- 1Department of VIP Region, Collaborative Innovation Center for Cancer Medicine, State Key Laboratory of Oncology in South China, Guangdong Provincial Clinical Research Center for Cancer, Sun Yat-sen University Cancer Center, Guangzhou, China
- 2Collaborative Innovation Center for Cancer Medicine, State Key Laboratory of Oncology in South China, Guangdong Provincial Clinical Research Center for Cancer, Sun Yat-sen University Cancer Center, Guangzhou, China
- 3Department of Obstetrics and Gynecology, Zhuhai People’s Hospital, Zhuhai Hospital Affiliated with Jinan University, Zhuhai, China
Background: It is necessary to find latent indicators to predict the survival of alcohol-associated liver disease (ALD) patients. Leukocyte telomere length (LTL) was regarded as an indicator of prognosis in several diseases. However, the relationships between LTL and survival as well as cause-specific mortality in ALD patients were still unknown.
Objective: This study aimed at exploring the underlying link between LTL and the risk of mortality in patients with ALD.
Methods: The LTL and survival data were gathered from the National Health and Nutrition Examination Survey (NHANES) 1999–2002. The connection between LTL and mortality was assessed by Cox regression models and stratified analyses. The non-linear relationship was explored by restricted cubic spline (RCS) analysis. Sensitivity analyses were used to evaluate the robustness of our findings.
Results: LTL was a negative factor for all-cause mortality (all p-value < 0.05). The risk of cardiovascular disease (CVD)-related death was decreased in Q3 (p < 0.001) and Q4 levels of LTL (p < 0.001) compared with the Q1 group. Shorter LTL resulted in higher cancer-caused mortality (p = 0.03) in the Q2 group. Longer LTL improved survival especially for elder patients (p for trend < 0.001) or men (p for trend = 0.001). Moreover, there were L-shaped correlations between LTL and all-cause mortality (p for non-linearity = 0.02), as well as cancer-related mortality (p for non-linearity < 0.001). Four sensitivity analyses proved the robustness of our findings.
Conclusion: Our research found that longer LTL improved survival in patients with ALD and decreased CVD and cancer-related mortality. LTL decreased all-cause mortality especially for patients older than 65 years or men. LTL might be a useful biomarker for prognosis among patients with ALD. More prospective studies are needed to assess the relevance between LTL and mortality and explore the underlying mechanisms between them.
1 Introduction
Alcohol-related liver disease (ALD) is a common type of liver condition, responsible for approximately a quarter of cirrhosis-related deaths. There are approximately 2.18 million patients with ALD in the United States (1). Approximately 10% of ALD patients experienced further aggravation of their cirrhosis (2, 3). Furthermore, ALD accounts for a large number of patients who received liver transplantation in the United States (4). ALD has a poor prognosis, and symptoms, such as jaundice, hepatorenal syndrome, and hepatopulmonary syndrome, usually occur at the last stage (5, 6). The mortality of ALD is currently 1.75 times higher than 20 years ago in the United States (7). Because of this, it is necessary to find biomarkers for predicting the survival of ALD patients. Though several imaging techniques and prognostic models have provided pertinent evidence to assess the process of ALD, it still remains controversial (8–11).
Telomeres are located at the end of the chromosomes and their function is to maintain DNA stability (12). Generally, telomeres become shorter as the cells divide. When the length becomes extremely short, cells will become senescent or apoptotic (13). Thereby, leukocyte telomere length (LTL) was regarded as an index of the biological aging process of cells, and it is a common phenomenon occurring in any cellular tissues (14). LTL reflected the senescent status of circulating immune cells (12). LTL was reported to be related to many diseases, such as multiple sclerosis (MS), malignant neoplasms, and cardiovascular diseases (CVDs) (15–19). Longer LTL decreased the risk of NAFLD incidence and mediated the link between age and NAFLD in the UK Biobank (20). Another recent Mendelian randomization study illustrated that there was no clear association between LTL and ALD (21). Nevertheless, we still do not know how LTL affects prognosis in patients with ALD. LTL was related to advanced liver disease among old people, and it had a negative relationship with all-cause mortality in patients with liver disease (22). Furthermore, the level of LTL was considered as a symbol of all-cause mortality in certain populations (23, 24). Thereby, the telomere length of leukocytes had a positive influence on the prognosis of age-related diseases (25–28). However, it remains uncertain whether LTL was a prospective predictor of survival and cause-specific death in individuals with ALD.
Therefore, we hypothesized that LTL might correlate with all-cause and cause-specific mortality in ALD patients. Our study was aimed at exploring the latent link between LTL and all-cause and cause-specific mortality in patients with ALD from the United States so that high-risk patients can take effective measures to improve their survival.
2 Methods
2.1 Study population
The data in this retrospective research were sourced from NHANES 1999–2002 and were acquired from the website https://www.cdc.gov/nchs/nhanes/index.htm. Every enrolled participant was asked to provide an informed consent in written form. The National Center for Health Statistics Research Ethics Review Board (NCHS ERB) approved, censored, and ratified the program.
After screening, data from 2,519 participants after screening were analyzed based on specific inclusion and exclusion criteria. The exclusion criteria were as follows: (a) did not have ALD, (b) missing data on LTL, (c) younger than 20 years, (d) missing data on covariates, and (e) follow-up data. The detailed selection procedure is displayed in Figure 1.
2.2 ALD status
ALD was identified according to a previous study. In summary, adults were classified as ALD if they met the following criteria: (1) consumed over 28 g of alcohol daily for women and over 42 g for men in the past year, (2) had high levels of liver enzymes such as AST and ALT, and (3) did not have HCV/HBV or NAFLD (29).
2.3 Study variables
In the “laboratory” portion of the NHANES interview, LTL was collected. Polymerase chain reaction was used to evaluate the telomere length. The relative ratio against standard reference DNA (T/S) was determined through calculation. The base pairs were modified for analysis using information based on previous research (12, 24). The detailed information was shown on the NHANSE website.
2.4 Other covariates
From the demographic information section, we gathered data on age, gender, race, marital status, level of education, the income-to-poverty ratio (PIR), and smoking habits. Data on albumin (g/L), C-reactive protein (CRP, mg/dL), alanine aminotransferase (ALT, U/L), aspartate transaminase (AST, U/L), alkaline phosphatase (ALP, U/L), total bilirubin (mg/dL), and lactate dehydrogenase (LDH, U/L) were gathered from the examination information section and used as indicators of liver function. The history of hypertension, DM, and other comorbidities was based on questionnaire data.
2.5 Follow-up data
The follow-up of this study ended on 31 December 2019. Death from all reasons was defined as all-cause mortality. Other causes such as CVD (I00–I09, I11, I13, I20–I51, and I60–I69), cancer (C00–C97), DM (E10–E14), chronic lower respiratory diseases (J40–J47), Alzheimer’s disease (G30), nephritis, nephrotic syndrome, and nephrosis (N00–N07, N17–N19, and N25–N27), accidents (unintentional injuries) (V01–X59 and Y85–Y86), and influenza and pneumonia (J09–J18) were categorized according to the International Statistical Classification of Diseases and Related Health Issues (ISCDRH).
2.6 Statistical methods
Sample weights were considered in our study in accordance with the NCHS analytic guidelines. All methods in this study were performed in accordance with the relevant guidelines and regulations in the NHANES website. Continuous variables were described as means ± standard errors and categorical variables were shown as number (percentage), respectively. We divided TLT into quadrants; the ranges of LTL in Q1, Q2, Q3, and Q4 were (0.39,0.834], (0.83,0.98], (0.98,1.16], and (1.157,9.42], respectively. t-test, Mann–Whitney U test, and chi-square test were employed for comparing normally distributed data, non-normally distributed continuous data, and categorical variables, respectively. The association between LTL and all-cause or cause-specific mortality was explored by four Cox regression models. No adjustments for covariates were made in Model 1. Model 2 was modified to account for age, gender, and race. Model 3 was additionally modified by taking into account education, smoke, hypertension, and diabetes, building upon the adjustments made in Model 2. Model 4 was calibrated for additional laboratory parameters, such as albumin, CRP, ALT, AST, ALP, total bilirubin, and LDH. Furthermore, prognostic differences among the four levels of TLT were estimated using Kaplan–Meier analysis and the log-rank test. The non-linear relationship was evaluated by restricted cubic spline (RCS) analyses. Stratified analysis was carried out to assess the variances among different subgroups. Finally, the robustness of our findings was evaluated by performing four sensitivity analyses in this research. The first and second analyses were carried out after excluding individuals who died within 1 year or 2 years of follow-up. The third analysis was conducted after excluding individuals with cancer. The fourth analysis did not adjust for NHANES survey weights.
