- Department of Gastroenterology, Shengjing Hospital of China Medical University, Shenyang, China
Nonalcoholic fatty liver disease (NAFLD) is characterized by over 5% hepatic fat accumulation without secondary causes. The prevalence of NAFLD has escalated in recent years due to shifts in dietary patterns and socioeconomic status, making it the most prevalent chronic liver disease and a significant public health concern globally. Serum uric acid (SUA) serves as the end product of purine metabolism in the body and is intricately linked to metabolic syndrome. Elevated SUA levels have been identified as an independent risk factor for the incidence and progression of NAFLD. This paper reviews the relationship between SUA and NAFLD, the underlying mechanisms of SUA involved in NAFLD, and the potential benefits of SUA-lowering therapy in treating NAFLD. The aim is to raise awareness of SUA management in patients with NAFLD, and to encourage further investigation into pharmacological interventions in this area.
1 Introduction
Nonalcoholic fatty liver disease (NAFLD) is a clinicopathological condition characterized by over-accumulation of fat in the liver, defined as steatosis in over 5% of hepatocytes, in the absence of alcohol consumption and other definitive factors causing liver damage (1, 2). The prevalence of NAFLD has been steadily rising due to changes in lifestyle and dietary patterns, making it the most common chronic liver ailment globally (3, 4). The global prevalence of NAFLD is 30.1% (5), with Asian countries reporting a prevalence of 29.6% (6). NAFLD not only has the potential to progress to cirrhosis and liver cancer but is also linked to cardiovascular and cerebrovascular diseases, peripheral vascular diseases, diabetes mellitus, cholelithiasis, and other conditions, as well as an increased risk of various malignant tumors such as colorectal, breast, and pancreatic cancers (7–9). NAFLD is a serious threat to the health of human beings, and it has become a major global concern (10, 11). Given the widespread use of the term NAFLD in existing literature, despite recent proposals to rename it as metabolic-associated fatty liver disease (MAFLD) (12), this review will continue to refer to it as NAFLD.
Uric acid (UA) is the final product of purine compound breakdown in the liver (13), with xanthine oxidase (XO) playing a crucial role in its production by catalyzing the oxidation from hypoxanthine to xanthine and then to UA (14). Abnormalities in UA metabolism are associated with several chronic systemic diseases like hypertension, atherosclerosis, diabetes mellitus, and dyslipidemia (15–17). The relationship between serum uric acid (SUA) levels and NAFLD severity has gained attention in recent years, with SUA being a significant factor independently correlated with the severity of NAFLD independently of other metabolic markers (18). Studies have reported a 21% increase in NAFLD risk for every 1 mg/dL rise in SUA levels (19), with hyperuricemia further elevating the risk of significant liver fibrosis in NAFLD patients (20).
In order to gain a deeper comprehension of the correlation between SUA and NAFLD, this review aims to synthesize current research findings to aid in the management of SUA levels in NAFLD patients.
2 Relationship between SUA and NAFLD
A Meta-analysis has shown a pooled odds ratio of 1.88 in NAFLD patients with higher SUA levels compared to those with lower levels, with SUA levels being associated with NAFLD across various subgroups regardless of study quality, study design, sample size, age, gender, or country (21). Elevated SUA levels are also linked to the severity and progression of NAFLD, with studies indicating a strong correlation between SUA levels and the degree of steatosis, inflammation of the lobules, cirrhosis development, and elevated liver enzymes in NAFLD patients (22, 23). While obesity is a known risk factor for NAFLD (24), increased SUA levels in non-obese individuals significantly heighten the risk of NAFLD, surpassing that in obese patients with normal SUA levels (25). Table 1 provides an overview of relevant studies on the relationship between SUA on NAFLD (18, 22, 25–61).
3 Underlying mechanisms of SUA involved in NAFLD
The pathogenesis of NAFLD has transitioned from the “two-hit” theory to the “multiple hit” hypothesis, which suggests that various factors collectively contribute to the development of NAFLD in genetically susceptible individuals (63, 64). These factors include steatosis, inflammation, oxidative stress (OS), metabolic dysfunction, and insulin resistance (IR) (63, 64). The precise mechanisms through which SUA is involved in NAFLD remain incompletely understood. SUA plays a role in the onset and progression of NAFLD through processes such as OS, inflammatory responses, disturbances in lipid metabolism, and IR, as shown in Figure 1.
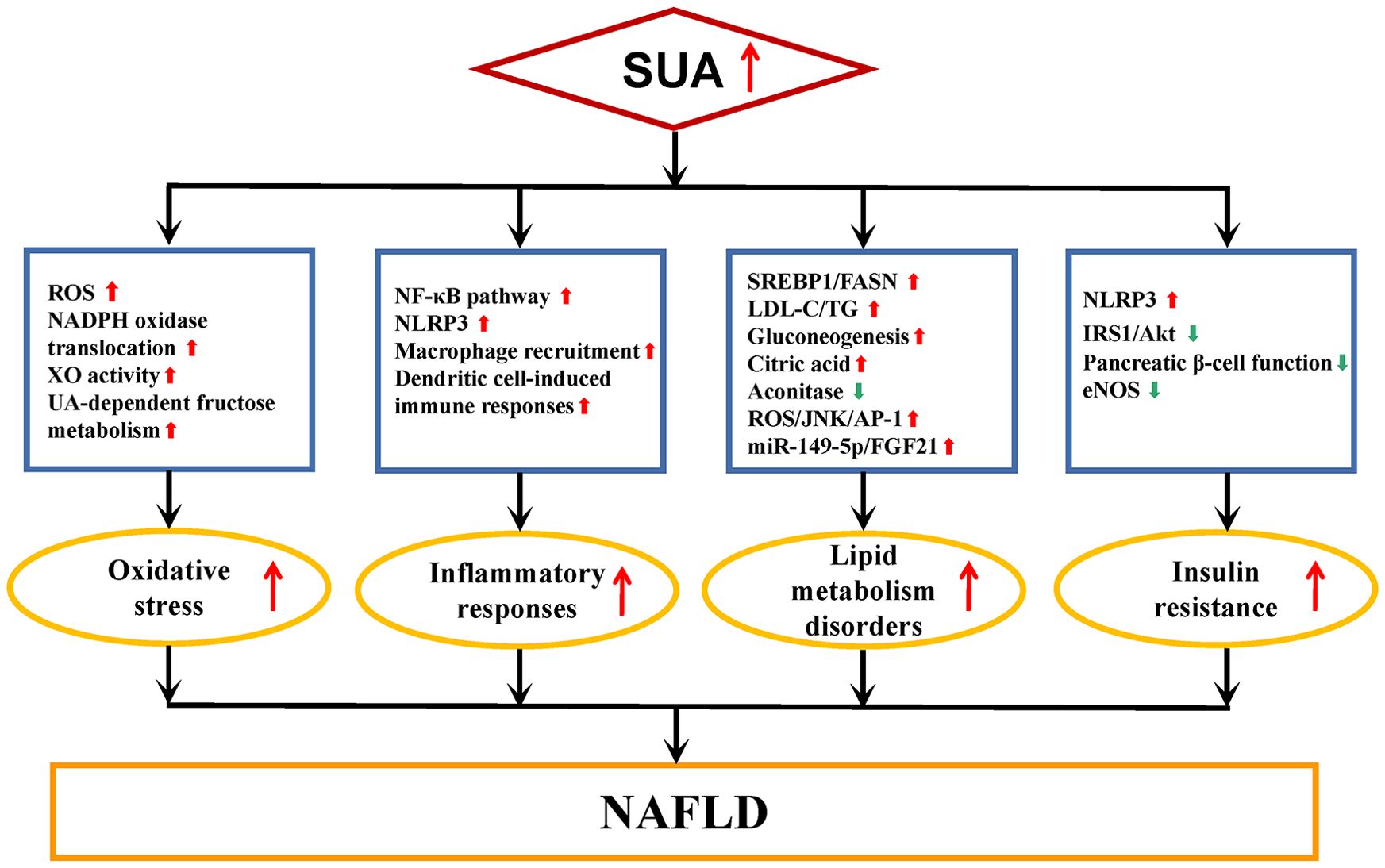
Figure 1. Underlying mechanisms of SUA involved in NAFLD. NAFLD, nonalcoholic fatty liver disease; SUA, serum uric acid; ROS, reactive oxygen species; UA, uric acid; NADPH, nicotinamide adenine dinucleotide phosphate; XO, xanthine oxidase; NF-κB, nuclear factor kappa-B; NLRP3, nucleotide-binding oligomerization domain-like receptor family pyrin domain containing 3; SREBP1, sterol regulatory element binding protein 1; FASN, fatty acid synthase; LDL-C, low-density lipoprotein cholesterol; TG, liver fat content; JNK, c-Jun N-terminal kinase; AP-1, activator protein-1; FGF21, fibroblast growth factors 21; IRS1, insulin receptor substrate 1; Akt, protein kinase B; eNOS, endothelial nitric oxide synthase. Red arrows, up-regulation of expression or enhanced activity. Green arrows, down-regulation of expression or reduced activity.
