- Hebei Key Laboratory of Chinese Medicine Research on Cardio-Cerebrovascular Disease, Hebei University of Chinese Medicine, Shijiazhuang, China
Background: Oxidative stress is widely acknowledged as a key pathogenic mechanism in diabetic nephropathy (DN). In recent years, the role of oxidative stress in DN has garnered increasing attention. However, no bibliometric analysis has yet been conducted on the relationship between oxidative stress and DN. This study aims to systematically analyze the relevant literature, identify trends in research, assess current hotspots, and predict future directions.
Methods: We retrieved literature related to oxidative stress and DN from the Web of Science Core Collection database. We analyzed data on publication volume, countries/regions, institutions, journals, keywords, and other relevant metrics using VOSviewer, the Bibliometrix R package, and CiteSpace.
Results: From 2014 to 2024, a total of 4076 publications related to oxidative stress and DN were published across 755 journals, showing a consistent upward trend each year. China and the United States are the leading contributors in this field and demonstrate close collaborative efforts. The top contributors by country, institution, journal, and author include: China (1919 publications), Jilin University and Central South University (69 publications each), BIOMEDICINE & PHARMACOTHERAPY (117 publications), and Prof. Sun Lin (33 publications). The most frequent keyword is “oxidative stress” (3683 occurrences). In the co-citation analysis, Alicic RZ’s 2017 study was the most cited (144 citations). These findings highlight the critical importance of investigating the pathogenesis of DN from the oxidative stress perspective.
Conclusion: This study demonstrates a steady increase in research on oxidative stress in DN since 2014, highlighting its central role in the pathogenesis of DN. Future research should focus on the molecular mechanisms of oxidative stress in DN and explore its therapeutic potential, to provide new strategies for the prevention and treatment of DN.
1 Introduction
Diabetes mellitus (DM) is a global public health concern, affecting millions of individuals worldwide. In 2021, global healthcare expenditures related to diabetes amounted to approximately USD 966 billion, with projections indicating a rise to USD 1.045 trillion by 2045 (1). DM leads to various vascular complications, including diabetic nephropathy (DN), diabetic retinopathy (DR), neuropathy, and cardiovascular diseases (CVD) (2). DN is one of the most common and severe complications of DM (3), and it is a leading cause of end-stage renal disease (ESRD) (4). Approximately one-third of DM patients progress to DN, and its prevalence has been rising alarmingly (5). The clinical manifestations of DN primarily include hypertension, edema, and proteinuria, which may progress to renal failure or even life-threatening conditions (6).
The pathogenesis of DN involves several interconnected processes, including oxidative stress, activation of the renin-angiotensin-aldosterone system, and inflammatory responses (7–9). Numerous studies have identified oxidative stress as one of the primary mechanisms underlying DN (10, 11), with prolonged hyperglycemia acting as a trigger for its onset (12). Once oxidative stress occurs, the balance between oxidants and antioxidants is disrupted (13). Excessive production of reactive oxygen species (ROS) and reactive nitrogen species (RNS) leads to renal damage, particularly by ROS, which include peroxides, superoxides, and hydroxyl radicals—key contributors to kidney disease (14, 15). Antioxidants, which can be classified as either enzymatic or non-enzymatic, neutralize ROS to maintain cellular homeostasis (16). Excessive ROS production and collapse of the antioxidant system lead to the continuous oxidation of macromolecules, including lipids, proteins, and DNA, ultimately causing cellular dysfunction (8, 17). Research has shown that ROS-induced damage to glomerular endothelial cells, mesangial cells, podocytes, and renal tubular epithelial cells has accelerated the progression of DN (18–20). Consequently, oxidative stress is intricately linked to DN, and recent research has increasingly focused on the potential of medicinal plants and natural compounds in mitigating oxidative stress (21). Understanding the underlying mechanisms of oxidative stress is, therefore, crucial for preventing DN and developing new therapeutic strategies.
Bibliometrics is a statistical method used to analyze large volumes of content or citations (22, 23), providing a quantitative description of existing literature (24). This approach enables researchers to gain insights into key authors, keywords, journals, and countries/regions within a specific research field, thereby facilitating an understanding of thematic evolution and emerging research trends (25, 26). To date, no bibliometric study has been conducted specifically on oxidative stress in the context of DN. This study aims to fill this gap.
Despite significant progress in research on oxidative stress mechanisms in DN, there is a lack of bibliometric studies specifically focusing on oxidative stress in DN. The primary objective of this bibliometric study is to systematically analyze the research landscape, identify hotspots, and explore emerging trends in the field of oxidative stress DN. By offering a comprehensive overview of key contributors and research dynamics, this study seeks to assist basic scientists in prioritizing research directions, facilitating interdisciplinary collaborations, and addressing under-investigated areas. The findings also offer critical insights to advance therapeutic strategies, foster translational applications, and inform resource allocation and policy-making in DN management.
2 Materials and methods
2.1 Search strategy
Literature data were independently retrieved, validated, and standardized by the authors (WXR) and (WZ) through the Web of Science Core Collection (WoSCC) database, utilizing the Science Citation Index Expanded. The search formula used was as follows:
Ts=(“diabetic nephropathy” OR “diabetic kidney disease”) AND Ts=(“oxidative stress” OR “ROS” OR “reactive oxygen species”).The language was set to English, and only original articles and reviews that met the criteria for this study were included. The search period was from January 1, 2014, to November 1, 2024. Retracted articles or those irrelevant to the topic were manually excluded. The online literature, including full records and references, was then exported in plain text format.
2.2 Data analysis
GraphPad Prism 10.1.1 was used to generate line graphs illustrating annual publication trends.
VOSviewer 1.6.20 is a tool designed to construct and visualize bibliometric maps. It creates maps of authors or journals based on co-citation data (27). In this study, VOSviewer was utilized to visualize collaborations among countries/regions, journals, institutions, and authors and to generate network visualizations.
CiteSpace 6.3.R1 (Drexel University, Philadelphia, USA) is a Java-based software developed by Professor Chaomei Chen. Its primary advantage is its ability to explore quantitative data and development trends in scientific research fields through visual analysis, enhancing the understanding of scientific progress (26, 28). In this study, CiteSpace was used to process the literature data collected from WoSCC, constructing visual networks of keywords and references. Additionally, burst detection, cluster analysis of co-cited references, and timeline visualizations were performed. Each node represents a unique keyword or reference, with the size of the node corresponding to its frequency of occurrence; larger nodes indicate higher frequency.
Bibliometrix, an R package developed by Massimo Aria and Corrado Cuccurullo for data analysis and visualization (29), was used in this study to analyze the country affiliations of corresponding authors and visualize the international collaboration network and journal data.
3 Results
3.1 Publication trends
A total of 4229 publications related to oxidative stress and DN were identified from 2014 to 2024.After excluding non-original articles and reviews (n=65), non-English articles (n=10), and manually screening 78 retracted or irrelevant publications, a final total of 4076 publications were retained (Figure 1). Since 2014, the number of publications has shown an overall upward trend, with declines observed only in 2015, 2017, and 2023 compared to the previous year. On average, 408 publications were published annually, with the highest number of publications occurring in 2022, which reached 518 articles (Figure 2).
3.2 Countries/regions and institutions distribution
3.2.1 Countries/regions
The 4076 publications were contributed by 3728 institutions across 101 countries/regions. As shown in Table 1, the country with the highest contribution is China (n=2025), followed by the United States (n=498), Japan (n=247), India (n=246), South Korea (n=184), Egypt (n=151), Saudi Arabia (n=132), Iran (n=132), Australia (n=102), and Italy (n=97). The top ten countries are distributed across Asia (n=6), Europe (n=1), Oceania (n=1), the Americas (n=1), and Africa (n=1). Figure 3A illustrates the collaborative relationships between countries/regions in this field. As shown in Figure 3B, each node represents a country or region, with node size indicating centrality, and the color of the circles representing different years. Both China and the United States occupy the most central positions. Figure 3C shows a density heatmap of countries/regions generated using VOSviewer, where darker colors indicate closer collaboration.