The analyses mentioned above were conducted using R (version 4.3.3) and R Studio. The R packages “rms”, “survey”, and “ggplot2” were used. p-value less than 0.05 was deemed to be statistically significant.
3 Results
3.1 Baseline characteristics of included patients in NHANES 1999–2002
Among the 2,519 enrolled ALD patients, 409 (9.04%) were older than 65 years and 2,110 (90.96%) were younger. There were 1,775 (69.02%) men. A total of 1,689 (82.70%) patients were Non-Hispanic white or black, 648 (7.40%) were Mexican American, and 182 (9.90%) patients were from other races or were multiracial. A total of 928 (31.71%) individuals had hypertension, and 257 (7.14%) had diabetes. Patients with a higher level of LTL seemed younger (p < 0.001). Fewer patients had hypertension (p < 0.001) or DM (p < 0.001) in the higher LTL group. Moreover, CRP (p < 0.001), ALP (p = 0.004), and LDH (p = 0.002) tended to decrease in higher levels of LTL, as shown in Table 1. We further analyzed whether LTL was related to comorbidity profile among patients with ALD. Except for hypertension or DM, patients with longer LTL had a lower risk of chronic kidney disease, CVD, peripheral arterial disease, and metabolic syndrome (all p < 0.05), as shown in Supplementary Table 1.
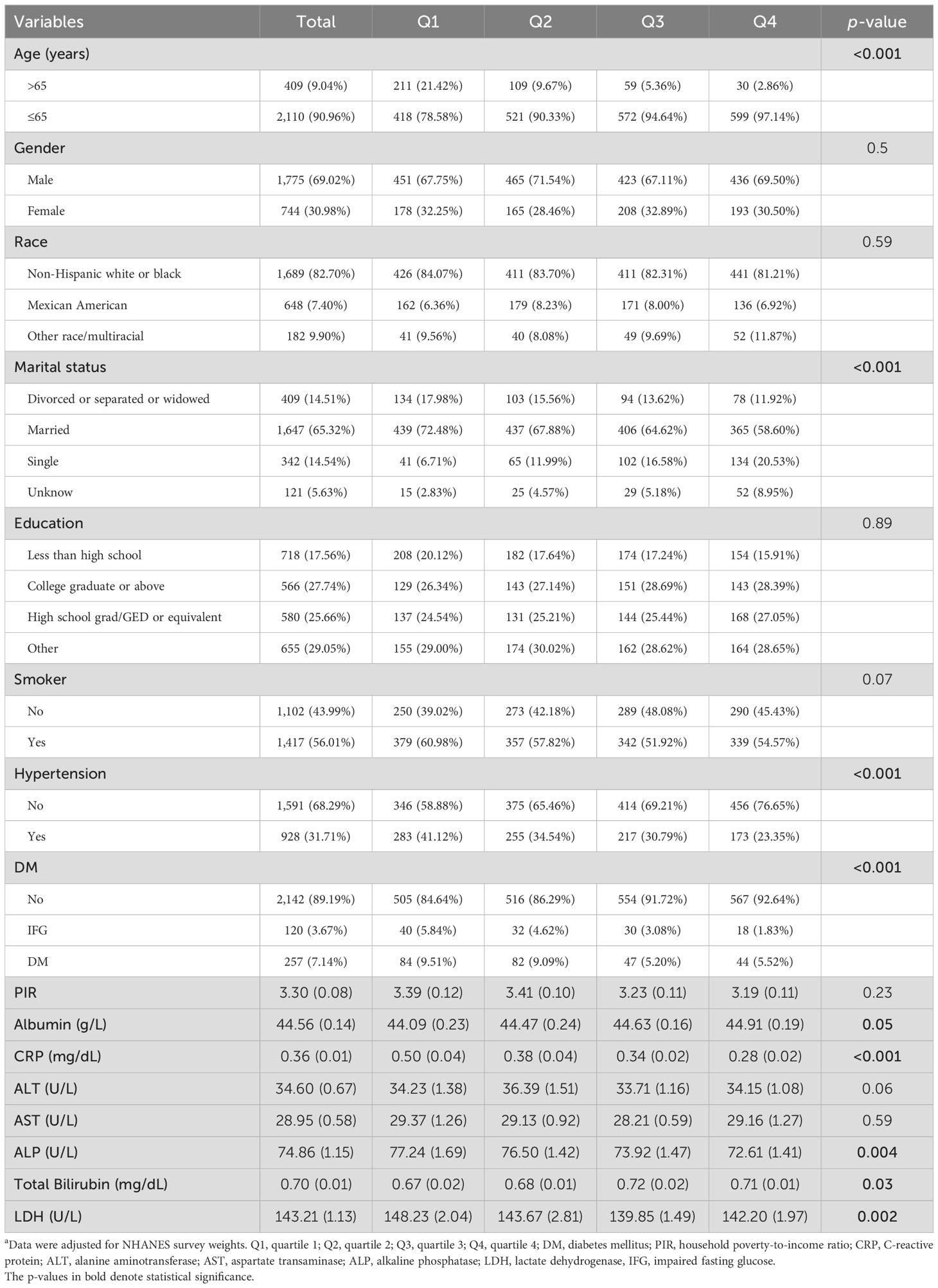
Table 1. Baseline characteristics of included patients in NHANES 1999–2002 a.
In addition, a total of 552 (15.49%) patients died at the end of follow-up. Mortality was the highest in the Q1 group, which had the lowest level of LTL (p < 0.001). Causes of death included accidents (4.30%), Alzheimer’s disease (4.52%), cancer (26.45%), CVD (28.99%), DM (3.26%), influenza or pneumonia (2.72%), kidney diseases (1.81%), chronic lower respiratory diseases (4.90%), and other causes (23.00%). The percentage of mortality was the highest in the Q4 group, suggesting that there was a relationship between higher LTL and decreased risk of death in patients with ALD (Table 2).
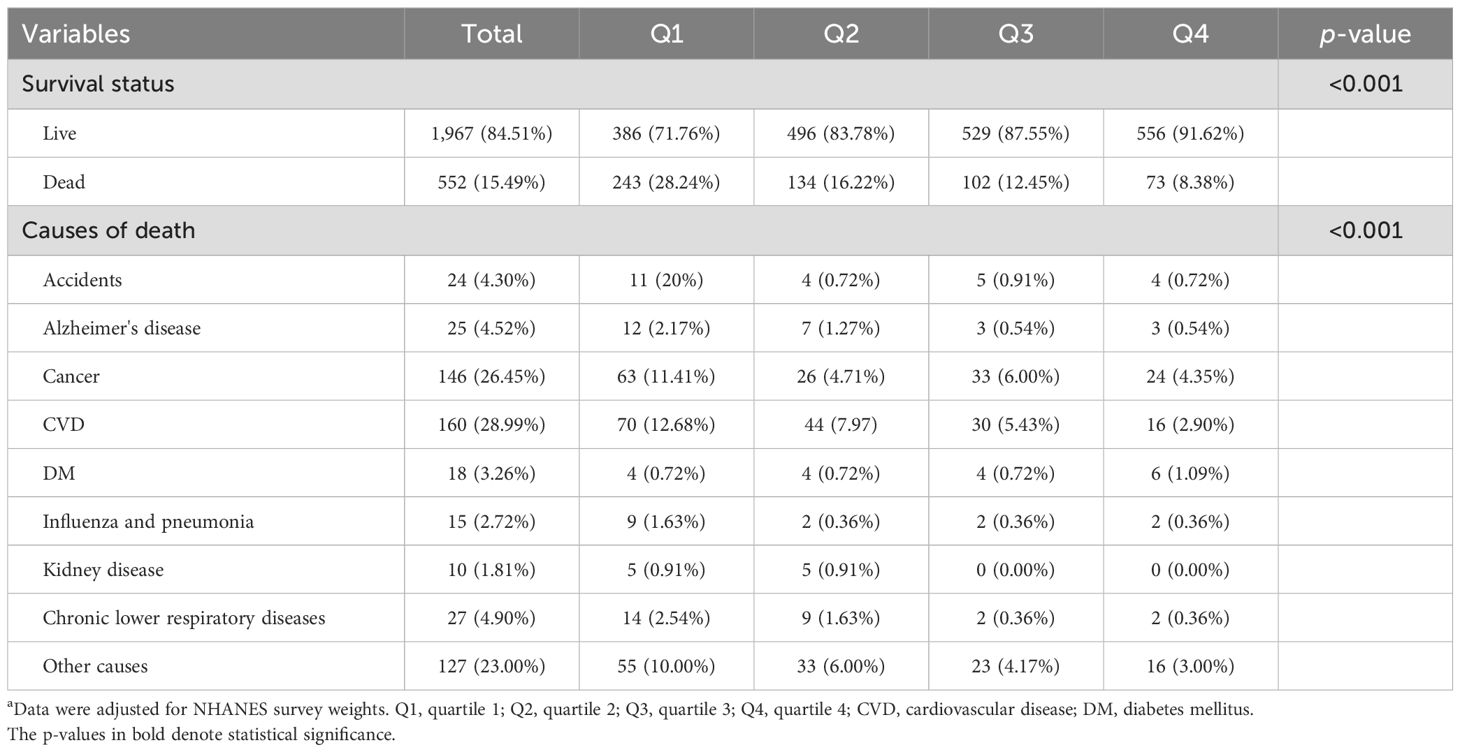
Table 2. Mortality distribution among ALD patients a.