3.1 Oxidative stress
OS is an important etiopathogenesis of NAFLD (65). Elevated levels of reactive oxygen species (ROS) under OS can lead to dysfunction in mitochondria and endoplasmic reticulum, as well as reduced antioxidant defenses in liver cells, resulting in inflammation, cell death, and fibrosis during NAFLD progression (66). Hepatocytes exposed to UA has been linked to mitochondrial OS mediated by the translocation of nicotinamide adenine dinucleotide phosphate (NADPH) oxidase (67). UA also enhances fat synthesis in hepatocytes by facilitating the transfer of NADPH oxidase subunit 4 to mitochondria, thereby increasing superoxide production (68). Xanthine oxidase (XO), the rate-limiting enzyme enzyme for UA production, generates ROS during catalyzing the oxidative hydroxylation of hypoxanthine and xanthine to produce UA (69). SUA levels can serve as an indicator of XO activity in NAFLD (70). In addition, 70% of fructose in the human body is metabolized by the liver, and fructose-rich diets can exacerbate NAFLD (71). Fructose can exacerbate NAFLD by promoting hepatic fat accumulation through both direct triglyceride (TG) synthesis from fructose metabolism and an uric acid-dependent pathway via mitochondrial OS (72).
3.2 Inflammatory responses
Inflammation is a fundamental component of NAFLD pathophysiology and is present throughout the disease progression (73). UA is a potent inflammatory inducer, capable of upregulating the expression of various inflammation markers in a dose-dependent manner (74). It may also trigger the activation of pro-inflammatory signaling pathways, such as nuclear factor kappa-B, leading to the expression of inflammatory molecules and exacerbating the inflammatory response in hepatocytes (74). The nucleotide-binding oligomerization domain-like receptor family pyrin domain containing 3 (NLRP3) inflammasome, a multiprotein complex involved in recognizing pathogens and molecular patterns, plays a crucial role in obesity, IR, and NAFLD progression (75–77). UA, as an injury-related molecular pattern, stimulates macrophage recruitment (78, 79), which then expresses NLRP3 inflammasome and generate significant quantities of IL-1β (80, 81), leading to chronic inflammation in hepatocytes. UA also exacerbate hepatic inflammation by inducing immune responses through dendritic cells (82).
3.3 Lipid metabolism
The onset of NAFLD is linked to disruptions in lipid metabolism (83). UA plays a role in regulating fatty acid synthase (FASN) through the sterol regulatory element binding protein 1 (SREBP1) signaling pathway, resulting in the accumulation of free fatty acids and compromised energy metabolism in HepG2 cells (84). Additionally, UA triggers the activation of SREBP-1c via endoplasmic reticulum stress, promoting lipid synthesis in hepatocytes, which in turn exacerbates intracellular fat accumulation and lipid degeneration in hepatocytes (85). UA exacerbates lipid metabolism disorders in NAFLD by oxidatively modifying low-density lipoprotein cholesterol and TG synthesis (86). Furthermore, UA facilitates the conversion of fructose to fructose 1-phosphate by activating fructokinase in hepatocytes, leading to fat accumulation through gluconeogenesis (87). Mitochondrial OS induced by UA inhibits aconitase in the Krebs cycle, causing citric acid buildup and stimulating enzymes involved in fatty acid synthesis, ultimately promoting de novo lipogenesis (72). UA significantly up-regulates the expression of miR-149-5p in hepatocytes, and FGF21, a downstream target of miR-149-5p and closely related to lipid metabolism, whose deficiency can lead to hepatic steatosis, so that uric acid aggravated hepatic fat accumulation through the miR-149-5p/FGF21 axis (67). UA upregulates the expression of lipogenic genes in hepatocytes via the ROS/JNK//AP-1 pathway, increasing triglyceride levels in HepG2 cells and leading to the accumulation of intracellular fat in hepatocytes (88).
3.4 Insulin resistance
NAFLD is closely linked to IR, with some considering NAFLD as a hepatic manifestation of IR (89, 90). Obesity is associated with the development of chronic low-grade inflammation, a condition exacerbated by the expansion of adipose tissue (91, 92). The inflammatory characteristics of adipose tissue enhance cytokine production, which in turn contributes to the development of IR (93, 94). In the state of IR, there is an upregulation of lipolysis in adipose tissue, resulting in an increased influx of free fatty acids to the liver (95). Concurrently, hyperinsulinemia stimulates lipogenesis in hepatocytes (96). These metabolic alterations culminate in lipid accumulation within the liver, leading to an increase in intracellular lipid peroxidation products and cytotoxic agents (97). Ultimately, these processes contribute to the onset and progression of NAFLD.The concentration of SUA was found to be independently related with IR (98). UA can inhibit insulin signaling pathways and induce IR by various mechanisms, including activation of the NLRP3 inflammasome and inhibition of IRS1/Akt pathway (72, 99). Elevated SUA levels cause the deposition of urate crystals in pancreatic islets, impairing pancreatic β-cell function and worsening IR (100). Reduced activity of endothelial nitric oxide synthase is also implicated in increased IR in individuals with hyperuricemia (17). Through the aforementioned mechanisms, UA intensifies the level of IR within the body and facilitates the advancement of NAFLD.
