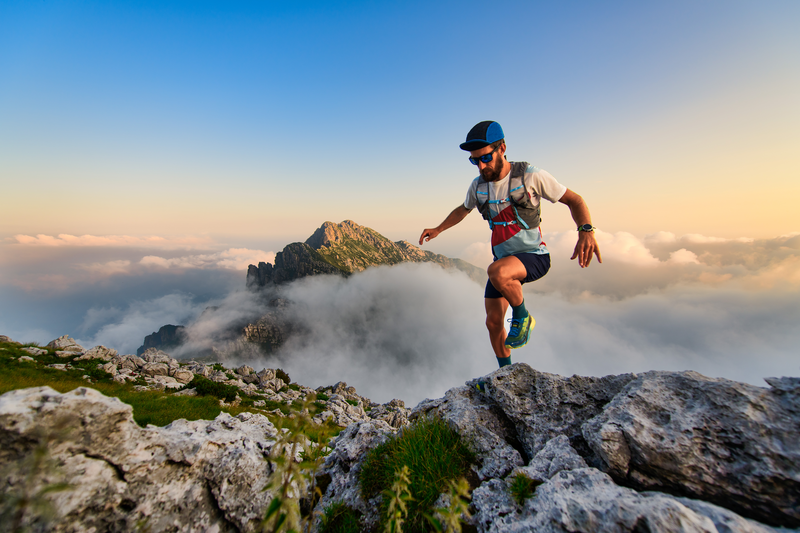
94% of researchers rate our articles as excellent or good
Learn more about the work of our research integrity team to safeguard the quality of each article we publish.
Find out more
REVIEW article
Front. Endocrinol. , 04 October 2024
Sec. Reproduction
Volume 15 - 2024 | https://doi.org/10.3389/fendo.2024.1456305
The reproductive efficiency of livestock is crucial for agricultural productivity and economic sustainability. One critical factor in successful fertilization and the viability of offspring is the quality of semen. Poor semen quality, especially in frozen-thawed semen used in artificial insemination (AI) have been shown to influence conception outcomes, resulting a negative impact on livestock production. Recent advancements in genetic research have identified specific markers linked to semen quality traits in various livestock species, such as cattle, sheep, goats, pigs, buffalo, and equines. These genetic markers are essential in screening males for breeding suitability, which in turn enhances selective breeding programs. Understanding these markers is crucial for improving reproductive performance and increasing productivity in livestock populations. This review offers a comprehensive overview of the genetic markers associated with semen quality in key livestock. It explores the underlying genetic mechanisms and their practical implications in animal breeding and management. The review underscores the importance of integrating genetic insights into breeding strategies to optimize reproductive efficiency and ensure the sustainable development of livestock industries.
The reproductive efficiency of livestock is a vital factor that significantly impacts agricultural productivity and economic sustainability. Semen quality is particularly crucial in determining successful fertilization and the resulting offspring’s outcomes (1, 2). Sperm disorders in frozen–thawed semen, widely used in artificial insemination (AI) technology, confer a risk of impaired fertility in livestock. In addition, poor quality may lead to male infertility (3, 4). The quality of semen plays a crucial role in the reproductive success of livestock by directly impacting fertility rates, genetic diversity, and overall herd productivity. The efficiency of breeding programs and the profitability of livestock farming depend on the ability to produce offspring with desirable traits.
The assessment of semen quality traditionally relies on conventional methods, which evaluate sperm motility, morphology, concentration, and ejaculate volume. Over time, there have been significant improvements in this assessment, allowing for a more comprehensive evaluation of sperm quality and fertility parameters (5). However, these methods do not consider the molecular characteristics of sperm cells, such as DNA integrity, oxidative status, or the presence of essential sperm proteins (6). This limitation can hinder the identification of molecular causes of subfertility. Several tests have been developed to predict semen quality, but a single, highly reliable test is not yet available (7, 8). Genetic markers provide valuable information about the genetic factors that determine semen quality (9, 10). They enable accurate predictions and early detection of problems before physical symptoms appear. These markers are crucial for monitoring how semen quality traits are inherited across generations, making them extremely valuable for breeding programs. By using genetic markers, we can make better choices when selecting individuals with the best traits (8, 11, 12). This also helps us to understand the genetic basis of semen quality and reduces the impact of environmental factors. As a result, the assessment of semen quality becomes more stable and reliable compared to traditional methods that rely solely on physical characteristics (9, 13).
Recent advancements in genetic research have enabled the identification of specific genetic markers associated with semen quality traits in various domestic livestock species (14–16), including sheep (17), goats (18), pigs (19), cattle (20), buffalo (21) and equines (22). In addition, these genetic markers also play a key role in screening the suitability of male for breeding purposes (23). Thus, understanding these genetic markers is essential for enhancing selective breeding programs, improving reproductive performance, and ultimately increasing the productivity of livestock populations. This review study aims to provide a comprehensive review of the genetic markers linked to semen quality in livestock, exploring the underlying genetic mechanisms and their practical implications in animal breeding and management.
This review provides a comprehensive overview of the genes associated with semen quality traits across various livestock species, including buffalo, cattle, equine, pigs, sheep, and goats. The majority of the data considered in this review were derived from publications spanning from 2010 to 2024, with an additional inclusion of data from ten articles published between 2003 and 2010. The literature search was conducted using databases such as Google Scholar, X-MOL, PubMed, and Scopus. Only articles published in English and indexed in SCI journals were considered. The search employed keywords such as ‘pigs,’ ‘cattle,’ ‘buffalo,’ ‘horses,’ ‘sheep,’ ‘goats,’ ‘semen quality traits,’ and ‘genetic markers associated with semen quality traits. Notably, data from book chapters, conference proceedings, and letters to the editor were excluded from this review. Finally, the DAVID online tool (https://david.ncifcrf.gov/tools.jsp) was utilized to identify the biological processes and signaling pathways of genes associated with semen quality traits in livestock.
Various approaches, such as genome-wide association studies (GWAS), transcriptomic analysis, and the candidate gene method, have been utilized to screen genes associated with semen quality traits in cattle bulls (24, 25). GWAS analysis involves evaluating genomes from multiple phenotypes to identify genetic markers that can predict the presence of a trait. Once these markers are identified, they can be used to understand how genes contribute to the traits. For example, a study found that DYRK1A, TEC, and TXK were associated with sperm motility based on GWAS analysis (10). Another study documented the association of GALNTL6, HMGB2, ADAM29, PRMT6, SCAPER, EDC3, and LIN28B with spermatogenesis, ejaculate volume, sperm concentration, and sperm motility (26). Similarly, GWAS analysis has led to the discovery of several genes associated with spermatogenesis, total sperm motility, and progressive sperm motility in Italian Holstein bulls (27), as shown in Table 1.
Recent studies have documented the association of PRM1, STK35, and IFT27 (28), FOXO4, FOXP3, GATA1, CYP27B1, EBP, KDM5C, LRRK2, and PME (29), and MARCH1 (14) with semen quality traits and bull fertility. Another study reported the upregulation of SPADH2, TIMP-2, PLA2G7, OAZ3, GPx4, and GSTM3 in bulls with reduced sperm motility and fertility (30). In contrast, the levels of caltrin and ADM were low in bulls with high ejaculate rejection rates, indicating a strong link between these proteins and sperm motility (30). Furthermore, FBXO39 was found to be differentially expressed in sperm cells and seminal plasma, showing a strong correlation with sperm motility in bulls (20). Recently, research compared the genetic marker profiles of seminal plasma from breeding bulls producing good and poor-quality semen (31). Consistently, a study conducted proteomic analysis of seminal plasma and found that CCL2, UQCRC2, and SAA1 were upregulated in the seminal plasma of poor-quality semen and negatively associated with sperm functions (32). Furthermore, NGF, EEF1A2, COL1A2, IZUMO4, PRSS1, COL1A1, WFDC2, COL1A1, COL2A1, COL1A2, SPP1, and PDGFA were found to have a positive effect on sperm function and were downregulated in the seminal plasma of poor-quality semen.
Interestingly, the association of TPT1, BOLA-DRA, CD74, RPS17, RPS28, RPS29, RPL14, RPL13, and RPS27A with sperm functionality, survival, oxidative stress, and bull fertility has been discovered (33). Furthermore, it has been revealed that POU4F2, GRIK1, NEDD4, FOXF1, RAD51B, WNT4, WNT5A, RIMS1 and PPP3CA were key genes associated with sperm head and tail disorders in cryopreserved semen of bulls (34). Consistently, another study found that polymorphisms in FSHR, INHA, INHAB, TNP2, and SPEF2 genes were significantly correlated with doublet ejaculate volume, sperm concentration, progressive motility, and total number of spermatozoa in bulls (35). These genes were found to be associated with sperm structural integrity, cellular communication, and DNA repair, all of which are important for spermatogenesis and sperm function.
Improving the quality of buffalo semen is a crucial focus in the fields of livestock genetics and reproductive biotechnology. Recent advancements have identified genetic markers in buffalo bulls that show promise in enhancing semen quality. For instance, one study found that variations in the Leptin gene are linked to higher progressive motility (PR), increased sperm concentration, total sperm count, and elevated levels of LH and testosterone hormones (81). Another research study identified significant associations between the expressions of MAPK3, RPL36AL, EXT2, RPS27A, RPS18, and RPS28 with progressive motility, acrosome integrity, functional membrane integrity, and overall fertility rate (82). Similarly, GWAS analysis discovered several genes, including TEKT2, SPEM1, PRM3, EQTN, PLCZ1, SPESP1, SPACA1, TNP1, and YBX2, that may influence sperm motility as well as the structural and functional membrane integrities of sperm (83). Furthermore, the association between RPL10, ZCCHC13, AKAP4, TSPAN6, RPL10, and RPS4X and sperm motility has been well established (84). Another study highlighted a positive correlation between GnRHR and percentages of sperm motility, sperm concentration, and live sperm count (85). Higher expression levels of PDZD8, GTF2F2, ZNF397, KIZ, LOH12CR1, ACRBP, PRSS37, CYP11B2, F13A1 and SPO11 were found in high-fertile spermatozoa, whereas overexpression of MT1A, ATP5F1, CS, TCRB, PRODH2, HARS, IDH3A, SRPK3, TUBB2B, GPR4, PMP2, CTSL1, TPPP2 and EGFL6 were reported in low-fertile spermatozoa (86). For easy reference, we have summarized the research progress on genes associated with semen quality traits in buffalo bulls in Table 2.
Fertility is essential for the overall reproductive success of sheep and goats, playing a vital role in the small ruminant industry. Similar to other livestock, semen quality is also crucial in sheep and goats for successful conception. Significant research has been conducted on the screening of genes and their association with semen quality traits in bucks and rams, including volume, gross motility, concentration, percent post-thaw motility, number of spermatozoa, and sperm abnormalities (100, 101). Previous GWAS studies consistently identified several candidate genes related to semen quality traits in sheep (102, 103) and goats (104). In order to improve clarity and facilitate understanding, we have provided a summary of studies on genes associated with semen quality traits in Table 3.