3.2 The association between telomere length and all-cause mortality in ALD patients
Table 3 illustrates the association between LTL and all kinds of mortality. Longer LTL reduced all-cause mortality in Q2 (HR = 0.54 [0.42–0.71], p < 0.001), Q3 (HR = 0.26 [0.14–0.48], p < 0.001), and Q4 (HR = 0.12 [0.06–0.25], p < 0.001) compared with the Q1 group in Model 1. In Model 2, LTL remained a protective factor with HRs and 95% CIs of 0.72 [0.55–0.96], 0.64 [0.45–0.90], and 0.46 [0.33–0.64] in the Q2, Q3, and Q4 groups (all p-value < 0.05), respectively. The difference still existed in Model 3 and Model 4.
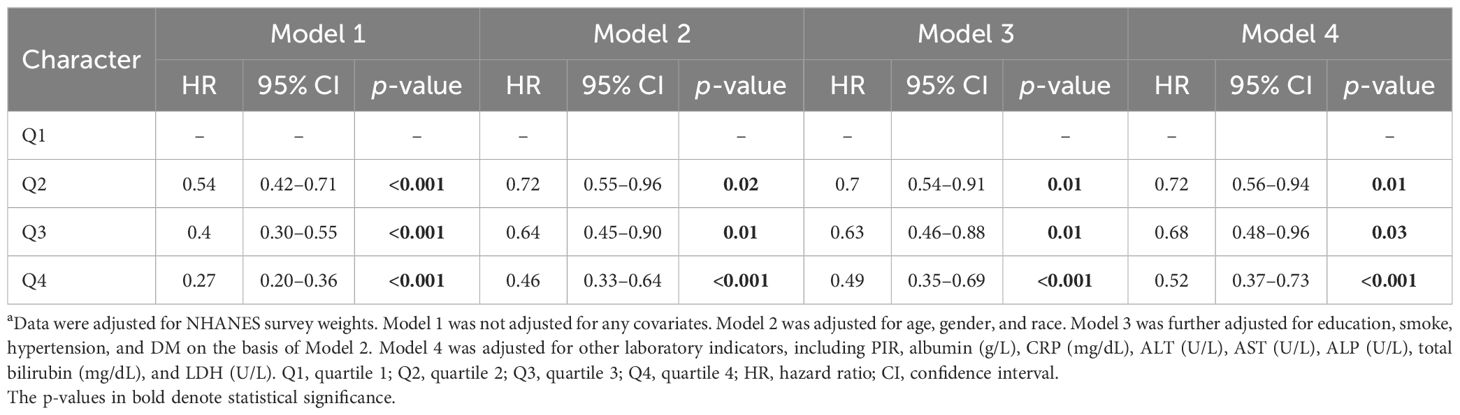
Table 3. The association of LTL with all-cause mortality a.
Consistently, LTL remained negatively associated with all-cause mortality when it was regarded as a continuous variable (Supplementary Table 2). Figure 2A shows the Kaplan–Meier survival curve (p < 0.001).
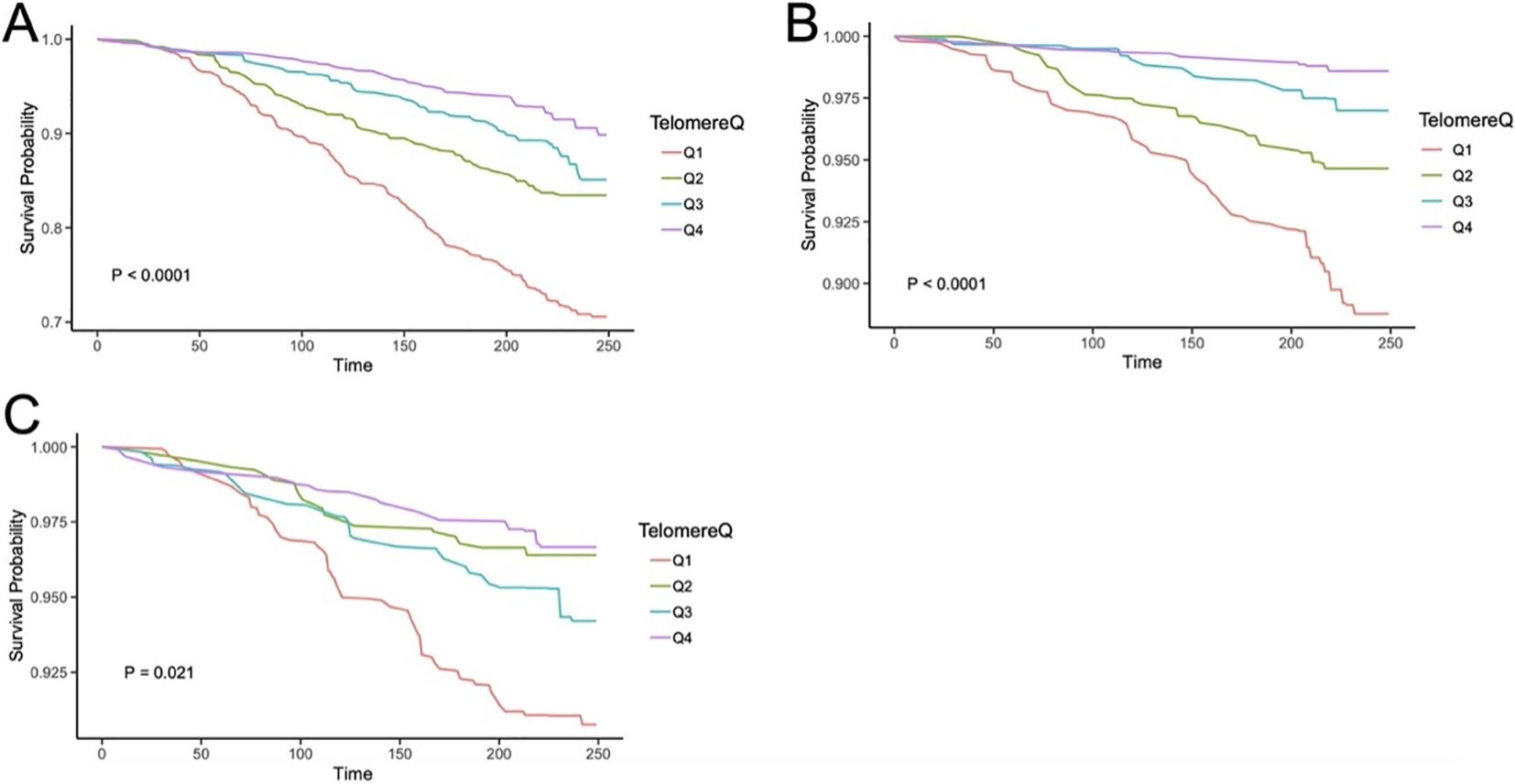
Figure 2. The Kaplan–Meier curves for LTL associating with all-cause mortality (A), CVD mortality (B), and cancer-caused mortality (C) in ALD patients. Q1, quartile 1; Q2, quartile 2; Q3, quartile 3; Q4, quartile 4; CVD, cardiovascular disease.
3.3 The correlation between LTL and cause-specific mortality in patients with ALD
As regards mortality caused by cardiovascular disease, LTL decreased the risk of death in the crude model (p < 0.001 for all groups) compared to the Q1 group. In Model 2, both the Q3 and Q4 groups had lower HRs (0.40 [0.23–0.67] for Q3, p < 0.001; 0.2 [0.1–0.3] for Q4, p < 0.001) compared with the Q1 group. The results in Model 3 and Model 4 were consistent with Model 2, as indicated in Table 4.