4 Potential benefits of SUA-lowering therapy in treating NAFLD
Despite the increasing global prevalence of NAFLD, there remain no FDA-approved pharmacological treatments specifically designed to address this condition, largely due to its intricate pathogenesis and multifactorial nature (101). Currently, lifestyle modifications, including substantial weight loss achieved through a low-calorie diet and increased physical activity, are regarded as the primary interventions for both the prevention and management of NAFLD, as weight reduction is correlated with a decrease in liver fat and may facilitate the reversal of disease progression (102). Bariatric surgery has the potential to decrease hepatic steatosis in obese patients with NAFLD (103). Nevertheless, it is important to note that NAFLD is not considered a valid indication for bariatric surgery (104). In terms of pharmacotherapy, the European and American Association for the Study of the Liver recommends the administration of vitamin E and pioglitazone exclusively for select patients diagnosed with NAFLD (105). More current research hotspots regarding therapeutic agents for NAFLD are mainly focused on drug selection against different metabolic targets (106). Given the significant relationship between SUA levels and NAFLD development, researchers have now recognized that SUA-lowering therapy may have a valuable contribution for improving NAFLD outcomes. XO inhibitors like febuxostat and allopurinol are commonly used to lower SUA levels and have shown promise in improving NAFLD in various studies.
Allopurinol, when administered at a daily dose of 100 mg to patients with hyperuricemia, has been found to lead to a significant reduction in the hepatic controlled attenuation parameter score after a three-month period (107). This medication has demonstrated the ability to decrease TG content in HepG2 cells and in the livers of NAFLD mice by inhibiting XO activity (108, 109). Moreover, allopurinol has shown efficacy in reducing histopathological scores and levels of interleukin-1 (IL-1) and IL-2 immunoexpression in the livers of NAFLD rats (110). By inhibiting NRLP3 inflammasome activation, allopurinol has been observed to mitigate hepatic steatosis and IR by lowering SUA levels (78). In diabetic rats, allopurinol has been found to improve hepatic OS and liver injury through the activation of the Nrf2/p62 pathway (111), as well as to reduce hepatic inflammation and lipid accumulation by decreasing hepatic thioredoxin levels (112). Additionally, allopurinol has been demonstrated to enhance fatty acid beta-oxidation in mouse livers and alleviate high fructose diet-induced hepatic steatosis in diabetic rats by modulating inflammation, lipid metabolism, and endoplasmic reticulum stress pathways (113, 114).
On the other hand, febuxostat has demonstrated a more favorable hepatic safety profile in gout patients with NAFLD (115). Treatment with febuxostat for 24 weeks has resulted in reduced SUA levels, as well as decreased levels of aspartate aminotransferase and alanine aminotransferase in NAFLD patients with hyperuricemia (116). In a nonalcoholic steatohepatitis (NASH) mouse model, febuxostat significantly lowered hepatic XO activity and UA levels, leading to improvements in IR, lipid peroxidation, and the accumulation of classically activated M1-like macrophages in the liver (116). Furthermore, febuxostat has been shown to reduce fat accumulation and ROS in HepG2 cells and NASH mice by downregulating the expression of NLRP3/caspase-1/IL-18/IL-1β and improving IR (117). Administration of febuxostat has also been found to normalize fatty acid oxidation-related genes, collagen deposition, fibrotic changes, lipid peroxidation, and inflammatory cytokine expressions in NASH mice (118).
While both XO inhibitors demonstrated comparable effects in lowering UA levels in the bloodstream, febuxostat exhibited a significant reduction in hepatic UA levels and XO activity in a NASH model in mice, a response not observed with allopurinol (116). This decrease in hepatic UA levels and XO activity was associated with a more pronounced prevention of specific NASH characteristics, including IR, lipid peroxidation, the aggregation of classically activated M1-like macrophages, and hepatic inflammation (116). These findings suggest that febuxostat may possess greater potential for ameliorating NAFLD in patients suffering from hyperuricemia.
5 Limitations
Currently, although advancements have been made in understanding the relationship between SUA levels and NAFLD and proposed a new direction and goal for solving the multifactorial problem of fatty liver, this area of research still faces several limitations. There is a pressing need for more fundamental experimental studies to elucidate the mechanisms through which SUA influences the development of NAFLD, particularly those mechanisms that are directly implicated in the pathogenesis of NAFLD, and the strengths and potential limitations of SUA and its direct association with OS. Observational studies investigating the effects of SUA-lowering therapies on NAFLD have primarily been conducted in preclinical settings or among specific populations with hyperuricemia. This is largely attributable to the insufficient recognition and valuation of SUA-lowering agents in clinical practice for the management of NAFLD, and SUA-lowering therapies on NAFLD is necessitated further investigation into their efficacy and safety, while also focusing on its effects on body weight, glucose and lipid metabolism, and liver tissue pathology. Furthermore, there is a notable absence of research conclusions regarding the use of SUA-lowering therapies for the prevention of NAFLD, as well as the impact of such treatments on NAFLD population without hyperuricemia. To address these gaps, more extensive, long-term, multi-center clinical studies are required to assess the potential benefits of these interventions.
6 Conclusion
In summary, there is a correlation between SUA and NAFLD, with SUA potentially exacerbating NAFLD through various pathways such as OS, inflammatory responses, lipid metabolism disturbances, and IR. Research has explored the potential benefits of SUA-lowering interventions in improving NAFLD. Given the global prevalence of NAFLD and the current limitations in treatment options, identifying novel therapeutic targets for NAFLD is imperative. Targeting XO inhibition as a SUA-lowering therapy may represent a promising avenue for future NAFLD management.
Author contributions
JF: Writing – original draft. DW: Writing – review & editing.
Funding
The author(s) declare financial support was received for the research, authorship, and/or publication of this article. This research was funded by Liaoning Provincial Science and Technology Programme Joint Fund for Doctoral Research Initiation Project, grant number 2023-BSBA-349.
Conflict of interest
The authors declare that the research was conducted in the absence of any commercial or financial relationships that could be construed as a potential conflict of interest.
Publisher’s note
All claims expressed in this article are solely those of the authors and do not necessarily represent those of their affiliated organizations, or those of the publisher, the editors and the reviewers. Any product that may be evaluated in this article, or claim that may be made by its manufacturer, is not guaranteed or endorsed by the publisher.