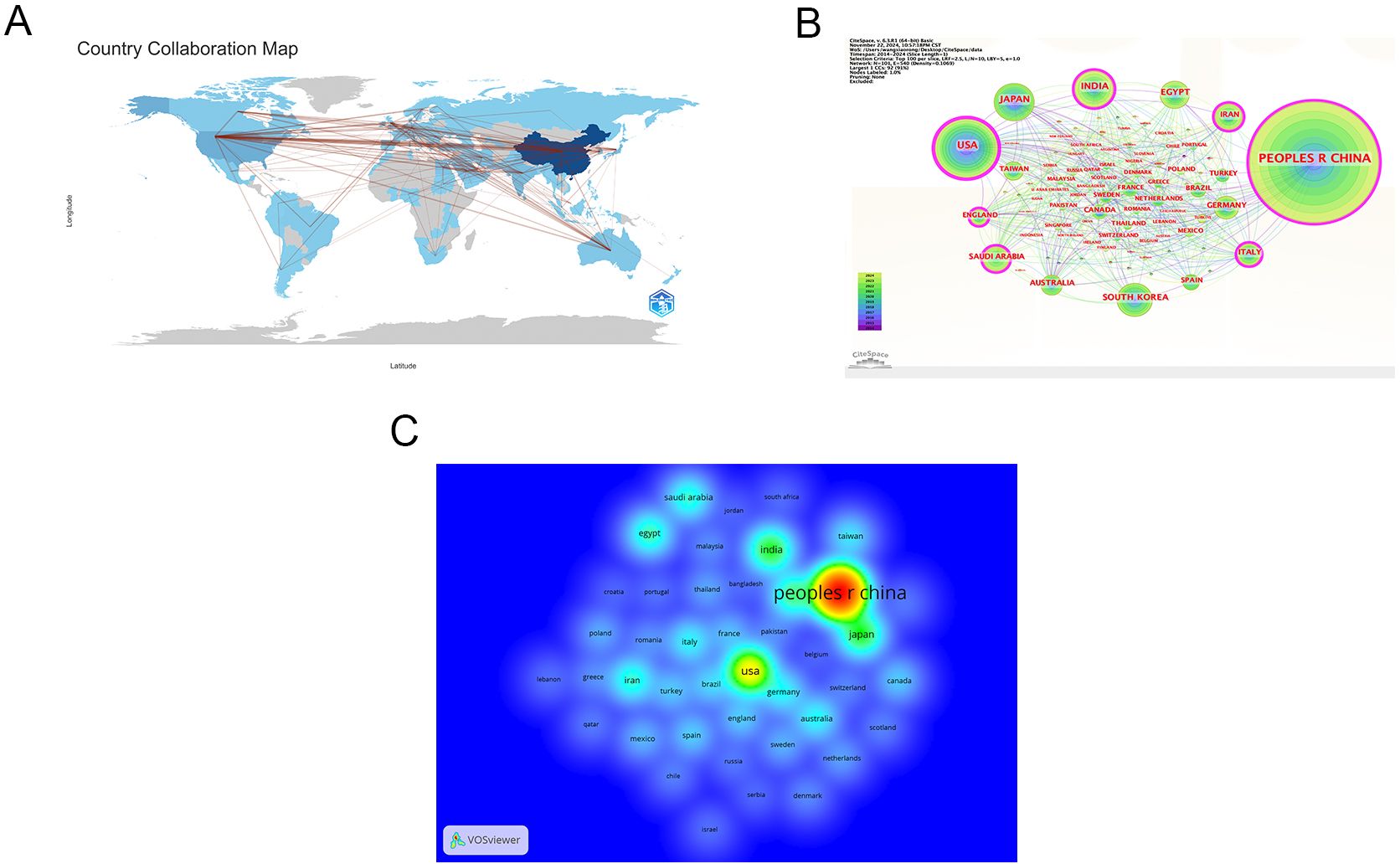
Figure 3. Countries/regions cooperation network. (A) Map of cooperation networks across countries/regions; (B) Map of central national/regional cooperation network; (C) Density map of national/regional cooperation network. Note:the Taiwan in the figure is China’s Taiwan Province. From: CiteSpace.
3.2.2 Institutions
Regarding institutions, a total of 3728 institutions contributed to the publications, with the top contributors being Jilin University (n=69) and Central South University (n=69), followed by China Medical University (n=61), Shanghai Jiao Tong University (n=58), and Shandong University (n=57), among others, as shown in Table 2. Notably, nine out of the top ten institutions by publication count are located in China. The visualization network of institutional collaborations (Figure 4) demonstrates that institutions within the same country tend to exhibit closer collaborative ties.
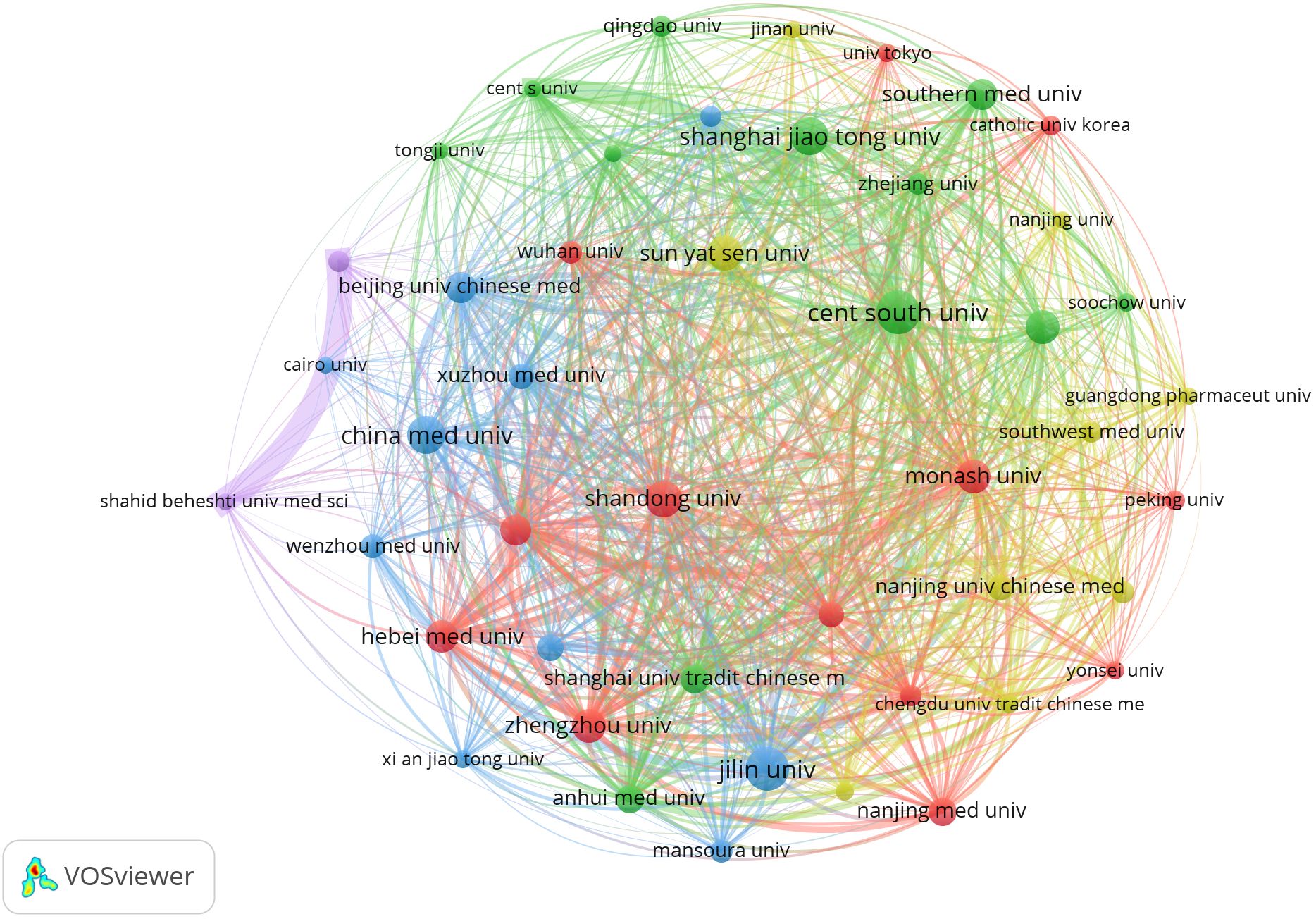
Figure 4. Cooperation network between major institutions. From: VOSviewer.
3.3 Journals and co-cited journals
3.3.1 Journals
The 4076 publications appeared in 755 journals. The top ten journals by publication count are listed in Table 3: INTERNATIONAL JOURNAL OF MOLECULAR SCIENCES (n=117), FRONTIERS IN PHARMACOLOGY (n=111), BIOMEDICINE & PHARMACOTHERAPY (n=99), LIFE SCIENCES (n=64), AMERICAN JOURNAL OF PHYSIOLOGY-RENAL PHYSIOLOGY (n=60), JOURNAL OF DIABETES RESEARCH (n=58), SCIENTIFIC REPORTS (n=53), PLOS ONE (n=52), EUROPEAN JOURNAL OF PHARMACOLOGY (n=51), and FRONTIERS IN ENDOCRINOLOGY (n=50). The highest impact factor among the top ten journals is found in BIOMEDICINE & PHARMACOTHERAPY (IF=6.9), while the lowest is in PLOS ONE (IF=2.9). The average impact factor is 5.4 (obtained from the 2024 Journal Citation Report), indicating that the articles published in these journals are generally of high quality. Figure 5A shows the publication timeline of the top five journals. Figure 5B illustrates the 28 core journals in this field. VOSviewer was used to filter journals with more than 20 publications, resulting in 43 journals, and a collaboration network between these journals was visualized (Figure 5C). Figure 5D shows the density heatmap of these journals, where darker colors indicate stronger collaboration.
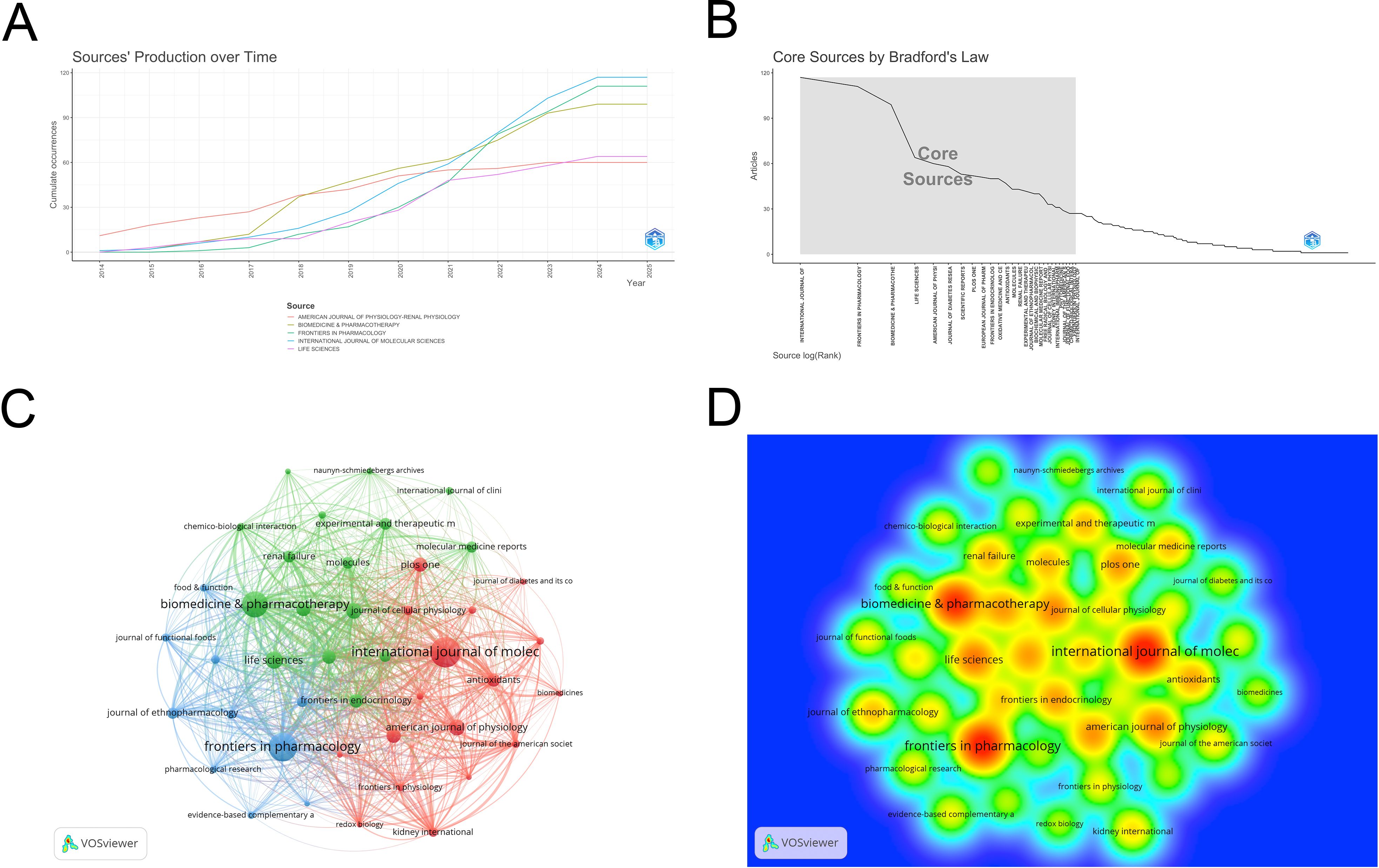
Figure 5. (A) Time chart of publications across five journals; (B) Core journal collection; (C) Journal cooperation network map; (D) Density map of journal cooperation network. From: VOSviewer.
