- 1Department of Endocrinology, Carol Davila University of Medicine and Pharmacy, Bucharest, Romania
- 2Department of Endocrinology and Diabetes, Elias Emergency University Hospital, Bucharest, Romania
- 3General Surgery Department, Ponderas Hospital, Bucharest, Romania
Introduction: Renal dysfunction is a recognized complication of obesity with an incompletely characterized pathophysiology. Improvement of glomerular filtration rate (GFR) after metabolic and bariatric surgery (MBS) has been reported across all classes of renal function. Inter-gender differences with regard to correlates of renal function have been described, but the influence of body composition is an understudied area. We aimed to explore determinants of renal function in obesity and to assess its variations after MBS, with a focus on body composition parameters in males and females, respectively.
Materials, methods: We conducted a retrospective study on 196 patients who underwent laparoscopic sleeve gastrectomy, evaluated preoperatively and 6 months after the intervention. Recorded data included clinical and biochemical assessment, as well as body composition estimation via dual-energy X-ray absorptiometry. Serum creatinine-based formulas were used for the estimation of GFR.
Results: We included a total of 196 patients (80 males and 116 females), with a mean age of 41.43 ± 10.79. Median baseline body mass index was 42.6 (6.61) kg/m2 and 6 months excess weight loss (EWL) reached 71.43 ± 17.18%, in females, estimated GFR correlated negatively with visceral adipose tissue (VAT) mass (rho=-.368) and this correlation was stronger in females with type 2 diabetes mellitus. Moreover, women in the third VAT mass tertile were 5 times more likely to have reduced GFR compared to the first tertile. Renal function improved after MBS across all classes of filtration. In males, this improvement correlated with EWL (rho=.358) and lean mass variation (rho=-.412), while in females it correlated with VAT mass variation (rho=-.266).
Conclusions: Our results are consistent with previous findings on the positive impact of MBS on renal function and suggest a more prominent impact of visceral adiposity on GFR in females.
1 Introduction
Obesity is a multisystem disease, that has undoubtedly reached pandemic proportions. In 2020, there were over 800 million adults living with obesity worldwide and current trends predict a prevalence of over 1.5 billion in 2035 (1). Parallelling the obesity epidemic, chronic kidney disease is also a major cause of morbi-mortality worldwide (2). Multiple epidemiologic studies have shown that excessive body weight is a risk factor for both the onset and the progression of kidney disease across all ages and across a wide range of co-existing pathologies (3–9).
A more in-depth analysis of this relationship reveals that the two entities are, in fact, intertwined, involving complex and multilayered mechanisms of augmenting the detrimental effects of one another (3, 10–12). Thus, research in the field of obesity-related renal dysfunction is challenging and the issue remains incompletely explored, despite the fact that the interest in this topic dates from decades ago (13). The diversity of body composition among patients with similar body mass index (BMI), including varying proportions of adipose tissue components (e.g. visceral vs subcutaneous adiposity) is widely acknowledged (14). The distinct influences body compartments exert on renal parameters might prove useful in shedding more light on this adipo-renal relationship.
Metabolic and bariatric surgery (MBS) is currently the most effective intervention in severe obesity, with clear benefits on related comorbidities (15). Among these, the postoperative improvement of renal function has been reported in the past years and a number of authors regard MBS as a short and long-term renoprotective intervention (16). Specifically, MBS is associated with improved glomerular filtration rate (GFR), decreased albuminuria and a lower rate of progression to end-stage-renal disease upon follow-up (17, 18).
In the current study, we aimed to explore baseline determinants of renal function, focusing on body composition parameters, as well as short-term postoperative dynamics in patients with obesity undergoing laparoscopic sleeve gastrectomy (LSG).
2 Methods
We conducted a retrospective study on a cohort of adult patients with obesity who benefited from LSG at an International Federation for the Surgery of Obesity and Metabolic Disorders (IFSO) accredited Center of Excellence in Romania. The study followed the standards of the Helsinki Committee for Human Rights and the design received approval from the local ethics committee. All patients provided written informed consent. Evaluations were performed preoperatively and 6 months after the intervention.
Inclusion criteria consisted of adult age (>18 years old) and meeting the indication for MBS as per guideline recommendations (19). Exclusion criteria were represented by previous bariatric therapy, documented kidney disease, family history positive for hereditary kidney disease, usage of nephrotoxic medication during the past year and excessive alcohol consumption (defined as 5 or more drinks on any day or 15 or more per week for men and 4 or more on any day or 8 or more drinks per week for women) (20). Of the 268 patients initially included, 33 had incomplete baseline evaluation, while an additional 39 were either lost to follow-up or had incomplete evaluation during their second visit. Consequently, 196 patients were analyzed in the present study.
2.1 Clinical and biological evaluation
Body weight and height were measured (both at baseline and postoperatively) using a standardized digital scale with fixed stadiometer. BMI was calculated as weight (in kilograms) divided by the square of height (in meters). Excess weight loss percentage (%EWL) was calculated as [(Baseline BMI − Postoperative BMI)/(Baseline BMI − 25)] × 100, as defined in literature (21). Arterial blood pressure (ABP) was assessed in-office, at brachial artery level, with the patient in seating position, using an electronic sphygmomanometer. Hypertension (HTN) was defined as systolic ABP ≥140 mmHg, diastolic ABP ≥90 mmHg, or antihypertensive medication use following a previously established diagnosis. Postoperative improvement of ABP was defined as normalized ABP with consistent or de-escalated medical treatment. Diabetes was defined per American Diabetes Association criteria (22) or by the use of anti-hyperglycemic drugs or medical nutritional therapy following a previously established positive diagnosis. Improvement of glycemic control was defined as improved glycated hemoglobin by at least 0.5% and/or de-escalation of medical treatment.
Both pre- and postoperative assays were performed in the same laboratory. Creatinine was determined through colorimetric enzymatic assay and glomerular filtration rate was estimated (eGFR) using the CKD Epidemiology Collaboration (CKD-EPI) 2021 serum creatinine-based formula, adjusted for standard body surface area of 1.73 m2 (23). Classes of renal function were defined as: hyperfiltration (≥ 125), normal function (90-124), mild dysfunction (60-89), mild-to-moderate dysfunction (45-59 ml/min/1.73 m2), in accordance with KDIGO guidelines and commonly employed hyperfiltration cut-off values (24–26). Renal function was also estimated using the formula proposed by Basolo and colleagues (eGFR = 53 + 0.7 × (140 − Age) × Weight/(96xSCr) × (0.85 if female)), aimed at characterizing hyperfiltration (27).
2.2 Scan procedure—dual-energy x-ray absorptiometry
Whole body DXA scans using Lunar iDXA Forma (GE Healthcare) were performed to estimate total and regional body composition. Visceral adipose tissue (VAT) mass was quantified using the CoreScan™ application. Appendicular skeletal mass index (ASMI) was calculated as the sum of upper and lower extremities lean mass expressed in kilograms over the square of height expressed in meters. Quality assurance and control procedures were carried out in accordance with manufacturer indications. All scans were performed by an ISCD-certified DXA technologist, using the same software for analysis.
2.3 Statistical analysis
Statistical analysis was performed using IBM®SPSS® v. 26.0. Parameter variations were defined as value6 months – valuebaseline. Qualitative data was reported as frequency (percentage). Quantitative data was assessed with Komologorov-Smirnov test and normally distributed data was reported as mean ± standard deviation (SD), while parameters with non-normal distribution were described by median and inter-quartile range (IQR). Subsequently, independent and paired t Student tests were performed to compare normally distributed parameters, while Mann-Whitney U and Wilcoxon Signed Rank test were employed for non-normally distributed data. Bivariate correlations were explored using Pearson’s r and Spearman’s rho, respectively. Binary and linear adjusted regression models were employed to further explore the predictive value of parameters. Confounders were included as covariates in binary and multinominal regression, in order to validate the results. All analyses were 2-tailed, with a cut-off p value of less than 0.05 for statistical significance.