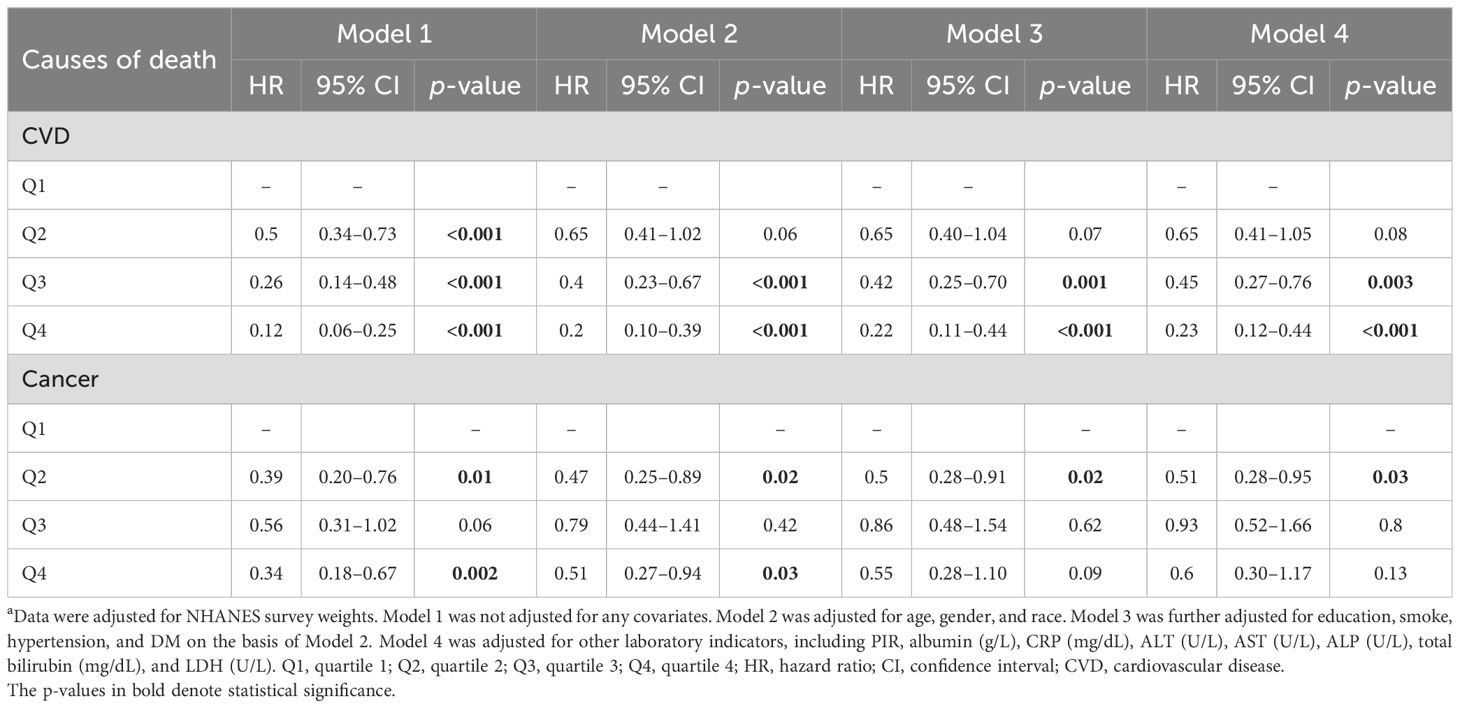
Table 4. The association of LTL with cause-specific mortality a.
In terms of cancer-related mortality, Q2 (HR = 0.39 [0.20–0.76], p = 0.013) and Q4 (HR = 0.34 [0.18–0.67], p = 0.002) had decreased HRs compared to the Q1 group in Model 1. For Model 2, the cancer-caused death decreased in the Q2 group (HR = 0.47 [0.25–0.89], p = 0.02) and the Q4 group (HR = 0.51 [0.27–0.94], p = 0.03), but not in the Q3 group (HR = 0.79 [0.44–1.40], p = 0.42). The negative correlation between LTL and mortality was still evident in the Q2 group according to Model 3 (HR = 0.5 [0.28–0.91], p = 0.02) or Model 4 (HR = 0.51 [0.28–0.95], p = 0.03).
When LTL was considered as a continuous factor, longer LTL was a protective factor from CVD mortality in the four models, while cancer-caused mortality declined with extended LTL only in Model 1 (see Supplementary Table 2). Their Kaplan–Meier survival curves are shown in Figures 2B, C (p values were < 0.001 for CVD death and 0.021 for cancer-caused death).
3.4 The correlation between LTL and mortality in various subgroups
Stratified analysis showed that longer LTL decreased all-cause mortality regardless of smoking status, hypertension, or DM (all p for trend < 0.05) among patients with ALD. As shown in Table 5, LTL was a positive factor for survival especially for elder patients (p for trend < 0.001) or men (p for trend = 0.001).
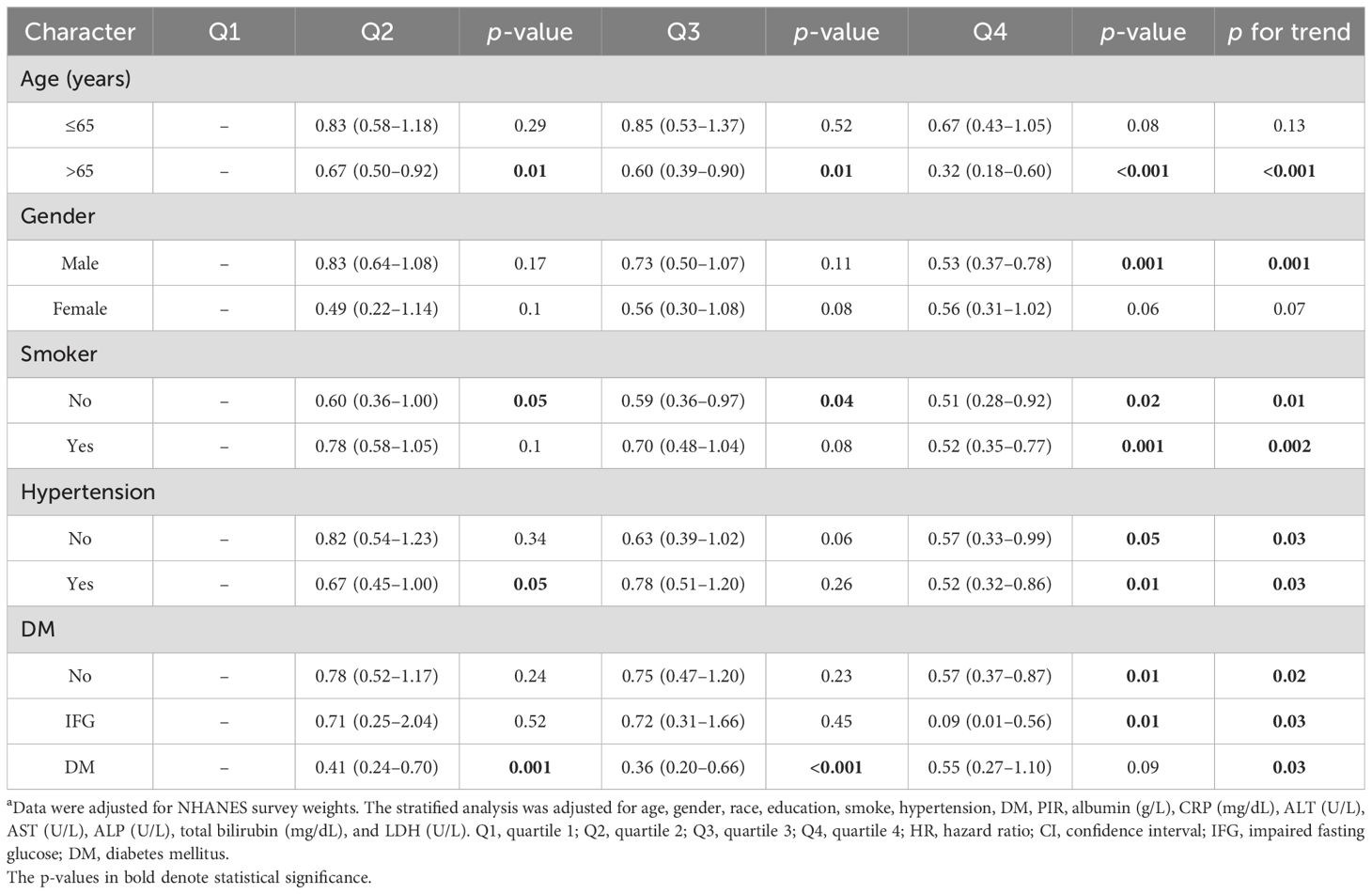
Table 5. The association between dietary iron intake and all-cause mortality in different subgroups a.