References
1. Lv H, Tao F, Peng L, Chen S, Ren Z, Chen J, et al. In Vitro Probiotic Properties of Bifidobacterium animalis subsp. lactis SF and Its Alleviating Effect on Non-Alcoholic Fatty Liver Disease. Nutrients. (2023) 15:1355. doi: 10.3390/nu15061355
2. Tsai CC, Chen YJ, Yu HR, Huang LT, Tain YL, Lin IC, et al. Long term N-acetylcysteine administration rescues liver steatosis via endoplasmic reticulum stress with unfolded protein response in mice. Lipids Health Dis. (2020) 19:105. doi: 10.1186/s12944-020-01274-y
3. Wang X, Ma B, Wen X, You H, Sheng C, Bu L, et al. Bone morphogenetic protein 4 alleviates nonalcoholic steatohepatitis by inhibiting hepatic ferroptosis. Cell Death Discovery. (2022) 8:234. doi: 10.1038/s41420-022-01011-7
4. Cong F, Zhu L, Deng L, Xue Q, Wang J. Correlation between nonalcoholic fatty liver disease and left ventricular diastolic dysfunction in non-obese adults: a cross-sectional study. BMC Gastroenterol. (2023) 23:90. doi: 10.1186/s12876-023-02708-4
5. Younossi ZM, Golabi P, Paik JM, Henry A, Van Dongen C, Henry L. The global epidemiology of nonalcoholic fatty liver disease (NAFLD) and nonalcoholic steatohepatitis (NASH): a systematic review. Hepatology. (2023) 77:1335–47. doi: 10.1097/HEP.0000000000000004
6. Li J, Zou B, Yeo YH, Feng Y, Xie X, Lee DH, et al. Prevalence, incidence, and outcome of non-alcoholic fatty liver disease in Asia, 1999-2019: a systematic review and meta-analysis. Lancet Gastroenterol Hepatol. (2019) 4:389–98. doi: 10.1016/S2468-1253(19)30039-1
7. Chhatwal J, Dalgic OO, Chen W, Samur S, Bethea ED, Xiao J, et al. Analysis of a simulation model to estimate long-term outcomes in patients with nonalcoholic fatty liver disease. JAMA Netw Open. (2022) 5:e2230426. doi: 10.1001/jamanetworkopen.2022.30426
8. Adams LA, Anstee QM, Tilg H, Targher G. Non-alcoholic fatty liver disease and its relationship with cardiovascular disease and other extrahepatic diseases. Gut. (2017) 66:1138–53. doi: 10.1136/gutjnl-2017-313884
9. Li AA, Ahmed A, Kim D. Extrahepatic manifestations of nonalcoholic fatty liver disease. Gut Liver. (2020) 14:168–78. doi: 10.5009/gnl19069
10. Chu X, Li L, Yan W, Ma H. 4-octyl itaconate prevents free fatty acid-induced lipid metabolism disorder through activating nrf2-AMPK signaling pathway in hepatocytes. Oxid Med Cell Longev. (2022) 2022:5180242. doi: 10.1155/2022/5180242
11. Lee J, Kim T, Yang H, Bae SH. Prevalence trends of non-alcoholic fatty liver disease among young men in Korea: A Korean military population-based cross-sectional study. Clin Mol Hepatol. (2022) 28:196–206. doi: 10.3350/cmh.2021.0371
12. Zeng Y, He H, An Z. Advance of serum biomarkers and combined diagnostic panels in nonalcoholic fatty liver disease. Dis Markers. (2022) 2022:1254014. doi: 10.1155/2022/1254014
13. Soliman MM, Nassan MA, Aldhahrani A, Althobaiti F, Mohamed WA. Molecular and histopathological study on the ameliorative impacts of petroselinum crispum and apium graveolens against experimental hyperuricemia. Sci Rep. (2020) 10:9512. doi: 10.1038/s41598-020-66205-4
14. Li X, Yan Z, Carlström M, Tian J, Zhang X, Zhang W, et al. Mangiferin ameliorates hyperuricemic nephropathy which is associated with downregulation of AQP2 and increased urinary uric acid excretion. Front Pharmacol. (2020) 11:49. doi: 10.3389/fphar.2020.00049
15. Li C, Hsieh MC, Chang SJ. Metabolic syndrome, diabetes, and hyperuricemia. Curr Opin Rheumatol. (2013) 25:210–6. doi: 10.1097/BOR.0b013e32835d951e
16. Katsiki N, Dimitriadis GD, Mikhailidis DP. Serum uric acid and diabetes: from pathophysiology to cardiovascular disease. Curr Pharm Des. (2021) 27:1941–51. doi: 10.2174/1381612827666210104124320
17. Sharaf El Din UAA, Salem MM, Abdulazim DO. Uric acid in the pathogenesis of metabolic, renal, and cardiovascular diseases: A review. J Adv Res. (2017) 8:537–48. doi: 10.1016/j.jare.2016.11.004
18. Sirota JC, McFann K, Targher G, Johnson RJ, Chonchol M, Jalal DI. Elevated serum uric acid levels are associated with non-alcoholic fatty liver disease independently of metabolic syndrome features in the United States: Liver ultrasound data from the National Health and Nutrition Examination Survey. Metabolism. (2013) 62:392–9. doi: 10.1016/j.metabol.2012.08.013
19. Yuan H, Yu C, Li X, Sun L, Zhu X, Zhao C, et al. Serum uric acid levels and risk of metabolic syndrome: A dose-response meta-analysis of prospective studies. J Clin Endocrinol Metab. (2015) 100:4198–207. doi: 10.1210/jc.2015-2527
20. Yen PC, Chou YT, Li CH, Sun ZJ, Wu CH, Chang YF, et al. Hyperuricemia Is Associated with Significant Liver Fibrosis in Subjects with Nonalcoholic Fatty Liver Disease, but Not in Subjects without It. J Clin Med. (2022) 11:1445. doi: 10.3390/jcm11051445
21. Sun Q, Zhang T, Manji L, Liu Y, Chang Q, Zhao Y, et al. Association between serum uric acid and non-alcoholic fatty liver disease: an updated systematic review and meta-analysis. Clin Epidemiol. (2023) 15:683–93. doi: 10.2147/CLEP.S403314
22. Huang Q, Yu J, Zhang X, Liu S, Ge Y. Association of the serum uric acid level with liver histology in biopsy-proven non-alcoholic fatty liver disease. BioMed Rep. (2016) 5:188–92. doi: 10.3892/br.2016.698
23. Afzali A, Weiss NS, Boyko EJ, Ioannou GN. Association between serum uric acid level and chronic liver disease in the United States. Hepatology. (2010) 52:578–89. doi: 10.1002/hep.23717
24. Polyzos SA, Kountouras J, Mantzoros CS. Obesity and nonalcoholic fatty liver disease: From pathophysiology to therapeutics. Metabolism. (2019) 92:82–97. doi: 10.1016/j.metabol.2018.11.014
25. Liu J, Xu C, Ying L, Zang S, Zhuang Z, Lv H, et al. Relationship of serum uric acid level with non-alcoholic fatty liver disease and its inflammation progression in non-obese adults. Hepatol Res. (2017) 47:E104–12. doi: 10.1111/hepr.12734