3.3.2 Co-cited journals
In the co-citation analysis, 10,908 co-cited journals were identified. As shown in Table 3, the most co-cited journal is KIDNEY INTERNATIONAL (n=9158). The highest impact factor among the top ten co-cited journals is NEW ENGLAND JOURNAL OF MEDICINE (IF=96.2), while the lowest is PLOS ONE (IF=2.9), with an average impact factor of 17.5, indicating that the co-cited journals are generally of high quality. The collaboration network of co-cited journals is shown in Figure 6A, where many journals exhibit collaborative links. Figure 6B displays the heatmap of co-cited journals, with higher intensity colors representing more frequent collaborations.
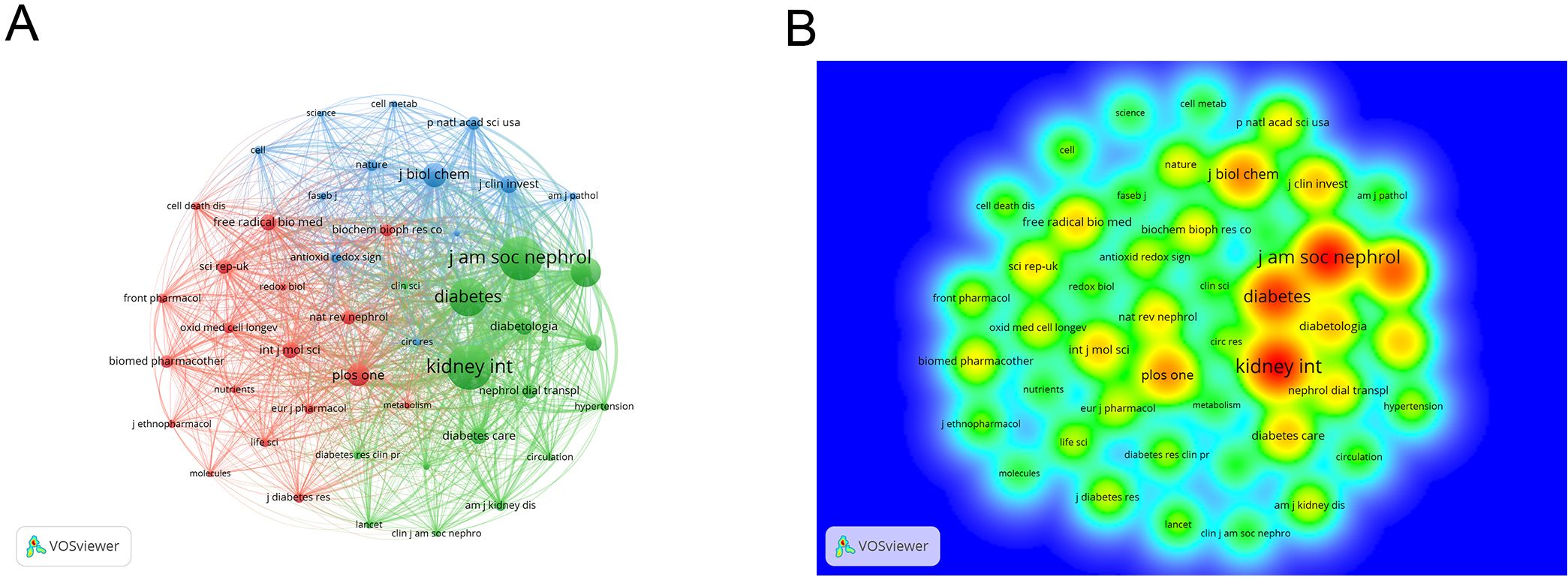
Figure 6. (A) Co-cited journal cooperation network map; (B) Density map of co-cited journal cooperation network. From: VOSviewer.
3.4 Authors and co-cited authors
3.4.1 Authors
Among the 4076 publications related to oxidative stress in DN, a total of 19,372 authors contributed. The top ten authors by publication count are listed in Table 4. The five authors with the highest number of publications are: Sun Lin (n=33), Li Ping (n=29), Cooper, Mark E (n=27), Shi Yonghong (n=24), and Li Yan (n=24). A visualization map was generated for authors who published more than 10 articles (Figure 7A). Sun Lin has the largest node, indicating that he published the most related articles. The collaboration between authors is evident, with notable partnerships such as between Sun Lin and Xiao Li, and Wu Meiming and Shi Yonghong. Figure 7B shows the country distribution of corresponding authors.
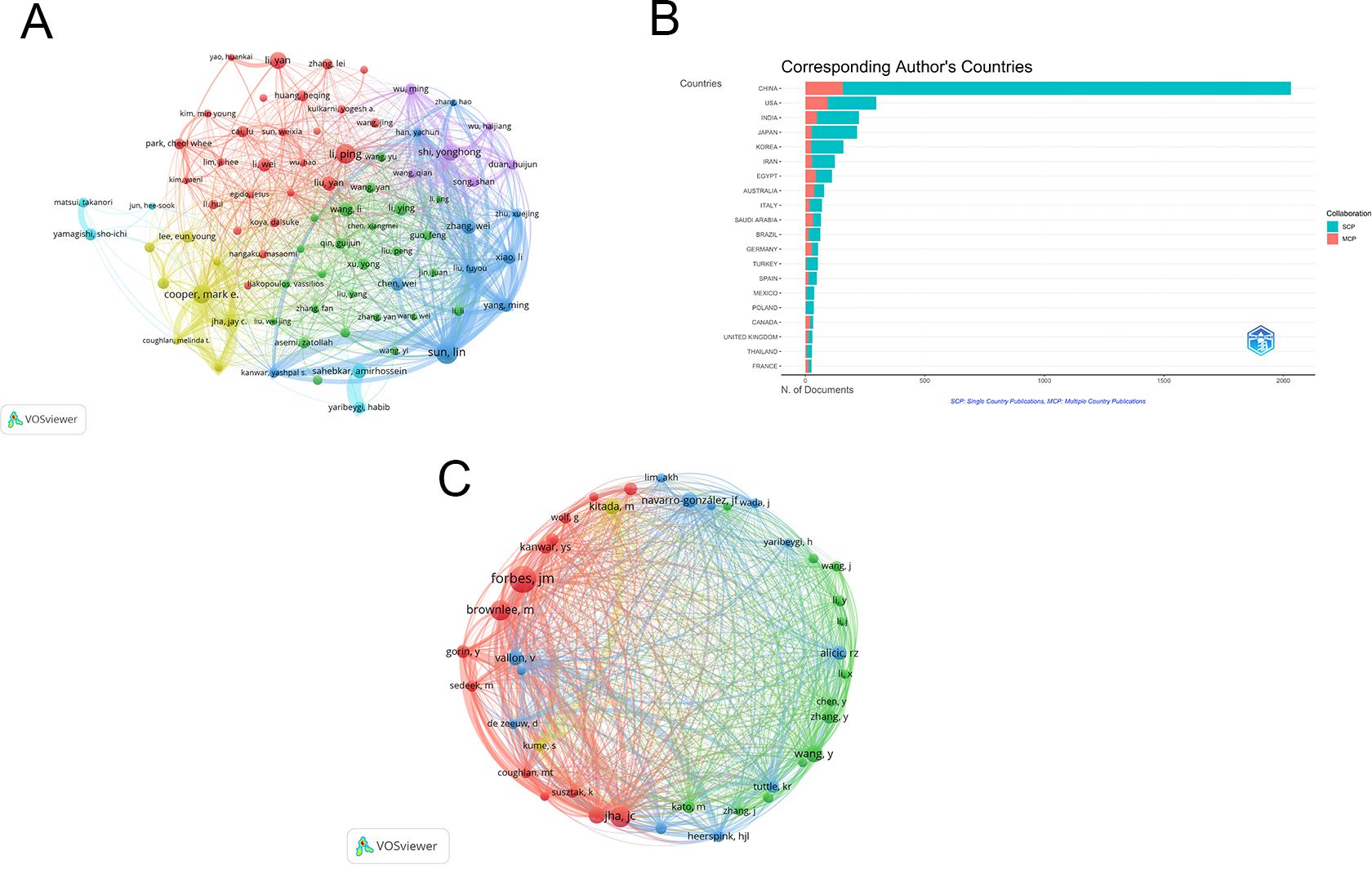
Figure 7. (A) Authors cooperation network map; (B) Countries of corresponding authors. SCP: The corresponding author is from abroad. MCP: The corresponding author is from their own country; (C) Co-cited authors cooperation network map. From: VOSviewer.
