 antibody, was observed in the PVWJ and VSM from umbilical veins. In the overweight group, both regions showed a higher percentage of labeled cells when compared to the control group (Table 6), indicating greater cell proliferation in these regions in the group (Figure 5).
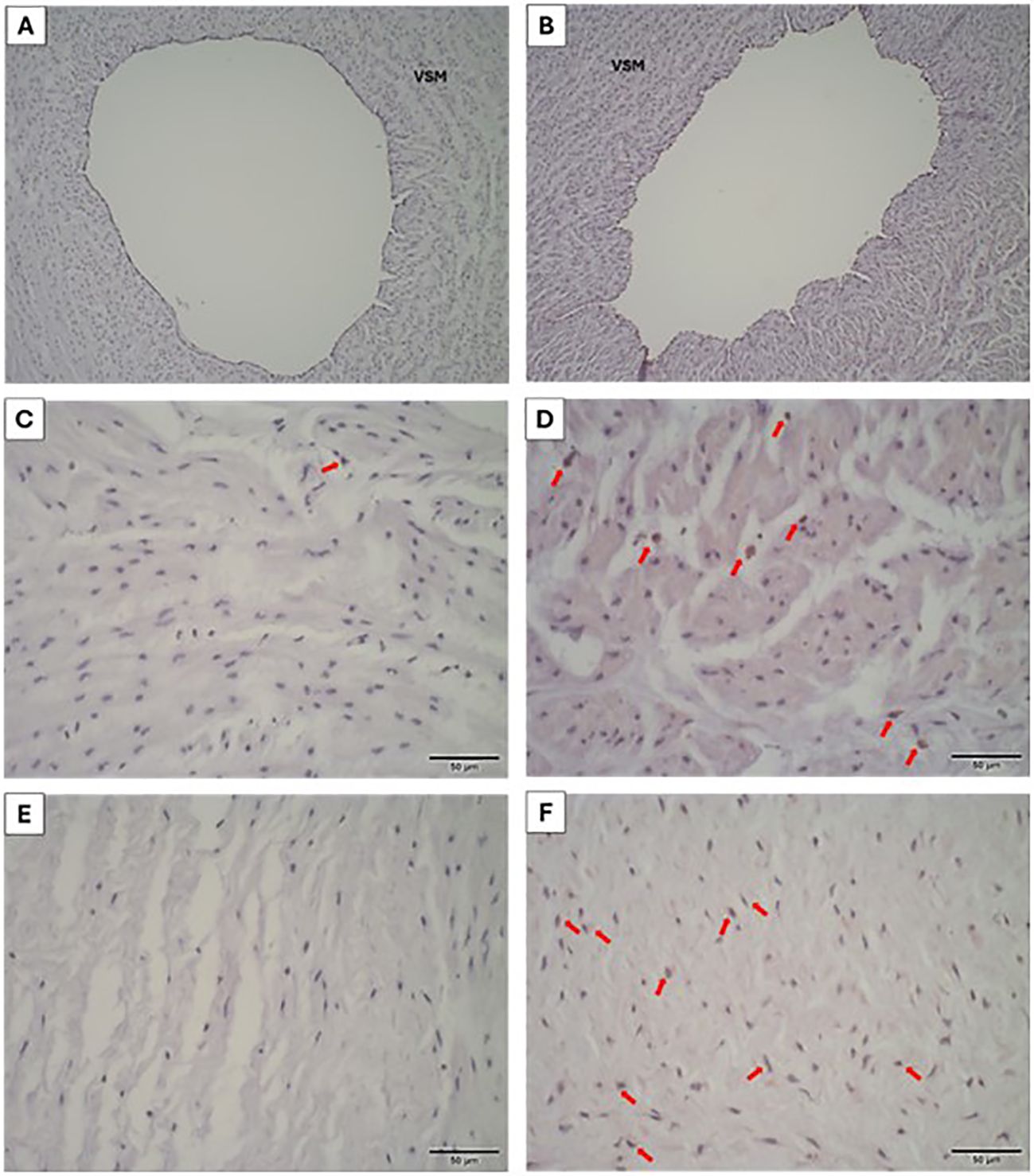
Figure 5. Cell proliferation in the umbilical vein from control (A, C, E) and overweight (B, D, F) groups. Positive staining (red arrows) for KI-67 in the VSM (C, D) and PVWJ (E, F) regions (IHC, 400x).