3.5 The non-linear relationship between LTL and mortality in ALD patients
The significant non-linear relationship between LTL and all-cause mortality is demonstrated in Figure 3A. The curve appeared L-shaped (p for non-linearity = 0.02). All-cause mortality decreased sharply when LTL increased to 1.36 and tended to flatten subsequently. LTL did not exhibit a non-linear correlation with CVD mortality (p for non-linearity = 0.95) (Figure 3B). When it came to cancer-related mortality, the L-shaped curve was also found to have a tendency to decrease mortality and LTL (p for non-linearity < 0.001) (Figure 3C).
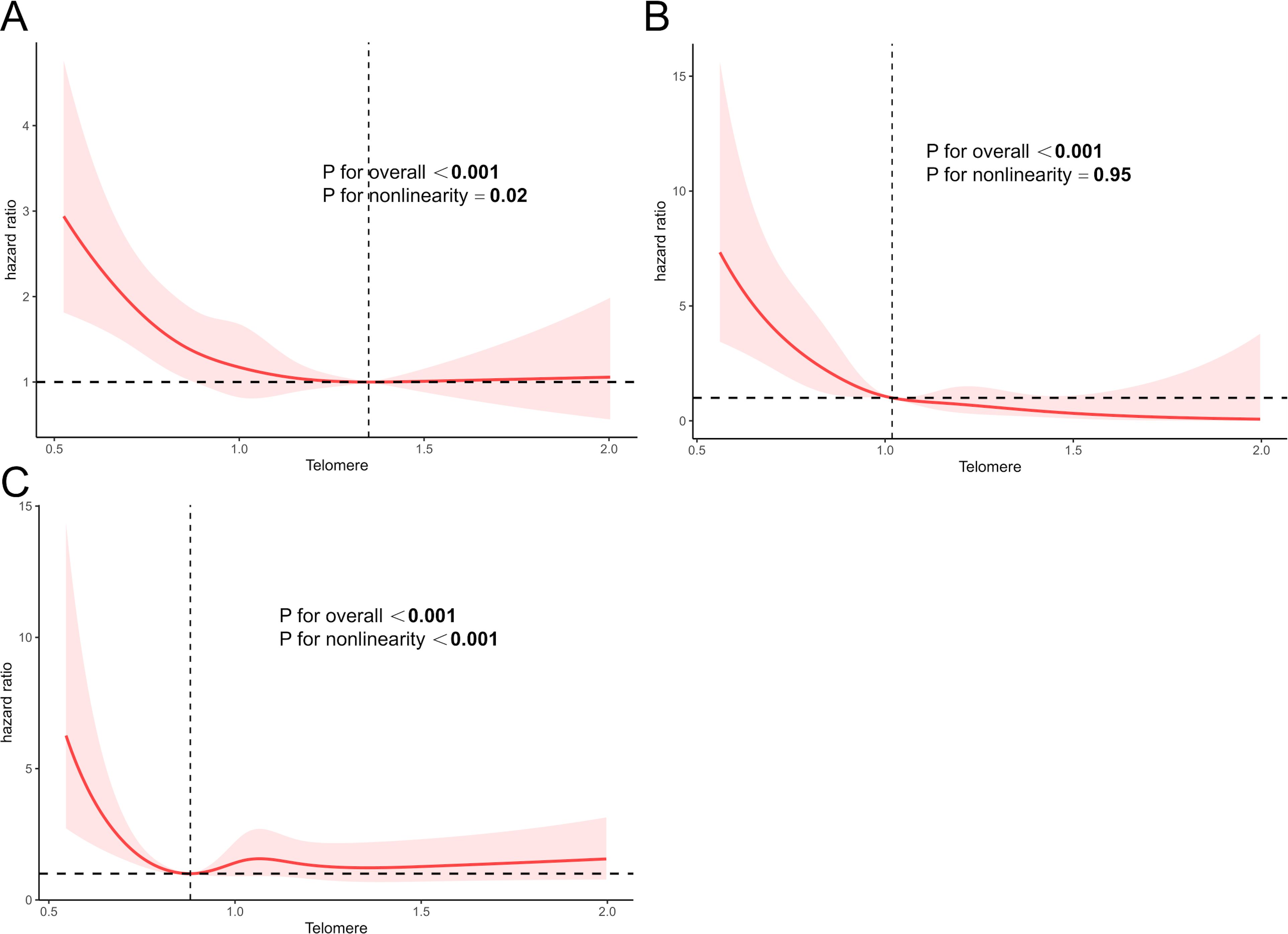
Figure 3. The RCS curve between LTL with all-cause mortality (A), CVD mortality (B), and cancer-caused mortality (C) in participants with ALD. Q1, quartile 1; Q2, quartile 2; Q3, quartile 3; Q4, quartile 4; CVD, cardiovascular disease. The RCS models were adjusted for age, gender, race, education, smoke, hypertension, DM, and other laboratory indicators, such as PIR, albumin (g/L), CRP (mg/dL), ALT (U/L), AST (U/L), ALP (U/L), total bilirubin (mg/dL), and LDH (U/L).
3.6 Sensitivity analyses
Sensitivity analyses were conducted to evaluate the robustness of our findings. The findings remained robust after excluding individuals who died within 1 year (Table 6) or 2 years of follow-up (Supplementary Table 3), excluding individuals with cancer (Supplementary Table 4), or not adjusting for NHANES survey weights (Supplementary Table 5). All-cause mortality and CVD-related mortality still declined with higher levels of LTL. Though there was no statistical significance for cancer-caused death in some sensitivity analyses, HR still declined in higher levels of LTL compared with the lowest group.
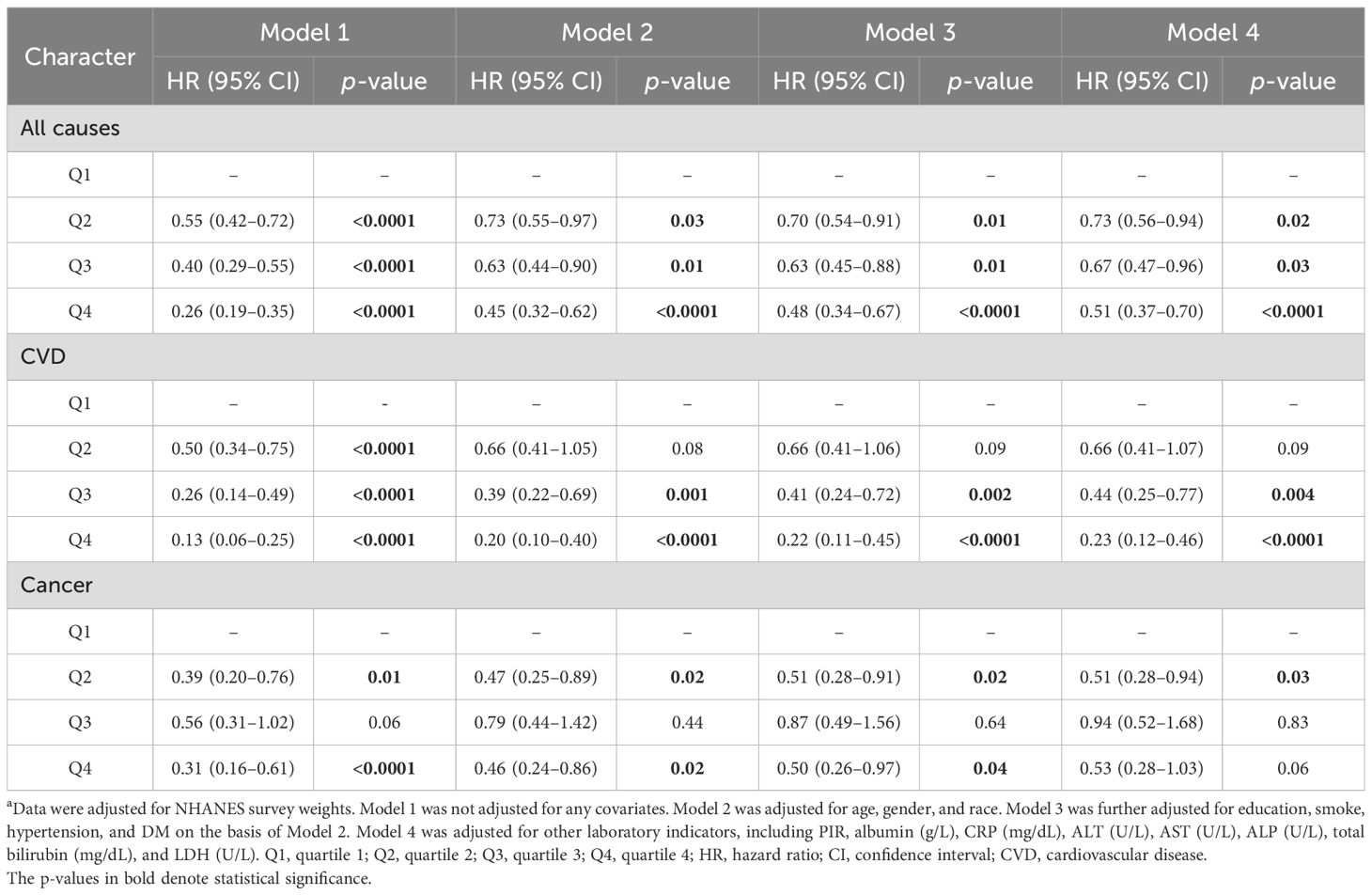
Table 6. The association between LTL and mortality after excluding individuals who died within 1-year follow-up a.