3.4.2 Co-cited authors
Among 83,410 co-cited authors, the most frequently co-cited author is Forbes, JM (n=669), followed by Jha, JC (n=508), Brownlee, M (n=501), Wang, Y (n=416), Sharma, K (n=402), Kitada, M (n=382), Vallon, V (n=374), Navarro-González, JF (n=374), Alicic, RZ (n=332), and Kanwar, YS (n=316), as shown in Table 4. A visualization network map was created for co-cited authors with more than 300 co-citations (Figure 7C). The node representing Forbes, JM is the largest, reflecting his position as the most co-cited author. The network also highlights close collaborations among several co-cited authors, such as Kitada, M and Coughlan, ML, and Jha, JC and Gorin, Y, indicating frequent communication and collaboration among these researchers.
3.5 Keywords
Table 5 lists the ten most frequently occurring keywords in the analysis: oxidative stress (n=3683), diabetic nephropathy (n=2338), expression (n=715), nephropathy (n=549), activation (n=530), inflammation (n=480), injury (n=480), kidney disease (n=411), mechanisms (n=390), and chronic kidney disease (n=467). In Figure 8A, oxidative stress and DN are represented as the largest nodes. The density map of the keyword co-occurrence network shows that oxidative stress and DN are positioned at the center (Figure 8B). The centrality of keywords also reflects the research frontiers; keywords with strong centrality include oxidative stress, DN, and activation. All keywords were clustered, and six types of labels were calculated (Figure 8C). These clusters include diabetic kidney disease (DKD), growth factor beta, DN, lipid peroxidation, diabetes mellitus, and phosphorylation. Figure 8D shows the timeline of keyword cluster changes.
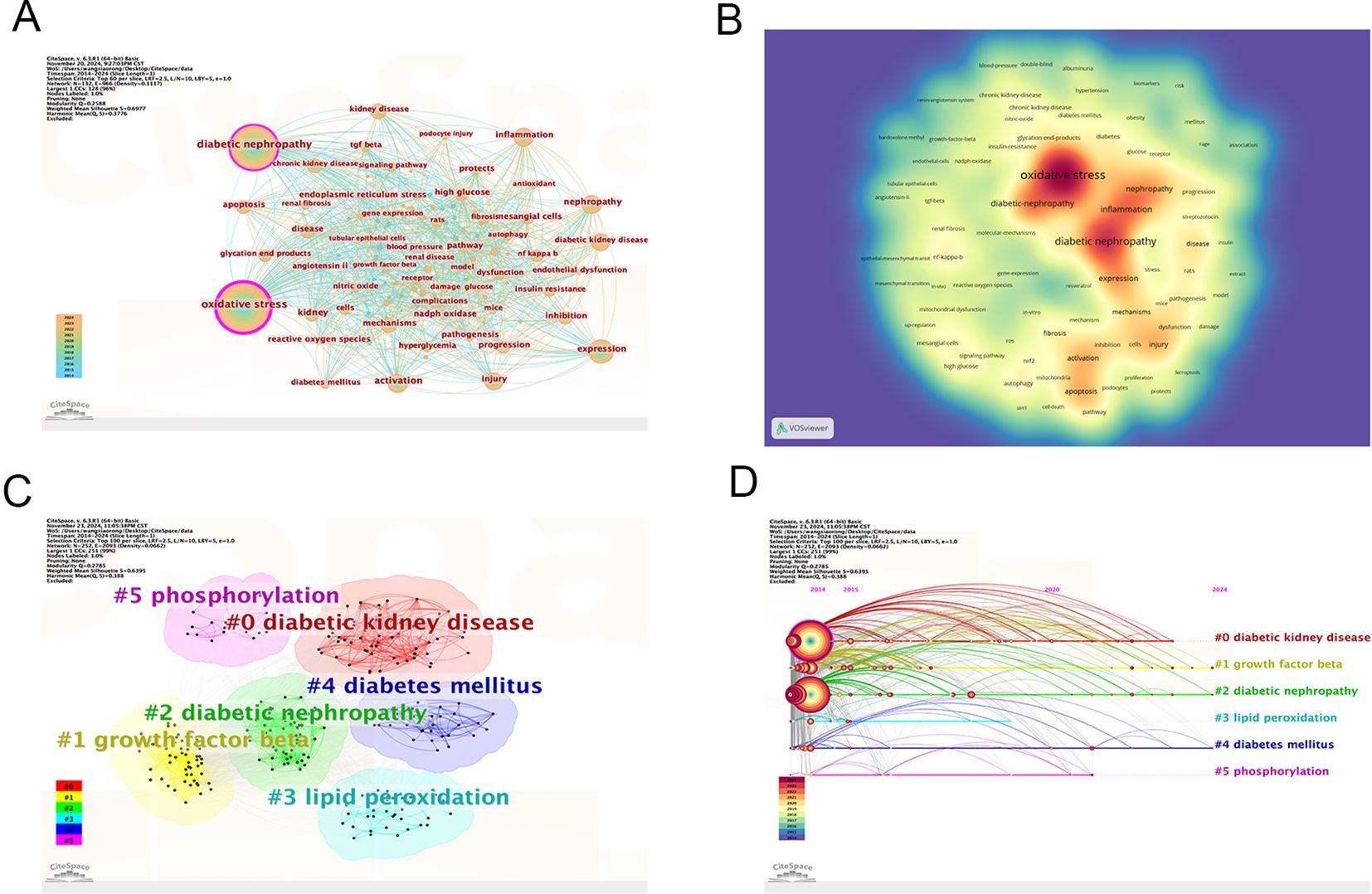
Figure 8. Bibliographic analysis of keywords. (A) Visualization of keywords; (B) Density map of keyword cooperation network; (C) Clustering map of keyword topics; (D) Timeline view of keywords. From: CiteSpace.
According to the burst detection analysis (Figure 9), the strongest keyword burst is “gut microbiota” (13.81), which has emerged as a recent hot topic.
3.6 Analysis of references
CiteSpace was used to perform cluster analysis and visualization of co-cited references. The clustering analysis of co-cited references effectively presents the research themes and emerging hotspots. The top ten most cited references are listed in Table 6, with the most frequently cited being Alicic RZ, 2017 (n=144). Other highly cited articles include Samasu, 2021 (n=126), Perkovic V, 2019 (n=117), Sagoo MK, 2018 (n=91), Jha JC, 2016 (n=86), and Umanath K, 2018 (n=82). The map of cited references shows that the larger the circle, the more frequent the citation (Figure 10A). In the cluster analysis (Figure 10B), seven clusters were identified, labeled as follows: #0 DKD, #1 Targeting Inflammation, #2 Mesangial Cell, #3 NADPH Oxidase, #4 ATF-CHOP Pathway, #5 Sodium-Glucose Cotransporter, and #6 Mitochondrial Dysfunction. These findings suggest that the current research hotspots in this field focus on therapeutic prospects and extracellular matrix accumulation. The timeline of reference clusters (Figure 10C) illustrates the evolution of these clusters over time. In the burst detection analysis of co-cited references (Figure 10D), the most significant citation burst was observed for Alicic RZ, 2017 (48.91). Recent citation bursts were observed for the following articles: Umanath K, 2018 (22.6), Forbes JM, 2018 (20.19), Selby NM, 2020 (23.39), Li SW, 2021 (19.81), Tang GY, 2021 (16.58), and Sagoo MK, 2020 (16.58).
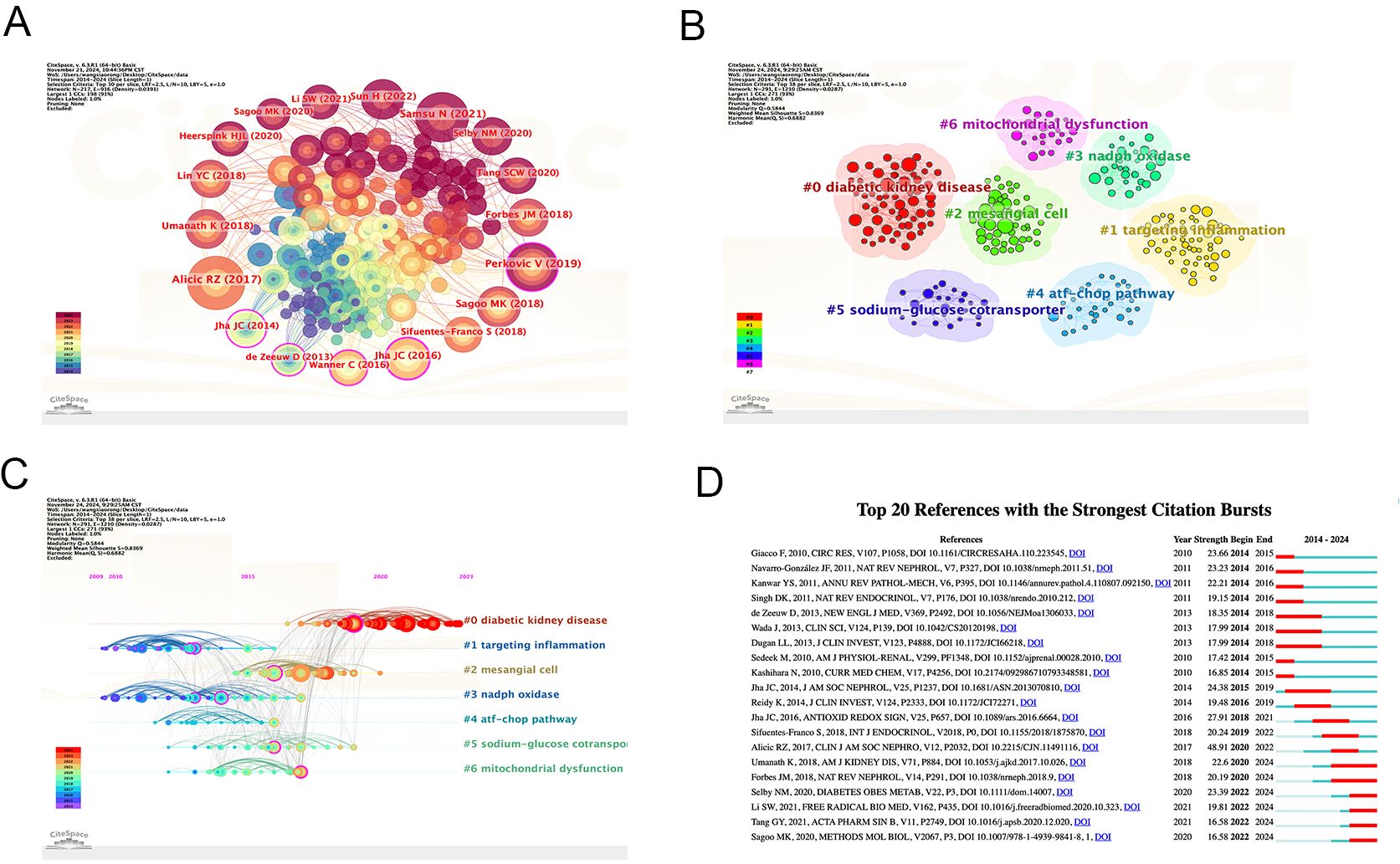
Figure 10. Bibliographic analysis of cited references. (A) Map of cited references; (B) Clustering map of cited reference topics; (C) Timeline view of references; (D) The top 20 references with the strongest citation bursts in research. From: CiteSpace.