The use of AI in swine production allows for the selection of boars based on their desirable production traits. However, AI heightens the importance of each boar’s reproductive performance, necessitating the evaluation of semen samples for their fertilization potential at boar stations (117). The pig industry aims to maximize the number of insemination doses produced from each boar ejaculate, which requires boars to produce high-quality semen characterized by high motility, progressive motility, and low levels of morphological defects, in large quantities (a high number of sperm cells per ejaculate) (118).
Spermatogenesis and fertilization are complex processes regulated by numerous genes. For instance, ACTN1 and ACTG2 significantly impact semen volume per ejaculate and sperm motility (117). Lin et al. identified several candidate genes, including gonadotropin-releasing hormone receptor (GNRHR), prolactin (PRL), prolactin receptor (PRLR), follicle-stimulating hormone beta (FSHB), luteinizing hormone beta (LHB), follistatin (FST), inhibin alpha (INHA), inhibin beta A (INHBA), retinol-binding protein 4 (RBP4), androgen receptor (AR), relaxin (RLN), acrosin (ACR), osteopontin (OPN), and β-actin (ACTB) that were associated with sperm quality traits such as sperm concentration, motility, semen volume per ejaculate, plasma droplets rate, and abnormal sperm rate (119–121). Further studies have highlighted the roles of phospholipase C zeta (PLCz), cyclooxygenase isoenzyme type 2 (COX-2) (122), and cluster-of-differentiation antigen 9 (CD9) (123), along with estrogen receptor 1 (ESR1) and ESR2, in spermatogenesis and semen quality traits like sperm concentration, motility, semen volume, plasma droplet rate, and abnormal spermatozoa rate (124, 125). TEX14 has been associated with spermatogenic arrest and subsequent infertility in boars (126). Similarly, TK17b and HECW2 are linked to severe defects in sperm acrosome and chromatin, causing infertility (127). Genes such as EGF, PTGS2, and PRLR have been positively correlated with semen volume per ejaculate, sperm motility, percentage of normal sperm, percentage of sperm with proximal plasma droplets, and total sperm count per ejaculate (128). The DAZL gene has been associated with lower sperm motility and concentration in boars (129). Polymorphisms in genes like CD9 (g.358A>T), ESR1 (g.35756T>C), and PLCz (g.158T>C) have been linked to sperm motility (130–132). Additionally, RAMP2 and GIMAP6 were identified through RNA-seq analysis and found to be associated with sperm DNA fragmentation in boars (133). Similarly, another study revealed through RNA-analysis that genes such as FOS, NFATC3, EAF2, BAMBI, PTPRU, PTPN2, ND6, ACADM, and FGF-14 were associated with spermatogenesis, energy metabolism and poor semen freezability (134).
Genome-wide association studies (GWAS) have been used to identify genetic markers associated with semen quality traits in boars. A study reported the link of mitochondrial methionyl-tRNA formyltransferase (MTFMT) is associated with sperm motility (135). Similarly, another research identified PLA2G4A, PTGS2, and HPGDS as markers associated with motility, progressive motility, the number of sperm cells per ejaculate, and total morphological defects, all using GWAS (136). Accordingly, the association of PRMT6, Sox5, PEX10, SIRPA, and SIRPG with oligozoospermia in Han Chinese Population has been explored (137). Furthermore, a GWAS analysis revealed several key genes and their association with semen quality traits and spermiogenesis including TDRD5, QSOX1, BLK, TIMP3, THRA, CSF3, and ZPBP1 with number of sperm cells, PPP2R2B, NEK2, NDRG, ADAM7, SKP2, and RNASET2 with sperm motility; SH2B1, BLK, LAMB1, VPS4A, SPAG9, LCN2, and DNM1 with sperm progressive motility, GHR, SELENOP, SLC16A5, SLC9A3R1, and DNAI2 with total morphological abnormalities (138). Interestingly, genetic markers have also been identified through GWAS analysis that were associated sperm morphology, deformities and semen qualities (139). Several other genes such CHD2, KATNAL2, SLC14A2, ABCA1, PRM1, OAZ3, DNAJB8, TPPP2, IQCJ, ACTR2, HARS and TNP have been found to be correlated with percentage of head and neck abnormalities, abnormal acrosomes and motile spermatozoa (140). To facilitate understanding, a summary of studies on genes associated with semen quality traits is provided in the accompanying Table 4.
Genetic factors are a major contributor to the wide range of semen quality observed in different horse populations (159–161). This variability has a significant impact on breeding success and reproductive efficacy in horses. Genetic traits influence important parameters like sperm motility, morphology, and overall viability, which are essential for successful fertilization. Recent GWAS analysis have identified specific genes associated with seminal traits, such as sperm concentration and motility (162, 163). For example, studies have highlighted the role of cysteine-rich secretory proteins (CRISP1, CRISP2, CRISP3), as well as other genes like SIRT1, PGK2, CCT8, SOD1, and GLIPR1L1, which have been linked to important semen quality traits (164–167). These genes play crucial roles in the structure and function of sperm cells, influencing their ability to fertilize an egg. Further GWAS research has discovered associations between additional genes, such as NME8, OR2AP1, and OR6C4, and sperm motility in stallions (22). The significance of these findings lies in the potential use of these genetic markers in selective breeding programs to improve reproductive outcomes in horses. Marker-based approaches using microsatellites have also provided insight into the genetic basis of semen quality. Variants within candidate genes like SPATA1, PRLR, ACE, FKBP6, SP17, PLCz1, and FSHB have been linked to sperm motility, which directly impacts the pregnancy rate per cycle, especially in German Warmblood horses (168–173). These genes are involved in critical processes such as sperm-egg fusion and the acrosome reaction, highlighting their importance in reproductive success. Furthermore, a recent study identified the gene SCN8A, associated with sperm motility. SCN8A encodes a sodium channel found in the flagellum and around the neck of mammalian spermatozoa, suggesting its role in regulating motility (174). Overall, this suggests that genetic variations in these genes may influence semen quality by affecting sperm development, survival in the reproductive tract, or capacitation and acrosome reaction.
In this review, we used the DAVID online software (175, 176) to analyze the pathways and functions of genes related to semen quality in livestock. Among the genes analyzed, we focused on those associated with hormonal regulation and receptor activity, including GnRHR, LHR, LHβ, FSHβ, ESR1, ESR, PRLR, INHBA, INHA, INHBC, INHBE, MAD2, SMAD3, TGFB2, TGFBR1, TGFBR2, and FSHR. Our analysis revealed their involvement in several key signaling pathways: the transforming growth factor-beta (TGF-β) signaling pathway (bta04350), prolactin signaling pathway (bta04917), cAMP signaling pathway (bta04024), and Hippo signaling pathway (bta04390).
Consistent with our findings, the literature suggests that components of the Hippo signaling pathway play a critical role in spermatogenesis and sexual maturity in male reproductive tracts of Hu sheep (177). Additionally, disruptions in Hippo signaling have been linked to sperm morphological abnormalities and infertility in patients with autosomal dominant polycystic kidney disease (178). The TGF-β signaling pathway is crucial for testis development and spermatogenesis and is implicated in maintaining male tract homeostasis and function (179). Notably, studies have shown that the absence of IGF1 in sperm plasma membranes correlates with infertility (180), and the presence of TGFβ1 and TGFβ2 in porcine seminal plasma is associated with semen quality (181). Furthermore, TGF-β has been reported to modulate the immune environment of the female genital tract post-semen delivery during mating or artificial insemination (182). The cAMP signaling pathway is identified as a pivotal mechanism in gamete development, sperm capacitation, and fertilization, and it is targeted in infertility therapies (183, 184). Its role is further evidenced in regulating sperm motility in stallions (185) and has been implicated in affecting sperm motility in dairy goats via the alkaline dilution effect (186). The essential role of the prolactin signaling pathway is also underscored in our findings.
Further analysis revealed that genes involved in energy metabolism, mitochondrial function spermatogenesis, sperm development, sperm motility and structure (TEKT, TNP, PRM1, TNP, CDH, HSPA, DAZL, STRA, DPY19L2, KIT, MEIOC and KLHL10 etc.), significantly regulate other signaling pathways, including MAPK (bta04010), cytoskeleton in muscle cells (bta04820), and PI3K-Akt signaling pathway (bta04151). The biological functions of these genes are summarized in Table 5 and Figure 1. Additionally, genes implicated in apoptosis (CATSPER1, BCL2, BAX and CASP3) influence pathways such as Apoptosis - multiple species (bta04215) and p53 signaling pathway (bta04115). The p53 signaling pathway is noted for its role in maintaining semen quality by ensuring the quantity and quality of mature sperm and regulating reproductive processes such as genomic integrity and germ cell pools (187). Moreover, the SPATA18-P53 pathway is crucial for controlling mitochondrial quality by eliminating oxidative proteins, as oxidative stress can adversely affect sperm motility and quality by upregulating p53 expression (188). Lastly, genes related to the antioxidant response (SOD1, SOD2) significantly regulate the Peroxisome signaling pathway (bta04146). Peroxisome proliferator-activated receptor gamma (PPARγ) is suggested to link lipid metabolism with overall reproductive functions, providing essential energy from glucose and fat metabolism for sperm physiology and influencing male fertility (189, 190).
Figure 1. Schematic representation of genes involved in important biological processes and their associations with semen quality. This figure illustrates the relationships between various genes and their roles in biological processes such as sperm maturation, DNA integrity, spermatogenesis, oxidative stress response, and sperm motility. Please note that this figure is based on speculative information rather than validated data, and the depicted relationships should be interpreted with caution.
The integration of genomics, transcriptomics, proteomics, and metabolomics data is crucial for understanding the complex regulatory networks that impact semen quality. By considering all of these factors together, we can uncover interactions between genes, proteins, and metabolites that are not evident when studying each omics layer independently. Although this review identifies many genetic markers associated with semen quality, it is important for future research to focus on the functional validation of these markers. Technologies like CRISPR-Cas9 and RNA interference (RNAi) can be utilized to confirm the roles of these genes in spermatogenesis and fertility. In addition to genetic markers, epigenetic modifications such as DNA methylation, histone modification, and non-coding RNAs may also play significant roles in semen quality. It is essential for future studies to explore how these epigenetic factors influence gene expression related to sperm function. By conducting comparative studies across different livestock species, we may be able to identify conserved genetic pathways and markers that are crucial for reproductive success. This would provide insights that can be applied across species. However, it is important to note that while the review identifies numerous genetic markers associated with semen quality, many of these markers have not been functionally validated. This limitation hinders the direct application of these findings in breeding programs. Furthermore, it is worth considering that the genetic markers identified are often specific to certain species, limiting their generalizability across different livestock species. This poses a challenge for developing universal breeding strategies. Semen quality is a multifactorial trait influenced by various genes, environmental factors, and their complex interactions. Due to the complexity of these interactions, it is difficult to identify single markers that can reliably predict fertility outcomes. Differences in breed, animal age, and health status are critical factors that significantly influence semen quality. These variables should be carefully considered in future research studies to ensure comprehensive and accurate findings.