3 Results
The study included a total of 196 patients (80 males and 116 females), with ages ranging from 19 to 68 and a mean of 41.43 ± 10.79, slightly lower in males versus females (p=.08). The diagnosis of obesity had been established for an average of 18.21 ± 10.51 years, similar between the two genders. Median baseline BMI was 42.6 (6.61) kg/m2 and 6 months EWL reached 71.43 ± 17.18%, with no inter-gender differences.
T2DM and HTN baseline prevalences were 18.9% and 49.5%, with 6 months de-escalation of treatment reported in 51.3% and 43.2% of patients, respectively. Fasting plasma glucose, as well as total cholesterol, VLDL-cholesterol and triglycerides levels showed significant decline at 6 months.
Males tended to demonstrate greater 6 months percentual variations of body composition compartments - with the exception of total lean mass variation, which was similar between the two genders. Table 1 summarizes the evolution of aforementioned parameters in males and females respectively.
Analysis of gender-specific determinants of renal function revealed a number of differences. Specifically, in females, eGFR correlated negatively with VAT mass (rho=-.368, p<.001), uric acid (rho=-.396, p<.001), glycemia (rho=-.419, p<.001) and VLDL-cholesterol (rho=-.239, p=.001). eGFR-VAT mass correlation was present irrespective of T2DM status, but was stronger in females with T2DM (rho=-.529, p=0.02). None of these results were replicable in males. Moreover, when dividing females according to menopause status (75% premenopausal, 25% postmenopausal), all of the correlations were lost in postmenopausal state.
To further explore the relationship between renal filtration and visceral adiposity, we compared renal function across VAT mass tertiles in females. Women in the third VAT mass tertile were 5 times more likely to have eGFR<90ml/min/1.73m2 compared to the first tertile (OR=5.2, 95%CI = [1.29,20.99], p=0.02), in a model adjusted for BMI, number of years living with obesity, ASMI, T2DM and smoking status. However, the model lost significance when also accounting for menopausal state. It should be noted that patients demonstrating hyperfiltration were excluded from this analysis in order to ensure adequate comparison.
Stratification of baseline eGFR by standard classes of renal function revealed a very small prevalence of hyperfiltration in our group (1%), with the majority of patients demonstrating mild renal dysfunction (54.6%), followed by normal function (40.3%) and mild-to-moderate dysfunction (4.1%). Postoperatively, renal filtration improved across all defined classes, as depicted in Figure 1. Specific variations (expressed ml/min/1.73m2) were as follows: an increase from 55.01 (8.4) to 68.63 (20.97) in mild-to-moderate dysfunction class and from 79.35 (11.26) to 88.06 (19.64) in mild dysfunction class patients. In normal function class, baseline and 6 months eGFR values were 98.88 (13.75) and 106.92 (18.87), respectively (p=0.09). Finally, in the two cases exhibiting renal hyperfiltration per standard criteria, eGFR decreased postoperatively. The alternative approach of Basolo et al. (27) for evaluating hyperfiltration in patients with obesity determined the reclassification of 151 patients (77%) as exhibiting hyperfiltration and showed a significant postoperative reduction in their eGFR (145.1 (25.08) vs. 120.64 (28.55), p<.001) (Figure 2). We also considered a surrogate marker, eGFR/ASMI (aimed at controlling for postoperative muscle mass loss) and confirmed a similar pattern.
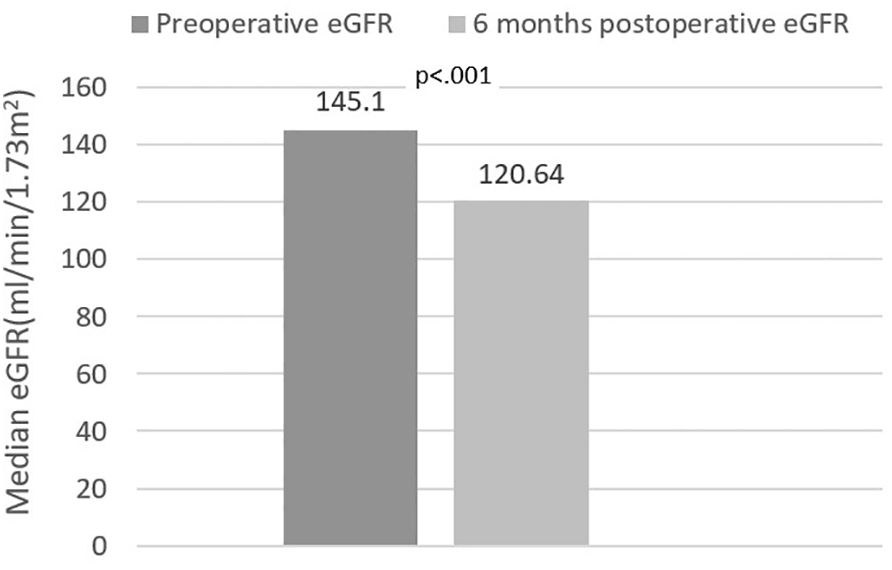
Figure 2. Estimated glomerular filtration rate (eGFR) variation in hyperfiltration class [eGFR formula - Basolo et al. (27)].
Finally, we analyzed correlates of renal function variation in all patients exhibiting either increase or decrease of eGFR. Inter-gender differences were evident once more. In males, eGFR variation correlated (p<.001 for all) with EWL (rho=.358) (Figure 3), lean mass (rho=-.412) and ASMI (rho=-.374) variations; in females, on the other hand, eGFR variation only correlated (p=0.01) with VAT mass (rho=-.266) (Figure 4) and LDL (rho=-.279) variations. VAT mass variation was an independent predictor (β=-.246, p=.02) of eGFR variation in females in a model adjusted for baseline eGFR, menopause status, EWL, ASMI variation, LDL variation and additionally for the improvement of both T2DM and HTN (model data: R=.6, R2=.36, p<.001). Conversely, in males, EWL was an independent predictor of eGFR variation (β=.32, p=.01) in an equivalent model – adjusted for baseline eGFR, ASMI variation, VAT mass variation and for the improvement of T2DM and HTN (model data: R=.63, R2=.4, p<.001).
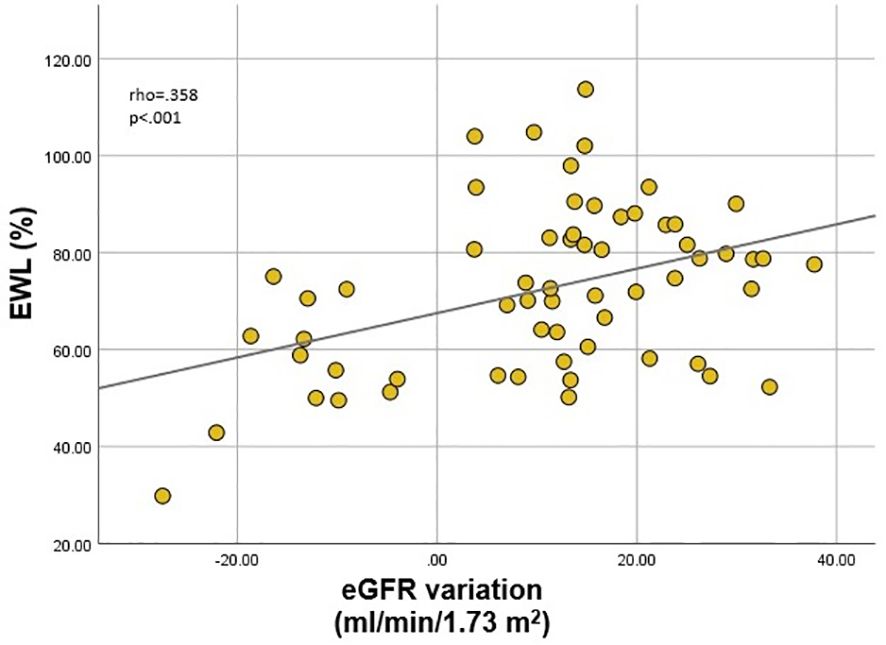
Figure 3. Correlation between estimated glomerular filtration rate (eGFR) variation and excess weight loss (EWL) in males.