26. Ding Y, Tang Z, Wang M, Wang M, Zhang R, Zhang L, et al. Combining serum uric acid and fatty liver index to improve prediction quality of nonalcoholic fatty liver disease. Saudi J Gastroenterol. (2023) 29:191–8. doi: 10.4103/sjg.sjg_484_22
27. Park H, Park KY, Kim M, Park HK, Hwang HS. Association between serum uric acid level and non-alcoholic fatty liver disease in Koreans. Asian BioMed (Res Rev News). (2022) 16:15–22. doi: 10.2478/abm-2022-0003
28. He J, Ye J, Sun Y, Feng S, Chen Y, Zhong B. The additive values of the classification of higher serum uric acid levels as a diagnostic criteria for metabolic-associated fatty liver disease. Nutrients. (2022) 14:3587. doi: 10.3390/nu14173587
29. Wang M, Wang M, Zhang R, Zhang L, Ding Y, Tang Z, et al. A combined association of serum uric acid, alanine aminotransferase and waist circumference with non-alcoholic fatty liver disease: a community-based study. PeerJ. (2022) 10:e13022. doi: 10.7717/peerj.13022
30. Tang Y, Xu Y, Liu P, Liu C, Zhong R, Yu X, et al. No evidence for a causal link between serum uric acid and nonalcoholic fatty liver disease from the dongfeng-tongji cohort study. Oxid Med Cell Longev. (2022) 2022:6687626. doi: 10.1155/2022/6687626
31. Catanzaro R, Sciuto M, He F, Singh B, Marotta F. Non-alcoholic fatty liver disease: correlation with hyperuricemia in a European Mediterranean population. Acta Clin Belg. (2022) 77:45–50. doi: 10.1080/17843286.2020.1783907
32. Hu Y, Li Q, Min R, Deng Y, Xu Y, Gao L. The association between serum uric acid and diabetic complications in patients with type 2 diabetes mellitus by gender: a cross-sectional study. PeerJ. (2021) 9:e10691. doi: 10.7717/peerj.10691
33. Chen YL, Li H, Li S, Xu Z, Tian S, Wu J, et al. Prevalence of and risk factors for metabolic associated fatty liver disease in an urban population in China: a cross-sectional comparative study. BMC Gastroenterol. (2021) 21:212. doi: 10.1186/s12876-021-01782-w
34. Cui Y, Liu J, Shi H, Hu W, Song L, Zhao Q. Serum uric acid is positively associated with the prevalence of nonalcoholic fatty liver in non-obese type 2 diabetes patients in a Chinese population. J Diabetes Complications. (2021) 35:107874. doi: 10.1016/j.jdiacomp.2021.107874
35. Bao T, Ying Z, Gong L, Du J, Ji G, Li Z, et al. Association between serum uric acid and nonalcoholic fatty liver disease in nonobese postmenopausal women: A cross-sectional study. Sci Rep. (2020) 10:10072. doi: 10.1038/s41598-020-66931-9
36. Ma Z, Xu C, Kang X, Zhang S, Li H, Tao L, et al. Changing trajectories of serum uric acid and risk of non-alcoholic fatty liver disease: a prospective cohort study. J Transl Med. (2020) 18:133. doi: 10.1186/s12967-020-02296-x
37. Wei F, Li J, Chen C, Zhang K, Cao L, Wang X, et al. Higher serum uric acid level predicts non-alcoholic fatty liver disease: A 4-year prospective cohort study. Front Endocrinol (Lausanne). (2020) 11:179. doi: 10.3389/fendo.2020.00179
38. Sandra S, Lesmana CRA, Purnamasari D, Kurniawan J, Gani RA. Hyperuricemia as an independent risk factor for non-alcoholic fatty liver disease (NAFLD) progression evaluated using controlled attenuation parameter-transient elastography: Lesson learnt from tertiary referral center. Diabetes Metab Syndr. (2019) 13:424–8. doi: 10.1016/j.dsx.2018.10.001
39. Abbasi S, Haleem N, Jadoon S, Farooq A. Association of non-alcoholic fatty liver disease with serum uric acid. J Ayub Med Coll Abbottabad. (2019) 31:64–6.
40. Oral A, Sahin T, Turker F, Kocak E. Relationship between serum uric acid levels and nonalcoholic fatty liver disease in non-obese patients. Medicina (Kaunas). (2019) 55:600. doi: 10.3390/medicina55090600
41. Bai JX, Shu RM, Huang Y, Peng Z. Correlation between serum uric acid and risk of new-onset nonalcoholic fatty liver disease: a 5-year observational cohort study. Zhonghua Gan Zang Bing Za Zhi. (2018) 26:271–5. doi: 10.3760/cma.j.issn.1007-3418.2018.04.008
42. Yang H, Li D, Song X, Liu F, Wang X, Ma Q, et al. Joint associations of serum uric acid and ALT with NAFLD in elderly men and women: a Chinese cross-sectional study. J Transl Med. (2018) 16:285. doi: 10.1186/s12967-018-1657-6
43. Yang C, Yang S, Feng C, Zhang C, Xu W, Zhang L, et al. Associations of hyperuricemia and obesity with remission of nonalcoholic fatty liver disease among Chinese men: A retrospective cohort study. PloS One. (2018) 13:e0192396. doi: 10.1371/journal.pone.0192396
44. Zheng X, Gong L, Luo R, Chen H, Peng B, Ren W, et al. Serum uric acid and non-alcoholic fatty liver disease in non-obesity Chinese adults. Lipids Health Dis. (2017) 16:202. doi: 10.1186/s12944-017-0531-5
45. Yu XL, Shu L, Shen XM, Zhang XY, Zheng PF. Gender difference on the relationship between hyperuricemia and nonalcoholic fatty liver disease among Chinese: An observational study. Med (Baltimore). (2017) 96:e8164. doi: 10.1097/MD.0000000000008164
46. Yang C, Yang S, Xu W, Zhang J, Fu W, Feng C. Association between the hyperuricemia and nonalcoholic fatty liver disease risk in a Chinese population: A retrospective cohort study. PloS One. (2017) 12:e0177249. doi: 10.1371/journal.pone.0177249
47. Zhou Z, Song K, Qiu J, Wang Y, Liu C, Zhou H, et al. Associations between serum uric acid and the remission of non-alcoholic fatty liver disease in chinese males. PloS One. (2016) 11:e0166072. doi: 10.1371/journal.pone.0166072
48. Hossain IA, Faruque MO, Akter S, Bhuiyan FR, Rahman MK, Ali L. Elevated levels of serum uric acid and insulin resistance are associated with nonalcoholic fatty liver disease among prediabetic subjects. Trop Gastroenterol. (2016) 37:101–11.