3.5 BMI and fetal weight correlation
The correlation test showed a positive association between BMI and vein thickness (r=0.357; p=0.035) and type III collagen in vascular smooth muscle (r=0.567; p=0.022), as shown in Figure 2 and Table 7. Fetal weight negatively correlated with the lumen area (r=0.4038; p=0.027) and positively correlated with the wall/lumen area (r=0.4050; p=0.023), Table 8. For those other parameters, no significant correlations were found.
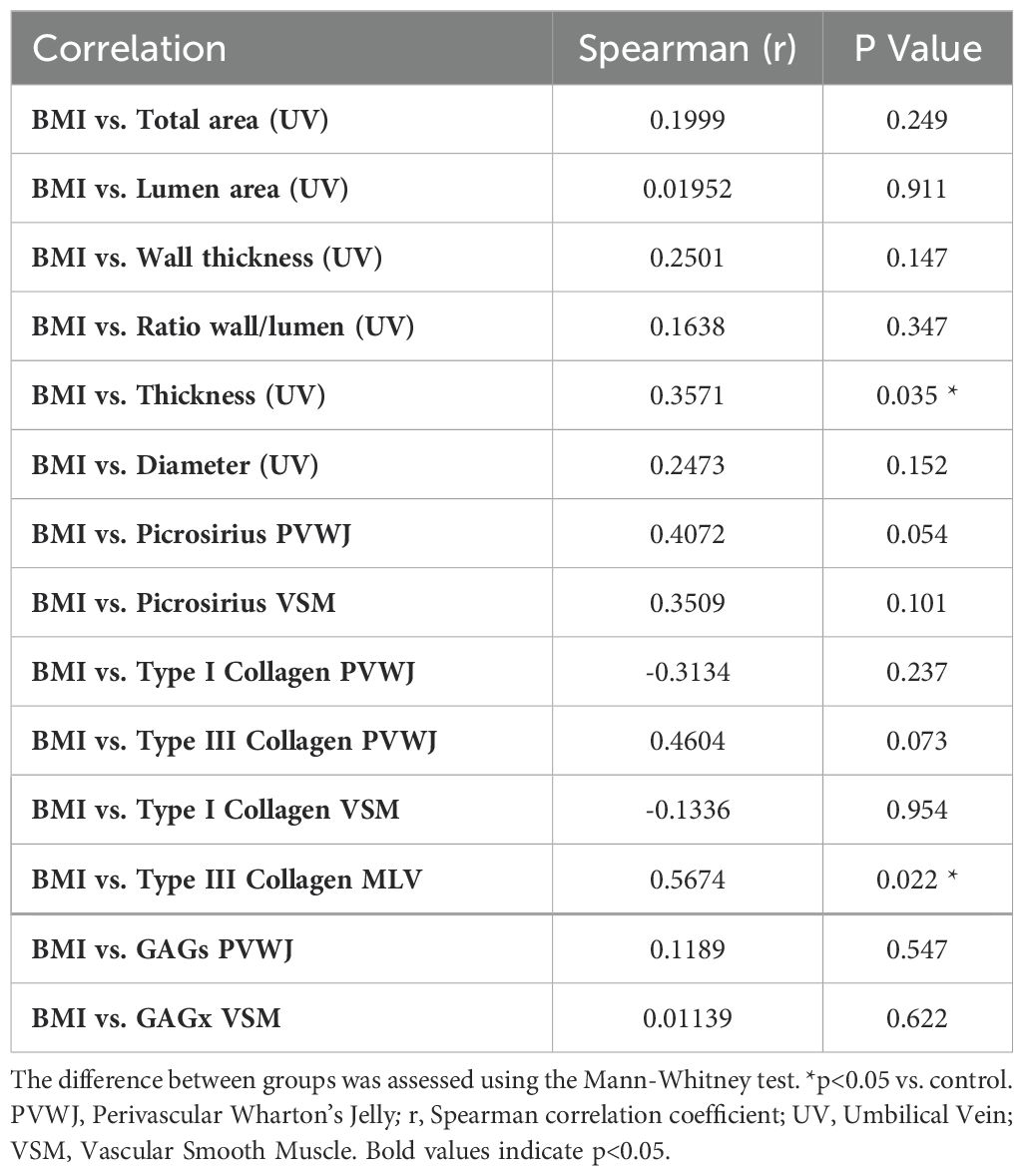
Table 7. Correlations values between BMI and umbilical vein histological parameters from the control and overweight groups.