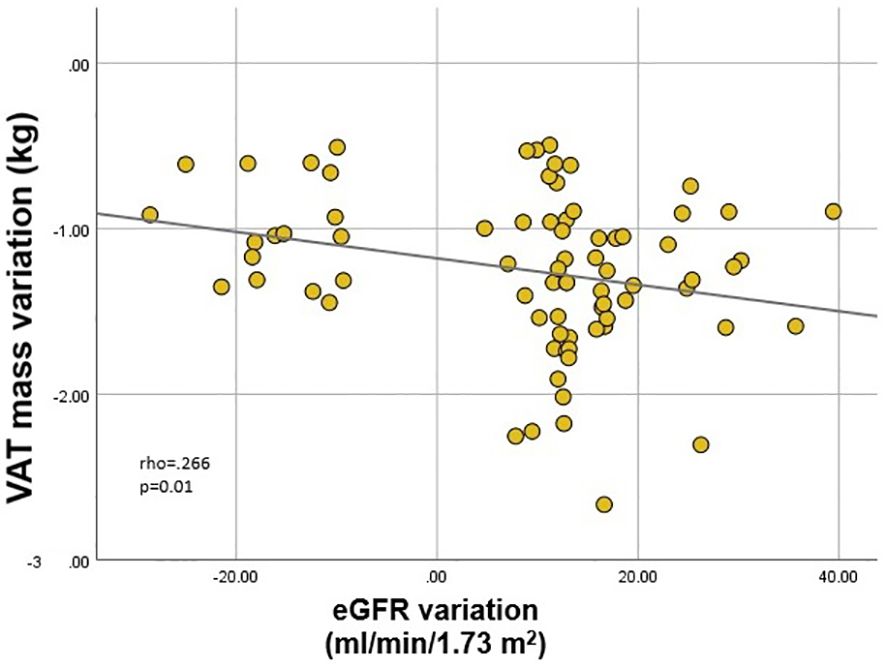
Figure 4. Correlation between estimated glomerular filtration rate (eGFR) variation and visceral adipose tissue (VAT) mass variation in females.
4 Discussion
In the present study we investigated the relationship between creatinine-based estimated glomerular filtration rate and DXA-evaluated body composition, as well as basic biochemical parameters in a cohort of patients with severe obesity undergoing LSG. Gender-oriented analysis identified interesting differences in the factors influencing renal function in males vs. females, both at baseline, as well as when considering early (6 months) postoperative variation.
Estimation of renal function by means of serum creatinine levels is currently the most widespread method used in clinical practice for both screening and monitoring and it also constitutes the basis of renal function staging (28). The CKD-EPI formula is believed to yield more accurate results and is, hence, favored by profile societies (23, 24, 28, 29). Its main limitations derive from the influence of muscle mass and dietary protein intake on serum creatinine levels (30), from the delayed increase in creatinine compared to the onset of renal function decline (31) and from the fact that the equations were developed on a population mainly in the normal BMI range (32). These lead to inaccuracies both in the general population with obesity, as well as in assessing the dynamics after MBS (31–35). Various means of resolving these shortcomings have been proposed in literature, with mostly unsatisfying results. The most popular is de-adjusting from standard body surface area (BSA) of 1.73m2 and adjusting for calculated BSA (de-indexed equations). However, this method consistently overestimates renal function, since muscle mass, and not total body mass is actually the main contributor to the estimation bias (36, 37). In this regard, Nankivell and colleagues demonstrated that ASMI constitutes the best correlate of eGFR error (34). Considering these results, we used the eGFR provided by CKD-EPI (2021) formula, adjusted for standard body surface area and corrected our analyses for ASMI and ASMI variation in order to provide accuracy. Through this, we were able to stratify patients in classes of renal function and assess their dynamics in compliance with guideline recommendations and in line with standard clinical practice. Renal dysfunction is reported to be more prevalent among persons with obesity (11, 38, 39) and our results are in line with previous findings, with the majority of patients exhibiting mild or mild-to-moderate dysfunction at baseline. An ample study, with an 8 million person-year follow-up, elegantly demonstrated that the risk of end-stage CKD progressively increases with the increase of BMI. Particularly, patients with severe obesity had a 7-fold increase in risk compared to normal weight controls (4). Additionally, in a more recent meta-analysis comprising a total of 3 million patients, obesity was associated with an almost two fold increase in the risk of renal function decline) over a 10 year follow-up (6). The largest epidemiologic study regarding CKD in the general Romanian population reported a cumulative prevalence of reduced kidney function (defined as eGFR<60ml/min/1.73m2) of 3.76% (40). Comparatively, in our cohort, mild-to-moderate renal dysfunction had a prevalence of 4.1%. However, the majority of our patients (56.4%) exhibited mild dysfunction, on which the aforementioned study did not report.
Postoperatively, we identified significant increase in eGFR in patients exhibiting subnormal renal function at baseline; this was paralleled by a statistically insignificant (p=.09) less ample eGFR increase in patients classified as having normal baseline renal function (Figure 1). Interestingly, the patients exhibiting hyperfiltration, demonstrated postoperative decrease of eGFR; however, the very small number of subjects pertaining to this category precludes these results from being statistically relevant. Based on the assumption that currently used formulas underestimate the prevalence of renal hyperfiltration (36, 41), Basolo and colleagues devised a new formula, specifically aimed at more accurately identifying hyperfiltration in patients with obesity (27). Using this approach, we reclassified the majority of the patients (77%) as exhibiting hyperfiltration and confirmed a significant postoperative decrease of eGFR in this class (Figure 2). However, the authors specifically excluded patients with renal dysfunction from their original analysis; therefore, while these results provide further confirmation of eGFR improvement across all renal function classes, re-thinking the stratification of all patients based on the proposed formula is likely to be inaccurate. These outcomes were also confirmed by the comparable variation of eGFR/ASMI [analogous to the procedure employed by Favre and colleagues who used eGFR over lean body mass in their study (42)]. There is currently a robust body of literature with similar results, pointing to the beneficial role of MBS-induced weight loss on renal function (16–18, 33, 43–45). Improvement of GFR and proteinuria are evident in the first months-to-years following surgery, but positive effects have been recorded up to 15 years postoperatively (43).