4 Discussion
This is the first study to explore the structural correlations and temporal dynamics of DN pathogenesis from the perspective of oxidative stress. Using VOSviewer, the Bibliometrix R package, and CiteSpace, we conducted a comprehensive analysis of publications in this field since 2014, producing visual representations, such as quantitative data on authors, journals, countries/regions, keywords, and references. These results provide valuable insights into the research hotspots and trends in this area.
4.1 Overview
As more individuals with poorly controlled DM develop DN (30, 31), the global focus on understanding the mechanisms of DN has intensified. As a result, the number of publications related to oxidative stress in DN has steadily increased over the past decade, with an average of 408 publications per year. This growth highlights oxidative stress as one of the key pathogenic mechanisms in DN, attracting increasing attention from researchers.
China and the United States lead the research on oxidative stress in DN, supported by their strong economies, which provide significant funding for such studies. Other Asian countries, such as India, Japan, and Iran, have also made notable contributions, likely due to the higher incidence of DN in these populations (32). The top five institutions contributing to this research are all located in China, which may be linked to China’s high DM prevalence and large population of DM patients (33). Although Chinese institutions dominate the academic output in oxidative stress research, their distribution is uneven, with most concentrated in economically developed cities and limited collaboration between them.
Regarding authors, Sun Lin is the leading contributor, with 33 publications. He is affiliated with the Department of Nephrology at Xiangya Second Hospital of Central South University in Hunan, China. His latest study, published in October 2023, investigates how DsbA-L interacts with catalase in peroxisomes to improve tubular oxidative damage in DN. His team demonstrated that DsbA-L can enhance the activity and content of catalase, thereby enriching our understanding of the mechanisms underlying renal tubular injury in DN (34). Additionally, in April 2023, Sun Lin’s team explored mitochondrial oxidative stress and suggested that mitochondrial-activated transcription factor 5 (ATF5) may serve as a potential target for DN prevention (35). Forbes, JM, based at the University of Queensland in Brisbane, Australia, is the most co-cited author in this field, underscoring his authoritative role in this area. However, both Sun Lin and Forbes JM primarily collaborate with domestic scholars in the same field, with limited international cooperation. This lack of global collaboration is a common issue in the field. We believe that international collaboration within the same research domain could foster complementary strengths and should be encouraged to deepen our understanding of DN pathogenesis.
When exploring oxidative stress in DN, key journals, publications, and core authors provide valuable insights. We would first look at high-impact journals with a large number of publications, such as INTERNATIONAL JOURNAL OF MOLECULAR SCIENCES (n=117), FRONTIERS IN PHARMACOLOGY (n=111), and BIOMEDICINE & PHARMACOTHERAPY (n=99). Additionally, searching other core journals (Figure 5B), beyond just the top ten, would provide broader and more specific information to advance our understanding of the field.
4.2 Hotspots and frontiers
Keyword analysis is crucial for identifying research hotspots and trends, as it effectively reflects the core research themes. The most frequent keywords in this study are DN and oxidative stress, which serve as the main axis of the research, with other topics revolving around these two focal points.
In the burst detection analysis, gut microbiota exhibited the strongest burst intensity. Disruption of the gut-kidney axis and microbial metabolites has been reported to exacerbate oxidative stress, accelerating the onset and progression of DN (36). Studies have shown that gut microbiota remodeling, by suppressing oxidative stress and inflammation, can improve DN (37, 38). Other recent emerging keywords include autophagy, as it is known that autophagy and oxidative stress interact to regulate DN kidney injury (39), with more researchers exploring the complex relationship between the two (40). Theodomir et al. pointed out that geniposide enhances ULK1-mediated autophagy to mitigate oxidative stress in DN (41). Keywords with lower burst strength are also worth attention, as they represent emerging research areas. The two keywords with the lowest burst strength are empagliflozin and podocytes. Empagliflozin is a selective SGLT2 inhibitor (42). Related studies have indicated that this drug can improve DN by counteracting hyperglycemia, inhibiting oxidative stress, and reducing inflammation (43). Research by Kelly et al. suggests that empagliflozin may improve DN through both glucose-dependent and independent mechanisms (44). Podocyte injury is a major cause of albuminuria in DN, and oxidative stress plays a crucial role in podocyte damage (45).
Clusters, to some extent, indicate the research frontiers. In the keyword clustering analysis (Figure 8C), the identified clusters include DKD, growth factor beta, DN, DM, lipid peroxidation, and phosphorylation. DKD is a broad term that encompasses various functional and structural kidney changes caused by DM, including DN (46). Oxidative stress plays a pivotal role in both DKD and DN, with chronic hyperglycemia acting as a core trigger and mitochondria serving as the primary site of ROS generation (47). Persistent hyperglycemia disrupts mitochondrial function, causing oxidative pathway dysregulation and excessive ROS production, which ultimately induces oxidative stress (48).
The impact of oxidative stress differs across the stages of DKD and DN. In the early stages of DN, although no significant structural damage is observed, oxidative stress triggered by hyperglycemia begins to exert its effects (49, 50). Excessive advanced glycation end-products (AGEs) and polyol pathway flux activate protein kinase C (PKC) and TGF-β signaling pathways, which further amplify ROS production (51, 52). This results in macromolecular damage to proteins, lipids, and DNA in kidney cells (53, 54). At this stage, ROS functions as a secondary messenger, activating multiple signaling pathways, including TGF-β, and inducing their phosphorylation (55). This process accelerates the depletion of cellular antioxidants, ultimately resulting in the onset of oxidative stress (56). At this stage, oxidative stress primarily impairs renal tubular epithelial cell function, weakens the antioxidant defense system, and causes mild kidney dysfunction (57). For instance, lipid peroxidation of podocyte membranes induced by ROS disrupts cellular integrity, leading to podocyte loss and damage to the glomerular filtration barrier, resulting in microalbuminuria (58). Additionally, ROS generated by oxidative stress in mesangial cells promote extracellular matrix (ECM) deposition, laying the foundation for advanced kidney fibrosis (59, 60).
As DN progresses to later stages, structural damage becomes more pronounced (61), and the effects of oxidative stress intensify (62). Oxidative stress not only exacerbates damage to renal tubular and glomerular endothelial cells but also accelerates renal fibrosis (63). ROS promote tubulointerstitial damage and activate fibroblasts, leading to interstitial fibrosis and eventually causing severe structural and functional kidney impairment (64). Specifically, oxidative stress disrupts the tight junctions of glomerular endothelial cells, increasing vascular permeability and allowing large molecules, such as proteins, to leak into the urine, thereby worsening proteinuria. Additionally, oxidative stress alters hemodynamics, further aggravating glomerular injury (65). As tubulointerstitial damage and fibrosis advance, the role of oxidative stress in late-stage DN becomes increasingly pronounced (66).
Co-cited references represent the background and baseline of the research field. The most frequently cited reference is Alicic RZ, 2017, which systematically investigates the risk factors and pathophysiological changes of DN (9). This study exhibits the strongest citation burst and lays a solid theoretical foundation for further research on DN. In the reference cluster analysis, Cluster 1 focuses on targeting inflammation, with oxidative stress being one of the main drivers of inflammatory responses (67). Excessive ROS activate inflammatory signaling pathways such as NF-κB and MAPK, promoting the release of inflammatory mediators and exacerbating renal injury (11, 68). Meanwhile, inflammatory factors such as TNF-α and IL-1β activate NADPH oxidase, leading to increased ROS production and forming a positive feedback loop between oxidative damage and inflammation, thereby accelerating DN progression (69). Cluster 3 focuses on NADPH oxidase, the only known enzyme family responsible for ROS production, which includes seven isoforms: Nox1, Nox2, Nox3, Nox4, Nox5, DUOX1, and DUOX2 (70–72). Among these, Nox4 is a major research target and has been extensively studied (73). Mitochondrial dysfunction (#6) is characterized by excessive ROS production within the mitochondria, a reduction in ATP, mutations in mitochondrial DNA, and subsequent mitochondrial swelling and rupture (74). Excessive ROS modulate the phosphorylation of Bcl-2 proteins, which induces changes in mitochondrial membrane permeability, ultimately leading to mitochondrial dysfunction (75). Reference clusters offer valuable guidance for future research directions.