In conclusion, the identification and understanding of genetic markers associated with semen quality traits in livestock have the potential to significantly enhance reproductive efficiency and genetic improvement in animal breeding programs. Thanks to advancements in genomic technologies and molecular biology, we can now pinpoint specific genes and genetic variations that impact semen quality, including sperm motility, concentration, morphology, and overall fertility. This knowledge is vital for developing targeted breeding strategies that aim to improve these traits, ultimately leading to enhanced reproductive outcomes and increased productivity in livestock populations. By incorporating genetic markers into selective breeding programs, livestock producers can achieve higher fertility rates, improve genetic diversity, and increase economic benefits. Future research should focus on validating these genetic markers across different breeds and environments to ensure their practical application in diverse farming systems. Ultimately, integrating genetic insights into reproductive management practices will play a crucial role in ensuring the sustainability and profitability of livestock industries worldwide. Furthermore, this review is based on data from various studies, but inconsistencies in study design, sample sizes, and analytical methods across studies can lead to conflicting results. This variability complicates the synthesis of findings and the identification of reliable markers.
MK: Conceptualization, Data curation, Formal analysis, Investigation, Methodology, Project administration, Software, Supervision, Validation, Visualization, Writing – original draft, Writing – review & editing, Funding acquisition, Resources. WC: Data curation, Investigation, Methodology, Software, Writing – original draft, Writing – review & editing. SN: Conceptualization, Data curation, Writing – review & editing. XL: Data curation, Investigation, Methodology, Software, Writing – review & editing. HL: Conceptualization, Investigation, Software, Writing – review & editing. YC: Data curation, Investigation, Methodology, Software, Writing – review & editing. XK: Formal analysis, Writing – review & editing. YL: Conceptualization, Software, Writing – review & editing. IA: Data curation, Methodology, Software, Writing – review & editing. YH: Investigation, Methodology, Software, Validation, Writing – review & editing. YP: Investigation, Methodology, Software, Writing – review & editing. CW: Conceptualization, Funding acquisition, Investigation, Project administration, Resources, Software, Supervision, Validation, Visualization, Writing – original draft, Writing – review & editing. MZ: Conceptualization, Funding acquisition, Project administration, Supervision, Validation, Visualization, Writing – original draft, Writing – review & editing.
The author(s) declare financial support was received for the research, authorship, and/or publication of this article. This research was funded by the National Key R&D Program of China (grant numbers 2022YFD1600103; 2023YFD1302004), The Shandong Province Modern Agricultural Technology System Donkey Industrial Innovation Team (grant number SDAIT-27), Livestock and Poultry Breeding Industry Project of the Ministry of Agriculture and Rural Affairs (grant number 19211162), Open Project of Liaocheng University Animal Husbandry Discipline (grant number 319312101–14), Open Project of Shandong Collaborative Innovation Center for Donkey Industry Technology (grant number 3193308), Research on Donkey Pregnancy Improvement (grant number K20LC0901), Liaocheng University Scientific Research Fund (grant number 318052025), the National Natural Science Foundation of China (grant no. 31671287) and Key R&D Program Project of Shandong Province (2021TZXD012) We express our sincere gratitude to the Liaocheng Research Institute of Donkey High-efficiency Breeding and Ecological Feeding, Liaocheng University for providing us financial support.
The authors declare that the research was conducted in the absence of any commercial or financial relationships that could be construed as a potential conflict of interest.
All claims expressed in this article are solely those of the authors and do not necessarily represent those of their affiliated organizations, or those of the publisher, the editors and the reviewers. Any product that may be evaluated in this article, or claim that may be made by its manufacturer, is not guaranteed or endorsed by the publisher.
CAPZA3: Capping actin protein of muscle Z-line subunit alpha 3
CAPZB: Capping actin protein of muscle Z-line subunit beta
CASP3: Caspase 3
CATSPER1: Cation channel sperm associated 1
CDH2: Cadherin 2
CDH1: Cadherin 1
TGFB2: Transforming growth factor beta 2
ACTB: Actin beta
ACTR2: Actin related protein 2
ADAD1: Adenosine deaminase domain containing 1
ATP1B1: ATPase Na+/K+ transporting subunit beta 1
CFAP58: Cilia and flagella associated protein 58
CRISP3: Cysteine-rich secretory protein 3
CROCC2: Ciliary rootlet coiled-coil, rootletin family member 2
CSNK1G2: Casein kinase 1 gamma 2
DAZL: Deleted in azoospermia like
DICER1: Dicer 1, ribonuclease III
DPY19L2: Dpy-19 like 2
ESR1: Estrogen receptor 1
FSHR: Follicle stimulating hormone receptor
GNRHR: Gonadotropin releasing hormone receptor
HOOK1: Hook microtubule tethering protein 1
FYN: FYN proto-oncogene, Src family tyrosine kinase
ICAM1: Intercellular adhesion molecule 1
HSPA4L: Heat shock protein family A (HSP70) member 4 like
INHA: Inhibin subunit alpha
INHBA: inhibin subunit beta A
INHBC: Inhibin subunit beta C
INHBE: Inhibin subunit beta E
JAM3: Junctional adhesion molecule 3
KIT: KIT proto-oncogene, receptor tyrosine kinase
KLHL10: Kelch like family member 10
MAP3K1: Mitogen-activated protein kinase 1
MDC1: Mediator of DNA damage checkpoint 1
MEIOC: Meiosis specific with coiled-coil domain
TEKT3: Tektin 3
TGFB2: Transforming growth factor beta 2
TGFBR1: Transforming growth factor beta receptor 1
TNF: Tumor necrosis factor
TNP1: Transition protein 1
TUBB: Tubulin beta class I
VCAM1: Vascular cell adhesion molecule 1
ZSWIM7: Zinc finger SWIM-type containing 7
TNP2: Transition protein 2
MOV10L1: Mov10 like RISC complex RNA helicase 1
MSH2: MutS homolog 2
MTNR1A: Melatonin receptor 1A
NOS2: Nitric oxide synthase 2
OAZ3: Ornithine decarboxylase antizyme 3
PPP1CC: Protein phosphatase 1 catalytic subunit gamma
PRKAR1B: Protein kinase cAMP-dependent type I regulatory subunit beta
PRLR: Prolactin receptor
PRM1: Protamine 1
RAB33B: RAB33B, member RAS oncogene family
RAD51B: RAD51 paralog B
SMAD3: SMAD family member 3
SPAG6: Sperm associated antigen 6
SRC: SRC proto-oncogene, non-receptor tyrosine kinase
STRA8: Stimulated by retinoic acid 8
TDRD5: Tudor domain containing 5
SUN5: Sad1 and UNC84 domain containing 5
SYCE3: Synaptonemal complex central element protein 3
1. Abu-Halima M, Becker LS, Al Smadi MA, Abdul-Khaliq H, Raeschle M, Meese E. Sperm motility annotated genes: Are they associated with impaired fecundity? Cells. (2023) 12:1239. doi: 10.3390/cells12091239
2. Ribas-Maynou J, Barranco I, Salas-Huetos A. Sperm quality and fertility of livestock animals. Animals. (2023) 13:604. doi: 10.3390/ani13040604
3. Graziani A, Rocca MS, Vinanzi C, Masi G, Grande G, De Toni L, et al. Genetic causes of qualitative sperm defects: A narrative review of clinical evidence. Genes. (2024) 15:600. doi: 10.3390/genes15050600
4. Linn E, Ghanem L, Bhakta H, Greer C, Avella M. Genes regulating spermatogenesis and sperm function associated with rare disorders. Front Cell Dev Biol. (2021) 9:634536. doi: 10.3389/fcell.2021.634536
5. Jung M, Rüdiger K, Schulze M. In vitro measures for assessing boar semen fertility. Reprod Domest animals. (2015) 50:20–4. doi: 10.1111/rda.2015.50.issue-S2
6. Altmäe S, Salumets A. A novel genomic diagnostic tool for sperm quality? Reprod BioMedicine Online. (2011) 22:405–7. doi: 10.1016/j.rbmo.2010.12.009
7. Llavanera M. Evaluation of sperm quality and male fertility: the use of molecular markers in boar sperm and seminal plasma. Anim Reprod Science. (2024) 28:107545. doi: 10.1016/j.anireprosci.2024.107545
8. Selvaraju S, Parthipan S, Somashekar L, Binsila BK, Kolte AP, Arangasamy A, et al. Current status of sperm functional genomics and its diagnostic potential of fertility in bovine (Bos taurus). Syst Biol Reprod Med. (2018) 64:484–501. doi: 10.1080/19396368.2018.1444816
9. Reyer H, Abou-Soliman I, Schulze M, Henne H, Reinsch N, Schoen J, et al. Genome-wide association analysis of semen characteristics in piétrain boars. Genes. (2024) 15:382. doi: 10.3390/genes15030382
10. Abril-Parreño L, Carthy TR, Keogh K, Štiavnická M, O’Meara C, Lonergan P, et al. Genome-wide association study reveals candidate markers related to field fertility and semen quality traits in Holstein-Friesian bulls. animal. (2023) 17:100841. doi: 10.1016/j.animal.2023.100841
11. Selvaraju S, Parthipan S, Somashekar L, Kolte AP, Krishnan Binsila B, Arangasamy A, et al. Occurrence and functional significance of the transcriptome in bovine (Bos taurus) spermatozoa. Sci Rep. (2017) 7:42392. doi: 10.1038/srep42392