Obesity negatively impacts renal dynamics through a combination of direct and indirect mechanism; of the latter, the two most important are HTN and T2DM, both of which are widely recognized complications of obesity and also major risk factors for the development of kidney disease. However, direct pathogenic ways, occurring even in the absence of overt HTN/T2DM are believed to be of equal importance. These include hemodynamic alterations and increased sodium reabsorption (leading to initial hyperfiltration, followed by GFR decline), adipokine and cytokine dysregulation, insulin resistance, oxidative stress and direct lipotoxicity (3, 10–12). Visceral adipose tissue (VAT) is recognized as the metabolically active, pathogenic compartment, linked to aforementioned alterations (46), as opposed to subcutaneous adipose tissue (SAT), which serves as a buffer for excess energy and has positive metabolic impact (47, 48). Obesity-related adipose tissue dysfunction is characterized by chronic inflammation and M1-polarized macrophage infiltration and activation, all of which occur predominantly in VAT (47, 49). Kidney morphology and physiology (e.g. high vascularization, not paralleled by equally effective local anti-inflammatory guards) determine increased renal sensitivity towards inflammation-induced damage (50). Studies have tied various pro-inflammatory molecules (e.g. tumor necrosis factor-alpha (TNF-α), interleukins 1β and 6 (IL-1β, IL-6) etc.) to the onset of chronic kidney disease (CKD) (50). A landmark study including over 3000 patients revealed that higher circulating TNF-α and fibrinogen levels are associated with more rapid decline of eGFR in patients with established CKD (51). More recently, involvement of TNF-α in renal fibrosis, as well as the benefits of its inhibition have been described in murine aristolochic acid-induced nephropathy (considered superior to previously employed murine models) (52). Moriconi et al. aimed to assess the relationship between IL-1β/Caspase-1, insulin sensitivity and early-stage obesity-related renal damage, namely hyperfiltration. Apart from demonstrating the capacity of IL-1β to predict hyperfiltration in patients with severe obesity, their study had another valuable result – patients in whom GFR did not normalize after MBS also showed failure to normalize circulating IL-1β/Caspase-1 levels (53). These results suggest a pathogenic role of the inflammasome in kidney dysfunction, as well as the potential for achieving renoprotection through targeted anti-inflammatory therapy, in concert with weight reduction. Similar strategies are being considered in diabetic kidney disease, in which renal benefits of medication with potential anti-inflammatory effect (e.g. type 2 sodium-glucose cotransporter inhibitors, glucagon-like peptide-1 receptor agonists, renin-angiotensin-aldosterone system (RAAS) inhibitors) are evidently superior to those of medication that solely achieves tight glycemic control or reduction of arterial blood pressure (54).
Apart from the mechanisms detailed above, it is noteworthy that peri- and intrarenal accumulation of VAT also increases local pressure, activating sympathetic and RAA systems and directly contributes to glomerular lesions (55, 56). In line with these concepts, central adiposity (i.e. various estimates of VAT), perirenal adipose tissue and renal sinus fat exhibit a stronger correlation with the occurrence of obesity-related renal complications than BMI or subcutaneous adipose tissue (57). In a multicentric study using bioelectrical impedance analysis aimed to assess the ability of various obesity indicators to predict renal impairment (GFR decline/new-onset proteinuria) during a 6-year follow-up, only visceral fat area (with a cut-off of 100 cm2) proved to be a sensitive marker (58). Kataoka and colleagues found that VAT to SAT ratio serves as a predictor of eGFR decline in CKD patients, with a greater impact in females and in patients with lower absolute values of VAT (59). Interestingly, the relationship between VAT mass and CKD development seems to be stronger in patients with lower body weight. In a large scale cohort study (11000 subjects, 5.6-year follow-up), VAT was a predictor of CKD onset only in patients with normal BMI (60).
Accurate estimation of VAT mass has been a topic of great interest in the past years and surrogate markers such as lipid accumulation product (LAP), visceral adiposity index (VAI) (61), novel VAI (NVAI) (62), Chinese-population specific cVAI (63) and, more recently, the metabolic score for visceral fat (METS-VF) (64) have been widely used in studies of obesity-related renal affliction. However, DXA has the advantage of providing a direct and accurate assessment of body composition and the CoreScan™ application has been validated against MRI and CT, with satisfactory concordance (65).
In a meta-analysis including seven studies, Fang and colleagues concluded that VAI is a useful tool for predicting CKD (area under the curve 0.77, prediction rate of 73% when considering a 50% pre-test probability) (66). Another ample study revealed that both LAP and VAI are associated with increase odds of CKD incidence (61). Interestingly, in our study, the correlation between eGFR and VAT mass, along with various other markers of lipotoxicity and inflammation (uric acid, VLDL-cholesterol) was only apparent in females, and not in males. Moreover, when analyzing VAT mass tertiles, the odds of reduced eGFR (<90ml/min/1.73m2) was 5 times higher in women in the third VAT mass tertile compared to those in the first one, in a model adjusted for major confounders. This is in line with other reported findings. For example, in an ample community-based study in Taiwan, involving approximately 2000 subjects, VAI was significantly associated with the presence of CKD in females, but not in males (67). In another large study, comprising 5355 subjects, Xu and colleagues demonstrated a negative correlation between eGFR and CVAI and found CVAI to more accurately predict renal dysfunction in females compared to males (63). Similarly, in a study involving 400 middle-aged and elderly subjects, VAI was an independent predictor of CKD in females, but not in males (68). Another interesting study based on the KORA cohort, revealed a negative association between cystatin-based eGFR and MRI estimated VAT only in women. However, the authors found no correlation between creatinine-based eGFR and VAT (69). Similarly, in a study including approximately 35000 participants from the NHANES database, no association between VAI and eGFR could be established; nevertheless, visceral adiposity was associated with increased odds of chronic kidney disease and albuminuria in females (70).
In contrast, a number of studies reported negative correlation between VAT and renal function in males, rather than in females. For example, in a longitudinal study including almost 7000 non-diabetic patients, VAI was found to be an independent predictor of renal function decline only in men over a period of follow-up of 8.6 years (71). Similarly, in a cross-sectional study conducted by Seong et al. and including almost 5000 subjects, VAI and LAP correlated with CKD exclusively in males (72). Yu and colleagues found METS-VF to be an independent predictor of CKD, exhibiting superiority to other obesity indices in both sexes, but more prominently in men (73).
Males and females differ not only in body size, but also in body composition. Men tend to have higher BMIs, as well as a higher absolute value of VAT and VAT: SAT ratio when compared to premenopausal women (74, 75). Similarly, male and female kidneys show differences that go beyond size, namely in histology and physiology (76, 77). For example, inter-gender variability in the abundance and functionality of renal transporters has been described (77), explaining differences in water and solute handling (e.g. a more rapid and efficient natriuretic response to high salt diet in females (78)). These provide a basis for the discrepancies in CKD evolution (known to be slower in premenopausal women than in men (77)) on one hand, and might explain the gender-specific renal-VAT interaction, on the other. Gonadal hormones are likely to have the greatest impact on these dissimilarities, influencing both body composition parameters and renal function directly. Estrogen promotes expansion of SAT, rather than VAT, reduces RAAS activation, promotes nitric oxide- mediated vasodilation and attenuates inflammation (75, 79). Of note, three types of renal estrogen receptors have been described and estrogen is believed to also exert its direct renoprotective actions, of which we mention attenuation of glomerular hypertrophy, reduction of mesangial expansion and decrease of fibrosis, mainly via ERα (80–83).
Menopause brings about a reversal of the female “advantage” in adiposity polarization and CKD progression, further supporting the central protective role of estrogen (81). The global consequences of estrogen decline (e.g. inflammatory milieu alterations (84)) further enhance the negative renal impact. In line with these findings, in our study, menopause status influenced all preoperative correlates of renal function. An ample prospective study, with a 15-year follow-up, interestingly showed that women with low estimated endogenous estrogen exposure had a higher risk of developing CKD later in life (79), while, in a nationwide Korean study, the use of menopausal hormone therapy was associated with a reduced risk of end-stage renal disease (83). However, in the context of obesity, conflicting results have been reported, with a number of deleterious effects especially in female subjects (85–87). Moreover, the dysregulation of systems involved in estrogen-mediated renal protection (e.g. RAAS) (88) might render estrogen less effective in the context of obesity (75). In their studies on murine models, Rodríguez-Rodríguez and colleagues elegantly assessed the combined renal effects of obesity and menopause. Ovariectomized obese mice had the most detrimental metabolic and renal responses to obesogenic diet and also exhibited the most severe lipotoxic and inflammatory profile in renal tissue (81, 91). These results suggest concerted deleterious renal effects of obesity and menopause and highlight the importance of weight control in menopause and perimenopausal transition as a renoprotective measure.