In recent years, inhibiting oxidative stress has become a major therapeutic strategy for DN. The antioxidant bioactivity of medicinal plants in DN treatment warrants attention. Studies have shown that mushrooms collected from Turkey exhibit strong antioxidant potential (76). The round-stemmed mushroom from safe areas has also been confirmed as a good source of antioxidants (77). Bahare et al. reported that Achillea spp. demonstrates powerful antioxidant effects in both cell and animal models (78). Propolis, a natural remedy, has also shown potential in improving kidney function by mitigating oxidative damage (79). Other studies have explored novel synthetic organic selenium compounds that exhibit antioxidant effects on rat kidneys (80). Hydrogen sulfide has also been shown to protect kidneys by reducing ROS and inhibiting oxidative stress (81, 82). Metformin and angiotensin receptor blockers (ARBs) have also been found to reduce oxidative stress markers and enhance antioxidant enzyme activity in the kidney, providing therapeutic benefits for DN (83, 84). Traditional Chinese Medicine (TCM) has significantly contributed to the treatment of DN. 6-Shogaol, a major active component of ginger, inhibits oxidative stress and restores Nrf2 expression in the kidneys, thereby improving DN (85). Mao et al. demonstrated that Huangkui capsules protect the kidneys by controlling MDA, SOD, and Nox4 expression in DN (86). Tongxinluo, a novel Chinese herbal compound, significantly reduces Nox4 expression in DN rats, inhibits oxidative stress, and decreases podocyte apoptosis (87). Zuogui Wan has been shown to protect kidneys by reducing oxidative stress and inhibiting apoptosis in DN (88).
5 Strengths and limitations
In comparison to traditional reviews, this study utilizes statistical techniques to analyze 4076 publications, providing a more intuitive and comprehensive reflection of the research hotspots and development trends in this field from 2014 to the present. The results provide valuable insights for researchers. However, this approach has some limitations. Although the WoSCC database is one of the most commonly used and authoritative databases, its data resources represent only a specific scope of a particular field. Additionally, this study analyzed only English-language publications, which may have excluded important articles published in other languages. Finally, the existence of synonyms could introduce bias into the results. To mitigate these issues, the search strategy must be used with caution. Nonetheless, from a bibliometric perspective, we believe that our results accurately reflect the research on oxidative stress related to DN since 2014.
6 Conclusion
In conclusion, since 2014, publications focusing on the role of oxidative stress in DN have steadily increased, underscoring its pivotal role in DN pathogenesis. Oxidative stress drives kidney damage through mechanisms like lipid peroxidation, mitochondrial dysfunction, and inflammation, making it a central target for therapeutic research. This study provides critical insights for basic scientists to identify research trends, prioritize key areas, and facilitate future investigations into molecular mechanisms and the development of innovative antioxidant therapies. A deeper understanding of these processes is essential for advancing treatment strategies and improving clinical outcomes in DN patients.
Data availability statement
The original contributions presented in the study are included in the article/supplementary material. Further inquiries can be directed to the corresponding authors.
Author contributions
XW: Conceptualization, Methodology, Writing – original draft. ZW: Funding acquisition, Methodology, Writing – original draft. TH: Conceptualization, Funding acquisition, Writing – original draft. XC: Visualization, Writing – review & editing. XJ: Visualization, Writing – review & editing. CZ: Writing – review & editing. SY: Writing – review & editing. YG: Visualization, Writing – review & editing. XZ: Conceptualization, Project administration, Supervision, Writing – review & editing. WG: Conceptualization, Funding acquisition, Project administration, Supervision, Writing – review & editing.
Funding
The author(s) declare financial support was received for the research, authorship, and/or publication of this article. This work was funded by the Major Special Project of National Natural Science Foundation of China (grant number 32141005), the Hebei University of Chinese Medicine 2024 Graduate Student Innovation Funding Project (grant number XCXZZBS2024001), and the Hebei University of Chinese Medicine 2023 Graduate Student Innovation Funding Project (grant number CXZZSS2023087).
Conflict of interest
The authors declare that the research was conducted in the absence of any commercial or financial relationships that could be construed as a potential conflict of interest.
Publisher’s note
All claims expressed in this article are solely those of the authors and do not necessarily represent those of their affiliated organizations, or those of the publisher, the editors and the reviewers. Any product that may be evaluated in this article, or claim that may be made by its manufacturer, is not guaranteed or endorsed by the publisher.
References
1. Sun H, Saeedi P, Karuranga S, Pinkepank M, Ogurtsova K, Duncan BB, et al. IDF Diabetes Atlas: Global, regional and country-level diabetes prevalence estimates for 2021 and projections for 2045. Diabetes Res Clin Pract. (2022) 183:109119. doi: 10.1016/j.diabres.2021.109119
2. Yu MG, Gordin D, Fu J, Park K, Li Q, King GL. Protective factors and the pathogenesis of complications in diabetes. Endocrine Rev. (2024) 45:227. doi: 10.1210/endrev/bnad030
3. American Diabetes Association. Diagnosis and classification of diabetes mellitus. Diabetes Care. (2013) 36:S67–74. doi: 10.2337/dc13-S067
4. Tang A, Zhang Y, Wu L, Lin Y, Lv L, Zhao L, et al. Klotho’s impact on diabetic nephropathy and its emerging connection to diabetic retinopathy. Front Endocrinol (Lausanne). (2023) 14:1180169. doi: 10.3389/fendo.2023.1180169
5. Paul P, Chacko L, Dua TK, Chakraborty P, Paul U, Phulchand VV, et al. Nanomedicines for the management of diabetic nephropathy: present progress and prospects. Front Endocrinol (Lausanne). (2023) 14:1236686. doi: 10.3389/fendo.2023.1236686
6. Wang H, Zhang R, Wu X, Chen Y, Ji W, Wang J, et al. The wnt signaling pathway in diabetic nephropathy. Front Cell Dev Biol. (2022) 9:701547. doi: 10.3389/fcell.2021.701547
7. Jha JC, Banal C, Chow BSM, Cooper ME, Jandeleit-Dahm K. Diabetes and kidney disease: role of oxidative stress. Antioxid Redox Signal. (2016) 25:657–84. doi: 10.1089/ars.2016.6664
8. Darenskaya M, Kolesnikov S, Semenova N, Kolesnikova L. Diabetic nephropathy: significance of determining oxidative stress and opportunities for antioxidant therapies. Int J Mol Sci. (2023) 24:12378. doi: 10.3390/ijms241512378
9. Alicic RZ, Rooney MT, Tuttle KR. Diabetic kidney disease. Clin J Am Soc Nephrol. (2017) 12:2032–45. doi: 10.2215/CJN.11491116
10. Samsu N. Diabetic nephropathy: challenges in pathogenesis, diagnosis, and treatment. BioMed Res Int. (2021) 2021:1497449. doi: 10.1155/2021/1497449
11. Jin Q, Liu T, Qiao Y, Liu D, Yang L, Mao H, et al. Oxidative stress and inflammation in diabetic nephropathy: role of polyphenols. Front Immunol. (2023) 14:1185317. doi: 10.3389/fimmu.2023.1185317
12. Wang N, Zhang C. Oxidative stress: A culprit in the progression of diabetic kidney disease. Antioxid (Basel). (2024) 13:455. doi: 10.3390/antiox13040455
13. Su S, Ma Z, Wu H, Xu Z, Yi H. Oxidative stress as a culprit in diabetic kidney disease. Life Sci. (2023) 322:121661. doi: 10.1016/j.lfs.2023.121661
14. Goycheva P, Petkova-Parlapanska K, Georgieva E, Karamalakova Y, Nikolova G. Biomarkers of oxidative stress in diabetes mellitus with diabetic nephropathy complications. Int J Mol Sci. (2023) 24:13541. doi: 10.3390/ijms241713541
15. Sakashita M, Tanaka T, Inagi R. Metabolic changes and oxidative stress in diabetic kidney disease. Antioxid (Basel). (2021) 10:1143. doi: 10.3390/antiox10071143
16. Mladenov M, Lubomirov L, Grisk O, Avtanski D, Mitrokhin V, Sazdova I, et al. Oxidative stress, reductive stress and antioxidants in vascular pathogenesis and aging. Antioxid (Basel). (2023) 12:1126. doi: 10.3390/antiox12051126
17. Forbes JM, Coughlan MT, Cooper ME. Oxidative stress as a major culprit in kidney disease in diabetes. Diabetes. (2008) 57:1446–54. doi: 10.2337/db08-0057
18. Wu J, Wang Z, Cai M, Wang X, Lo B, Li Q, et al. GPR56 promotes diabetic kidney disease through eNOS regulation in glomerular endothelial cells. Diabetes. (2023) 72:1652–63. doi: 10.2337/db23-0124
19. Liao J, Liu B, Chen K, Hu S, Liu Z-Y, Li Y-X, et al. Galangin attenuates oxidative stress-mediated apoptosis in high glucose-induced renal tubular epithelial cells through modulating renin–angiotensin system and PI3K/AKT/mTOR pathway. Toxicol Res (Camb). (2021) 10:551–60. doi: 10.1093/toxres/tfab009
20. Jia M, Lin L, Xun K, Li D, Wu W, Sun S, et al. Indoxyl sulfate aggravates podocyte damage through the TGF-β1/Smad/ROS signaling pathway. Kidney Blood Pressure Res. (2024) 1:385–96. doi: 10.1159/000538858
21. Selamoglu Z, Dusgun C, Akgul H, Gulhan MF. In-vitro antioxidant activities of the ethanolic extracts of some contained-allantoin plants. Iran J Pharm Res. (2017) 16:92–8.