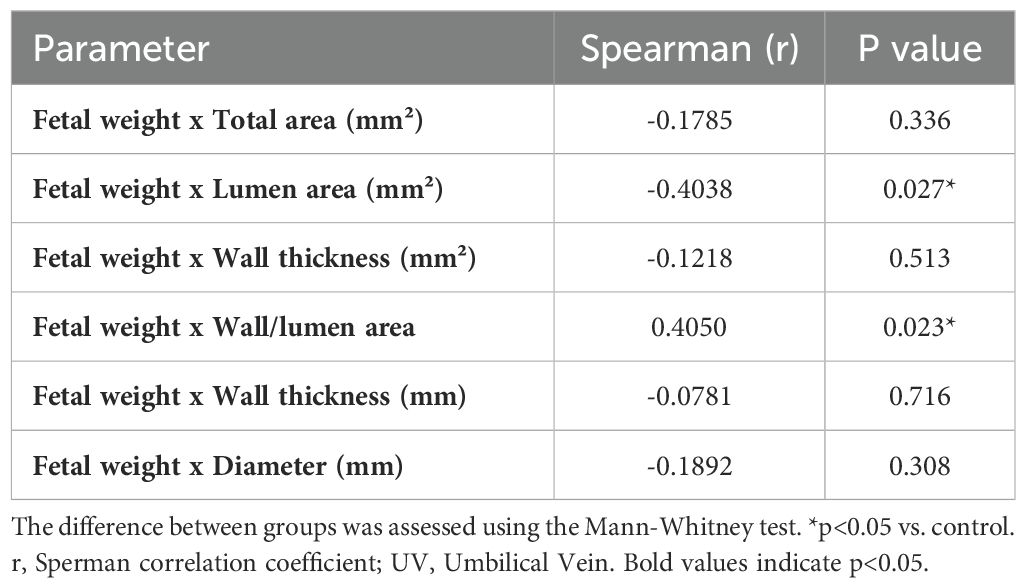
Table 8. Correlations values between fetal weight and umbilical vein histological parameters from the control and overweight groups.
4 Discussion
In the present study, it was demonstrated that the umbilical vein undergoes extensive remodeling in overweight women, increasing the caliber of this vessel through hypertrophy and hyperplasia. Furthermore, greater collagen deposition was observed in the perivascular region, with a predominance of type III collagen in the smooth muscle region, but without changes in glycosaminoglycan deposition. Additionally, a correlation between BMI and vein thickness and type III collagen in smooth muscle was established.
The umbilical vein plays a crucial role during pregnancy, being responsible for transporting blood rich in oxygen and nutrients from the placenta to the fetus (13). This vein connects the placenta to the fetal liver, where blood is distributed throughout the fetal body through fetal circulation. This ensures that the fetus receives the nutrients and oxygen necessary for proper development (14). The blood flow in the umbilical vein is influenced by the helical pattern of the umbilical vessels (15) with an internal pressure of around 2 to 9 mmHg, with an acceleration of 10-22 cm/s. Under normal conditions, the vascular wall responds to shear stress exerted by the blood through the production of vasoactive factors and cytokines (16).
Vascular remodeling occurs as a body response to different stimuli, both physiological and pathological. This process involves changes in the structure and function of blood vessels, such as arteries and veins, and can occur because of the maternal environment, including high blood pressure (17), hyperglycemia (18) and obesity. The vascular remodeling contributes to congenital malformations and reproductive losses, but the mechanisms related to these associations are still unclear (19).
Vascular smooth muscle cells alteration is related to vascular remodeling, since they may switch from contractile to synthetic phenotype (20). This plasticity is necessary to adapt to the different conditions of the vascular tissue upon signs of stress or injury (21). Recent studies have demonstrated that the synthetic phenotype is complex and may acquire characteristics of different mesenchymal lineages, including osteoblastic, chondrocyte and adipocytic (22). This implies that local factors present in the pathological environment probably drive part of this inadequate differentiation. The phenotypic switching contributes to changes in the primary function of these cells from regulation of vessel tone, blood pressure, and blood flow through cells focused on producing extracellular matrix components, assuming a proliferative and migratory phenotype (23).