Another noteworthy result of our research is the stronger eGFR-VAT mass correlation in female patients with obesity and T2DM versus female patients with obesity, but no T2DM diagnosis. A very recent study by Liu and colleagues using DXA scans to quantify visceral adiposity in patients with T2DM, revealed additive interaction between diabetes and visceral adiposity on the occurrence of albuminuria. Higher visceral adiposity was also found to induce a stronger correlation between T2DM and albuminuria (89).
There are multiple hypotheses regarding post-MBS renal function improvement, derived from the positive impact on all aforementioned obesity-related dysregulations. Most of the studies found that improvement of renal filtration does not correlate with the degree of weight loss, but rather with the amelioration of metabolic profile and comorbidities, as a consequence of VAT and ectopic adipose tissue reduction (35, 43, 44, 90). However, we revealed a positive correlation between eGFR variation and EWL in male patients with obesity, paralleled by negative correlations with lean mass and ASMI reductions (which may be considered logical in light of the influence on creatinine). These results were not replicated in females. Instead, similar to the baseline strong influence of visceral adiposity, VAT mass variation proved to be an independent predictor of eGFR variation in a model adjusted for the main confounders. Interestingly, this held significance regardless of menopause status, suggesting beneficial effects of VAT mass reduction in both states.
To our knowledge, no other studies have reported on the correlation between DXA-estimated VAT and GFR dynamics before and after MBS, neither globally, nor through gender-oriented analysis. The availability of DXA also provides an additional strength of our study, namely the possibility to assess and adjust for the influence of ASMI. The main limitations stem from the inherent issues with creatinine-based eGFR, as discussed above. Although ideal, direct measurement or GFR is unfeasible in clinical practice. However, estimating GFR via equations based on both creatinine and cystatin C might increase reliability (31, 32). Secondly, albuminuria was not assessed in our cohort and therefore we could solely analyze renal function, rather than chronic kidney disease. Finally, while benefiting from a fairly ample evaluation, there are still confounders that should be considered when interpreting these results, such as dietary intake, physical activity levels, and socioeconomic status, which may influence both obesity (along with body composition parameters) and renal function (including the estimation of GFR).
The present study concentrated on the early impact of MBS on renal function. Added value would be provided by long-term assessment. However, the most significant improvement in GFR seems to occur in the early postoperative timeframe (6-12 months), as pointed out by a meta-analysis by Huang et al. (17). Finally, our results should be confirmed on larger and more diverse cohorts, since we included a fairly low number of patients pertaining to the same ethnic group.
5 Conclusion
In the current study, we demonstrated a positive impact of MBS on obesity-related renal dysfunction and outlined gender-specific determinants of baseline eGFR and of postoperative eGFR variation. Our results suggest a stronger impact of visceral adiposity and lipotoxicity on renal pathology in females with obesity. This warrants further exploration, as it may guide future personalized therapeutic approaches.
Data availability statement
The raw data supporting the conclusions of this article will be made available by the authors, without undue reservation.
Ethics statement
The studies involving humans were approved by Carol Davila University Ethics Committee. The studies were conducted in accordance with the local legislation and institutional requirements. The participants provided their written informed consent to participate in this study.
Author contributions
MP: Writing – review & editing, Writing – original draft, Visualization, Software, Resources, Methodology, Investigation, Formal analysis, Data curation, Conceptualization. AS: Writing – review & editing, Writing – original draft, Visualization, Validation, Supervision, Formal analysis, Conceptualization. EM: Writing – review & editing, Writing – original draft, Validation, Resources, Methodology, Investigation, Formal analysis, Data curation. CC: Writing – review & editing, Writing – original draft, Resources, Conceptualization. SF: Writing – review & editing, Writing – original draft, Visualization, Validation, Supervision, Resources, Conceptualization.
Funding
The author(s) declare that no financial support was received for the research, authorship, and/or publication of this article.
Acknowledgments
Publication of this paper was supported by the University of Medicine and Pharmacy Carol Davila, through the institutional program Publish not Perish.
Conflict of interest
The authors declare that the research was conducted in the absence of any commercial or financial relationships that could be construed as a potential conflict of interest.
Publisher’s note
All claims expressed in this article are solely those of the authors and do not necessarily represent those of their affiliated organizations, or those of the publisher, the editors and the reviewers. Any product that may be evaluated in this article, or claim that may be made by its manufacturer, is not guaranteed or endorsed by the publisher.
References
1. World Obesity Federation. World Obesity Atlas. London: World Obesity Federation (2024). Available at: https://data.worldobesity.org/publications/?cat=22.
2. Bello AK, Okpechi IG, Levin A, Ye F, Damster S, Arruebo S, et al. An update on the global disparities in kidney disease burden and care across world countries and regions. Lancet Glob Heal. (2024) 12:e382–95. doi: 10.1016/S2214-109X(23)00570-3
3. Tsuboi N, Okabayashi Y. The renal pathology of obesity: structure-function correlations. Semin Nephrol. (2021) 41:296–306. doi: 10.1016/j.semnephrol.2021.06.002
4. Hsu Cy, McCulloch CE, Iribarren C, Darbinian J, Go AS. Body mass index and risk for end-stage renal disease. Ann Intern Med. (2006) 144:21–8. doi: 10.7326/0003-4819-144-1-200601030-00006
5. Garofalo C, Borrelli S, Minutolo R, Chiodini P, De Nicola L, Conte G. A systematic review and meta-analysis suggests obesity predicts onset of chronic kidney disease in the general population. Kidney Int. (2017) 91:1224–35. doi: 10.1016/j.kint.2016.12.013
6. Pinto KRD, Feckinghaus CM, Hirakata VN. Obesity as a predictive factor for chronic kidney disease in adults: systematic review and meta-analysis. Braz J Med Biol Res = Rev Bras Pesqui medicas e Biol. (2021) 54:e10022. doi: 10.1590/1414-431X202010022
7. Wang S, Qin A, Dong L, Tan J, Zhou X, Qin W. Association of obesity with the development of end stage renal disease in IgA nephropathy patients. Front Endocrinol (Lausanne). (2023) 14:1094534. doi: 10.3389/fendo.2023.1094534
8. Munkhaugen J, Lydersen S, Widerøe TE, Hallan S. Prehypertension, obesity, and risk of kidney disease: 20-year follow-up of the HUNT I study in Norway. Am J Kidney Dis Off J Natl Kidney Found. (2009) 54:638–46. doi: 10.1053/j.ajkd.2009.03.023
9. Mohammedi K, Chalmers J, Herrington W, Li Q, Mancia G, Marre M, et al. Associations between body mass index and the risk of renal events in patients with type 2 diabetes. Nutr Diabetes. (2018) 8:7. doi: 10.1038/s41387-017-0012-y
10. Zhu Q, Scherer PE. Immunologic and endocrine functions of adipose tissue: implications for kidney disease. Nat Rev Nephrol. (2018) 14:105–20. doi: 10.1038/nrneph.2017.157
11. Nawaz S, Chinnadurai R, Al-Chalabi S, Evans P, Kalra PA, Syed AA, et al. Obesity and chronic kidney disease: A current review. Obes Sci Pract. (2023) 9:61–74. doi: 10.1002/osp4.629
12. Lakkis JI, Weir MR. Obesity and kidney disease. Prog Cardiovasc Dis. (2018) 61:157–67. doi: 10.1016/j.pcad.2018.07.005
13. Kambham N, Markowitz GS, Valeri AM, Lin J, D’Agati VD. Obesity-related glomerulopathy: an emerging epidemic. Kidney Int. (2001) 59:1498–509. doi: 10.1046/j.1523-1755.2001.0590041498.x
14. Camhi SM, Bray GA, Bouchard C, Greenway FL, Johnson WD, Newton RL, et al. The relationship of waist circumference and BMI to visceral, subcutaneous, and total body fat: sex and race differences. Obesity. (2011) 19:402–8. doi: 10.1038/oby.2010.248
15. Liao J, Yin Y, Zhong J, Chen Y, Chen Y, Wen Y, et al. Bariatric surgery and health outcomes: An umbrella analysis. Front Endocrinol (Lausanne). (2022) 13:1016613. doi: 10.3389/fendo.2022.1016613
16. Friedman AN, Cohen RV. Bariatric surgery as a renoprotective intervention. Curr Opin Nephrol Hypertens. (2019) 28:537–44. doi: 10.1097/MNH.0000000000000539
17. Huang H, Lu J, Dai X, Li Z, Zhu L, Zhu S, et al. Improvement of renal function after bariatric surgery: a systematic review and meta-analysis. Obes Surg. (2021) 31:4470–84. doi: 10.1007/s11695-021-05630-4
18. Moriconi D, Nannipieri M, Dadson P, Rosada J, Tentolouris N, Rebelos E. The beneficial effects of bariatric-surgery-induced weight loss on renal function. Metabolites. (2022) 12:967. doi: 10.3390/metabo12100967
19. Eisenberg D, Shikora SA, Aarts E, Aminian A, Angrisani L, Cohen RV, et al. 2022 American society of metabolic and bariatric surgery (ASMBS) and international federation for the surgery of obesity and metabolic disorders (IFSO) indications for metabolic and bariatric surgery. Obes Surg. (2023) 33:3–14. doi: 10.1007/s11695-022-06332-1
20. National Institute on Alcohol Abuse and Alcoholism. Drinking levels defined . Available online at: https://www.niaaa.nih.gov/alcohol-health/overview-alcohol-consumption/moderate-binge-drinking. (Accessed March 26, 2024).