22. Ellegaard O, Wallin JA. The bibliometric analysis of scholarly production: How great is the impact? Scientometrics. (2015) 105:1809–31. doi: 10.1007/s11192-015-1645-z
23. Wang S, Zhou H, Zheng L, Zhu W, Zhu L, Feng D, et al. Global trends in research of macrophages associated with acute lung injury over past 10 years: A bibliometric analysis. Front Immunol. (2021) 12:669539. doi: 10.3389/fimmu.2021.669539
24. Zhang L, Zhang H, Xie Q, Xiong S, Jin F, Zhou F, et al. A bibliometric study of global trends in diabetes and gut flora research from 2011 to 2021. Front Endocrinol (Lausanne). (2022) 13:990133. doi: 10.3389/fendo.2022.990133
25. Ke L, Lu C, Shen R, Lu T, Ma B, Hua Y. Knowledge mapping of drug-induced liver injury: A scientometric investigation (2010–2019). Front Pharmacol. (2020) 11:842. doi: 10.3389/fphar.2020.00842
26. Chen C, Song M. Visualizing a field of research: A methodology of systematic scientometric reviews. PloS One. (2019) 14(10):e0223994. doi: 10.1371/journal.pone.0223994
27. van Eck NJ, Waltman L. Software survey: VOSviewer, a computer program for bibliometric mapping. Scientometrics. (2010) 84:523–38. doi: 10.1007/s11192-009-0146-3
28. Chen C, Chen Y. Searching for clinical evidence in citeSpace. AMIA Annu Symp Proc. (2005) 2005:121–5.
29. Aria M, Cuccurullo C. bibliometrix: An R-tool for comprehensive science mapping analysis. J Informetrics. (2017) 11:959–75. doi: 10.1016/j.joi.2017.08.007
30. Espinel E, Agraz I, Ibernon M, Ramos N, Fort J, Serón D. Renal biopsy in type 2 diabetic patients. J Clin Med. (2015) 4:998–1009. doi: 10.3390/jcm4050998
31. Lytvyn Y, Bjornstad P, Pun N, Maahs DM, Perkins B, Cherney DZI. New and old agents in the management of diabetic nephropathy. Curr Opin Nephrol Hypertens. (2016) 25:232–9. doi: 10.1097/MNH.0000000000000214
32. Kopel J, Pena-Hernandez C, Nugent K. Evolving spectrum of diabetic nephropathy. World J Diabetes. (2019) 10:269–79. doi: 10.4239/wjd.v10.i5.269
33. Xu J, Chen F, Liu T, Wang T, Zhang J, Yuan H, et al. Brain functional networks in type 2 diabetes mellitus patients: A resting-state functional MRI study. Front Neurosci. (2019) 13:239. doi: 10.3389/fnins.2019.00239
34. Liu Y, Chen W, Li C, Li L, Yang M, Jiang N, et al. DsbA-L interacting with catalase in peroxisome improves tubular oxidative damage in diabetic nephropathy. Redox Biol. (2023) 66:102855. doi: 10.1016/j.redox.2023.102855
35. Liu Y, Zhang L, Zhang S, Liu J, Li X, Yang K, et al. ATF5 regulates tubulointerstitial injury in diabetic kidney disease via mitochondrial unfolded protein response. Mol Med. (2023) 29:57. doi: 10.1186/s10020-023-00651-4
36. Zhang L, Wang Z, Zhang X, Zhao L, Chu J, Li H, et al. Alterations of the gut microbiota in patients with diabetic nephropathy. Microbiol Spectr. (2022) 10:e00324–22. doi: 10.1128/spectrum.00324-22
37. Cai T-T, Ye X-L, Li R-R, Chen H, Wang Y-Y, Yong H-J, et al. Resveratrol modulates the gut microbiota and inflammation to protect against diabetic nephropathy in mice. Front Pharmacol. (2020) 11:1249. doi: 10.3389/fphar.2020.01249
38. Huang W, Man Y, Gao C, Zhou L, Gu J, Xu H, et al. Short-chain fatty acids ameliorate diabetic nephropathy via GPR43-mediated inhibition of oxidative stress and NF-κB signaling. Oxid Med Cell Longev. (2020) 2020:4074832. doi: 10.1155/2020/4074832
39. Han Y-P, Liu L-J, Yan J-L, Chen M-Y, Meng X-F, Zhou X-R, et al. Autophagy and its therapeutic potential in diabetic nephropathy. Front Endocrinol (Lausanne). (2023) 14:1139444. doi: 10.3389/fendo.2023.1139444
40. Sureshbabu A, Ryter SW, Choi ME. Oxidative stress and autophagy: Crucial modulators of kidney injury. Redox Biol. (2015) 4:208–14. doi: 10.1016/j.redox.2015.01.001
41. Dusabimana T, Park EJ, Je J, Jeong K, Yun SP, Kim HJ, et al. Geniposide improves diabetic nephropathy by enhancing ULK1-mediated autophagy and reducing oxidative stress through AMPK activation. Int J Mol Sci. (2021) 22:1651. doi: 10.3390/ijms22041651
42. Deng L, Yang Y, Xu G. Empagliflozin ameliorates type 2 diabetes mellitus-related diabetic nephropathy via altering the gut microbiota. Biochim Biophys Acta (BBA) Mol Cell Biol Lipids. (2022) 1867:159234. doi: 10.1016/j.bbalip.2022.159234
43. Mujalli A, Farrash WF, Obaid AA, Khan AA, Almaimani RA, Idris S, et al. Improved glycaemic control and nephroprotective effects of empagliflozin and paricalcitol co-therapy in mice with type 2 diabetes mellitus. Int J Mol Sci. (2023) 24:17380. doi: 10.3390/ijms242417380
44. Hudkins KL, Li X, Holland AL, Swaminathan S, Alpers CE. Regression of diabetic nephropathy by treatment with empagliflozin in BTBR ob/ob mice. Nephrol Dialysis Transplant. (2022) 37:847–59. doi: 10.1093/ndt/gfab330
45. Barutta F, Bellini S, Kimura S, Hase K, Corbetta B, Corbelli A, et al. Protective effect of the tunneling nanotube-TNFAIP2/M-sec system on podocyte autophagy in diabetic nephropathy. Autophagy. (2023) 19:505–24. doi: 10.1080/15548627.2022.2080382
46. Di Paolo S, Fiorentino M, De Nicola L, Reboldi G, Gesualdo L, Barutta F, et al. Indications for renal biopsy in patients with diabetes. Joint position statement of the Italian Society of Nephrology and the Italian Diabetes Society. Nutrition Metab Cardiovasc Dis. (2020) 30:2123–32. doi: 10.1016/j.numecd.2020.09.013
47. Ratliff BB, Abdulmahdi W, Pawar R, Wolin MS. Oxidant mechanisms in renal injury and disease. Antioxid Redox Signal. (2016) 25:119–46. doi: 10.1089/ars.2016.6665
48. Park J, Seo E, Jun H-S. Bavachin alleviates diabetic nephropathy in db/db mice by inhibition of oxidative stress and improvement of mitochondria function. Biomed Pharmacother. (2023) 161:114479. doi: 10.1016/j.biopha.2023.114479
49. Abd El-Hameed AM. Polydatin-loaded chitosan nanoparticles ameliorates early diabetic nephropathy by attenuating oxidative stress and inflammatory responses in streptozotocin-induced diabetic rat. J Diabetes Metab Disord. (2020) 19:1599–607. doi: 10.1007/s40200-020-00699-7
50. Kishore L, Kaur N, Singh R. Distinct biomarkers for early diagnosis of diabetic nephropathy. Curr Diabetes Rev. (2017) 13:598–605. doi: 10.2174/1573399812666161207123007
51. Lin Y-C, Chang Y-H, Yang S-Y, Wu K-D, Chu T-S. Update of pathophysiology and management of diabetic kidney disease. J Formosan Med Assoc. (2018) 117:662–75. doi: 10.1016/j.jfma.2018.02.007
52. Jung C-Y, Yoo T-H. Pathophysiologic mechanisms and potential biomarkers in diabetic kidney disease. Diabetes Metab J. (2022) 46:181–97. doi: 10.4093/dmj.2021.0329
53. Verma S, Singh P, Khurana S, Ganguly NK, Kukreti R, Saso L, et al. Implications of oxidative stress in chronic kidney disease: a review on current concepts and therapies. Kidney Res Clin Pract. (2021) 40:183–93. doi: 10.23876/j.krcp.20.163
54. Honda T, Hirakawa Y, Nangaku M. The role of oxidative stress and hypoxia in renal disease. Kidney Res Clin Pract. (2019) 38:414–26. doi: 10.23876/j.krcp.19.063
55. Hou Y, Shi Y, Han B, Liu X, Qiao X, Qi Y, et al. The antioxidant peptide SS31 prevents oxidative stress, downregulates CD36 and improves renal function in diabetic nephropathy. Nephrol Dialysis Transplant. (2018) 33:1908–18. doi: 10.1093/ndt/gfy021
56. Ma X, Ma J, Leng T, Yuan Z, Hu T, Liu Q, et al. Advances in oxidative stress in pathogenesis of diabetic kidney disease and efficacy of TCM intervention. Ren Fail. (2023) 45:2146512. doi: 10.1080/0886022X.2022.2146512
57. Al Hroob AM, Abukhalil MH, Alghonmeen RD, Mahmoud AM. Ginger alleviates hyperglycemia-induced oxidative stress, inflammation and apoptosis and protects rats against diabetic nephropathy. Biomed Pharmacother. (2018) 106:381–9. doi: 10.1016/j.biopha.2018.06.148
58. Chen J, Zhang Q, Guo J, Gu D, Liu J, Luo P, et al. Single-cell transcriptomics reveals the ameliorative effect of rosmarinic acid on diabetic nephropathy-induced kidney injury by modulating oxidative stress and inflammation. Acta Pharm Sin B. (2024) 14:1661–76. doi: 10.1016/j.apsb.2024.01.003