4 Discussion
LTL was considered as a prognostic factor in many metabolic diseases (30, 31). Aging played a key role in the course of liver fibrosis, and alcohol abuse was a major factor for liver disease progression (32). The condition of the livers of ALD patients was associated with the shortening of telomere length (33). The shrinkage of telomere contributed to cellular senescence and was recognized as a biomarker of hepatic cirrhosis separate from the kind of liver diseases including ALD (34). Furthermore, some lines of evidence supported the correlation of shortened telomeres with many liver diseases. Telomere length decreased gradually as the liver tissue deteriorated (35). Moreover, the deficiency of telomerase in liver fibrosis mouse models demonstrated a higher level of cirrhosis (36). Our research showed a negative relationship between LTL and all-cause death among ALD patients, which was consistent with a previous study (22). However, we further focused on the comprehensive impact of LTL on prognosis, including all-cause mortality, CVD, and cancer-related mortalities, and we took more covariates into consideration in prognostic models, including hematological indicators in particular. Additionally, we described the non-linear relationship between LTL and all-cause mortality as well as cancer-caused death. To the best of our knowledge, this is the first research to demonstrate L-shaped correlations between LTL and all-cause mortality. Our results indicated that LTL played an important role during the progression of liver disease, and that LTL shortening was a potential marker of abnormal liver function and worse prognosis. The corresponding comprehensive strategies should be adopted to enhance liver function according to the levels of LTL in patients with ALD so as to improve their quality of life and survival. Further studies should be performed to have a better insight into the role played by LTL in predicting mortality among ALD patients.
As was known, the abnormality of telomerase had an influence on the telomere length in various metabolic disorders, including DM, CVD, and NAFLD (14, 37, 38). Diminished LTL caused a higher risk of CVD and early death (39, 40). Shortening LTL might aggravate oxidative stress or destroy the inflammation system (41, 42). Subsequently, excess immune reaction and redundant oxidative stress were regarded as latent influencing factors for CVD (43, 44). Approximately 30% of ALD patients died from CVD in our research and longer LTL prevented patients from dying from CVD. LTL might work as a crucial predictor of cardiovascular prognosis for individuals with ALD. Moreover, relevant interventions are needed to adjust telomere length to improve patient survival. On the other hand, approximately one-third of hepatocellular cancer cases worldwide were associated with alcohol according to statistics (45). LTL shortening activated p53, which was pivotal to cell apoptosis and proliferation, and the mutation of p53 was common during carcinogenesis (46). We found that cancer-caused mortality had a higher tendency to decrease LTL. Optimized treatment strategies are needed to prove the advantage of LTL as a latent therapeutic method for ALD. More investigations are required to validate cancer-related deaths among people with ALD. In general, clinicians should examine heart function and screen tumors promptly to prevent CVD- or cancer-related death in ALD patients with shorter LTL.
Generally, women live longer than men, and gender plays various roles during the aging process (47, 48). In this study, we found that longer TLT decreased all-cause mortality especially for patients older than 65 years or male patients, which implied that sex hormones might affect the correlation between LTL and ALD. Aging was recognized as a risk factor for ALD, similar to other chronic liver diseases (49). However, there were few studies about gender differences in the aging progression. It was reported that estrogen activated telomerase (50). Men exhibited shorter life expectancies and more rapid telomere shortening. Additionally, sex-specific LTL reduction may have been a contributing factor (51, 52). Larger clinical studies are needed to confirm the role of LTL in improving survival in different genders. In addition, more laboratory experiments are needed to explore the specific mechanisms of sex hormones, LTL, and patient survival.
The increased expression of telomere binding protein possibly induced telomere shortening in patients with alcohol-associated cirrhosis (33). As a result, either the mutation of telomere-associated genes or other harmful factors might destroy the normal function of maintaining an active regenerative ability to avoid injury and fibrosis progression in the liver tissue (53, 54). Research on the influence of telomeres in ALD remained unclear, although their potential role during the ALD process was interesting. There were several opinions regarding the mechanisms about the association between LTL and liver diseases. First of all, telomerase reverse transcriptase (TERT) was an important gene for telomerase (55). Telomere shortening accelerated the devastation of liver tissue and was related to chronic inflammation. Vice versa, chronic inflammation destroyed the function of telomeres, and damaged telomeres further promoted tissue inflammation through secreting cytokine contained interleukin or TNF-α (56). The mutation of telomere-related gene (TRG) mutations significantly increased the risk of developing liver disease (57). The mutations of telomerase RNA component (TERC) sabotaged their enzymatic activity and reduced telomere length among patients with cirrhosis caused by NAFLF, ALD, or HCV infection (54). Secondly, the shortening of telomere enhanced oxidative stress by facilitating the generation of ROS and caused illnesses (58). Thirdly, shorter telomeres resulted in the dysfunction of stem cells and undermined the capacity to self-replicate, which caused tissue destruction and lowered the survival rate (59–61). More studies are needed to find the probable mechanism or molecular pathways on how alcohol influences telomere length and how telomere shortening impacts the progression of ALD.
Our study demonstrated the relationship between LTL and mortality from different aspects in patients with ALD on the basis of a large sample from NHANES 1999–2002. The robustness of our findings was confirmed by sensitivity analyses. Inevitably, there were still some limitations. First of all, we did not obtain the causation and sequence of influence between LTT and survival because of the cross-sectional and retrospective design of the study. Secondly, there might be other unknown indicators that affected the results of this study. Finally, we did not analyze how LTL affects survival in ALD due to the scarcity of more factors of liver function and the history of drug use, surgery, or liver transplantation. Further clinical investigation is warranted to substantiate this perspective. Overall, better prognosis related to LTL was a complicated field of research including various molecular mechanisms. More data and further exploration are required to evaluate a more detailed relationship between them.
5 Conclusion
In conclusion, our research found that LTL was an effective predictive factor for survival among patients with ALD, especially for patients >65 years or male patients. Furthermore, longer leukocyte telomere and all-cause mortality as well as cancer-related mortality had an L-shaped non-linear relationship. The causal link between LTL and survival needs to be validated by future studies to help provide effective strategies for high-risk ALD patients to prolong their life.
Data availability statement
Publicly available datasets were analyzed in this study. This data can be found here: https://www.cdc.gov/nchs/nhanes/index.htm.
Ethics statement
The National Center for Health Statistics Research Ethics Review Board (NCHS ERB) approved, censored and ratified the program. The studies were conducted in accordance with the local legislation and institutional requirements. Written informed consent for participation in this study was provided by the participants’ legal guardians/next of kin.
Author contributions
JY: Writing – original draft, Writing – review & editing, Conceptualization, Data curation, Methodology. HG: Conceptualization, Investigation, Writing – original draft. CJ: Investigation, Software, Writing – original draft. JD: Data curation, Software, Writing – review & editing. JX: Methodology, Visualization, Writing – review & editing. YZ: Methodology, Visualization, Writing – review & editing. WH: Validation, Visualization, Writing – review & editing. LX: Project administration, Resources, Writing – review & editing.
Funding
The author(s) declare that no financial support was received for the research, authorship, and/or publication of this article.
Acknowledgments
We would particularly like to acknowledge the staff members of NHANES for their wonderful data support.
Conflict of interest
The authors declare that the research was conducted in the absence of any commercial or financial relationships that could be construed as a potential conflict of interest.
Publisher’s note
All claims expressed in this article are solely those of the authors and do not necessarily represent those of their affiliated organizations, or those of the publisher, the editors and the reviewers. Any product that may be evaluated in this article, or claim that may be made by its manufacturer, is not guaranteed or endorsed by the publisher.