21. Montero PN, Stefanidis D, Norton HJ, Gersin K, Kuwada T. Reported excess weight loss after bariatric surgery could vary significantly depending on calculation method: a plea for standardization. Surg Obes Relat Dis Off J Am Soc Bariatr Surg. (2011) 7:531–4. doi: 10.1016/j.soard.2010.09.025
22. American Diabetes Association Professional Practice Committee. 2. Diagnosis and classification of diabetes: standards of care in diabetes—2024. Diabetes Care. (2023) 47:S20–42. doi: 10.2337/dc24-S002
23. Inker LA, Eneanya ND, Coresh J, Tighiouart H, Wang D, Sang Y, et al. New creatinine- and cystatin C–based equations to estimate GFR without race. N Engl J Med. (2021) 385:1737–49. doi: 10.1056/nejmoa2102953
24. Levin A, Stevens PE, Bilous RW, Coresh J, De Francisco ALM, De Jong PE, et al. KDIGO 2012 clinical practice guideline for the evaluation and management of chronic kidney disease: Chapter 1: Definition and classification of CKD. Kidney Int Suppl. (2013) 3:19–62. doi: 10.1038/KISUP.2012.64
25. Cachat F, Combescure C, Cauderay M, Girardin E, Chehade H. A systematic review of glomerular hyperfiltration assessment and definition in the medical literature. Clin J Am Soc Nephrol. (2015) 10:382–9. doi: 10.2215/CJN.03080314
26. Helal I, Fick-Brosnahan GM, Reed-Gitomer B, Schrier RW. Glomerular hyperfiltration: Definitions, mechanisms and clinical implications. Nat Rev Nephrol. (2012) 8:293–300. doi: 10.1038/nrneph.2012.19
27. Basolo A, Salvetti G, Giannese D, Genzano SB, Ceccarini G, Giannini R, et al. Obesity, hyperfiltration, and early kidney damage: A new formula for the estimation of creatinine clearance. J Clin Endocrinol Metab. (2023) 108:3280–6. doi: 10.1210/clinem/dgad330
28. Levey AS, Coresh J, Tighiouart H, Greene T, Inker LA. Measured and estimated glomerular filtration rate: current status and future directions. Nat Rev Nephrol. (2020) 16:51–64. doi: 10.1038/s41581-019-0191-y
29. Lemoine S, Guebre-Egziabher F, Sens F, Nguyen-Tu MS, Juillard L, Dubourg L, et al. Accuracy of GFR estimation in obese patients. Clin J Am Soc Nephrol. (2014) 9:720–7. doi: 10.2215/CJN.03610413
30. Kashani K, Rosner MH, Ostermann M. Creatinine: From physiology to clinical application. Eur J Intern Med. (2020) 72:9–14. doi: 10.1016/j.ejim.2019.10.025
31. Zou LX, Sun L, Nicholas SB, Lu Y, SS K, Hua R. Comparison of bias and accuracy using cystatin C and creatinine in CKD-EPI equations for GFR estimation. Eur J Intern Med. (2020) 80:29–34. doi: 10.1016/j.ejim.2020.04.044
32. Friedman AN, Moe S, Fadel WF, Inman M, Mattar SG, Shihabi Z, et al. Predicting the glomerular filtration rate in bariatric su. Am J Nephrol. (2014) 39:8–15. doi: 10.1159/000357231
33. Clerte M, Wagner S, Carette C, Brodin-Sartorius A, Vilaine É, Alvarez JC, et al. The measured glomerular filtration rate (mGFR) before and 6 months after bariatric surgery: A pilot study. Nephrol Ther. (2017) 13:160–7. doi: 10.1016/j.nephro.2016.10.002
34. Nankivell BJ, Nankivell LFJ, Elder GJ, Gruenewald SM. How unmeasured muscle mass affects estimated GFR and diagnostic inaccuracy. EClinicalMedicine. (2020) 29–30:1–9. doi: 10.1016/j.eclinm.2020.100662
35. Rothberg AE, McEwen LN, Herman WH. Severe obesity and the impact of medical weight loss on estimated glomerular filtration rate. PloS One. (2020) 15:e0228984. doi: 10.1371/journal.pone.0228984
36. Delanaye P, Krzesinski JM. Indexing of renal function parameters by body surface area: intelligence or folly? Nephron Clin Pract. (2011) 119:c289–92. doi: 10.1159/000330276
37. Donadio C, Moriconi D, Berta R, Anselmino M. Estimation of urinary creatinine excretion and prediction of renal function in morbidly obese patients: new tools from body composition analysis. Kidney Blood Press Res. (2017) 42:629–40. doi: 10.1159/000481630
38. Hojs R, Ekart R, Bevc S, Vodošek Hojs N. Chronic kidney disease and obesity. Nephron. (2023) 147:660–4. doi: 10.1159/000531379
39. Sharma I, Liao Y, Zheng X, Kanwar YS. New pandemic: obesity and associated nephropathy. Front Med. (2021) 8:673556. doi: 10.3389/fmed.2021.673556
40. Moţa E, Popa SG, Moţa M, Mitrea A, Penescu M, Tuţă L, et al. Prevalence of chronic kidney disease and its association with cardio-metabolic risk factors in the adult Romanian population: the PREDATORR study. Int Urol Nephrol. (2015) 47:1831–8. doi: 10.1007/s11255-015-1109-7
41. Chang AR, Zafar W, Grams ME. Kidney function in obesity-challenges in indexing and estimation. Adv Chronic Kidney Dis. (2018) 25:31–40. doi: 10.1053/j.ackd.2017.10.007
42. Favre G, Anty R, Canivet C, Clément G, Ben-Amor I, Tran A, et al. Determinants associated with the correction of glomerular hyper-filtration one year after bariatric surgery. Surg Obes Relat Dis. (2017) 13:1760–6. doi: 10.1016/j.soard.2017.07.018
43. Shulman A, Andersson-Assarsson JC, Sjöström CD, Jacobson P, Taube M, Sjöholm K, et al. Remission and progression of pre-existing micro- and macroalbuminuria over 15 years after bariatric surgery in Swedish Obese Subjects study. Int J Obes. (2021) 45:535–46. doi: 10.1038/s41366-020-00707-z
44. Bilha SC, Nistor I, Nedelcu A, Kanbay M, Scripcariu V, Timofte D, et al. The effects of bariatric surgery on renal outcomes: a systematic review and meta-analysis. Obes Surg. (2018) 28:3815–33. doi: 10.1007/s11695-018-3416-4
45. Friedman AN, Wahed AS, Wang J, Courcoulas AP, Dakin G, Hinojosa MW, et al. Effect of bariatric surgery on CKD risk. J Am Soc Nephrol. (2018) 29:1289–300. doi: 10.1681/ASN.2017060707
46. Stefan N. Causes, consequences, and treatment of metabolically unhealthy fat distribution. Lancet Diabetes Endocrinol. (2020) 8:616–27. doi: 10.1016/S2213-8587(20)30110-8
47. Chait A, den Hartigh LJ. Adipose tissue distribution, inflammation and its metabolic consequences, including diabetes and cardiovascular disease. Front Cardiovasc Med. (2020) 7:22. doi: 10.3389/fcvm.2020.00022