In pregnancies complicated by obesity, there may be dysregulation in proliferative control in umbilical cord cells. The percentage of cells positive for the cell proliferation marker, KI-67, was higher in the overweight group. In addition to the association with increased vessel caliber resulting from the need to adapt to the adverse environment, factors that are elevated in obesity, such as leptin, induce the proliferation of VSM cells, using the nuclear factor kappa B (NF-kB) and kinase pathways. regulated by extracellular signals (ERK) 1/2 (24). Hence, PVWJ displays some paracrine actions, and releases several substances including growth factors, cytokines, chemokines, exosomes, and microparticles (25), and in theory, these molecules may directly modulate VSM cell proliferation (26). This may occur even due to the interaction of endothelium and PVWJ, since it was demonstrated that the secretome of human endothelial cell-differentiated mesenchymal stem cell enhanced proliferation and wound healing in vivo, in a type-2 diabetes model (27). It was shown that maternal obesity negatively affects PVWJ, suggesting that epigenetic mechanisms may impact PVWJ mesenchymal stromal/stem cells since the methylation of CpG sites was enhanced in samples from obese women (27). However, new, more in-depth studies are necessary to highlight the molecular mechanisms involved in the VSM cell proliferation observed in the umbilical vein.
The presence of arterial stiffness is commonly reported during obesity, both in humans (28–30) and in murine studies (31, 32). The vascular mechanical properties are dependent on the quantity and organization of extracellular matrix components, mainly in relation to collagen and elastin (33).
Collagen is crucial to the normal function and longevity of blood vessels, providing the structure and strength necessary to withstand the demands of the circulatory system (34). Total collagen deposition was increased in the perivascular region of the umbilical vein, indicating a response to structural changes resulting from obesity, in addition to an increase in the proliferation of cells in this region. These findings are consistent with the literature, in which changes in vessel conformation in response to stress signals increase the deposition of extracellular matrix components and promote cell proliferation (35). Furthermore, type III collagen is increased in the VSM of the umbilical vein from overweight pregnant women. A similar observation was done in the umbilical vein of pregnant women with pre-eclampsia (36). Type III collagen has different characteristics from type I, as it does not have high density, its fibers are thinner and have less resistance, allowing the tissue to undergo greater distension (37). In this sense, we can infer that vascular adaptation occurs in the face of remodeling induced by excess weight, in the same way that occurs in the brachial arteries of overweight individuals (38).
A comparison between the saphenous vein and the umbilical vein showed that the first one is stiffer, considering a greater amount of elastin and collagen (39). With this information in mind, the umbilical vein from the control group showed greater stiffness than the overweight group. This fact impacts on the capacity of the vessel to influence the blood flow. In other conditions such as preeclampsia, reduced elastin expression was observed in umbilical cord veins (40). Defective elastin is associated with disorganized elastic fiber deposition and VSM proliferation, causing arterial stiffening (41, 42).
Another extracellular matrix parameter analyzed was the glycosaminoglycans distribution. These molecules form an important network for the diffusion of substances, due to their hydrophilic characteristic, facilitating vessel nutrition (43). However, these polysaccharides also have an important effect on vascular mechanics, contributing to the elastic capacity of the tissue (44). In our data, there were no differences in the number of GAGs in the vascular wall or extracellular matrix. Analysis of the umbilical vein from preeclamptic women displayed similar results (45).
The alterations mentioned above may contribute to several undesirable scenarios regarding fetal health. For example, intrauterine growth restriction may occur due to decreased umbilical vein capacity to provide oxygen and nutrients. Yet, reduced nutritional/oxygen apport elicits commitment to organ development. Yet, fibrosis can increase the risk of hypoxia in the fetus, resulting in perinatal asphyxia, and impacting neurological development (46). Another complication due to low oxygenation and undernutrition is fetal stress, a condition that may be monitored during prenatal examination, leading to interventions that include early birth (47).