49. Ballestri S, Nascimbeni F, Romagnoli D, Lonardo A. The independent predictors of non-alcoholic steatohepatitis and its individual histological features.: Insulin resistance, serum uric acid, metabolic syndrome, alanine aminotransferase and serum total cholesterol are a clue to pathogenesis and candidate targets for treatment. Hepatol Res. (2016) 46:1074–87. doi: 10.1111/hepr.12656
50. Lin H, Li Q, Liu X, Ma H, Xia M, Wang D, et al. Liver fat content is associated with elevated serum uric acid in the chinese middle-aged and elderly populations: shanghai changfeng study. PloS One. (2015) 10:e0140379. doi: 10.1371/journal.pone.0140379
51. Wu SJ, Zhu GQ, Ye BZ, Kong FQ, Zheng ZX, Zou H, et al. Association between sex-specific serum uric acid and non-alcoholic fatty liver disease in Chinese adults: a large population-based study. Med (Baltimore). (2015) 94:e802. doi: 10.1097/MD.0000000000000802
52. Shih MH, Lazo M, Liu SH, Bonekamp S, Hernaez R, Clark JM. Association between serum uric acid and nonalcoholic fatty liver disease in the US population. J Formos Med Assoc. (2015) 114:314–20. doi: 10.1016/j.jfma.2012.11.014
53. Liang J, Pei Y, Gong Y, Liu XK, Dou LJ, Zou CY, et al. Serum uric acid and non-alcoholic fatty liver disease in non-hypertensive Chinese adults: the Cardiometabolic Risk in Chinese (CRC) study. Eur Rev Med Pharmacol Sci. (2015) 19:305–11.
54. Liu PJ, Ma F, Lou HP, Zhu YN, Chen Y. Relationship between serum uric acid levels and hepatic steatosis in non-obese postmenopausal women. Climacteric. (2014) 17:692–9. doi: 10.3109/13697137.2014.926323
55. Sertoglu E, Ercin CN, Celebi G, Gurel H, Kayadibi H, Genc H, et al. The relationship of serum uric acid with non-alcoholic fatty liver disease. Clin Biochem. (2014) 47:383–8. doi: 10.1016/j.clinbiochem.2014.01.029
56. Xie Y, Wang M, Zhang Y, Zhang S, Tan A, Gao Y, et al. Serum uric acid and non-alcoholic fatty liver disease in non-diabetic Chinese men. PloS One. (2013) 8:e67152. doi: 10.1371/journal.pone.0067152
57. Cai W, Wu X, Zhang B, Miao L, Sun YP, Zou Y, et al. Serum uric acid levels and non-alcoholic fatty liver disease in Uyghur and Han ethnic groups in northwestern China. Arq Bras Endocrinol Metabol. (2013) 57:617–22. doi: 10.1590/s0004-27302013000800006
58. Hwang IC, Suh SY, Suh AR, Ahn HY. The relationship between normal serum uric acid and nonalcoholic fatty liver disease. J Korean Med Sci. (2011) 26:386–91. doi: 10.3346/jkms.2011.26.3.386
59. Ryu S, Chang Y, Kim SG, Cho J, Guallar E. Serum uric acid levels predict incident nonalcoholic fatty liver disease in healthy Korean men. Metabolism. (2011) 60:860–6. doi: 10.1016/j.metabol.2010.08.005
60. Xu C, Yu C, Xu L, Miao M, Li Y. High serum uric acid increases the risk for nonalcoholic Fatty liver disease: a prospective observational study. PloS One. (2010) 5:e11578. doi: 10.1371/journal.pone.0011578
61. Kuo CF, Yu KH, Luo SF, Chiu CT, Ko YS, Hwang JS, et al. Gout and risk of non-alcoholic fatty liver disease. Scand J Rheumatol. (2010) 39:466–71. doi: 10.3109/03009741003742797
62. Li Y, Xu C, Yu C, Xu L, Miao M. Association of serum uric acid level with non-alcoholic fatty liver disease: a cross-sectional study. J Hepatol. (2009) 50:1029–34. doi: 10.1016/j.jhep.2008.11.021
63. Buzzetti E, Pinzani M, Tsochatzis EA. The multiple-hit pathogenesis of non-alcoholic fatty liver disease (NAFLD). Metabolism. (2016) 65:1038–48. doi: 10.1016/j.metabol.2015.12.012
64. Deng A, Liu F, Tang X, Wang Y, Xie P, Yang Q, et al. Water extract from artichoke ameliorates high-fat diet-induced non-alcoholic fatty liver disease in rats. BMC Complement Med Ther. (2022) 22:308. doi: 10.1186/s12906-022-03794-9
65. Hong T, Chen Y, Li X, Lu Y. The role and mechanism of oxidative stress and nuclear receptors in the development of NAFLD. Oxid Med Cell Longev. (2021) 2021:6889533. doi: 10.1155/2021/6889533
66. Gonzalez A, Huerta-Salgado C, Orozco-Aguilar J, Aguirre F, Tacchi F, Simon F, et al. Role of oxidative stress in hepatic and extrahepatic dysfunctions during nonalcoholic fatty liver disease (NAFLD). Oxid Med Cell Longev. (2020) 2020:1617805. doi: 10.1155/2020/1617805
67. Xie D, Zhao H, Lu J, He F, Liu W, Yu W, et al. High uric acid induces liver fat accumulation via ROS/JNK/AP-1 signaling. Am J Physiol Endocrinol Metab. (2021) 320:E1032–43. doi: 10.1152/ajpendo.00518.2020
68. Brennan P, Clare K, George J, Dillon JF. Determining the role for uric acid in non-alcoholic steatohepatitis development and the utility of urate metabolites in diagnosis: An opinion review. World J Gastroenterol. (2020) 26:1683–90. doi: 10.3748/wjg.v26.i15.1683
69. Saha T, Kumar P, Sepay N, Ganguly D, Tiwari K, Mukhopadhyay K, et al. Multitargeting antibacterial activity of a synthesized mn2+ Complex of curcumin on gram-positive and gram-negative bacterial strains. ACS Omega.. (2020) 5:16342–57. doi: 10.1021/acsomega.9b04079
70. Nakatsu Y, Seno Y, Kushiyama A, Sakoda H, Fujishiro M, Katasako A, et al. The xanthine oxidase inhibitor febuxostat suppresses development of nonalcoholic steatohepatitis in a rodent model. Am J Physiol Gastrointest Liver Physiol. (2015) 309:G42–51. doi: 10.1152/ajpgi.00443.2014
71. Francey C, Cros J, Rosset R, Crézé C, Rey V, Stefanoni N, et al. The extra-splanchnic fructose escape after ingestion of a fructose-glucose drink: An exploratory study in healthy humans using a dual fructose isotope method. Clin Nutr ESPEN. (2019) 29:125–32. doi: 10.1016/j.clnesp.2018.11.008
72. Lanaspa MA, Sanchez-Lozada LG, Choi YJ, Cicerchi C, Kanbay M, Roncal-Jimenez CA, et al. Uric acid induces hepatic steatosis by generation of mitochondrial oxidative stress: potential role in fructose-dependent and -independent fatty liver. J Biol Chem. (2012) 287:40732–44. doi: 10.1074/jbc.M112.399899