59. Wang K, Zheng X, Pan Z, Yao W, Gao X, Wang X, et al. Icariin Prevents Extracellular Matrix Accumulation and Ameliorates Experimental Diabetic Kidney Disease by Inhibiting Oxidative Stress via GPER Mediated p62-Dependent Keap1 Degradation and Nrf2 Activation. Front Cell Dev Biol. (2020) 8:559. doi: 10.3389/fcell.2020.00559
60. Liu Y, Li H, Wang S, Yin W, Wang Z. Ibrolipim attenuates early-stage nephropathy in diet-induced diabetic minipigs: Focus on oxidative stress and fibrogenesis. Biomed Pharmacother. (2020) 129:110321. doi: 10.1016/j.biopha.2020.110321
61. Kriz W, Löwen J, Gröne H-J. The complex pathology of diabetic nephropathy in humans. Nephrol Dial Transplant. (2023) 38:2109–19. doi: 10.1093/ndt/gfad052
62. Gupta S, Dominguez M, Golestaneh L. Diabetic kidney disease: an update. Med Clin North Am. (2023) 107:689–705. doi: 10.1016/j.mcna.2023.03.004
63. Deng J, Liu Y, Liu Y, Li W, Nie X. The multiple roles of fibroblast growth factor in diabetic nephropathy. J Inflammation Res. (2021) 14:5273–90. doi: 10.2147/JIR.S334996
64. Xue R, Xiao H, Kumar V, Lan X, Malhotra A, Singhal PC, et al. The molecular mechanism of renal tubulointerstitial inflammation promoting diabetic nephropathy. Int J Nephrol Renovasc Dis. (2023) 16:241–52. doi: 10.2147/IJNRD.S436791
65. Shen Y, Chen W, Lin K, Zhang H, Guo X, An X, et al. Notoginsenoside Fc, a novel renoprotective agent, ameliorates glomerular endothelial cells pyroptosis and mitochondrial dysfunction in diabetic nephropathy through regulating HMGCS2 pathway. Phytomedicine. (2024) 126:155445. doi: 10.1016/j.phymed.2024.155445
66. Liu Z, Nan P, Gong Y, Tian L, Zheng Y, Wu Z. Endoplasmic reticulum stress-triggered ferroptosis via the XBP1-Hrd1-Nrf2 pathway induces EMT progression in diabetic nephropathy. Biomed Pharmacother. (2023) 164:114897. doi: 10.1016/j.biopha.2023.114897
67. Shang Y, Yan C-Y, Li H, Liu N, Zhang H-F. Tiliroside protects against diabetic nephropathy in streptozotocin-induced diabetes rats by attenuating oxidative stress and inflammation. World J Diabetes. (2024) 15:2220–36. doi: 10.4239/wjd.v15.i11.2220
68. Wu X, Pan C, Chen R, Zhang S, Zhai Y, Guo H. BML-111 attenuates high glucose-induced inflammation, oxidative stress and reduces extracellular matrix accumulation via targeting Nrf2 in rat glomerular mesangial cells. Int Immunopharmacol. (2020) 79:106108. doi: 10.1016/j.intimp.2019.106108
69. Zhuang L-G, Zhang R, Jin G-X, Pei X-Y, Wang Q, Ge X-X. Asiaticoside improves diabetic nephropathy by reducing inflammation, oxidative stress, and fibrosis: An in vitro and in vivo study. World J Diabetes. (2024) 15:2111–22. doi: 10.4239/wjd.v15.i10.2111
70. Wingler K, Hermans J, Schiffers P, Moens A, Paul M, Schmidt H. NOX1, 2, 4, 5: counting out oxidative stress. Br J Pharmacol. (2011) 164:866–83. doi: 10.1111/j.1476-5381.2011.01249.x
71. Lee ES, Kim HM, Lee SH, Ha KB, Bae YS, Lee SJ, et al. APX-115, a pan-NADPH oxidase inhibitor, protects development of diabetic nephropathy in podocyte specific NOX5 transgenic mice. Free Radical Biol Med. (2020) 161:92–101. doi: 10.1016/j.freeradbiomed.2020.09.024
72. Njeim R, Alkhansa S, Fornoni A. Unraveling the crosstalk between lipids and NADPH oxidases in diabetic kidney disease. Pharmaceutics. (2023) 15:1360. doi: 10.3390/pharmaceutics15051360
73. Yang Q, Wu F, Wang J, Gao L, Jiang L, Li H-D, et al. Nox4 in renal diseases: An update. Free Radical Biol Med. (2018) 124:466–72. doi: 10.1016/j.freeradbiomed.2018.06.042
74. Zhang PN, Zhou MQ, Guo J, Zheng HJ, Tang J, Zhang C, et al. Mitochondrial dysfunction and diabetic nephropathy: nontraditional therapeutic opportunities. J Diabetes Res. (2021) 2021:1010268. doi: 10.1155/2021/1010268
75. Kakkar P, Singh BK. Mitochondria: a hub of redox activities and cellular distress control. Mol Cell Biochem. (2007) 305:235–53. doi: 10.1007/s11010-007-9520-8
76. Sevindik M, Akgul H, Selamoglu Z, Braidy N. Antioxidant and antigenotoxic potential of infundibulicybe geotropa mushroom collected from northwestern Turkey. Oxid Med Cell Longev. (2020) 2020:5620484. doi: 10.1155/2020/5620484
77. Sevindik M, Akgul H, Bal C, Selamoglu Z. Phenolic contents, oxidant/antioxidant potential and heavy metal levels in cyclocybe cylindracea. Indian J Pharm Educ Res. (2018) 52:437–41. 10.5530/ijper.52doi: .3.50
78. Salehi B, Selamoglu Z, Sevindik M, Fahmy NM, Al-Sayed E, El-Shazly M, et al. Achillea spp.: A comprehensive review on its ethnobotany, phytochemistry, phytopharmacology and industrial applications. Cell Mol Biol (Noisy-le-grand). (2020) 66:78–103. doi: 10.14715/cmb/2020.66.4.13
79. Talas ZS, Ozdemir I, Ciftci O, Cakir O, Gulhan MF, Pasaoglu OM. Role of propolis on biochemical parameters in kidney and heart tissues against l-NAME induced oxidative injury in rats. Clin Exp Hypertension. (2014) 36:492–6. doi: 10.3109/10641963.2013.863322
80. Talas ZS, Ozdemir I, Yilmaz I, Gok Y. Antioxidative effects of novel synthetic organoselenium compound in rat lung and kidney. Ecotoxicol Environ Saf. (2009) 72:916–21. doi: 10.1016/j.ecoenv.2007.11.012
81. Ahmed HH, Taha FM, Omar HS, Elwi HM, Abdelnasser M. Hydrogen sulfide modulates SIRT1 and suppresses oxidative stress in diabetic nephropathy. Mol Cell Biochem. (2019) 457:1–9. doi: 10.1007/s11010-019-03506-x
82. Elbassuoni EA, Аziz NM, Habeeb WN. The role of activation of KАTP channels on hydrogen sulfide induced renoprotective effect on diabetic nephropathy. J Cell Physiol. (2020) 235:5223–8. doi: 10.1002/jcp.29403
83. Alhaider AA, Korashy HM, Sayed-Ahmed MM, Mobark M, Kfoury H, Mansour MA. Metformin attenuates streptozotocin-induced diabetic nephropathy in rats through modulation of oxidative stress genes expression. Chemico-Biological Interact. (2011) 192:233–42. doi: 10.1016/j.cbi.2011.03.014
84. Ren H, Shao Y, Wu C, Ma X, Lv C, Wang Q. Metformin alleviates oxidative stress and enhances autophagy in diabetic kidney disease via AMPK/SIRT1-FoxO1 pathway. Mol Cell Endocrinol. (2020) 500:110628. doi: 10.1016/j.mce.2019.110628
85. Xu Y, Bai L, Chen X, Li Y, Qin Y, Meng X, et al. 6-Shogaol ameliorates diabetic nephropathy through anti-inflammatory, hyperlipidemic, anti-oxidative activity in db/db mice. Biomed Pharmacother. (2018) 97:633–41. doi: 10.1016/j.biopha.2017.10.084
86. Mao Z-M, Shen S-M, Wan Y-G, Sun W, Chen H-L, Huang M-M, et al. Huangkui capsule attenuates renal fibrosis in diabetic nephropathy rats through regulating oxidative stress and p38MAPK/Akt pathways, compared to α-lipoic acid. J Ethnopharmacol. (2015) 173:256–65. doi: 10.1016/j.jep.2015.07.036
87. Cui F, Gao Y, Zhao W, Zou D, Zhu Z, Wu X, et al. Effect of tongxinluo on podocyte apoptosis via inhibition of oxidative stress and P38 pathway in diabetic rats. Evid Based Complement Alternat Med. (2016) 2016:5957423. doi: 10.1155/2016/5957423
Keywords: diabetic nephropathy, oxidative stress, VOSviewer, Bibliometrix R package, CiteSpace
Citation: Wang X-r, Wu Z, He T-t, Chen X-h, Jin X-f, Zuo C-y, Yang S-z, Gao Y, Zhou X-h and Gao W-j (2025) Global research hotspots and trends in oxidative stress-related diabetic nephropathy: a bibliometric study. Front. Endocrinol. 15:1451954. doi: 10.3389/fendo.2024.1451954
Received: 20 June 2024; Accepted: 19 December 2024;
Published: 10 January 2025.
Edited by:
Ricardo Espinosa-Tanguma, Autonomous University of San Luis Potosí, MexicoReviewed by:
Zeliha Selamoglu, Niğde Ömer Halisdemir University, TürkiyeDenisha R. Spires, Augusta University, United States
Katy Sanchez-Pozos, Hospital Juárez de México, Mexico
Copyright © 2025 Wang, Wu, He, Chen, Jin, Zuo, Yang, Gao, Zhou and Gao. This is an open-access article distributed under the terms of the Creative Commons Attribution License (CC BY). The use, distribution or reproduction in other forums is permitted, provided the original author(s) and the copyright owner(s) are credited and that the original publication in this journal is cited, in accordance with accepted academic practice. No use, distribution or reproduction is permitted which does not comply with these terms.
*Correspondence: Xiao-hong Zhou, enhoMTk3MDNAMTYzLmNvbQ==; Wei-juan Gao, Z3dqNjA4OEAxNjMuY29t
†These authors have contributed equally to this work and share first authorship