Supplementary material
The Supplementary Material for this article can be found online at: https://www.frontiersin.org/articles/10.3389/fendo.2024.1462591/full#supplementary-material
References
1. Sepanlou S, Safiri C, Bisignano KS, Ikuta S, Merat M, Safiri S, et al. The global, regional, and national burden of cirrhosis by cause in 195 countries and territories, 1990-2017: a systematic analysis for the Global Burden of Disease Study 2017. Lancet Gastroenterol Hepatol. (2020) 5:245–66. doi: 10.1016/S2468-1253(19)30349-8
2. Gao B, Bataller R. Alcoholic liver disease: pathogenesis and new therapeutic targets. Gastroenterology. (2011) 141:1572–85. doi: 10.1053/j.gastro.2011.09.002
3. Singal AK, Mathurin P. Diagnosis and treatment of alcohol-associated liver disease: A review. JAMA. (2021) 326:165–76. doi: 10.1001/jama.2021.7683
4. Lee BP, Vittinghoff E, Dodge JL, Cullaro G, Terrault NA. National trends and long-term outcomes of liver transplant for alcohol-associated liver disease in the United States. JAMA Intern Med. (2019) 179:340–8. doi: 10.1001/jamainternmed.2018.6536
5. Wu X, Fan X, Miyata T, Kim A, Cajigas-Du Ross CK, Ray S, et al. Recent advances in understanding of pathogenesis of alcohol-associated liver disease. Annu Rev Pathol. (2023) 18:411–38. doi: 10.1146/annurev-pathmechdis-031521-030435
6. Simonetto DA, Gines P, Kamath PS. Hepatorenal syndrome: pathophysiology, diagnosis, and management. BMJ. (2020) 370:m2687. doi: 10.1136/bmj.m2687
7. Julien J, Ayer T, Bethea ED, Tapper EB, Chhatwal J. Projected prevalence and mortality associated with alcohol-related liver disease in the USA, 2019-40: a modelling study. Lancet Public Health. (2020) 5:e316–e23. doi: 10.1016/S2468-2667(20)30062-1
8. Rahimi E, Pan J-J. Prognostic models for alcoholic hepatitis. biomark Res. (2015) 3:20. doi: 10.1186/s40364-015-0046-z
9. Altamirano J, López-Pelayo H, Michelena J, Jones PD, Ortega L, Ginès P, et al. Alcohol abstinence in patients surviving an episode of alcoholic hepatitis: Prediction and impact on long-term survival. Hepatology. (2017) 66:1842–53. doi: 10.1002/hep.29338
10. Morales-Arráez D, Ventura-Cots M, Altamirano J, Abraldes JG, Cruz-Lemini M, Thursz MR, et al. The MELD score is superior to the maddrey discriminant function score to predict short-term mortality in alcohol-associated hepatitis: A global study. Am J Gastroenterol. (2022) 117:301–10. doi: 10.14309/ajg.0000000000001704
11. Forrest EH, Fisher NC, Singhal S, Brind A, Haydon G, O’Grady J, et al. Comparison of the Glasgow alcoholic hepatitis score and the ABIC score for the assessment of alcoholic hepatitis. Am J Gastroenterol. (2010) 105:701–2. doi: 10.1038/ajg.2009.645
12. Blackburn EH, Epel ES, Lin J. Human telomere biology: A contributory and interactive factor in aging, disease risks, and protection. Science. (2015) 350:1193–8. doi: 10.1126/science.aab3389
13. Calado RT, Young NS. Telomere diseases. N Engl J Med. (2009) 361:2353–65. doi: 10.1056/NEJMra0903373
14. Shin HK, Park JH, Yu JH, Jin Y-J, Suh YJ, Lee J-W, et al. Association between telomere length and hepatic fibrosis in non-alcoholic fatty liver disease. Sci Rep. (2021) 11:18004. doi: 10.1038/s41598-021-97385-2
15. Schneider CV, Schneider KM, Teumer A, Rudolph KL, Hartmann D, Rader DJ, et al. Association of telomere length with risk of disease and mortality. JAMA Intern Med. (2022) 182:291–300. doi: 10.1001/jamainternmed.2021.7804
16. Liao Q, He J, Tian F-F, Bi F-F, Huang K. A causal relationship between leukocyte telomere length and multiple sclerosis: A Mendelian randomization study. Front Immunol. (2022) 13:922922. doi: 10.3389/fimmu.2022.922922
17. Cortez Cardoso Penha R, Smith-Byrne K, Atkins JR, Haycock PC, Kar S, Codd V, et al. Common genetic variations in telomere length genes and lung cancer: a Mendelian randomisation study and its novel application in lung tumour transcriptome. Elife. (2023) 12:e83118. doi: 10.7554/eLife.83118.sa2
18. Sullivan SM, Cole B, Lane J, Meredith JJ, Langer E, Hooten AJ, et al. Predicted leukocyte telomere length and risk of myeloid neoplasms. Hum Mol Genet. (2023) 32:2996–3005. doi: 10.1093/hmg/ddad126
19. Aung N, Wang Q, van Duijvenboden S, Burns R, Stoma S, Raisi-Estabragh Z, et al. Association of longer leukocyte telomere length with cardiac size, function, and heart failure. JAMA Cardiol. (2023) 8:808–15. doi: 10.1001/jamacardio.2023.2167
20. Tang L, Li D, Ma Y, Cui F, Wang J, Tian Y. The association between telomere length and non-alcoholic fatty liver disease: a prospective study. BMC Med. (2023) 21:427. doi: 10.1186/s12916-023-03136-7
21. Zhu S, Yang M, Wang T, Ding Z. Causal relationships between telomere length and liver disease: a Mendelian randomization study. Front Genet. (2023) 14:1164024. doi: 10.3389/fgene.2023.1164024
22. Rattan P, Penrice DD, Ahn JC, Ferrer A, Patnaik M, Shah VH, et al. Inverse association of telomere length with liver disease and mortality in the US population. Hepatol Commun. (2022) 6:399–410. doi: 10.1002/hep4.1803
23. Rode L, Nordestgaard BG, Bojesen SE. Peripheral blood leukocyte telomere length and mortality among 64,637 individuals from the general population. J Natl Cancer Inst. (2015) 107:djv074. doi: 10.1093/jnci/djv074
24. Needham BL, Rehkopf D, Adler N, Gregorich S, Lin J, Blackburn EH, et al. Leukocyte telomere length and mortality in the National Health and Nutrition Examination Survey, 1999-2002. Epidemiology. (2015) 26:528–35. doi: 10.1097/EDE.0000000000000299
25. Duckworth A, Gibbons MA, Allen RJ, Almond H, Beaumont RN, Wood AR, et al. Telomere length and risk of idiopathic pulmonary fibrosis and chronic obstructive pulmonary disease: a mendelian randomisation study. Lancet Respir Med. (2021) 9:285–94. doi: 10.1016/S2213-2600(20)30364-7
26. Cheng F, Carroll L, Joglekar MV, Januszewski AS, Wong KK, Hardikar AA, et al. Diabetes, metabolic disease, and telomere length. Lancet Diabetes Endocrinol. (2021) 9:117–26. doi: 10.1016/S2213-8587(20)30365-X
27. Kessler MD, Damask A, O’Keeffe S, Banerjee N, Li D, Watanabe K, et al. Common and rare variant associations with clonal haematopoiesis phenotypes. Nature. (2022) 612:301–9. doi: 10.1038/s41586-022-05448-9
28. Spyridopoulos I, von Zglinicki T. Telomere length predicts cardiovascular disease. BMJ. (2014) 349:g4373. doi: 10.1136/bmj.g4373
29. Dang K, Hirode G, Singal AK, Sundaram V, Wong RJ. Alcoholic liver disease epidemiology in the United States: A retrospective analysis of 3 US databases. Am J Gastroenterol. (2020) 115:96–104. doi: 10.14309/ajg.0000000000000380
30. Xiong L, Yang G, Guo T, Zeng Z, Liao T, Li Y, et al. 17-year follow-up of association between telomere length and all-cause mortality, cardiovascular mortality in individuals with metabolic syndrome: results from the NHANES database prospective cohort study. Diabetol Metab Syndr. (2023) 15:247. doi: 10.1186/s13098-023-01206-7
31. Chen Z, Shen Y, He J, Shen Y, Zhu W, Wu X, et al. Longer leukocyte telomere length increases cardiovascular mortality in type 2 diabetes patients. J Diabetes. (2023) 15:325–31. doi: 10.1111/1753-0407.13376
32. Poynard T, Mathurin P, Lai C-L, Guyader D, Poupon R, Tainturier M-H, et al. A comparison of fibrosis progression in chronic liver diseases. J Hepatol. (2003) 38:257–65. doi: 10.1016/S0168-8278(02)00413-0