12. Somashekar L, Selvaraju S, Parthipan S, Patil SK, Binsila BK, Manjunatha V, et al. Comparative sperm protein profiling in bulls differing in fertility and identification of phosphatidylethanolamine-binding protein 4, a potential fertility marker. Andrology. (2017) 5:1032–51. doi: 10.1111/andr.2017.5.issue-5
13. Robertson MJ, Chambers C, Spanner EA, de Graaf SP, Rickard JP. The assessment of sperm DNA integrity: implications for assisted reproductive technology fertility outcomes across livestock species. Biology. (2024) 13:539. doi: 10.3390/biology13070539
14. Mukherjee A, Gali J, Kar I, Datta S, Roy M, Acharya AP, et al. Candidate genes and proteins regulating bull semen quality: A review. Trop Anim Health Production. (2023) 55:212. doi: 10.1007/s11250-023-03617-0
15. Liu Y, Cao J, Gai K, Cong B, Guo S, Xing K, et al. Research progress on the genes regulating sperm motility in domestic animals. Chin Anim Husbandry Veterinary Med. (2023) 50:4958–72. doi: 10.16431/j.cnki.1671-7236.2023.12.018
16. Ďuračka M, Benko F, Tvrdá E. Molecular markers: A new paradigm in the prediction of sperm freezability. Int J Mol Sci. (2023) 24:3379. doi: 10.3390/ijms24043379
17. Li T, Wang H, Luo R, Shi H, Su M, Wu Y, et al. Identification and functional assignment of genes implicated in sperm maturation of Tibetan sheep. Animals. (2023) 13:1553. doi: 10.3390/ani13091553
18. Wijayanti D, Luo Y, Bai Y, Pan C, Qu L, Guo Z, et al. New insight into copy number variations of goat SMAD2 gene and their associations with litter size and semen quality. Theriogenology. (2023) 206:114–22. doi: 10.1016/j.theriogenology.2023.05.012
19. Zhang X, Lin Q, Liao W, Zhang W, Li T, Li J, et al. Identification of new candidate genes related to semen traits in Duroc pigs through weighted single-step GWAS. Animals. (2023) 13:365. doi: 10.3390/ani13030365
20. Li W, Mi S, Zhang J, Liu X, Chen S, Liu S, et al. Integrating sperm cell transcriptome and seminal plasma metabolome to analyze the molecular regulatory mechanism of sperm motility in Holstein stud bulls. J Anim Science. (2023) 101:skad214. doi: 10.1093/jas/skad214
21. El Nagar AG, Salem MM, Amin AM, Khalil MH, Ashour AF, Hegazy MM, et al. A single-step genome-wide association study for semen traits of Egyptian buffalo bulls. Animals. (2023) 13:3758. doi: 10.3390/ani13243758
22. Nikitkina EV, Dementieva NV, Shcherbakov YS, Atroshchenko MM, Kudinov AA, Samoylov OI, et al. Genome-wide association study for frozen-thawed sperm motility in stallions across various horse breeds. Anim Biosciences. (2022) 35:1827. doi: 10.5713/ab.21.0504
23. Mapel XM, Kadri NK, Leonard AS, He Q, Lloret-Villas A, Bhati M, et al. Molecular quantitative trait loci in reproductive tissues impact male fertility in cattle. Nat Commun. (2024) 15:674. doi: 10.1038/s41467-024-44935-7
24. Modiba MC, Nephawe KA, Mdladla KH, Lu W, Mtileni B. Candidate genes in bull semen production traits: An information approach review. Veterinary Sci. (2022) 9:155. doi: 10.3390/vetsci9040155
25. Nogueira E, Tirpák F, Hamilton LE, Zigo M, Kerns K, Sutovsky M, et al. A non-synonymous point mutation in a WD-40 domain repeat of EML5 leads to decreased bovine sperm quality and fertility. Front Cell Dev Biol. (2022) 10:872740. doi: 10.3389/fcell.2022.872740
26. Ghoreishifar M, Vahedi SM, Salek Ardestani S, Khansefid M, Pryce JE. Genome-wide assessment and mapping of inbreeding depression identifies candidate genes associated with semen traits in Holstein bulls. BMC Genomics. (2023) 24:230. doi: 10.1186/s12864-023-09298-1
27. Ramirez-Diaz J, Cenadelli S, Bornaghi V, Bongioni G, Montedoro SM, Achilli A, et al. Identification of genomic regions associated with total and progressive sperm motility in Italian Holstein bulls. J Dairy Science. (2023) 106:407–20. doi: 10.3168/jds.2021-21700
28. Barkova OY, Starikova DA, Chistyakova IV. Analysis of correlation between PRM1, STK35, and IFT27 gene expression levels and Holstein bull semen quality parameters. Russian Agric Sci. (2024) 50:87–91. doi: 10.3103/S1068367424010038
29. Tan WL, Hudson NJ, Porto Neto LR, Reverter A, Afonso J, Fortes MR. An association weight matrix identified biological pathways associated with bull fertility traits in a multi-breed population. Anim Genet. (2024) 55:495–510. doi: 10.1111/age.13431
30. Ashwitha A, Ramesha KP, Ramesh P, Kootimole CN, Devadasan MJ, Ammankallu S, et al. Quantitative proteomics profiling of spermatozoa and seminal plasma reveals proteins associated with semen quality in Bos indicus bulls. J Proteomics. (2023) 273:104794. doi: 10.1016/j.jprot.2022.104794
31. Gebreyesus G, Secher JB, Lund MS, Kupisiewicz K, Ivask M, Hallap T, et al. Genetic parameters for bull effects on in vitro embryo production (IVP) and relationship between semen quality traits and IVP performance. Anim Reprod Science. (2024) 247:107436. doi: 10.1016/j.anireprosci.2024.107436
32. Elango K, Karuthadurai T, Kumaresan A, Sinha MK, Ebenezer Samuel King JP, Nag P, et al. High-throughput proteomic characterization of seminal plasma from bulls with contrasting semen quality. 3 Biotech. (2023) 13:60. doi: 10.1007/s13205-023-03474-6
33. Sinha MK, Kumaresan A, Rao Talluri T, Ebenezer Samuel King JP, Prakash MA, Nag P, et al. Single nucleotide polymorphisms cumulating to genetic variation for fertility in crossbred (Bos taurus × Bos indicus) bull spermatozoa. Anim Biotechnol. (2023) 34:2875–86. doi: 10.1080/10495398.2022.2124166
34. Nikitkina E, Dementieva N, Shcherbakov Y, Musidray A, Krutikova A, Bogdanova S, et al. Search for genetic associations with semen morphology after cryopreservation in bulls. Anim Reprod Science. (2022) 247:107117. doi: 10.1016/j.anireprosci.2022.107117
35. Nikitkina E, Krutikova A, Musidray A, Plemyashov K. Search for associations of FSHR, INHA, INHAB, PRL, TNP2 and SPEF2 genes polymorphisms with semen quality in Russian Holstein bulls (pilot study). Animals. (2021) 11:2882. doi: 10.3390/ani11102882
36. Dementieva NV, Dysin AP, Shcherbakov YS, Nikitkina EV, Musidray AA, Petrova AV, et al. Risk of sperm disorders and impaired fertility in frozen-thawed bull semen: A genome-wide association study. Animals. (2024) 14:251. doi: 10.3390/ani14020251
37. Wei X, Wang X, Yang C, Gao Y, Zhang Y, Xiao Y, et al. CFAP58 is involved in the sperm head shaping and flagellogenesis of cattle and mice. Development. (2024) 151:dev202608. doi: 10.1242/dev.202608
38. Pardede BP, Karja NW, Said S, Kaiin EM, Agil M, Sumantri C, et al. Bovine nucleoprotein transitions genes and protein abundance as valuable markers of sperm motility and the correlation with fertility. Theriogenology. (2024) 215:86–94. doi: 10.1016/j.theriogenology.2023.11.015
39. Ebenezer JP, Kumaresan A, Talluri TR, Sinha MK, Raval K, Nag P, et al. Genome-wide analysis identifies single nucleotide polymorphism variations and altered pathways associated with poor semen quality in breeding bulls. Reprod Domest Animals. (2022) 57:1143–55. doi: 10.1111/rda.14185
40. Hussain S, Alex R, Alyethodi RR, Sharma S, Verma N, Sirohi AS, et al. Development of a RAPD marker-based classification criterion for quality semen production in Holstein crossbred bulls. Reprod Domest Animals. (2021) 56:736–43. doi: 10.1111/rda.13912
41. Sweett H, Fonseca PA, Suárez-Vega A, Livernois A, Miglior F, Cánovas A. Genome-wide association study to identify genomic regions and positional candidate genes associated with male fertility in beef cattle. Sci Rep. (2020) 10:20102. doi: 10.1038/s41598-020-75758-3
42. Hiltpold M, Niu G, Kadri NK, Crysnanto D, Fang ZH, Spengeler M, et al. Activation of cryptic splicing in bovine WDR19 is associated with reduced semen quality and male fertility. PloS Genet. (2020) 16:e1008804. doi: 10.1371/journal.pgen.1008804
43. Elango K, Kumaresan A, Sharma A, Nag P, Prakash MA, Sinha MK, et al. Sub-fertility in crossbred bulls: Deciphering testicular level transcriptomic alterations between Zebu (Bos indicus) and crossbred (Bos taurus × Bos indicus) bulls. BMC Genomics. (2020) 21:502. doi: 10.1186/s12864-020-06907-1
44. Vineeth MR, Surya T, Sivalingam J, Kumar A, Niranjan SK, Dixit SP, et al. Genome-wide discovery of SNPs in candidate genes related to production and fertility traits in Sahiwal cattle. Trop Anim Health Production. (2020) 52:1707–15. doi: 10.1007/s11250-019-02180-x
45. Yin H, Zhou C, Shi S, Fang L, Liu J, Sun D, et al. Weighted single-step genome-wide association study of semen traits in Holstein bulls of China. Front Genet. (2019) 10:1053. doi: 10.3389/fgene.2019.01053
46. Borowska A, Szwaczkowski T, Kamiński S, Hering DM, Kordan W, Lecewicz M. Identification of genome regions determining semen quality in Holstein-Friesian bulls using information theory. Anim Reprod Science. (2018) 192:206–15. doi: 10.1016/j.anireprosci.2018.03.012
47. Kumari R, Ramesha KP, Kumar R, Divya P, Sinha B. Genetic polymorphism of aquaporin 7 gene and its association with semen quality in Surti bulls. Buffalo Bulletin. (2018) 37:191–8. Available at: https://kuojs.lib.ku.ac.th/index.php/BufBu/article/view/40.