73. Wang H, Li Y, Bian Y, Li X, Wang Y, Wu K, et al. Potential hepatoprotective effects of Cistanche deserticola Y.C. Ma: Integrated phytochemical analysis using UPLC-Q-TOF-MS/MS, target network analysis, and experimental assessment. Front Pharmacol. (2022) 13:1018572. doi: 10.3389/fphar.2022.1018572
74. Spiga R, Marini MA, Mancuso E, Di Fatta C, Fuoco A, Perticone F, et al. Uric acid is associated with inflammatory biomarkers and induces inflammation via activating the NF-κB signaling pathway in hepG2 cells. Arterioscler Thromb Vasc Biol. (2017) 37:1241–9. doi: 10.1161/ATVBAHA.117.309128
75. Stienstra R, van Diepen JA, Tack CJ, Zaki MH, van de Veerdonk FL, Perera D, et al. Inflammasome is a central player in the induction of obesity and insulin resistance. Proc Natl Acad Sci U.S.A. (2011) 108:15324–9. doi: 10.1073/pnas.1100255108
76. Vandanmagsar B, Youm YH, Ravussin A, Galgani JE, Stadler K, Mynatt RL, et al. The NLRP3 inflammasome instigates obesity-induced inflammation and insulin resistance. Nat Med. (2011) 17:179–88. doi: 10.1038/nm.2279
77. Wu X, Dong L, Lin X, Li J. Relevance of the NLRP3 inflammasome in the pathogenesis of chronic liver disease. Front Immunol. (2017) 8:1728. doi: 10.3389/fimmu.2017.01728
78. Kono H, Chen CJ, Ontiveros F, Rock KL. Uric acid promotes an acute inflammatory response to sterile cell death in mice. J Clin Invest. (2010) 120:1939–49. doi: 10.1172/JCI40124
79. Shi Y, Evans JE, Rock KL. Molecular identification of a danger signal that alerts the immune system to dying cells. Nature. (2003) 425:516–21. doi: 10.1038/nature01991
80. Wan X, Xu C, Lin Y, Lu C, Li D, Sang J, et al. Uric acid regulates hepatic steatosis and insulin resistance through the NLRP3 inflammasome-dependent mechanism. J Hepatol. (2016) 64:925–32. doi: 10.1016/j.jhep.2015.11.022
81. Zhao H, Lu J, He F, Wang M, Yan Y, Chen B, et al. Hyperuricemia contributes to glucose intolerance of hepatic inflammatory macrophages and impairs the insulin signaling pathway via IRS2-proteasome degradation. Front Immunol. (2022) 13:931087. doi: 10.3389/fimmu.2022.931087
82. Rock KL, Lai JJ, Kono H. Innate and adaptive immune responses to cell death. Immunol Rev. (2011) 243:191–205. doi: 10.1111/j.1600-065X.2011.01040.x
83. Aoyama Y, Naiki-Ito A, Xiaochen K, Komura M, Kato H, Nagayasu Y, et al. Lactoferrin prevents hepatic injury and fibrosis via the inhibition of NF-κB signaling in a rat non-alcoholic steatohepatitis model. Nutrients. (2021) 14:42. doi: 10.3390/nu14010042
84. Lou B, Wu H, Ott H, Bennewitz K, Wang C, Poschet G, et al. Increased circulating uric acid aggravates heart failure via impaired fatty acid metabolism. J Transl Med. (2023) 21:199. doi: 10.1186/s12967-023-04050-5
85. Choi YJ, Shin HS, Choi HS, Park JW, Jo I, Oh ES, et al. Uric acid induces fat accumulation via generation of endoplasmic reticulum stress and SREBP-1c activation in hepatocytes. Lab Invest. (2014) 94:1114–25. doi: 10.1038/labinvest.2014.98
86. Ozcelik F, Yiginer O. The relationship between serum uric acid levels and the major risk factors for the development of nonalcoholic fatty liver disease. Liver Int. (2016) 36:768–9. doi: 10.1111/liv.13063
87. Lima WG, Martins-Santos ME, Chaves VE. Uric acid as a modulator of glucose and lipid metabolism. Biochimie. (2015) 116:17–23. doi: 10.1016/j.biochi.2015.06.025
88. Chen S, Chen D, Yang H, Wang X, Wang J, Xu C. Uric acid induced hepatocytes lipid accumulation through regulation of miR-149-5p/FGF21 axis. BMC Gastroenterol. (2020) 20:39. doi: 10.1186/s12876-020-01189-z
89. Itabashi F, Hirata T, Kogure M, Narita A, Tsuchiya N, Nakamura T, et al. Combined associations of liver enzymes and obesity with diabetes mellitus prevalence: the tohoku medical megabank community-based cohort study. J Epidemiol. (2022) 32:221–7. doi: 10.2188/jea.JE20200384
90. Choi A, Kim JH, Chung HK, Ahn CW, Choi HJ, Kim YS, et al. The effects of C. lacerata on insulin resistance in type 2 diabetes patients. J Diabetes Res. (2022) 2022:9537741. doi: 10.1155/2022/9537741
91. Hotamisligil GS, Erbay E. Nutrient sensing and inflammation in metabolic diseases. Nat Rev Immunol. (2008) 8:923–34. doi: 10.1038/nri2449
92. Odegaard JI, Chawla A. Mechanisms of macrophage activation in obesity-induced insulin resistance. Nat Clin Pract Endocrinol Metab. (2008) 4:619–26. doi: 10.1038/ncpendmet0976
93. Olefsky JM, Glass CK. Macrophages, inflammation, and insulin resistance. Annu Rev Physiol. (2010) 72:219–46. doi: 10.1146/annurev-physiol-021909-135846
94. Shoelson SE, Herrero L, Naaz A. Obesity, inflammation, and insulin resistance. Gastroenterology. (2007) 132:2169–80. doi: 10.1053/j.gastro.2007.03.059
95. Nogueira JP, Cusi K. Role of insulin resistance in the development of nonalcoholic fatty liver disease in people with type 2 diabetes: from bench to patient care. Diabetes SpectrI. (2024) 37:20–8. doi: 10.2337/dsi23-0013
96. Kawano Y, Cohen DE. Mechanisms of hepatic triglyceride accumulation in non-alcoholic fatty liver disease. J Gastroenterol. (2013) 48:434–41. doi: 10.1007/s00535-013-0758-5
97. Sanchez-Lozada LG, Andres-Hernando A, Garcia-Arroyo FE, Cicerchi C, Li N, Kuwabara M, et al. Uric acid activates aldose reductase and the polyol pathway for endogenous fructose and fat production causing development of fatty liver in rats. J Biol Chem. (2019) 294:4272–81. doi: 10.1074/jbc.RA118.006158
98. Yoo TW, Sung KC, Shin HS, Kim BJ, Kim BS, Kang JH, et al. Relationship between serum uric acid concentration and insulin resistance and metabolic syndrome. Circ J. (2005) 69:928–33. doi: 10.1253/circj.69.928