48. Vela-Bernal S, Facchetti R, Dell’Oro R, Quarti-Trevano F, Lurbe E, Mancia G, et al. Anthropometric measures of adiposity as markers of kidney dysfunction: A cross-sectional study. High Blood Press Cardiovasc Prev. (2023) 30:467–74. doi: 10.1007/s40292-023-00600-6
49. Arabi T, Shafqat A, Sabbah BN, Fawzy NA, Shah H, Abdulkader H, et al. Obesity-related kidney disease: Beyond hypertension and insulin-resistance. Front Endocrinol (Lausanne). (2022) 13:1095211. doi: 10.3389/fendo.2022.1095211
50. Kadatane SP, Satariano M, Massey M, Mongan K, Raina R. The role of inflammation in CKD. Cells. (2023) 12:1581. doi: 10.3390/cells12121581
51. Amdur RL, Feldman HI, Gupta J, Yang W, Kanetsky P, Shlipak M, et al. Inflammation and progression of CKD: the CRIC study. Clin J Am Soc Nephrol. (2016) 11:1546–56. doi: 10.2215/CJN.13121215
52. Taguchi S, Azushima K, Yamaji T, Urate S, Suzuki T, Abe E, et al. Effects of tumor necrosis factor-α inhibition on kidney fibrosis and inflammation in a mouse model of aristolochic acid nephropathy. Sci Rep. (2021) 11:23587. doi: 10.1038/s41598-021-02864-1
53. Moriconi D, Antonioli L, Masi S, Bellini R, Pellegrini C, Rebelos E, et al. Glomerular hyperfiltration in morbid obesity: Role of the inflammasome signalling. Nephrology. (2022) 27:673–80. doi: 10.1111/nep.14077
54. Donate-Correa J, Ferri CM, Sánchez-Quintana F, Pérez-Castro A, González-Luis A, Martín-Núñez E, et al. Inflammatory cytokines in diabetic kidney disease: pathophysiologic and therapeutic implications. Front Med. (2020) 7:628289. doi: 10.3389/fmed.2020.628289
55. Grigoraș A, Balan RA, Căruntu ID, Giușcă SE, Lozneanu L, Avadanei RE, et al. Perirenal adipose tissue-current knowledge and future opportunities. J Clin Med. (2021) 10:1291. doi: 10.3390/jcm10061291
56. Spit KA, Muskiet MHA, Tonneijck L, Smits MM, Kramer MHH, Joles JA, et al. Renal sinus fat and renal hemodynamics: a cross-sectional analysis. MAGMA. (2020) 33:73–80. doi: 10.1007/s10334-019-00773-z
57. Xu S, Ma J, Zheng Y, Ren R, Li W, Zhao W, et al. Para-perirenal fat thickness is associated with reduced glomerular filtration rate regardless of other obesity-related indicators in patients with type 2 diabetes mellitus. PloS One. (2023) 18:1–15. doi: 10.1371/journal.pone.0293464
58. Miyasato Y, Oba K, Yasuno S, Matsuyama Y, Masuda I. Associations between visceral obesity and renal impairment in health checkup participants: a retrospective cohort study. Clin Exp Nephrol. (2020) 24:935–45. doi: 10.1007/s10157-020-01921-9
59. Kataoka H, Mochizuki T, Iwadoh K, Ushio Y, Kawachi K, Watanabe S, et al. Visceral to subcutaneous fat ratio as an indicator of a ≥30% eGFR decline in chronic kidney disease. PloS One. (2020) 15:e0241626. doi: 10.1371/journal.pone.0241626
60. Lee J, Min S, Oh SW, Oh S, Lee YH, Kwon H, et al. Association of intraabdominal fat with the risk of incident chronic kidney disease according to body mass index among Korean adults. PloS One. (2023) 18:1–14. doi: 10.1371/journal.pone.0280766
61. Bullen AL, Katz R, Kumar U, Gutierrez OM, Sarnak MJ, Kramer HJ, et al. Lipid accumulation product, visceral adiposity index and risk of chronic kidney disease. BMC Nephrol. (2022) 23:1–11. doi: 10.1186/s12882-022-03026-9
62. Sun Y, Yan Y, Liao Y, Chu C, Guo T, Ma Q, et al. The new visceral adiposity index outperforms traditional obesity indices as a predictor of subclinical renal damage in Chinese individuals: a cross-sectional study. BMC Endocr Disord. (2023) 23:78. doi: 10.1186/s12902-023-01330-5
63. Xu Y, Wang XY, Liu H, Jin D, Song X, Wang S, et al. A novel clinical diagnostic marker predicting the relationship between visceral adiposity and renal function evaluated by estimated glomerular filtration rate (eGFR) in the Chinese physical examination population. Lipids Health Dis. (2023) 22:1–11. doi: 10.1186/s12944-023-01783-6
64. Bello-Chavolla OY, Antonio-Villa NE, Vargas-Vázquez A, Viveros-Ruiz TL, Almeda-Valdes P, Gomez-Velasco D, et al. Metabolic Score for Visceral Fat (METS-VF), a novel estimator of intra-abdominal fat content and cardio-metabolic health. Clin Nutr. (2020) 39:1613–21. doi: 10.1016/j.clnu.2019.07.012
65. Kaul S, Rothney MP, Peters DM, Wacker WK, Davis CE, Shapiro MD, et al. Dual-energy X-ray absorptiometry for quantification of visceral fat. Obesity. (2012) 20:1313–8. doi: 10.1038/oby.2011.393
66. Fang T, Zhang Q, Wang Y, Zha H. Diagnostic value of visceral adiposity index in chronic kidney disease: a meta-analysis. Acta Diabetol. (2023) 60:739–48. doi: 10.1007/s00592-023-02048-5
67. Huang J, Zhou C, Li Y, Zhu S, Liu A, Shao X, et al. Visceral adiposity index, hypertriglyceridemic waist phenotype and chronic kidney disease in a southern Chinese population: a cross-sectional study. Int Urol Nephrol. (2015) 47:1387–96. doi: 10.1007/s11255-015-1040-y
68. Chen IJ, Hsu LT, Lu MC, Chen YJ, Tsou MT, Chen JY. Gender differences in the association between obesity indices and chronic kidney disease among middle-aged and elderly Taiwanese population: A community-based cross-sectional study. Front Endocrinol (Lausanne). (2021) 12:737586. doi: 10.3389/fendo.2021.737586
69. Mueller-Peltzer K, von Krüchten R, Lorbeer R, Rospleszcz S, Schulz H, Peters A, et al. Adipose tissue is associated with kidney function parameters. Sci Rep. (2023) 13:1–8. doi: 10.1038/s41598-023-36390-z
70. Qin Z, Chen X, Sun J, Jiang L. The association between visceral adiposity index and decreased renal function: A population-based study. Front Nutr. (2023) 10:1076301. doi: 10.3389/fnut.2023.1076301
71. Mousapour P, Barzin M, Valizadeh M, Mahdavi M, Azizi F, Hosseinpanah F. Predictive performance of lipid accumulation product and visceral adiposity index for renal function decline in non-diabetic adults, an 8.6-year follow-up. Clin Exp Nephrol. (2020) 24:225–34. doi: 10.1007/s10157-019-01813-7