48. Yathish HM, Kumar S, Chaudhary R, Mishra C, Sivakumar A, Kumar A, et al. Nucleotide variability of protamine genes influencing bull sperm motility variables. Anim Reprod Science. (2018) 193:126–39. doi: 10.1016/j.anireprosci.2018.04.060
49. Qin C, Yin H, Zhang X, Sun D, Zhang Q, Liu J, et al. Genome-wide association study for semen traits of the bulls in Chinese Holstein. Anim Genet. (2017) 48:80–4. doi: 10.1111/age.2017.48.issue-1
50. Hering DM, Olenski K, Kaminski S. Genome-wide association study for poor sperm motility in Holstein-Friesian bulls. Anim Reprod Science. (2014) 146:89–97. doi: 10.1016/j.anireprosci.2014.01.012
51. Hering DM, Olenski K, Kaminski S. Genome-wide association study for sperm concentration in Holstein-Friesian bulls. Reprod Domest Anim. (2014) 49:1008–14. doi: 10.1111/rda.2014.49.issue-6
52. Hering DM, Oleński K, Ruść A, Kaminski S. Genome-wide association study for semen volume and total number of sperm in Holstein-Friesian bulls. Anim Reprod Science. (2014) 151:126–30. doi: 10.1016/j.anireprosci.2014.10.022
53. Puglisi R, Gaspa G, Balduzzi D, Severgnini A, Vanni R, Macciotta NP, et al. Genome-wide analysis of bull sperm quality and fertility traits. Reprod Domest Animals. (2016) 51:840–3. doi: 10.1111/rda.2016.51.issue-5
54. Han Y, Peñagaricano F. Unravelling the genomic architecture of bull fertility in Holstein cattle. BMC Genet. (2016) 17:1. doi: 10.1186/s12863-016-0454-6
55. Gao Q, Ju Z, Zhang Y, Huang J, Zhang X, Qi C, et al. Association of TNP2 gene polymorphisms of the bta-miR-154 target site with the semen quality traits of Chinese Holstein bulls. PloS One. (2014) 9:e84355. doi: 10.1371/journal.pone.0084355
56. Sang L, Du QZ, Yang WC, Tang KQ, Yu JN, Hua GH, et al. Polymorphisms in follicle stimulation hormone receptor, inhibin alpha, inhibin beta A, and prolactin genes, and their association with sperm quality in Chinese Holstein bulls. Anim Reprod Science. (2011) 126:151–6. doi: 10.1016/j.anireprosci.2011.04.023
57. Pan Q, Ju Z, Huang J, Zhang Y, Qi C, Gao Q, et al. PLCz functional haplotypes modulating promoter transcriptional activity are associated with semen quality traits in Chinese Holstein bulls. PloS One. (2013) 8:e58795. doi: 10.1371/journal.pone.0058795
58. Liu X, Ju Z, Wang L, Zhang Y, Huang J, Li Q, et al. Six novel single-nucleotide polymorphisms in SPAG11 gene and their association with sperm quality traits in Chinese Holstein bulls. Anim Reprod Science. (2011) 129:14–21. doi: 10.1016/j.anireprosci.2011.10.003
59. Rorie RW, Williams CL, Lester TD. Association of osteopontin gene promoter single nucleotide polymorphisms with bull semen quality. Adv Reprod Sci. (2016) 4:1–7. doi: 10.4236/arsci.2016.41001
60. Kamiński S, Hering DM, Oleński K, Lecewicz M, Kordan W. Genome-wide association study for sperm membrane integrity in frozen-thawed semen of Holstein-Friesian bulls. Anim Reprod Science. (2016) 170:135–40. doi: 10.1016/j.anireprosci.2016.05.002
61. Liu J, Sun Y, Yang C, Zhang Y, Jiang Q, Huang J, et al. Functional SNPs of INCENP affect semen quality by alternative splicing mode and binding affinity with the target Bta-miR-378 in Chinese Holstein bulls. PloS One. (2016) 11:e0162730. doi: 10.1371/journal.pone.0162730
62. Cui X, Sun Y, Wang X, Yang C, Ju Z, Jiang Q, et al. A g.-1256 A> C in the promoter region of CAPN1 is associated with semen quality traits in Chinese Holstein bulls. Reproduction. (2016) 152:101–9. doi: 10.1530/REP-15-0535
63. Huang J, Guo F, Zhang Z, Zhang Y, Wang X, Ju Z, et al. PCK1 is negatively regulated by bta-miR-26a, and a single-nucleotide polymorphism in the 3′ untranslated region is involved in semen quality and longevity of Holstein bulls. Mol Reprod Dev. (2016) 83:217–25. doi: 10.1002/mrd.22613
64. Alyethodi RR, Deb R, Alex R, Kumar S, Singh U, Tyagi S, et al. Molecular markers, BM1500 and UMN2008, are associated with post-thaw motility of bull sperm. Anim Reprod Science. (2016) 174:143–9. doi: 10.1016/j.anireprosci.2016.10.001
65. Grant KE, De Oliveira RV, Hennington BS, Govindaraju A, Perkins A, Stokes J, et al. Sperm superoxide dismutase is associated with bull fertility. Reproduction Fertility Dev. (2016) 28:1405–13. doi: 10.1071/RD14399
66. Zhang S, Zhang Y, Yang C, Ju Z, Wang X, Jiang Q, et al. TNP1 functional SNPs in bta-miR-532 and bta-miR-204 target sites are associated with semen quality traits in Chinese Holstein bulls. Biol Reprod. (2015) 92:139. doi: 10.1530/REP-15-0126
67. Zhang S, Zhang Y, Yang C, Ju Z, Wang X, Jiang Q, et al. The g.-165 T> C rather than methylation is associated with semen motility in Chinese Holstein bulls by regulating the transcriptional activity of the HIBADH gene. PloS One. (2015) 10:e0127670. doi: 10.1371/journal.pone.0127670
68. Kumar S, Singh U, Deb R, Tyagi S, Mandal DK, Kumar M, et al. A SNP (g. 358A> T) at intronic region of CD9 molecule of crossbred bulls may associate with spermatozoal motility. Meta Gene. (2015) 5:140–3. doi: 10.1016/j.mgene.2015.07.004
69. Chen X, Wang Y, Zhu H, Hao H, Zhao X, Qin T, et al. Comparative transcript profiling of gene expression of fresh and frozen-thawed bull sperm. Theriogenology. (2015) 83:504–11. doi: 10.1016/j.theriogenology.2014.10.015
70. Hering DM, Lecewicz M, Kordan W, Majewska A, Kaminski S. Missense mutation in glutathione-S-transferase M1 gene is associated with sperm motility and ATP content in frozen-thawed semen of Holstein-Friesian bulls. Anim Reprod Science. (2015) 159:94–7. doi: 10.1016/j.anireprosci.2015.06.001
71. Guan F, Song XM, Li J, Wang HX, Liu YX, Jiang JF, et al. Effects of PRNP polymorphisms on sperm quality traits in Chinese Holstein bulls. J Appl Anim Res. (2014) 42:284–8. doi: 10.1080/09712119.2013.842486
72. Deb R, Kumar S, Singh U, Tyagi S, Mandal DK, Sengar G, et al. Evaluation of three bovine Y specific microsatellite loci as an alternative biomarkers for semen quality traits in crossbred bull. Anim Reprod Science. (2013) 142:121–5. doi: 10.1016/j.anireprosci.2013.09.015
73. Fortes MR, Reverter A, Kelly M, McCulloch R, Lehnert SA. Genome-wide association study for inhibin, luteinizing hormone, insulin-like growth factor 1, testicular size and semen traits in bovine species. Andrology. (2013) 1:644–50. doi: 10.1111/j.2047-2927.2013.00101.x
74. Ganguly I, Gaur GK, Kumar S, Mandal DK, Kumar M, Singh U, et al. Differential expression of protamine 1 and 2 genes in mature spermatozoa of normal and motility impaired semen producing Crossbred Frieswal (HF × Sahiwal) bulls. Res Veterinary Science. (2013) 94:256–62. doi: 10.1016/j.rvsc.2012.09.001
75. Sun LP, Du QZ, Song YP, Yu JN, Wang SJ, Sang L, et al. Polymorphisms in luteinizing hormone receptor and hypothalamic gonadotropin-releasing hormone genes and their effects on sperm quality traits in Chinese Holstein bulls. Mol Biol Rep. (2012) 39:7117–23. doi: 10.1007/s11033-012-1543-x
76. Yang WC, Tang KQ, Yu JN, Zhang CY, Zhang XX, Yang LG. Effects of Mbo II and BspM I polymorphisms in the gonadotropin releasing hormone receptor (GnRHR) gene on sperm quality in Holstein bulls. Mol Biol Rep. (2011) 38:3411–5. doi: 10.1007/s11033-010-0450-2
77. Hamilton CK, Verduzco-Gómez AR, Favetta LA, Blondin P, King WA. Testis-specific protein Y-encoded copy number is correlated to its expression and the field fertility of Canadian Holstein bulls. Sexual Dev. (2012) 6:231–9. doi: 10.1159/000338938
78. Liu X, Ju Z, Wang L, Zhang Y, Huang J, Qi C, et al. Effects of DraI, StyI, and MspI polymorphisms and haplotypic combinations of the transferrin (Tf) gene on the sperm quality of Chinese Holstein bulls. Afr J Microbiol Res. (2012) 6:594–602. doi: 10.5897/AJMR11.1429
79. Ma T, Liu J, Zhao R, Jiang H, Dai L, Zhao Y, et al. Association analysis of aquaporin 7 (AQP7) gene variants with semen quality and fertility in bulls. Turkish J Veterinary Anim Sci. (2011) 35:63–6. doi: 10.3906/vet-0908-33
80. Parthipan S, Selvaraju S, Somashekar L, Arangasamy A, Sivaram M, Ravindra JP. Spermatozoal transcripts expression levels are predictive of semen quality and conception rate in bulls (Bos taurus). Theriogenology. (2017) 98:41–9. doi: 10.1016/j.theriogenology.2017.04.042
81. Abdo SE, Abdelghany ES, Helal M, Sakr AM, Abu El-Magd M, Hegazy M, et al. Association of polymorphism in leptin gene with semen quality parameters in Egyptian buffaloes bulls. Egyptian J Veterinary Science. (2024) 56:501–10. doi: 10.21608/ejvs.2024.276609.1921
82. Swathi D, Ramya L, Archana SS, Krishnappa B, Binsila BK, Selvaraju S. Identification of hub genes and their expression profiling for predicting buffalo (Bubalus bubalis) semen quality and fertility. Sci Rep. (2023) 13:22126. doi: 10.1038/s41598-023-48925-5
83. Selvaraju S, Ramya L, Swathi D, Archana SS, Lavanya M, Krishnappa B, et al. Cryostress induces fragmentation and alters the abundance of sperm transcripts associated with fertilizing competence and reproductive processes in buffalo. Cell Tissue Res. (2023) 393:181–99. doi: 10.1007/s00441-023-03764-8
84. Swathi D, Ramya L, Archana SS, Lavanya M, Krishnappa B, Binsila BK, et al. X chromosome-linked genes in the mature sperm influence semen quality and fertility of breeding bulls. Gene. (2022) 839:146727. doi: 10.1016/j.gene.2022.146727