Furthermore, this study has limitations associated with the number of samples and collection origin, requiring a larger cohort, with a delimitation between overweight and obesity to infer the influences of each degree separately. Furthermore, here the components of the extracellular matrix were analyzed separately, and tests that demonstrate the interaction dynamics of these components can be used to better understand these findings.
In summary, our data show that maternal excess weight structurally alters the umbilical cord vessels, opening a range of possibilities to be explored in the field of umbilical cord histopathology associated with excess weight. Our findings have clinical relevance, as the context of obesity is common among pregnant women (48).
Data availability statement
The raw data supporting the conclusions of this article will be made available by the authors, without undue reservation.
Ethics statement
The studies involving humans were approved by Ethical Committee of the Federal University of Jataí. The studies were conducted in accordance with the local legislation and institutional requirements. Written informed consent for participation in this study was provided by the participants’ legal guardians/next of kin. Written informed consent was obtained from the individual(s) for the publication of any potentially identifiable images or data included in this article.
Author contributions
KS: Data curation, Formal analysis, Investigation, Methodology, Writing – original draft. LH: Data curation, Formal analysis, Investigation, Software, Writing – review & editing. FC: Data curation, Formal analysis, Investigation, Software, Writing – review & editing. GS: Formal analysis, Investigation, Methodology, Writing – review & editing. GC: Data curation, Investigation, Methodology, Writing – review & editing. JL: Conceptualization, Data curation, Formal analysis, Investigation, Methodology, Writing – review & editing. FA: Investigation, Methodology, Resources, Supervision, Writing – review & editing. FG: Conceptualization, Formal analysis, Resources, Supervision, Validation, Writing – review & editing. NL: Conceptualization, Resources, Supervision, Writing – review & editing, Formal analysis, Investigation, Methodology, Validation. PS: Conceptualization, Data curation, Funding acquisition, Project administration, Resources, Supervision, Writing – review & editing.
Funding
The author(s) declare financial support was received for the research, authorship, and/or publication of this article. This study was financed in part by the Coordination for the Improvement of Higher Education Personnel-Brazil (CAPES; Financing Code 001 to KS); and the National Council for Scientific and Technological Development (CNPQ; project 308899/2022-9 to FG).
Acknowledgments
We acknowledge the Coordination for the Improvement of Higher Education Personnel—Brazil (CAPES) and the National Council for Scientific and Technological Development (CNPq), Brazil for the financial support. We would like to thank the technical support provided by Sebastián San Martín from Biomedical Research Center, School of Medicine, Universidad de Valparaíso, Valparaíso, Chile for his analytical contributions.
Conflict of interest
The authors declare that the research was conducted in the absence of any commercial or financial relationships that could be construed as a potential conflict of interest.
The handling editor AED declared a past collaboration with the author FRG.
Publisher’s note
All claims expressed in this article are solely those of the authors and do not necessarily represent those of their affiliated organizations, or those of the publisher, the editors and the reviewers. Any product that may be evaluated in this article, or claim that may be made by its manufacturer, is not guaranteed or endorsed by the publisher.
References
1. Strauss A. Obesity in pregnant women: maternal, fetal, and transgenerational consequences. Eur J Clin Nutr. (2021) 75:1681–3. doi: 10.1038/s41430-021-01015-z
2. Wilson RM, Marshall NE, Jeske DR, Purnell JQ, Thornburg K, Messaoudi I. Maternal obesity alters immune cell frequencies and responses in umbilical cord blood samples. Pediatr Allergy Immunol. (2015) 26:344–51. doi: 10.1111/pai.2015.26.issue-4
3. Di Naro E, Ghezzi F, Raio L, Franchi M, D’Addario V. Umbilical cord morphology and pregnancy outcome. Eur J Obstetrics Gynecology Reprod Biol. (2001) 96:150–7. doi: 10.1016/S0301-2115(00)00470-X
4. Spurway J, Logan P, Pak S. The development, structure and blood flow within the umbilical cord with particular reference to the venous system. Australas J Ultrasound Med. (2012) 15:97–102. doi: 10.1002/j.2205-0140.2012.tb00013.x
5. Davies JE, Walker JT, Keating A. Concise review: Wharton’s Jelly: the rich, but enigmatic, source of mesenchymal stromal cells. Stem Cells Transl Med. (2017) 6:1620–30. doi: 10.1002/sctm.16-0492
6. Sobolewski K, Bańkowski E, Chyczewski L, Jaworski S. Collagen and glycosaminoglycans of Wharton’s Jelly. Neonatology. (1997) 71:11–21. doi: 10.1159/000244392
8. Melo LG, Gnecchi M, Ward CA, Dzau VJ. Vascular remodeling in health and disease. In: Cardiovascular Medicine. Springer London, London (2007). p. 1541–65.