72. Seong JM, Lee JH, Gi MY, Son YH, Moon AE, Park CE, et al. Gender difference in the association of chronic kidney disease with visceral adiposity index and lipid accumulation product index in Korean adults: Korean National Health and Nutrition Examination Survey. Int Urol Nephrol. (2021) 53:1417–25. doi: 10.1007/s11255-020-02735-0
73. Yu P, Meng X, Kan R, Wang Z, Yu X. Association between metabolic scores for visceral fat and chronic kidney disease: A cross-sectional study. Front Endocrinol (Lausanne). (2022) 13:1052736. doi: 10.3389/fendo.2022.1052736
74. Al-Chalabi S, Syed AA, Kalra PA, Sinha S. Mechanistic links between central obesity and cardiorenal metabolic diseases. Cardiorenal Med. (2024) 14:12–22. doi: 10.1159/000535772
75. Kataoka H, Nitta K, Hoshino J. Visceral fat and attribute-based medicine in chronic kidney disease. Front Endocrinol (Lausanne). (2023) 14:1097596. doi: 10.3389/fendo.2023.1097596
76. Layton AT, Sullivan JC. Recent advances in sex differences in kidney function. Am J Physiol Renal Physiol United States. (2019) 316:F328–31. doi: 10.1152/ajprenal.00584.2018
77. McDonough AA, Harris AN, Xiong LI, Layton AT. Sex differences in renal transporters: assessment and functional consequences. Nat Rev Nephrol. (2024) 20:21–36. doi: 10.1038/s41581-023-00757-2
78. Veiras LC, Girardi ACC, Curry J, Pei L, Ralph DL, Tran A, et al. Sexual dimorphic pattern of renal transporters and electrolyte homeostasis. J Am Soc Nephrol. (2017) 28:3504–17. doi: 10.1681/ASN.2017030295
79. Farahmand M, Ramezani Tehrani F, Khalili D, Cheraghi L, Azizi F. Endogenous estrogen exposure and chronic kidney disease; a 15-year prospective cohort study. BMC Endocr Disord. (2021) 21:155. doi: 10.1186/s12902-021-00817-3
80. Ma HY, Chen S, Du Y. Estrogen and estrogen receptors in kidney diseases. Ren Fail. (2021) 43:619–42. doi: 10.1080/0886022X.2021.1901739
81. Afonso-Alí A, Porrini E, Teixido-Trujillo S, Pérez-Pérez JA, Luis-Lima S, Acosta-González NG, et al. The role of gender differences and menopause in obesity-related renal disease, renal inflammation and lipotoxicity. Int J Mol Sci. (2023) 24:12984. doi: 10.3390/ijms241612984
82. Singh AP, Singh M, Kaur T, Buttar HS, Ghuman SS, Pathak D. Estradiol Benzoate Ameliorates Obesity-Induced Renal Dysfunction in Male Rats: Biochemical and Morphological Observations BT - Biochemistry of Cardiovascular Dysfunction in Obesity. Tappia PS, Bhullar SK, Dhalla NS, editors. Cham: Springer International Publishing (2020) p. 367–84. doi: 10.1007/978-3-030-47336-5_19
83. Ahn SY, Choi YJ, Kim J, Ko GJ, Kwon YJ, Han K. The beneficial effects of menopausal hormone therapy on renal survival in postmenopausal Korean women from a nationwide health survey. Sci Rep. (2021) 11:15418. doi: 10.1038/s41598-021-93847-9
84. Opoku AA, Abushama M, Konje JC. Obesity and menopause. Best Pract Res Clin Obstet Gynaecol. (2023) 88:102348. doi: 10.1016/j.bpobgyn.2023.102348
85. Stevenson FT, Wheeldon CM, Gades MD, Kaysen GA, Stern JS, van Goor H. Estrogen worsens incipient hypertriglyceridemic glomerular injury in the obese Zucker rat. Kidney Int. (2000) 57:1927–35. doi: 10.1046/j.1523-1755.2000.00042.x
86. Sun L, Chao F, Luo B, Ye D, Zhao J, Zhang Q, et al. Impact of estrogen on the relationship between obesity and renal cell carcinoma risk in women. EBioMedicine. (2018) 34:108–12. doi: 10.1016/j.ebiom.2018.07.010
87. Gades MD, Stern JS, van Goor H, Nguyen D, Johnson PR, Kaysen GA. Estrogen accelerates the development of renal disease in female obese Zucker rats. Kidney Int. (1998) 53:130–5. doi: 10.1046/j.1523-1755.1998.00746.x
88. Vargas-Castillo A, Tobon-Cornejo S, Del Valle-Mondragon L, Torre-Villalvazo I, Schcolnik-Cabrera A, Guevara-Cruz M, et al. Angiotensin-(1-7) induces beige fat thermogenesis through the Mas receptor. Metabolism. (2020) 103:154048. doi: 10.1016/j.metabol.2019.154048
89. Liu Y, Zhao D, Chai S, Zhang X. Association of visceral adipose tissue with albuminuria and interaction between visceral adiposity and diabetes on albuminuria. Acta Diabetol. (2024) 61:909–16. doi: 10.1007/s00592-024-02271-8
90. Gong X, Zeng X, Fu P. The impact of weight loss on renal function in individuals with obesity and type 2 diabetes: a comprehensive review. Front Endocrinol (Lausanne). (2024) 15:1320627. doi: 10.3389/fendo.2024.1320627
91. Rodríguez-Rodríguez AE, Donate-Correa J, Luis-Lima S, Díaz-Martín L, Rodríguez-González C, Pérez-Pérez JA, et al. Obesity and metabolic syndrome induce hyperfiltration, glomerulomegaly, and albuminuria in obese ovariectomized female mice and obese male mice. Menopause (2021) 28(11):1296–306. doi: 10.1097/GME.0000000000001842
Keywords: obesity, renal dysfunction, metabolic and bariatric surgery, sleeve gastrectomy, visceral adiposity, body composition
Citation: Popa MM, Sirbu AE, Malinici EA, Copaescu C and Fica S (2024) Obesity-related renal dysfunction: gender-specific influence of visceral adiposity and early impact of metabolic and bariatric surgery. Front. Endocrinol. 15:1440250. doi: 10.3389/fendo.2024.1440250
Received: 29 May 2024; Accepted: 25 September 2024;
Published: 14 October 2024.
Edited by:
Yayun Wang, Air Force Medical University, ChinaReviewed by:
Giovanni Casella, Sapienza University of Rome, ItalyDiego Moriconi, University of Pisa, Italy
Copyright © 2024 Popa, Sirbu, Malinici, Copaescu and Fica. This is an open-access article distributed under the terms of the Creative Commons Attribution License (CC BY). The use, distribution or reproduction in other forums is permitted, provided the original author(s) and the copyright owner(s) are credited and that the original publication in this journal is cited, in accordance with accepted academic practice. No use, distribution or reproduction is permitted which does not comply with these terms.
*Correspondence: Anca Elena Sirbu, YW5jYS5zaXJidUB1bWZjZC5ybw==