85. Mahmoud KG, Sakr AM, Ibrahim SR, Sosa AS, Hasanain MH, Nawito MF. GnRHR gene polymorphism and its correlation with semen quality in buffalo bulls (Bubalus bubalis). Iraqi J Veterinary Sci. (2021) 35:381–6. doi: 10.33899/ijvs.2020.126886.1407
86. Ma KM, Kumaresan A, Yadav S, Mohanty TK, Datta TK. Comparative proteomic analysis of high- and low-fertile buffalo bull spermatozoa for identification of fertility-associated proteins. Reprod Domest Animals. (2019) 54:786–94. doi: 10.1111/rda.13426
87. Wang S, Zhang Y, Cheng Y, Lu G, Yang R, Geng H, et al. Association of SNPs in GnRH gene with sperm quality traits of Chinese water buffalo. Reprod Domest Animals. (2020) 55:384–92. doi: 10.1111/rda.13634
88. Wang G, Hao L, Cheng Y, Li S, Zhang Y, Lv C, et al. Effects of GnRHR polymorphisms on sperm quality in Chinese water buffalo. Anim Reprod Science. (2017) 186:37–43. doi: 10.1016/j.anireprosci.2017.09.001
89. Reen JK, Kerekoppa R, Deginal R, Ahirwar MK, Kannegundla U, Chandra S, et al. Luteinizing hormone beta gene polymorphism and its effect on semen quality traits and luteinizing hormone concentrations in Murrah buffalo bulls. Asian-Australasian J Anim Sci. (2018) 31:1119–28. doi: 10.5713/ajas.17.0679
90. Cheng Y, Gu J, Xue H, Li Q, Liang M, Wang N, et al. Identification of four SNPs in LHB gene and their associations with sperm qualities of Chinese buffaloes. Anim Biotechnol. (2017) 28:168–73. doi: 10.1080/10495398.2016.1244068
91. Štiavnická M, Chaulot-Talmon A, Perrier JP, Hošek P, Kenny DA, Lonergan P, et al. Sperm DNA methylation patterns at discrete CpGs and genes involved in embryonic development are related to bull fertility. BMC Genomics. (2022) 23:379. doi: 10.1186/s12864-022-08614-5
92. Binsila BK, Archana SS, Ramya L, Swathi D, Selvaraju S, Gowda NS, et al. Elucidating the processes and pathways enriched in buffalo sperm proteome in regulating semen quality. Cell Tissue Res. (2021) 383:881–903. doi: 10.1007/s00441-020-03303-9
93. Liu R, Huang X, Sun Q, Hou Z, Yang W, Zhang J, et al. Comparative proteomic analyses of poorly motile swamp buffalo spermatozoa reveal low energy metabolism and deficiencies in motility-related proteins. Animals. (2022) 12:1706. doi: 10.3390/ani12131706
94. Karuthadurai T, Das DN, Kumaresan A, Sinha MK, Kamaraj E, Nag P, et al. Sperm transcripts associated with odorant binding and olfactory transduction pathways are altered in breeding bulls producing poor-quality semen. Front Veterinary Science. (2022) 9:799386. doi: 10.3389/fvets.2022.799386
95. Paul N, Kumaresan A, Das Gupta M, Nag P, Guvvala PR, Kuntareddi C, et al. Transcriptomic profiling of buffalo spermatozoa reveals dysregulation of functionally relevant mRNAs in low-fertile bulls. Front Veterinary Science. (2021) 7:609518. doi: 10.3389/fvets.2020.609518
96. Hou Z, Fu Q, Huang Y, Zhang P, Chen F, Li M, et al. Comparative proteomic identification buffalo spermatozoa during in vitro capacitation. Theriogenology. (2019) 126:303–9. doi: 10.1016/j.theriogenology.2018.12.025
97. Xiong Z, Zhang H, Huang B, Liu Q, Wang Y, Shi D, et al. Expression pattern of prohibitin, capping actin protein of muscle Z-line beta subunit and tektin-2 gene in Murrah buffalo sperm and its relationship with sperm motility. Asian-Australasian J Anim Sci. (2018) 31:1729–36. doi: 10.5713/ajas.18.0025
98. Rolim Filho ST, Ribeiro HF, de Camargo GM, Cardoso DF, Aspilcueta-Borquis RR, Tonhati H, et al. Identification of polymorphisms in the osteopontin gene and their associations with certain semen production traits of water buffaloes in the Brazilian Amazon. Reprod Domest Animals. (2013) 48:705–9. doi: 10.1111/rda.2013.48.issue-5
99. Selvaraju S, Reddy IJ, Nandi S, Rao SB, Ravindra JP. Influence of IGF-I on buffalo (Bubalus bubalis) spermatozoa motility, membrane integrity, lipid peroxidation and fructose uptake in vitro. Anim Reprod Sci. (2009) 113:60–70. doi: 10.1016/j.anireprosci.2008.08.011
100. Hodge MJ, de Las Heras-Saldana S, Rindfleish SJ, Stephen CP, Pant SD. QTLs and candidate genes associated with semen traits in Merino sheep. Animals. (2023) 13:2286. doi: 10.3390/ani13142286
101. Serrano M, Ramón M, Calvo JH, Jiménez MÁ, Freire F, Vázquez JM, et al. Genome-wide association studies for sperm traits in Assaf sheep breed. Animals. (2021) 15:100065. doi: 10.1016/j.animal.2020.100065
102. Getaneh M, Taye M, Alemayehu K, Haile A, Getachew T, Ayalew W. A review on candidate genes associated with sheep fertility traits: implications for genetic improvement of indigenous sheep breeds in developing countries. Ecol Genet Genomics. (2024) 31:100243. doi: 10.1016/j.egg.2024.100243
103. Wang K, Kang Z, Jiang E, Yan H, Zhu H, Liu J, et al. Genetic effects of DSCAML1 identified in genome-wide association study revealing strong associations with litter size and semen quality in goat (Capra hircus). Theriogenology. (2020) 146:20–5. doi: 10.1016/j.theriogenology.2020.01.079
104. Talouarn E, Bardou P, Palhière I, Oget C, Clément V, Consortium V, et al. Genome-wide association analysis on semen volume and milk yield using different strategies of imputation to whole genome sequence in French dairy goats. BMC Genet. (2020) 21:1–3. doi: 10.1186/s12863-020-0826-9
105. Xu H, Sun W, Pei S, Li W, Li F, Yue X. Identification of key genes related to postnatal testicular development based on transcriptomic data of testis in Hu sheep. Front Genet. (2022) 12:773695. doi: 10.3389/fgene.2021.773695
106. Liu J, Li W, Weng X, Yue X, Li F. Composition of fatty acids and localization of SREBP1 and ELOVL2 genes in cauda epididymides of Hu sheep with different fertility. Animals. (2022) 12:3302. doi: 10.3390/ani12233302
107. Pei S, Xu H, Wang L, Li F, Li W, Yue X. Copy number variation of ZNF280BY across eight sheep breeds and its association with testicular size of Hu sheep. J Anim Sci. (2022) 100:skac232. doi: 10.1093/jas/skac232
108. Fu X, Yang Y, Yan Z, Liu M, Wang X. Transcriptomic study of spermatogenesis in the testis of Hu sheep and Tibetan sheep. Genes. (2022) 13:2212. doi: 10.3390/genes13122212
109. Khan MK, Hossain MI, Momin MM. Impact of protein supplementation on semen quality, fertility, and BMP1R gene expression in sheep of Bangladesh. Trans Anim Sci. (2022) 6:txac072. doi: 10.1093/tas/txac072
110. Kianpoor S, Abdolmohammadi A, Hajarian H, Nikousefat Z, Khamisabadi H. Association of MTNR1A and CYP19 genes polymorphisms with sperm quality and testicular size in Sanjabi breed rams. Ann Anim Science. (2018) 18:699–711. doi: 10.2478/aoas-2018-0021
111. Bakhtiar R, Abdolmohammadi A, Hajarian H, Nikousefat Z, Kalantar-Neyestanaki D. Identification of g. 170G > A and g. 332G > A mutations in exon 3 of leptin gene (Bcnl and Cail) and their association with semen quality and testicular dimensions in Sanjabi rams. Anim Reprod Science. (2017) 179:49–56. doi: 10.1016/j.anireprosci.2017.01.016
112. Bai Y, Zhang T, Liu N, Wang C, Guo Z, Pan C, et al. Investigation of copy number variations (CNVs) of the goat PPP3CA gene and their effect on litter size and semen quality. Animals. (2022) 12:445. doi: 10.3390/ani12040445
113. Xu H, Zhang S, Duan Q, Lou M, Ling Y. Comprehensive analyses of 435 goat transcriptomes provides insight into male reproduction. Int J Biol Macromolecules. (2024) 255:127942. doi: 10.1016/j.ijbiomac.2023.127942
114. Nikbin S, Panandam JM, Yaakub H, Murugaiyah M. Association of novel SNPs in gonadotropin genes with sperm quality traits of Boer goats and Boer crosses. J Appl Anim Res. (2018) 46:459–66. doi: 10.1080/09712119.2017.1336441
115. Qu YH, Jian LY, Ce L, Ma Y, Xu CC, Gao YF, et al. Identification of candidate genes in regulation of spermatogenesis in sheep testis following dietary vitamin E supplementation. Anim Reprod Science. (2019) 205:52–61. doi: 10.1016/j.anireprosci.2019.04.003
116. Harighi MF, Wahid H, Thomson PC, Rafii MY, Jesse FF. Novel SNPs in the SPAG11 gene and association with testicular biometric variables in Boer goats and application of the levelled-container technique. Anim Reprod Science. (2019) 208:106113. doi: 10.1016/j.anireprosci.2019.106113
117. Wimmers K, Lin CL, Tholen E, Jennen DG, Schellander K, Ponsuksili S. Polymorphisms in candidate genes as markers for sperm quality and boar fertility. Anim Genet. (2005) 36:152–5. doi: 10.1111/j.1365-2052.2005.01267.x
118. Schulze M, Buder S, Rüdiger K, Beyerbach M, Waberski D. Influences on semen traits used for selection of young AI boars. Anim Reprod Science. (2014) 148:164–70. doi: 10.1016/j.anireprosci.2014.06.008
119. Lin CL, Ponsuksili S, Tholen E, Jennen DG, Schellander K, Wimmers K. Candidate gene markers for sperm quality and fertility of boar. Anim Reprod Science. (2006) 92:349–63. doi: 10.1016/j.anireprosci.2005.05.023
120. Lin CL, Jennen DG, Ponsuksili S, Tholen E, Tesfaye D, Schellander K, et al. Haplotype analysis of β-actin gene for its association with sperm quality and boar fertility. J Anim Breed Genet. (2006) 123:384–8. doi: 10.1111/j.1439-0388.2006.00622.x