33. Huda N, Kusumanchi P, Perez K, Jiang Y, Skill NJ, Sun Z, et al. Telomere length in patients with alcohol-associated liver disease: a brief report. J Investig Med. (2022) 70:1438–41. doi: 10.1136/jim-2021-002213
34. Wiemann SU, Satyanarayana A, Tsahuridu M, Tillmann HL, Zender L, Klempnauer J, et al. Hepatocyte telomere shortening and senescence are general markers of human liver cirrhosis. FASEB J. (2002) 16:935–42. doi: 10.1096/fj.01-0977com
35. Kitada T, Seki S, Kawakita N, Kuroki T, Monna T. Telomere shortening in chronic liver diseases. Biochem Biophys Res Commun. (1995) 211:33–9. doi: 10.1006/bbrc.1995.1774
36. Rudolph KL, Chang S, Millard M, Schreiber-Agus N, DePinho RA. Inhibition of experimental liver cirrhosis in mice by telomerase gene delivery. Science. (2000) 287:1253–8. doi: 10.1126/science.287.5456.1253
37. Mangge H, Herrmann M, Almer G, Zelzer S, Moeller R, Horejsi R, et al. Telomere shortening associates with elevated insulin and nuchal fat accumulation. Sci Rep. (2020) 10:6863. doi: 10.1038/s41598-020-63916-6
38. Kang SH, Cho Y, Jeong SW, Kim SU, Lee J-W. From nonalcoholic fatty liver disease to metabolic-associated fatty liver disease: Big wave or ripple? Clin Mol Hepatol. (2021) 27:257–69. doi: 10.3350/cmh.2021.0067
39. Vecoli C, Borghini A, Pulignani S, Mercuri A, Turchi S, Picano E, et al. Independent and combined effects of telomere shortening and mtDNA4977 deletion on long-term outcomes of patients with coronary artery disease. Int J Mol Sci. (2019) 20(21):5508. doi: 10.3390/ijms20215508
40. Fu H, Zhu Y, Lin L, Jiang P, Cai G, Zeng L, et al. Shorter leukocyte telomere length is associated with increased major adverse cardiovascular events or mortality in patients with essential hypertension. J Cardiovasc Transl Res. (2024). doi: 10.1007/s12265-024-10558-y
41. Masi S, Gkranias N, Li K, Salpea KD, Parkar M, Orlandi M, et al. Association between short leukocyte telomere length, endotoxemia, and severe periodontitis in people with diabetes: a cross-sectional survey. Diabetes Care. (2014) 37:1140–7. doi: 10.2337/dc13-2106
42. Martínez P, Blasco MA. Heart-breaking telomeres. Circ Res. (2018) 123:787–802. doi: 10.1161/CIRCRESAHA.118.312202
43. Senoner T, Dichtl W. Oxidative stress in cardiovascular diseases: still a therapeutic target? Nutrients. (2019) 11(9):2090. doi: 10.3390/nu11092090
44. Gavia-García G, Rosado-Pérez J, Arista-Ugalde TL, Aguiñiga-Sánchez I, Santiago-Osorio E, Mendoza-Núñez VM. Telomere length and oxidative stress and its relation with metabolic syndrome components in the aging. Biol (Basel). (2021) 10(4):253. doi: 10.3390/biology10040253
45. Zeng RW, Ong CEY, Ong EYH, Chung CH, Lim WH, Xiao J, et al. Global prevalence, clinical characteristics, surveillance, treatment allocation, and outcomes of alcohol-associated hepatocellular carcinoma. Clin Gastroenterol Hepatol. (2024) 22(12):2394–2402.e15.
46. Mishima M, Takai A, Takeda H, Iguchi E, Nakano S, Fujii Y, et al. TERT upregulation promotes cell proliferation via degradation of p21 and increases carcinogenic potential. J Pathol. (2024) 264:318–31. doi: 10.1002/path.v264.3
47. Huang Y, Li H, Liang R, Chen J, Tang Q. The influence of sex-specific factors on biological transformations and health outcomes in aging processes. Biogerontology. (2024) 25:775–91. doi: 10.1007/s10522-024-10121-x
48. Barrett ELB, Richardson DS. Sex differences in telomeres and lifespan. Aging Cell. (2011) 10:913–21. doi: 10.1111/j.1474-9726.2011.00741.x
49. Forrest EH, Evans CDJ, Stewart S, Phillips M, Oo YH, McAvoy NC, et al. Analysis of factors predictive of mortality in alcoholic hepatitis and derivation and validation of the Glasgow alcoholic hepatitis score. Gut. (2005) 54:1174–9. doi: 10.1136/gut.2004.050781
50. Kyo S, Takakura M, Kanaya T, Zhuo W, Fujimoto K, Nishio Y, et al. Estrogen activates telomerase. Cancer Res. (1999) 59:5917–21.
51. Bekaert S, De Meyer T, Rietzschel ER, De Buyzere ML, De Bacquer D, Langlois M, et al. Telomere length and cardiovascular risk factors in a middle-aged population free of overt cardiovascular disease. Aging Cell. (2007) 6:639–47. doi: 10.1111/j.1474-9726.2007.00321.x
52. Chen W, Kimura M, Kim S, Cao X, Srinivasan SR, Berenson GS, et al. Longitudinal versus cross-sectional evaluations of leukocyte telomere length dynamics: age-dependent telomere shortening is the rule. J Gerontol A Biol Sci Med Sci. (2011) 66:312–9. doi: 10.1093/gerona/glq223
53. Calado RT, Brudno J, Mehta P, Kovacs JJ, Wu C, Zago MA, et al. Constitutional telomerase mutations are genetic risk factors for cirrhosis. Hepatology. (2011) 53:1600–7. doi: 10.1002/hep.24173
54. Chaiteerakij R, Roberts LR. Telomerase mutation: a genetic risk factor for cirrhosis. Hepatology. (2011) 53:1430–2. doi: 10.1002/hep.24304
55. Totoki Y, Tatsuno K, Covington KR, Ueda H, Creighton CJ, Kato M, et al. Trans-ancestry mutational landscape of hepatocellular carcinoma genomes. Nat Genet. (2014) 46:1267–73. doi: 10.1038/ng.3126
56. Birch J, Victorelli S, Rahmatika D, Anderson RK, Jiwa K, Moisey E, et al. Telomere dysfunction and senescence-associated pathways in bronchiectasis. Am J Respir Crit Care Med. (2016) 193:929–32. doi: 10.1164/rccm.201510-2035LE
57. Sidali S, Borie R, Sicre de Fontbrune F, El Husseini K, Rautou P-E, Lainey E, et al. Liver disease in germline mutations of telomere-related genes: Prevalence, clinical, radiological, pathological features, outcome, and risk factors. Hepatology. (2024) 79:1365–80. doi: 10.1097/HEP.0000000000000667
58. Sahin E, Colla S, Liesa M, Moslehi J, Müller FL, Guo M, et al. Telomere dysfunction induces metabolic and mitochondrial compromise. Nature. (2011) 470:359–65. doi: 10.1038/nature09787
59. Marion RM, Strati K, Li H, Tejera A, Schoeftner S, Ortega S, et al. Telomeres acquire embryonic stem cell characteristics in induced pluripotent stem cells. Cell Stem Cell. (2009) 4:141–54. doi: 10.1016/j.stem.2008.12.010
60. Flores I, Canela A, Vera E, Tejera A, Cotsarelis G, Blasco MA. The longest telomeres: a general signature of adult stem cell compartments. Genes Dev. (2008) 22:654–67. doi: 10.1101/gad.451008
Keywords: alcohol-associated liver disease, all-cause mortality, leukocyte telomere length, NHANES, prognosis
Citation: Yi J, Guo H, Jiang C, Duan J, Xue J, Zhao Y, He W and Xia L (2024) Leukocyte telomere length decreased the risk of mortality in patients with alcohol-associated liver disease. Front. Endocrinol. 15:1462591. doi: 10.3389/fendo.2024.1462591
Received: 10 July 2024; Accepted: 12 November 2024;
Published: 12 December 2024.
Edited by:
Francesco Prattichizzo, MultiMedica Holding SpA (IRCCS), ItalyReviewed by:
Alexander E Berezin, Paracelsus Medical University, AustriaShinsuke Hidese, Teikyo University, Japan
Copyright © 2024 Yi, Guo, Jiang, Duan, Xue, Zhao, He and Xia. This is an open-access article distributed under the terms of the Creative Commons Attribution License (CC BY). The use, distribution or reproduction in other forums is permitted, provided the original author(s) and the copyright owner(s) are credited and that the original publication in this journal is cited, in accordance with accepted academic practice. No use, distribution or reproduction is permitted which does not comply with these terms.
*Correspondence: Liangping Xia, eGlhbHBAc3lzdWNjLm9yZy5jbg==