9. Martínez-Martínez E, Miana M, Jurado-López R, Bartolomé MV, Souza Neto FV, Salaices M, et al. The potential role of leptin in the vascular remodeling associated with obesity. Int J Obes. (2014) 38:1565–72. doi: 10.1038/ijo.2014.37
10. van Varik BJ, Rennenberg RJMW, Reutelingsperger CP, Kroon AA, de Leeuw PW, Schurgers LJ. Mechanisms of arterial remodeling: lessons from genetic diseases. Front Genet. (2012) 3. doi: 10.3389/fgene.2012.00290
11. Bedoya SAO, Conceição LG, Viloria MIV, Loures FH, Valente FL, Amorim RL, et al. Caracterização de colágenos tipos I e III no estroma do carcinoma de células escamosas cutâneo em cães. Arq Bras Med Vet Zootec. (2016) 68:147–54. doi: 10.1590/1678-4162-8484
12. Crowe A, Yue W. Semi-quantitative determination of protein expression using immunohistochemistry staining and analysis: an integrated protocol. Bio Protoc. (2019) 9. doi: 10.21769/BioProtoc.3465
13. Sánchez-Trujillo L, García-Montero C, Fraile-Martinez O, Guijarro LG, Bravo C, De Leon-Luis JA, et al. Considering the effects and maternofoetal implications of vascular disorders and the umbilical cord. Medicina (B Aires). (2022) 58:1754. doi: 10.3390/medicina58121754
14. Barbieri M, Di Martino DD, Ferrazzi EM, Stampalija T. Umbilical vein blood flow: State-of-the-art. J Clin Ultrasound. (2023) 51:318–25. doi: 10.1002/jcu.23412
15. de Laat M, Franx A, van Alderen E, Nikkels P, Visser G. The umbilical coiling index, a review of the literature. J Maternal-Fetal Neonatal Med. (2005) 17:93–100. doi: 10.1080/jmf.17.2.93.100
17. Lan Y, Yang Z, Huang M, Cui Z, Qi Y, Niu H. Morphological and structural changes of umbilical veins and clinical significance in preeclampsia. Hypertens Pregnancy. (2018) 37:105–10. doi: 10.1080/10641955.2017.1420799
18. Tenaw Goshu B. Histopathologic impacts of diabetes mellitus on umbilical cord during pregnancy. Pediatr Health Med Ther. (2022) 13:37–41. doi: 10.2147/PHMT.S323812
19. Ennazhiyil SV, Ramakrishnan P, Akshara V, Premlal K, Chitra S, Benjamin W, et al. Effects of gestational diabetes mellitus on umbilical cord morphology: A comparative study. J Clin Diagn Res. (2019) 13:AC01–04. doi: 10.7860/JCDR/2019/40085.12543
20. Xin Y, Zhang Z, Lv S, Xu S, Liu A, Li H, et al. Elucidating VSMC phenotypic transition mechanisms to bridge insights into cardiovascular disease implications. Front Cardiovasc Med. (2024) 11. doi: 10.3389/fcvm.2024.1400780
21. Shi J, Yang Y, Cheng A, Xu G, He F. Metabolism of vascular smooth muscle cells in vascular diseases. Am J Physiology-Heart Circulatory Physiol. (2020) 319:H613–31. doi: 10.1152/ajpheart.00220.2020
22. Elmarasi M, Elmakaty I, Elsayed B, Elsayed A, Al Zein J, Boudaka A, et al. Phenotypic switching of vascular smooth muscle cells in atherosclerosis, hypertension, and aortic dissection. J Cell Physiol. (2024) 239. doi: 10.1002/jcp.v239.4
23. Frismantiene A, Philippova M, Erne P, Resink TJ. Smooth muscle cell-driven vascular diseases and molecular mechanisms of VSMC plasticity. Cell Signal. (2018) 52:48–64. doi: 10.1016/j.cellsig.2018.08.019
24. Huang F, Xiong X, Wang H, You S, Zeng H. Leptin-induced vascular smooth muscle cell proliferation via regulating cell cycle, activating ERK1/2 and NF- B. Acta Biochim Biophys Sin (Shanghai). (2010) 42:325–31. doi: 10.1093/abbs/gmq025