121. Lin C, Tholen E, Jennen D, Ponsuksili S, Schellander K, Wimmers K. Evidence for effects of testis and epididymis expressed genes on sperm quality and boar fertility traits. Reprod Domest Animals. (2006) 41:538–43. doi: 10.1111/j.1439-0531.2006.00710.x
122. Kaewmala K, Uddin MJ, Cinar MU, Große-Brinkhaus C, Jonas E, Tesfaye D, et al. Investigation into association and expression of PLCz and COX-2 as candidate genes for boar sperm quality and fertility. Reprod Domest Animals. (2012) 47:213–23. doi: 10.1111/j.1439-0531.2011.01831.x
123. Kaewmala K, Uddin MJ, Cinar MU, Große-Brinkhaus C, Jonas E, Tesfaye D, et al. Association study and expression analysis of CD9 as candidate gene for boar sperm quality and fertility traits. Anim Reprod Science. (2011) 125:170–9. doi: 10.1016/j.anireprosci.2011.02.017
124. Gunawan A, Cinar MU, Uddin MJ, Kaewmala K, Tesfaye D, Phatsara C, et al. Investigation on association and expression of ESR2 as a candidate gene for boar sperm quality and fertility. Reprod Domest Animals. (2012) 47:782–90. doi: 10.1111/j.1439-0531.2011.01968.x
125. Gunawan A, Kaewmala K, Uddin MJ, Cinar MU, Tesfaye D, Phatsara C, et al. Association study and expression analysis of porcine ESR1 as a candidate gene for boar fertility and sperm quality. Anim Reprod Science. (2011) 128:11–21. doi: 10.1016/j.anireprosci.2011.08.008
126. Sironen A, Uimari P, Venhoranta H, Andersson M, Vilkki J. An exonic insertion within Tex14 gene causes spermatogenic arrest in pigs. BMC Genomics. (2011) 12:1. doi: 10.1186/1471-2164-12-591
127. Sironen A, Uimari P, Nagy S, Paku S, Andersson M, Vilkki J. Knobbed acrosome defect is associated with a region containing the genes STK17b and HECW2 on porcine chromosome 15. BMC Genomics. (2010) 11:1. doi: 10.1186/1471-2164-11-699
128. Huang SY, Song HL, Lin EC, Lee WC, Chiang JC, Tsou HL. Association of polymorphisms in epidermal growth factor, prostaglandin-endoperoxide synthase 2 and prolactin receptor genes with semen quality in Duroc boars. Asian-Australasian J Anim Sci. (2006) 19:793–8. doi: 10.5713/ajas.2006.793
129. Ma C, Li J, Tao H, Lei B, Li Y, Tong K, et al. Discovery of two potential DAZL gene markers for sperm quality in boars by population association studies. Anim Reprod Science. (2013) 143:97–101. doi: 10.1016/j.anireprosci.2013.10.002
130. Cho ES, Sa SJ, Kim KH, Lee MJ, Ko JH, Kim YJ, et al. Association study analysis of cluster-of-differentiation antigen 9 (CD9) gene polymorphism (g. 358A > T) for Duroc boar post-thawed semen motility and kinematic characteristics. J Embryo Transfer. (2015) 30:109–14. doi: 10.12750/JET.2015.30.2.109
131. Cho ES, Kim KH, Woo JS, Lee MJ, Ko JH, Kim YJ, et al. Association with post-thawed semen motility and kinematic characteristics of g. 35756T > C on estrogen receptor 1 (ESR1) gene in Duroc pigs. J Embryo Transfer. (2015) 30:143–7. doi: 10.12750/JET.2015.30.3.143
132. Sa SJ, Lee MJ, Kim KH, Woo JS, Ko JH, Kim YJ, et al. Association study analysis of phospholipase C zeta (PLCz) gene polymorphism (g. 158T > C) for Duroc boar post-thawed semen motility and kinematic characteristics. J Embryo Transfer. (2015) 30:137–42. doi: 10.12750/JET.2015.30.3.137
133. van Son M, Tremoen NH, Gaustad AH, Myromslien FD, Våge DI, Stenseth EB, et al. RNA sequencing reveals candidate genes and polymorphisms related to sperm DNA integrity in testis tissue from boars. BMC Veterinary Res. (2017) 13:1–3. doi: 10.1186/s12917-017-1279-x
134. Fraser L, Brym P, Pareek CS, Mogielnicka-Brzozowska M, Jastrzębski JP, Wasilewska-Sakowska K, et al. Transcriptome analysis of boar spermatozoa with different freezability using RNA-Seq. Theriogenology. (2020) 142:400–13. doi: 10.1016/j.theriogenology.2019.11.001
135. Diniz DB, Lopes MS, Broekhuijse ML, Lopes PS, Harlizius B, Guimarães SE, et al. A genome-wide association study reveals a novel candidate gene for sperm motility in pigs. Anim Reprod Science. (2014) 151:201–7. doi: 10.1016/j.anireprosci.2014.10.014
136. Marques DB, Bastiaansen JW, Broekhuijse ML, Lopes MS, Knol EF, Harlizius B, et al. Weighted single-step GWAS and gene network analysis reveal new candidate genes for semen traits in pigs. Genet Selection Evolution. (2018) 50:1–4. doi: 10.1186/s12711-018-0412-z
137. Xu M, Qin Y, Qu J, Lu C, Wang Y, Wu W, et al. Evaluation of five candidate genes from GWAS for association with oligozoospermia in a Han Chinese population. PloS One. (2013) 8:e80374. doi: 10.1371/journal.pone.0080374
138. Gao N, Chen Y, Liu X, Zhao Y, Zhu L, Liu A, et al. Weighted single-step GWAS identified candidate genes associated with semen traits in a Duroc boar population. BMC Genomics. (2019) 20:1. doi: 10.1186/s12864-019-6164-5
139. Zhao Y, Gao N, Li X, El-Ashram S, Wang Z, Zhu L, et al. Identifying candidate genes associated with sperm morphology abnormalities using weighted single-step GWAS in a Duroc boar population. Theriogenology. (2020) 141:9–15. doi: 10.1016/j.theriogenology.2019.08.031
140. Gòdia M, Reverter A, González-Prendes R, Ramayo-Caldas Y, Castelló A, Rodríguez-Gil JE, et al. A systems biology framework integrating GWAS and RNA-Seq to shed light on the molecular basis of sperm quality in swine. Genet Selection Evolution. (2020) 52:72. doi: 10.1186/s12711-020-00592-0
141. Zhao Y, Zhang L, Wang L, Zhang J, Shen W, Ma Y, et al. Identification and analysis of genes related to testicular size in 14-day-old piglets. Animals. (2024) 14:172. doi: 10.3390/ani14010172
142. Bu Y, Wang P, Li S, Li L, Zhang S, Wei H. Semen protein CRISP3 promotes reproductive performance of boars through immunomodulation. Int J Mol Sci. (2024) 25:2264. doi: 10.3390/ijms25042264
143. Li Y, Wang Y, An T, Tang Y, Shi M, Zhang W, et al. Non-thermal plasma promotes boar sperm quality through increasing AMPK methylation. Int J Biol Macromolecules. (2024) 257:128768. doi: 10.1016/j.ijbiomac.2023.128768
144. Ren H, Zhang Y, Bi Y, Wang H, Fang G, Zhao P. Target silencing of porcine SPAG6 and PPP1CC by shRNA attenuated sperm motility. Theriogenology. (2024) 219:138–46. doi: 10.1016/j.theriogenology.2024.02.030
145. Wang T, Feng Y, Chen D, Bai R, Tang J, Zhao Y, et al. Nonsynonymous SNPs within C7H15orf39 and NOS2 are associated with boar semen quality. Anim Biotechnol. (2023) 34:2106–10. doi: 10.1080/10495398.2022.2077213
146. Tang Y, Zhang B, Shi H, Yan Z, Wang P, Yang Q, et al. Molecular characterization, expression patterns and cellular localization of BCAS2 gene in male Hezuo pig. PeerJ. (2023) 11:e16341. doi: 10.7717/peerj.16341
147. Tang Y, Zhang B, Shi H, Yan Z, Wang P, Yang Q, et al. Cloning, expression analysis and localization of DAZl gene implicated in germ cell development of male Hezuo pig. Anim Biotechnol. (2023) 34:4000–14. doi: 10.1080/10495398.2023.2249953
148. Shi H, Yan Z, Du H, Tang Y, Song K, Yang Q, et al. Regulatory effects of the Kiss1 gene in the testis on puberty and reproduction in Hezuo and Landrance boars. Int J Mol Sci. (2023) 24:16700. doi: 10.3390/ijms242316700
149. Bai R, Chen D, Xiong H, Song H, Wang T, Yang X, et al. SPAG6 c. 900 T > C affects boar semen quality and blood-testis barrier function by creating a new splice acceptor site. Anim Genet. (2023) 54:446–56. doi: 10.1111/age.13330
150. Mańkowska A, Gilun P, Zasiadczyk Ł, Sobiech P, Fraser L. Expression of TXNRD1, HSPA4L and ATP1B1 genes associated with the freezability of boar sperm. Int J Mol Sci. (2022) 23:9320. doi: 10.3390/ijms23169320
151. Luc DD, Bo HX, Thinh NH, Thanh NC, Manh TX, Van Hung N, et al. Effect of ESR, FSHB and PRLR genes on sperm traits of Landrace and Yorkshire boars in the tropical environmental conditions of Vietnam. Indian J Anim Res. (2022) 56:129–34. doi: 10.18805/IJAR.B-1278
152. Mańkowska A, Brym P, Sobiech P, Fraser L. Promoter polymorphisms in STK35 and IFT27 genes and their associations with boar sperm freezability. Theriogenology. (2022) 189:199–208. doi: 10.1016/j.theriogenology.2022.06.023
153. Pang WK, Son JH, Ryu DY, Rahman MS, Park YJ, Pang MG. Heat shock protein family D member 1 in boar spermatozoa is strongly related to the litter size of inseminated sows. J Anim Sci Biotechnol. (2022) 13:42. doi: 10.1186/s40104-022-00689-0
154. Mei Q, Fu C, Sahana G, Chen Y, Yin L, Miao Y, et al. Identification of new semen trait-related candidate genes in Duroc boars through genome-wide association and weighted gene co-expression network analyses. J Anim Sci. (2021) 99:skab188. doi: 10.1093/jas/skab188
155. Ablondi M, Gòdia M, Rodriguez-Gil JE, Sánchez A, Clop A. Characterisation of sperm piRNAs and their correlation with semen quality traits in swine. Anim Genet. (2021) 52:114–20. doi: 10.1111/age.13022
156. Brym P, Wasilewska-Sakowska K, Mogielnicka-Brzozowska M, Mańkowska A, Paukszto Ł, Pareek CS, et al. Gene promoter polymorphisms in boar spermatozoa differing in freezability. Theriogenology. (2021) 166:112–23. doi: 10.1016/j.theriogenology.2021.02.018
157. Mańkowska A, Brym P, Paukszto Ł, Jastrzębski JP, Fraser L. Gene polymorphisms in boar spermatozoa and their association with post-thaw semen quality. Int J Mol Sci. (2020) 21:1902. doi: 10.3390/ijms21051902