99. Zhu Y, Hu Y, Huang T, Zhang Y, Li Z, Luo C, et al. High uric acid directly inhibits insulin signalling and induces insulin resistance. Biochem Biophys Res Commun. (2014) 447:707–14. doi: 10.1016/j.bbrc.2014.04.080
100. Han R, Zhang Y, Jiang X. Relationship between four non-insulin-based indexes of insulin resistance and serum uric acid in patients with type 2 diabetes: A cross-sectional study. Diabetes Metab Syndr Obes. (2022) 15:1461–71. doi: 10.2147/DMSO.S362248
101. Savari F, Mard SA. Nonalcoholic steatohepatitis: A comprehensive updated review of risk factors, symptoms, and treatment. Heliyon. (2024) 10:e28468. doi: 10.1016/j.heliyon.2024.e28468
102. Vilar-Gomez E, Martinez-Perez Y, Calzadilla-Bertot L, Torres-Gonzalez A, Gra-Oramas B, Gonzalez-Fabian L, et al. Weight loss through lifestyle modification significantly reduces features of nonalcoholic steatohepatitis. Gastroenterology. (2015) 149:367–78.e5; quiz e14-5. doi: 10.1053/j.gastro.2015.04.005
103. Tan CH, Al-Kalifah N, Ser KH, Lee YC, Chen JC, Lee WJ. Long-term effect of bariatric surgery on resolution of nonalcoholic steatohepatitis (NASH): An external validation and application of a clinical NASH score. Surg Obes Relat Dis. (2018) 14:1600–6. doi: 10.1016/j.soard.2018.05.024
104. Laursen TL, Hagemann CA, Wei C, Kazankov K, Thomsen KL, Knop FK, et al. Bariatric surgery in patients with non-alcoholic fatty liver disease - from pathophysiology to clinical effects. World J Hepatol. (2019) 11:138–49. doi: 10.4254/wjh.v11.i2.138
105. Chalasani N, Younossi Z, Lavine JE, Charlton M, Cusi K, Rinella M, et al. The diagnosis and management of nonalcoholic fatty liver disease: Practice guidance from the American Association for the Study of Liver Diseases. Hepatology. (2018) 67:328–57. doi: 10.1002/hep.29367
106. Portincasa P, Khalil M, Mahdi L, Perniola V, Idone V, Graziani A, et al. Metabolic dysfunction-associated steatotic liver disease: from pathogenesis to current therapeutic options. Int J Mol Sci. (2024) 25:5640. doi: 10.3390/ijms25115640
107. Al-Shargi A, El Kholy AA, Adel A, Hassany M, Shaheen SM. Allopurinol versus febuxostat: A new approach for the management of hepatic steatosis in metabolic dysfunction-associated steatotic liver disease. Biomedicines. (2023) 11:3074. doi: 10.3390/biomedicines11113074
108. Xu C. Hyperuricemia and nonalcoholic fatty liver disease: from bedside to bench and back. Hepatol Int. (2016) 10:286–93. doi: 10.1007/s12072-015-9682-5
109. Sun DQ, Wu SJ, Liu WY, Lu QD, Zhu GQ, Shi KQ, et al. Serum uric acid: a new therapeutic target for nonalcoholic fatty liver disease. Expert Opin Ther Targets. (2016) 20:375–87. doi: 10.1517/14728222.2016.1096930
110. Barişik V, Korkmaz HA, Çekdemir YE, Torlak D, Aktuğ H, Yavaşoğlu A, et al. The therapeutic effect of allopurinol in fatty liver disease in rats. Acta Endocrinol (Buchar). (2023) 19:155–62. doi: 10.4183/aeb.2023.155
111. Zeng F, Luo J, Han H, Xie W, Wang L, Han R, et al. Allopurinol ameliorates liver injury in type 1 diabetic rats through activating Nrf2. Int J Immunopathol Pharmacol. (2021) 35:20587384211031417. doi: 10.1177/20587384211031417
112. Wang W, Wang C, Ding XQ, Pan Y, Gu TT, Wang MX, et al. Quercetin and allopurinol reduce liver thioredoxin-interacting protein to alleviate inflammation and lipid accumulation in diabetic rats. Br J Pharmacol. (2013) 169:1352–71. doi: 10.1111/bph.12226
113. Liu J, Fan Y, Yu H, Xu T, Zhang C, Zhou L, et al. Allopurinol protects against cholestatic liver injury in mice not through depletion of uric acid. Toxicol Sci. (2021) 181:295–305. doi: 10.1093/toxsci/kfab034
114. Cho IJ, Oh DH, Yoo J, Hwang YC, Ahn KJ, Chung HY, et al. Allopurinol ameliorates high fructose diet induced hepatic steatosis in diabetic rats through modulation of lipid metabolism, inflammation, and ER stress pathway. Sci Rep. (2021) 11:9894. doi: 10.1038/s41598-021-88872-7
115. Lee JS, Won J, Kwon OC, Lee SS, Oh JS, Kim YG, et al. Hepatic safety of febuxostat compared with allopurinol in gout patients with fatty liver disease. J Rheumatol. (2019) 46:527–31. doi: 10.3899/jrheum.180761
116. Nishikawa T, Nagata N, Shimakami T, Shirakura T, Matsui C, Ni Y, et al. Xanthine oxidase inhibition attenuates insulin resistance and diet-induced steatohepatitis in mice. Sci Rep. (2020) 10:815. doi: 10.1038/s41598-020-57784-3
117. Tang W, Mu J, Chen QI, Li X, Liu H. The involvement and mechanism of febuxostat in non-alcoholic fatty liver disease cells. J Biol Regul Homeost Agents. (2018) 32:545–51.
Keywords: nonalcoholic fatty liver disease, serum uric acid, xanthine oxidase, oxidative stress, NLRP3 inflammasome, lipid metabolism, insulin resistance
Citation: Fan J and Wang D (2024) Serum uric acid and nonalcoholic fatty liver disease. Front. Endocrinol. 15:1455132. doi: 10.3389/fendo.2024.1455132
Received: 26 June 2024; Accepted: 11 November 2024;
Published: 28 November 2024.
Edited by:
Roger Gutiérrez-Juárez, National Autonomous University of Mexico, MexicoReviewed by:
Berwin Singh Swami Vetha, East Carolina University, United StatesJiachen Sun, Dana–Farber Cancer Institute, United States
Yongxiang Li, Baylor College of Medicine, United States
Copyright © 2024 Fan and Wang. This is an open-access article distributed under the terms of the Creative Commons Attribution License (CC BY). The use, distribution or reproduction in other forums is permitted, provided the original author(s) and the copyright owner(s) are credited and that the original publication in this journal is cited, in accordance with accepted academic practice. No use, distribution or reproduction is permitted which does not comply with these terms.
*Correspondence: Dongxu Wang, ZG9uZ3h1d2FuZ2R4QDEyNi5jb20=