25. KL P, Kandoi S, Misra R, V S, R K, Verma RS. The mesenchymal stem cell secretome: A new paradigm towards cell-free therapeutic mode in regenerative medicine. Cytokine Growth Factor Rev. (2019) 46:1–9. doi: 10.1016/j.cytogfr.2019.04.002
26. Wang Q, Yang Q, Wang Z, Tong H, Ma L, Zhang Y, et al. Comparative analysis of human mesenchymal stem cells from fetal-bone marrow, adipose tissue, and Warton’s jelly as sources of cell immunomodulatory therapy. Hum Vaccin Immunother. (2016) 12:85–96. doi: 10.1080/21645515.2015.1030549
27. Ormazabal V, Nova-Lampeti E, Rojas D, Zúñiga FA, Escudero C, Lagos P, et al. Secretome from human mesenchymal stem cells-derived endothelial cells promotes wound healing in a type-2 diabetes mouse model. Int J Mol Sci. (2022) 23. doi: 10.3390/ijms23020941
28. Kim HL, Ahn DW, Kim SH, Lee DS, Yoon SH, Zo JH, et al. Association between body fat parameters and arterial stiffness. Sci Rep. (2021) 11:20536. doi: 10.1038/s41598-021-00175-z
29. Safar ME, Czernichow S, Blacher J. Obesity, arterial stiffness, and cardiovascular risk. J Am Soc Nephrology. (2006) 17:S109–11. doi: 10.1681/ASN.2005121321
30. Zebekakis PE, Nawrot T, Thijs L, Balkestein EJ, van der Heijden-Spek J, Van Bortel LM, et al. Obesity is associated with increased arterial stiffness from adolescence until old age. J Hypertens. (2005) 23:1839–46. doi: 10.1097/01.hjh.0000179511.93889.e9
31. Gil-Ortega M, Martín-Ramos M, Arribas SM, González MC, Aránguez I, Ruiz-Gayo M, et al. Arterial stiffness is associated with adipokine dysregulation in non-hypertensive obese mice. Vascul Pharmacol. (2016) 77:38–47. doi: 10.1016/j.vph.2015.05.012
32. Gkousioudi A, Yu X, Ferruzzi J, Qian J, Wainford RD, Seta F, et al. Biomechanical properties of mouse carotid arteries with diet-induced metabolic syndrome and aging. Front Bioeng Biotechnol. (2022) 10. doi: 10.3389/fbioe.2022.862996
33. Aroor AR, Jia G, Sowers JR. Cellular mechanisms underlying obesity-induced arterial stiffness. Am J Physiology-Regulatory Integr Comp Physiol. (2018) 14:R387–98. doi: 10.1152/ajpregu.00235.2016
34. Halper J. Basic components of vascular connective tissue and extracellular matrix. Adv Pharmacol. (2018) 81:95–127. doi: 10.1016/bs.apha.2017.08.012
35. Xu J, Shi GP. Vascular wall extracellular matrix proteins and vascular diseases. Biochim Biophys Acta (BBA) - Mol Basis Disease. (2014) 1842:2106–19. doi: 10.1016/j.bbadis.2014.07.008
36. Romanowicz L, Jaworski S. Collagen of umbilical cord vein and its alterations in pre-eclampsia. Acta Biochim Pol. (2002) 49:451–8. doi: 10.18388/abp.2002_3804
37. Wittig C, Szulcek R. Extracellular matrix protein ratios in the human heart and vessels: how to distinguish pathological from physiological changes? Front Physiol. (2021) 12:708656. doi: 10.3389/fphys.2021.708656
38. Chung WB, Hamburg NM, Holbrook M, Shenouda SM, Dohadwala MM, Terry DF, et al. The brachial artery remodels to maintain local shear stress despite the presence of cardiovascular risk factors. Arterioscler Thromb Vasc Biol. (2009) 29:606–12. doi: 10.1161/ATVBAHA.108.181495
39. Alhosseini Hamedani B, Navidbakhsh M, Ahmadi Tafti H. Comparison between mechanical properties of human saphenous vein and umbilical vein. BioMed Eng Online. (2012) 11:59. doi: 10.1186/1475-925X-11-59