158. Zhao L, Ma C, Song H, Feng Y, Li Y, Xia X, et al. H2AFZ, RNF4 and NR4A1 loci are associated with boar semen quality by population association studies. Anim Biotechnol. (2019) 30:311–6. doi: 10.1080/10495398.2018.1521825
159. Sieme H, Distl O. Genomics and fertility in stallions. J Equine Vet Sci. (2012) 32:467–70. doi: 10.1016/j.jevs.2012.06.016
160. Suliman Y, Becker F, Wimmers K. Implication of transcriptome profiling of spermatozoa for stallion fertility. Reprod Fertil. Dev. (2018) 30:1087–98. doi: 10.1071/RD17188
161. Laseca N, Anaya G, Peña Z, Pirosanto Y, Molina A, Demyda Peyrás S. Impaired reproductive function in equines: From genetics to genomics. Animals. (2021) 11:393. doi: 10.3390/ani11020393
162. Schrimpf R, Dierks C, Martinsson G, Sieme H, Distl O. Genome-wide association study identifies phospholipase C zeta 1 (PLCz1) as a stallion fertility locus in Hanoverian warmblood horses. PloS One. (2014) 9:e109675. doi: 10.1371/journal.pone.0109675
163. Raudsepp T, McCue ME, Das PJ, Dobson L, Vishnoi M, Fritz KL, et al. Genome-wide association study implicates testis-sperm specific FKBP6 as a susceptibility locus for impaired acrosome reaction in stallions. PloS Genet. (2012) 8:e1003139. doi: 10.1371/journal.pgen.1003139
164. Restrepo G, Rojano B, Usuga A. Relationship of cysteine-rich secretory protein-3 gene and protein with semen quality in stallions. Reprod Domest Animals. (2019) 54:39–45. doi: 10.1111/rda.2019.54.issue-1
165. Usuga A, Rojano BA, Restrepo G. Association of the cysteine-rich secretory protein-3 (CRISP-3) and some of its polymorphisms with the quality of cryopreserved stallion semen. Reproduction Fertility Dev. (2018) 30:563–9. doi: 10.1071/RD17044
166. Gottschalk M, Metzger J, Martinsson G, Sieme H, Distl O. Genome-wide association study for semen quality traits in German Warmblood stallions. Anim Reprod Science. (2016) 171:81–6. doi: 10.1016/j.anireprosci.2016.06.002
167. Hamann H, Jude R, Sieme H, Mertens U, Töpfer-Petersen E, Distl O, et al. A polymorphism within the equine CRISP3 gene is associated with stallion fertility in Hanoverian Warmblood horses. Anim Genet. (2007) 38:259–64. doi: 10.1111/j.1365-2052.2007.01594.x
168. Giesecke K, Hamann H, Stock K, Klewitz J, Martinsson G, Distl O, et al. Evaluation of ACE, SP17, and FSHB as candidates for stallion fertility in Hanoverian warmblood horses. Anim. Reprod Sci. (2011) 126:200–6. doi: 10.1016/j.anireprosci.2011.05.007
169. Giesecke K, Hamann H, Sieme H, Distl O. Evaluation of prolactin receptor (PRLR) as candidate gene for male fertility in Hanoverian warmblood horses. Reprod Domest. Anim. (2010) 45:e124–30. doi: 10.1111/j.1439-0531.2009.01533.x
170. Giesecke K, Hamann H, Stock KF, Woehlke A, Sieme H, Distl O. Evaluation of SPATA1-associated markers for stallion fertility. Anim Genet. (2009) 40:359–65. doi: 10.1111/j.1365-2052.2008.01844.x
171. Ball BA, Gravance CG, Wessel MT, Sabeur K. Activity of angiotensin-converting enzyme (ACE) in reproductive tissues of the stallion and effects of angiotensin II on sperm motility. Theriogenology. (2003) 59:901–14. doi: 10.1016/S0093-691X(02)01127-5
172. Pérez-Rico A, Crespo F, Sanmartín ML, De Santiago A, Vega-Pla JL. Determining ACTB, ATP5B and RPL32 as optimal reference genes for quantitative RT-PCR studies of cryopreserved stallion semen. Anim Reprod Science. (2014) 149:204–11. doi: 10.1016/j.anireprosci.2014.08.007
173. Blommaert D, Sergeant N, Delehedde M, Jouy N, Mitchell V, Franck T, et al. Expression, localization, and concentration of A-kinase anchor protein 4 (AKAP4) and its precursor (proAKAP4) in equine semen: Promising marker correlated to the total and progressive motility in thawed spermatozoa. Theriogenology. (2019) 131:52–60. doi: 10.1016/j.theriogenology.2019.03.011
174. Gmel AI, Burger D, Neuditschko M. A novel QTL and a candidate gene are associated with the progressive motility of Franches-Montagnes stallion spermatozoa after thaw. Genes. (2021) 12:1501. doi: 10.3390/genes12101501
175. Sherman BT, Hao M, Qiu J, Jiao X, Baseler MW, Lane HC, et al. DAVID: a web server for functional enrichment analysis and functional annotation of gene lists (2021 update). Nucleic Acids Res. (2022) 215:W216–W221. doi: 10.1093/nar/gkac194
176. Huang DW, Sherman BT, Lempicki RA. Systematic and integrative analysis of large gene lists using DAVID Bioinformatics Resources. Nat Protoc. (2009) 4:44–57. doi: 10.1038/nprot.2008.211
177. Zhang GM, Zhang TT, An SY, El-Samahy MA, Yang H, Wan YJ, et al. Expression of Hippo signaling pathway components in Hu sheep male reproductive tract and spermatozoa. Theriogenology. (2019) 126:239–48. doi: 10.1016/j.theriogenology.2018.12.029
178. Shi WH, Zhou ZY, Ye MJ, Qin NX, Jiang ZR, Zhou XY, et al. Sperm morphological abnormalities in autosomal dominant polycystic kidney disease are associated with the Hippo signaling pathway via PC1. Front Endocrinol. (2023) 14:1130536. doi: 10.3389/fendo.2023.1130536
179. Nafchi HG, Azizi Y, Halvaei I. The role of growth factors in human sperm parameters: A review of in vitro studies. Int J Reprod Biomed. (2022) 20:807–18. doi: 10.18502/ijrm.v20i10.12265
180. Sánchez-Luengo S, Fernández PJ, Romeu A. Insulin growth factors may be implicated in human sperm capacitation. Fertil. Steril. (2005) 83:1064–6. doi: 10.1016/j.fertnstert.2004.12.003
181. O’Leary S, Armstrong DT, Robertson SA. Transforming growth factor-β (TGFβ) in porcine seminal plasma. Reprod Fertil. Dev. (2011) 23:748–58. doi: 10.1071/RD11001
182. Padilla L, Barranco I, Martínez-Hernández J, Parra A, Parrilla I, Pastor LM, et al. Extracellular vesicles would be involved in the release and delivery of seminal TGF-β isoforms in pigs. Front Vet Sci. (2023) 10:1102049. doi: 10.3389/fvets.2023.1102049
183. Tesarik J, Mendoza-Tesarik R. Cyclic adenosine monophosphate: a central player in gamete development and fertilization, and possible target for infertility therapies. Int J Mol Sci. (2022) 23:15068. doi: 10.3390/ijms232315068
184. Qu X, Han Y, Chen X, Lv Y, Zhang Y, Cao L, et al. Inhibition of 26S proteasome enhances AKAP3-mediated cAMP-PKA signaling during boar sperm capacitation. Anim. Reprod Sci. (2022) 247:107079. doi: 10.1016/j.anireprosci.2022.107079
185. Lasko J, Schlingmann K, Klocke A, Mengel GA, Turner R. Calcium/calmodulin and cAMP/protein kinase-A pathways regulate sperm motility in the stallion. Anim. Reprod Sci. (2012) 132:169–77. doi: 10.1016/j.anireprosci.2012.05.007
186. He Q, Gao F, Wu S, Wang S, Xu Z, Xu X, et al. Alkaline dilution alters sperm motility in dairy goat by affecting sAC/cAMP/PKA pathway activity. Int J Mol Sci. (2023) 24:1771. doi: 10.3390/ijms24021771
187. Ajayi AF, Akhigbe RE. In vivo exposure to codeine induces reproductive toxicity: role of HER2 and p53/Bcl-2 signaling pathway. Heliyon. (2020) 6:e05458. doi: 10.1016/j.heliyon.2020.e05589
188. Liang K, Yao L, Wang S, Zheng L, Qian Z, Ge Y, et al. miR-125a-5p increases cellular DNA damage of aging males and perturbs stage-specific embryo development via Rbm38-p53 signaling. Aging Cell. (2021) 20:e13508. doi: 10.1111/acel.v20.12
189. Brauns AK, Heine M, Tödter K, Baumgart-Vogt E, Lüers GH, Schumacher U. A defect in the peroxisomal biogenesis in germ cells induces a spermatogenic arrest at the round spermatid stage in mice. Sci Rep. (2019) 9:9553. doi: 10.1038/s41598-019-45991-6
Keywords: livestock, selective breeding, genetic markers, semen quality traits, reproductive efficiency
Citation: Khan MZ, Chen W, Naz S, Liu X, Liang H, Chen Y, Kou X, Liu Y, Ashraf I, Han Y, Peng Y, Wang C and Zahoor M (2024) Determinant genetic markers of semen quality in livestock. Front. Endocrinol. 15:1456305. doi: 10.3389/fendo.2024.1456305
Received: 28 June 2024; Accepted: 09 September 2024;
Published: 04 October 2024.
Edited by:
Sellappan Selvaraju, National Institute of Animal Nutrition and Physiology (ICAR), IndiaReviewed by:
Zhihua Ju, Shandong Academy of Agricultural Sciences, ChinaCopyright © 2024 Khan, Chen, Naz, Liu, Liang, Chen, Kou, Liu, Ashraf, Han, Peng, Wang and Zahoor. This is an open-access article distributed under the terms of the Creative Commons Attribution License (CC BY). The use, distribution or reproduction in other forums is permitted, provided the original author(s) and the copyright owner(s) are credited and that the original publication in this journal is cited, in accordance with accepted academic practice. No use, distribution or reproduction is permitted which does not comply with these terms.
*Correspondence: Changfa Wang, d2FuZ2NoYW5nZmFAbGN1LmVkdS5jbg==; Muhammad Zahoor Khan, emFob29ya2hhdHRhazkxQHlhaG9vLmNvbQ==; Muhammad Zahoor, bXVoYW1tYWQuemFob29yQG1lZGlzaW4udWlvLm5v
Disclaimer: All claims expressed in this article are solely those of the authors and do not necessarily represent those of their affiliated organizations, or those of the publisher, the editors and the reviewers. Any product that may be evaluated in this article or claim that may be made by its manufacturer is not guaranteed or endorsed by the publisher.
Research integrity at Frontiers
Learn more about the work of our research integrity team to safeguard the quality of each article we publish.