40. Junek T, Baum O, Läuter H, Vetter K, Matejevic D, Graf R. Pre-eclampsia associated alterations of the elastic fibre system in umbilical cord vessels. Anat Embryol (Berl). (2000) 201:291–303. doi: 10.1007/s004290050318
41. Mochizuki S, Brassart B, Hinek A. Signaling pathways transduced through the elastin receptor facilitate proliferation of arterial smooth muscle cells. J Biol Chem. (2002) 277:44854–63. doi: 10.1074/jbc.M205630200
42. Urbán Z, Riazi S, Seidl TL, Katahira J, Smoot LB, Chitayat D, et al. Connection between elastin haploinsufficiency and increased cell proliferation in patients with supravalvular aortic stenosis and Williams-Beuren syndrome. Am J Hum Genet. (2002) 71:30–44. doi: 10.1086/341035
43. Lepedda AJ, Nieddu G, Formato M, Baker MB, Fernández-Pérez J, Moroni L. Glycosaminoglycans: from vascular physiology to tissue engineering applications. Front Chem. (2021) 9:680836. doi: 10.3389/fchem.2021.680836
44. Mattson JM, Turcotte R, Zhang Y. Glycosaminoglycans contribute to extracellular matrix fiber recruitment and arterial wall mechanics. Biomech Model Mechanobiol. (2017) 16:213–25. doi: 10.1007/s10237-016-0811-4
45. Romanowicz L, Sobolewski K. Extracellular matrix components of the wall of umbilical cord vein and their alterations in pre-eclampsia. J Perinat Med. (2000) 28:140–146. doi: 10.1515/JPM.2000.019
46. Ghesquière L, Perbet R, Lacan L, Hamoud Y, Stichelbout M, Sharma D, et al. Associations between fetal heart rate variability and umbilical cord occlusions-induced neural injury: An experimental study in a fetal sheep model. Acta Obstet Gynecol Scand. (2022) 101:758–70. doi: 10.1111/aogs.14352
47. Maršál K. Physiological adaptation of the growth-restricted fetus. Best Pract Res Clin Obstet Gynaecol. (2018) 49:37–52. doi: 10.1016/j.bpobgyn.2018.02.006
Keywords: collagen fibers, elastin fibers, glycosaminoglycans, KI-67, umbilical cord
Citation: Souza KBdS, Hochberger LC, Castrignano Camargo FE, Silva GS, Camargo GC, Mello JPL, Alcantara Dos Santos FC, Giachini FR, Lobato NdS and Souto PCdS (2024) Umbilical vein remodeling is associated with pregestational maternal overweight. Front. Endocrinol. 15:1483364. doi: 10.3389/fendo.2024.1483364
Received: 19 August 2024; Accepted: 29 October 2024;
Published: 20 December 2024.
Edited by:
Alicia E. Damiano, University of Buenos Aires, ArgentinaReviewed by:
Jimena Beltrame, CONICET Centro de Estudios Farmacológicos y Botánicos (CEFYBO), ArgentinaPaola Ayala, Pontifical Javeriana University, Colombia
Copyright © 2024 Souza, Hochberger, Castrignano Camargo, Silva, Camargo, Mello, Alcantara Dos Santos, Giachini, Lobato and Souto. This is an open-access article distributed under the terms of the Creative Commons Attribution License (CC BY). The use, distribution or reproduction in other forums is permitted, provided the original author(s) and the copyright owner(s) are credited and that the original publication in this journal is cited, in accordance with accepted academic practice. No use, distribution or reproduction is permitted which does not comply with these terms.
*Correspondence: Fernanda Regina Giachini, ZmVybmFuZGFnaWFjaGluaUBob3RtYWlsLmNvbQ==; ZmVybmFuZGEudml0b3Jpbm9AdWZtdC5icg==